Abstract
Water shortage threatens agricultural sustainability in the Huang-Huai-Hai Plain of China. Thus, we investigated the effect of supplemental irrigation (SI) on the root growth, soil water variation, and grain yield of winter wheat in this region by measuring the moisture content in different soil layers. Prior to SI, the soil water content (SWC) at given soil depths was monitored to calculate amount of irritation water that can rehydrate the soil to target SWC. The SWC before SI was monitored to depths of 20, 40, and 60 cm in treatments of W20, W40, and W60, respectively. Rainfed treatment with no irrigation as the control (W0). The mean root weight density (RWD), triphenyl tetrazolium chloride reduction activity (TTC reduction activity), soluble protein (SP) concentrations as well as catalase (CAT), and superoxide dismutase (SOD) activities in W40 and W60 treatments were significantly higher than those in W20. The RWD in 60–100 cm soil layers and the root activity, SP concentrations, CAT and SOD activities in 40–60 cm soil layers in W40 treatment were significantly higher than those in W20 and W60. W40 treatment is characterized by higher SWC in the upper soil layers but lower SWC in the 60–100-cm soil layers during grain filling. The soil water consumption (SWU) in the 60–100 cm soil layers from anthesis after SI to maturity was the highest in W40. The grain yield, water use efficiency (WUE), and irrigation water productivity were the highest in W40, with corresponding mean values of 9169 kg ha−1, 20.8 kg ha−1 mm−1, and 35.5 kg ha−1 mm−1. The RWD, root activities, SP concentrations, CAT and SOD activities, and SWU were strongly positively correlated with grain yield and WUE. Therefore, the optimum soil layer for SI of winter wheat after jointing is 0–40 cm.
Winter wheat (Triticum aestivum L.) production in the Huang-Huai-Hai Plain of China accounts for more than 60% of the entire wheat production in the country (National Bureau of Statistics of China, Citation2013); however, the production is threatened by severe water deficits. In this region, approximately 150–180 mm precipitation falls during the winter wheat growing season and more than 70% of irrigation water is required to maintain the high production of winter wheat (Liu et al., Citation2011; Zhang et al., Citation1999). In this region, traditionally, wheat crop is irrigated with up to 310 mm water and the water use efficiency (WUE) is approximately 13.2 kg ha−1 mm−1 (Sun et al., Citation2011; Zhang et al., Citation2011). Moreover, greater exploitation of groundwater use has caused the water table to recede at the rate of approximately 1 m per year for the last 20 years (Chen et al., Citation2009; Sun et al., Citation2006). To cope with these serious problems, supplemental irrigation (SI) techniques with limited water application at critical crop growth stages that substantially improve yield and WUE, should be urgently developed and adopted (Abourached et al., Citation2008; Garciay Garcia et al., Citation2009; Li et al., Citation2010; Oweis & Hachum, Citation2006; Wang et al., Citation2014).
The root system, an important organ that significantly influences crop growth and yield formation, functions in the absorption of soil moisture and nutrients (Kong et al., Citation2010; Monti & Zatta, Citation2009; Zhang et al., Citation2009). The soil water content (SWC) strongly affects the distribution of roots (Lv et al., Citation2010; Wang et al., Citation2004). Drought stress or waterlogging restricts root growth and reduces root density (Hayashi et al., Citation2013; Ma et al., Citation1999; Xue et al., Citation2003). Moderate SWC can increase root density, particularly in the deep soil layers, favors soil water absorption at depth and increases grain yield and WUE (Li et al., Citation2010; Qi et al., Citation2012; Zhang et al., Citation2009). Large variations in root growth are related to variations in SWC (Wang et al., Citation2006; Xue et al., Citation2003, Citation2010). Xue et al. (Citation2003) showed that the winter wheat root water uptake, grain yield, and WUE are significantly higher in irrigated crops than in rainfed crops during grain filling because of the higher root density in the irrigated crops. Zhang et al. (Citation2004) found the distribution of water uptake from the soil layers and the distribution of root length density to be equivalent; the scarcity of roots in the deep soil layers restricts soil water use and limits wheat production.
Irrigation regimes applied during the winter wheat growing season significantly affect the root density, depth, and activity (Chen et al., Citation2014; Kang et al., Citation2002; Li et al., Citation2010; Wang et al., Citation2014; Zhang et al., Citation2004). Wang et al. (Citation2014) reported that irrigation significantly increases the root weight density (RWD) in the 0–100 cm soil layers, particularly facilitating root growth in the deep soil layers. Suitable irrigation amount promotes root penetration, increases root density and utilization of water in deep soil, and consolidates the connection between water saving and high yield (Ram et al., Citation2013; Xue et al., Citation2010). Modulation in the activities of antioxidant enzymes may be one of the important factors in tolerance to environmental stress (Huseynova, Citation2012). Li et al. (Citation2006) recommended that moderate irrigation can increase soluble protein (SP) concentrations and root catalase (CAT) and superoxide dismutase (SOD) activities to a significantly larger extent than rainfed, seriously water stressed, or excessive irrigation conditions. Shi et al. (Citation1998) showed that light water stress [60% field capacity (FC)] produces no differences between SP concentrations and root CAT and SOD activities in comparison with control treatment (80% FC) and 60% FC can be as the lower limit index of soil moisture for normal growth and development of wheat. However, studies of irrigation practices that seek to match soil moisture, especially at different soil layers, and their effect on root distribution, root activity and senescence, water use at different soil layers, and yield production of winter wheat are relatively limited.
In this study, a new SI method that recharges SWC at critical developmental stages in three soil layers (0–20, 0–40, and 0–60 cm) and brought the SWC in each measured soil layer to 65% FC at jointing and 70% FC at anthesis. The objectives of this study are (1) to investigate root distribution, RA, and root senescence of winter wheat in response to SI; (2) to determine the response of soil water changes during grain filling, soil water use, and yield production of winter wheat to SI by measuring soil moisture; and (3) to clarify the relationships between RWD, root activities, root antioxidant enzyme activities, soil water consumption, grain yield, and WUE of winter wheat.
Materials and methods
Experimental site
Field experiments were conducted from October 2012 to June 2014 in the Shijiawangzi village, Yanzhou, Shandong Province, China (116°41′E, 35°42′N). The village is located in the centre of the Huang-Huai-Hai plain, and the environment is typical and representative of the plain. The area has a warm, temperate, semi-humid continental monsoon climate, an annual average temperature of 13.6 °C, annual accumulated sunshine of 2461 h, annual precipitation of 621.2 mm with 228.8 mm during the winter wheat growing season (the precipitation conditions are shown in Figure ) and a groundwater depth of 25 m. The soil is cinnamon (Cooperative Research Group on Chinese Soil Taxonomy [CRGCST], Citation2001). The organic matter (15.9 g kg−1), total nitrogen (1.2 g kg−1), available phosphorus (30.9 mg kg−1), and potassium (114.5 mg kg−1) in the topsoil of the experimental plots were measured using the potassium dichromate colorimetric method, the Kjeldahl method, the sodium bicarbonate method, and the ammonium acetate method, respectively, details can be found in Lu (Citation2000). The soil bulk density and SWC of each 20 cm soil layer of the top 0–200 cm are listed in Table . The precipitation during the 2012–2013 and 2013–2014 growing seasons was 225.0 and 170.0 mm, respectively. The precipitation distribution is shown in Figure .
Figure 1. The monthly precipitation during 2012–2014, the average value during the previous 15 years (Average 15).
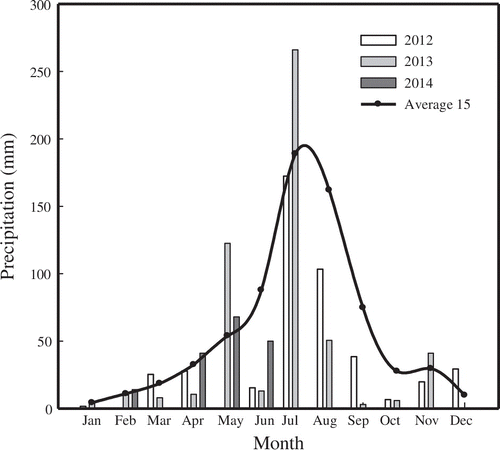
Table 1. Soil bulk density and soil water content of 20 cm layers in the top 0–200-cm soil profile of the experimental field.
Experimental design
Four treatments were designed: a rainfed (W0) treatment with no irrigation as the control and three SI treatments: the SWC at given soil depths was monitored prior to SI to calculate amount of irritation water that can rehydrate the soil to target SWC. The SWC before SI was monitored to depths of 20, 40, and 60 cm in treatments of W20, W40, and W60, respectively. All of the SI treatments brought the relative SWC in each measured soil layer to 65% FC at jointing (Z31, first node detectable) and 70% FC at anthesis (Z61, beginning of anthesis) (Zadoks et al., Citation1974). The required amounts of SI were calculated by the equation described by Cuenca (Citation1989) and Jalilian et al. (Citation2012):
where CIR (mm) is the amount of SI; ybd (g cm−3) is the soil bulk density; Dh (cm) is the thickness of the soil layer measured for SWC before SI (Table ); θt (%) is the target SWC on a weight basis after SI; and θn (%) is the SWC on a weight basis before SI. θt was calculated as follows:
Table 2. The target relative soil water content (θtr) and actual relative soil water content (θa) in the supplemental irrigation treatments (W20, W40 and W60) after jointing and anthesis in 2012–2013 and 2013–2014 growing season, the amount of supplemental irrigation (CIR) is also indicated.
where θmax (%) is the FC and θtr (%) is the target relative SWC (65% FC at jointing and 70% FC at anthesis in this study, respectively). A flow meter was used to measure the amount of water applied. CIR for each SI treatment (W20, W40 and W60) is shown in Table .
All treatments were replicated thrice and followed a randomized scheme. Each experimental plot was 4 × 4 m in size, with a 2.0-m wide zone without irrigation positioned between the two adjacent plots to prevent contamination.
Crop management
All plots were supplied with 240 kg N ha−1, 150 kg P2O5 ha−1, and 150 kg K2O ha−1. All P and K fertilizers and half of the N fertilizer were applied pre-sowing, and the remaining N fertilizer was top-dressed at the jointing stage. The high-yielding wheat (Triticum aestivum L.) cultivar Jimai22 was used in the experiments. Wheat seeds were sown at a density of 180 plants m−2 on 10 October 2012 and 9 October 2013. Wheat seedling shoot growth ceased at the beginning of December and recommenced at the end of February. During this period, the average daily temperature was below 0 °C. Wheat plants were harvested on 12 June 2013 and 6 June 2014.
Soil water measurement
Soil samples were collected using a soil corer (4.0 cm diameter) at 20-cm increments down to 200 cm in all experimental plots. SWC (gravimetric water content, %) was determined using the oven-drying method (Gardner, Citation1986).
where FW is the soil sample fresh weight (g); DW is the soil sample dry weight (g).
Measurements were performed before sowing (Z00), 1 day before irrigation, and 3 days after irrigation at both jointing (Z31) and anthesis (Z61) and at maturity (Z90). Three soil samples were collected at random locations from each plot.
Root sampling and RWD
Roots were sampled from each treatment (three replicates) were taken at anthesis and 10, 20, and 30 days after anthesis (DAA). Following to the description of Xue et al. (Citation2003) and Zhang et al. (Citation2009), we carefully removed the aboveground parts prior to root sampling and collected root samples at 20 cm increments down to 100 cm at anthesis and 20 cm increments down to 60 cm at 10 DAA, 20 DAA, and 30 DAA. Two cores per plot were collected: one within the crop row and one midway between rows. The resultant mixture of roots and soil was then placed in a polythene bag and washed with tap water. The soil and roots were carefully separated and refrigerated for further testing. The dry weight of the roots was determined by drying in an oven at 80 °C.
Root biochemical assays
Roots were sampled from each experimental plot, as described in the method above, by 20-cm increments to 60 cm at 10 DAA, 20 DAA, and 30 DAA. According to Li et al. (Citation2011), the root activity was determined using the TTC method (Lindström & Nyström, Citation1987), and represented by the triphenyl tetrazolium chloride (TTC) reduction activity. The root CAT activity was assayed by measuring the initial rate of H2O2 disappearance (Kato & Shimizu, Citation1987); the SOD activity was assayed by measuring the inhibition of the photoreduction of nitro blue tetrazolium (NBT) following the method of Giannopolitis & Ries (Citation1977); malondialdehyde (MDA) concentrations of flag leaves were assayed according to Quan et al. (Citation2004); and SP concentrations of flag leaves were measured according to the coomassie brilliant blue G250 method described by Read & Northcote (Citation1981). Details can be found in Wang et al. (Citation2013).
Crop water use
Total crop seasonal evapotranspiration (ETc) was calculated using the soil water balance equation (Chattaraj et al., Citation2013; Lv et al., Citation2011) for the growing season as follows:
where ETc (mm) is the total water consumption during the winter wheat growing season; P (mm) is the precipitation during the winter wheat growing season; CIR (mm) is the amount of SI; ΔW (mm) is the soil water storage at sowing minus the soil water storage at harvesting in the 0–200 cm soil layers; R (mm) is the surface run-off; and D (mm) is the downward flux below the crop root zone. The soil water measurements indicated that the drainage at the site is negligible. Therefore, deep percolation was not accounted for in this study (Ali et al., Citation2007; Lv et al., Citation2011).
WUE of crop was determined as the ratio of grain yield to ETc (Wang et al., Citation2011); Irrigation water productivity (IWP) was calculated as follows:
where ΔGY is the difference of grain yield between irrigated treatment and rainfed treatment; ΔETc is the difference of ETc between irrigated treatment and rainfed treatment (Karrou & Oweis, Citation2012).
Statistical analysis
Statistical analysis employed standard analysis of variance (ANOVA) using SPSS 13.0 software (SPSS Inc., Chicago, IL, USA.). Data for RWD, RA, root SP concentrations, CAT and SOD activities, MDA concentration, grain yield, and WUE were statistically analyzed at an α = 0.05 level of significance to determine whether significant differences exist among different treatments.
Results
Root distribution at anthesis
The vertical root distribution in the soil column at anthesis is presented in Figure . RWD decreased with depth in all treatments. The highest average RWD in the 0–100 cm soil layer (2.7 × 10−4 g cm−3) was associated with the W40 treatment. The RWD values for the W60, W20, and W0 treatments were 2.6 × 10−4, 2.1 × 10−4, and 1.7 × 10−4 g cm−3, respectively, and the differences among treatments were significant.
Figure 3. Responses of root weight density in the 0–100-cm soil layers to different treatments at anthesis in the 2012–2013 and 2013–2014 growing seasons, rainfed (W0), the soil water content before SI was monitored to depths of 20 cm (W20), 40 cm (W40) and 60 cm (W60), respectively, and bringing the soil moisture to 65% field capacity (FC) at jointing and 70% FC at anthesis. The same letter in the figure is not significant different at p = 0.05 by LSD test. Vertical bars are standard errors.
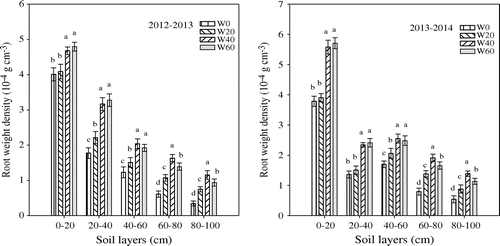
The 0–20-cm RWD between W40 and W60 did not differ and was significantly higher than that of W0 and W20. The RWD values in the 20–40-cm soil layer for W60 were higher than that for W40 and the difference was significant in the 2012–2013 growing season. In contrast, in the 40–100 cm soil layer, the RWD associated with W40 was higher than that in W60 and the difference was significant in the 60–100 cm soil layer. The lowest RWD was that of the 0–100 cm soil layer in the W0 treatment.
Root activity after anthesis
The TTC reduction activity in 0–60 cm soil layers during grain filling in 2012–2013 and 2013–2014 growing seasons was show in Table . The mean TTC reduction activity in W40 was the highest (47.0 mg g−1 h−1), which did not differ from that of W60, but was significantly higher than those in W20 and W0 with corresponding mean values of 39.0 and 25.8 mg g−1 h−1, respectively.
Table 3. The mean value of triphenyl tetrazolium chloride (TTC) reduction activity in 0–60-cm soil layers during grain filling of root in 2012–2013 and 2013–2014 growing seasons under different treatments.
TTC reduction activity decreased with soil depth and grain filling (Figure ). At 10 DAA, the TTC reduction activity in the 0–20, 20–40, and 40–60 cm soil layers did not differ between W40 and W60 and was higher than that for W20 and W0. At 20 DAA, the TTC reduction activity in the 0–20, 20–40, and 40–60 cm soil layers for W40 was higher than that for W20 and W0. However, the differences between W40 and W60 were not significant. At 30 DAA, the TTC reduction activity in the 0–20, 20–40, and 40–60 cm soil layers for W40 was higher than that for W60. The differences were significant in the 40–60 cm soil layer in the 2013–2014 growing season. The TTC reduction activity for W20 was significantly lower than that for W60. The W0 treatment was the lowest in TTC reduction activity in both growing seasons.
Root senescence after anthesis
The mean values of the root catalase (CAT) activities, the superoxide dismutase (SOD) activities, and the soluble protein (SP) concentrations in the 0–60 cm soil layer during grain filling are listed in Table . The W40 and W60 treatments resulted in significantly higher root CAT and SOD activities and SP concentrations than the W20 and W0 treatments. W0 treatment had the highest MDA concentration, whereas the MDA values for W20, W40, and W60 did not differ.
Table 4. The mean values of catalase (CAT) and superoxide dismutase (SOD) activities, soluble protein (SP) concentrations and malondialdehyde (MDA) concentrations of roots in 0–60 cm soil layers during grain filling under different treatments.
Root CAT and SOD activities and SP concentrations after anthesis decreased with soil depth (Figure ), whereas the MDA concentration increased. CAT and SOD root activities and SP concentrations in the 0–20-, 20–40-, and 40–60-cm soil layers did not differ among W20, W40, and W60 at 10 DAA. At 20 DAA, the CAT and SOD activities in the 20–40- and 40–60-cm soil layers and the SP concentrations in the 40–60-cm soil layer for W40 and W60 were significantly higher than those for W20. At 30 DAA, the CAT and SOD activities in the 0–20- and 20–40-cm soil layers and the SP concentrations in the 20–40-cm soil layer did not differ between W40 and W60. However, the CAT and SOD activities and SP concentrations in the 40–60-cm soil layer for W40 were significantly higher than those for W60. The W20 treatment was the lowest in CAT and SOD activities in all soil layers. The W0 treatment had the highest MDA concentration and the lowest CAT and SOD activities and SP concentrations in each soil layer during grain filling.
Figure 5. Changes in malondialdehyde (MDA) concentration, soluble protein (SP) concentration, and catalase (CAT) and superoxide dismutate (SOD) activities in winter wheat root at different soil layers after anthesis in different treatments in the 2013–2014 growing seasons. The same letter in the figure is not significant different at p = 0.05 by LSD test. Vertical bars are standard errors.
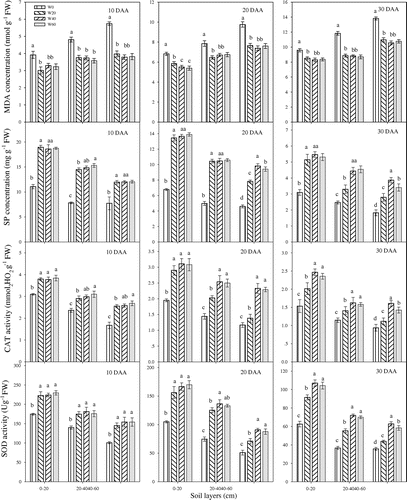
Soil water content and consumption after anthesis
The SWC in the 0–100 cm soil layer was significantly affected by SI at jointing and anthesis (Figure ). In the 2012–2013 growing season, at anthesis after SI, SWC in the 0–100 cm soil layers in W3 was the highest, and the values in the 0–80 cm soil layers in W40 were lower than that in W60, whereas the values in the 80–100-cm soil layer in W40 were higher than that in W60. At 20 DAA, SWC in the 0–80-cm soil layers did not differ between W40 and W60 and was significantly higher than that in W20. The SWC in the 80–100 cm soil layer did not differ in all treatments. At maturity, SWC in the 0–20- and 20–40-cm soil layers in W60 was the highest, followed by W40 and W20, but the value in W20 was lower than W40; the SWC in the 40–60 cm soil layer did not differ between W20 and W40; the SWC in the 80–100-cm soil layer in W20 and W60 was higher than that in W40.
Figure 6. Soil water content (SWC) at anthesis after SI, 20 DAA and maturity in 0–100 cm soil layers for SI treatments in the 2012–2013 and 2013–2014 growing season. The horizontal bars are standard errors.
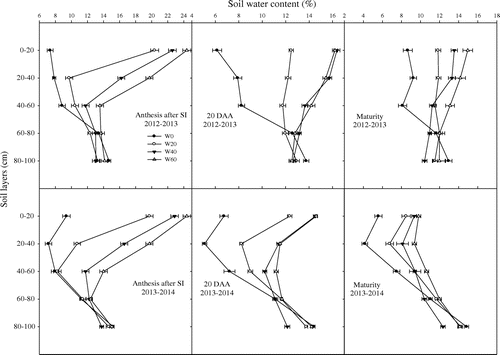
In the 2013–2014 growing season, at anthesis after SI, SWC in the 0–20-, 20–40-, and 40–60-cm soil layers in W3 was the highest, followed by W40 and W20. The SWC in the 60–80 cm soil layer did not differ between treatments (Figure ). At 20 DAA, the SWC in the 0–20-, 20–40- and 40–60-cm soil layers did not differ between W40 and W60 but was significantly higher than that in W20. The SWC in the 60–80- and 80–100-cm soil layers for W20 and W60 was significantly higher than W40. At maturity, the SWC in the 0–20-, 20–40-, and 40–60-cm soil layers in W60 was significantly higher than those in W40 and W20, and the SWC in the 60–80- and 80–100-cm soil layers in both W20 and W60 was higher than that in W40.
Figure shows the soil water consumption (SWU) from anthesis after SI to maturity. The SWU in 0–60 cm soil layers increased in the order of W60 > W40 > W20; however, the SWU in 40–60 cm soil layers for W40 and W60 did not differ in the 2012–2013 growing season. The SWU in the 60–100 cm soil layers increased in the order of W40 > W60 > W40 in both seasons.
Grain yield and water use
The highest grain yield, WUE, and irrigation water productivity (IWP) with corresponding mean values of 9168 kg ha−1, 20.8 kg ha−1 mm−1, and 35.5 kg ha−1 mm−1 were associated with the W40 treatment (Table ) and were followed by those associated with W60 and W20. W0 was associated with the lowest grain yield and WUE. Compared with W40, the mean value of grain yield was lower by 20.7 and 4.3%, WUE was lower by 11.9 and 5.7% and IWP was lower by 20.3 and 18.7% in W20 and W60 in both growing seasons.
Table 5. The crop evapotranspiration (ETc), grain yield, water use efficiency (WUE), and irrigation water productivity (IWP) in 2012–2013 and 2013–2014 growing seasons under different treatments.
Correlation analysis
The correlations between RWD and SWU, RWD and grain yield, and RWD and WUE in both seasons are analyzed and presented in Table . SWU, grain yield, and WUE showed significant positive correlation with RWD in all soil layers. The correlation between RWD and SWU in the 0–40 cm soil layer was stronger than in the 40–100 cm layer, whereas the RWD–grain yield and RWD–WUE correlations in the 0–40-cm soil layer were lower than in the 40–100-cm layer. Hence, high RWD makes better use of the soil water, particularly in the 0–40-cm soil layer, and the high RWD in the 40–100 cm soil layer significantly improves the grain yield and WUE.
Table 6. Correlation analysis between root weight density in different soil layers and soil water consumption (SWU) after anthesis after SI, grain yield and water use efficiency (WUE), n = 24.
There was significant positive correlation among RWD, root TTC reduction activity, CAT and SOD activities, SP concentrations, SWU from anthesis after SI to maturity, grain yield and WUE; however, the MDA concentration was strongly negatively correlated with RWD, TTC reduction activity, CAT, SOD, SP, grain yield, and WUE (Table ). SWU was strongly correlated with grain yield and WUE, whereas SWU, grain yield and WUE were more strongly correlated with RWD and TTC reduction activity.
Table 7. Correlation analysis between root weight density (RWD), root TTC reduction activities (TTCRA), malondialdehyde (MDA) concentration, catalase (CAT), superoxide dismutate (SOD) activities, soluble protein (SP) concentration, soil water consumption (SWU) from anthesis after SI to maturity, grain yield and water use efficiency (WUE), n = 24.
Discussion
Irrigation greatly affects the growth and development of crop root systems. Studies show that suitable amount of irrigation for wheat has greater root length density (Zhang et al., Citation2004) and RWD (Xue et al., Citation2003) than excessive or too little amount of irrigation for wheat and rainfed wheat. We found that irrigation significantly increases RWD in the 0–100-cm soil layer (Figure ). Wang et al. (Citation2014) and Xue et al. (Citation2010) found that appropriate irrigation regimes (75–150 mm irrigation water) with lower water stress could increase RWD under field conditions and facilitate root growth in deep soil layers. Li et al. (Citation2010) reported that under restricted irrigation regimes, irrigation of 120 mm only at jointing increased the root density in soil layers deeper than 30 cm, whereas irrigation of 40 mm at jointing, heading, and milking resulted in the highest root density in soil layers shallower than 30 cm. In the present study, the mean irrigation amount in W2 was 95.2 mm, which was significantly higher than that in W1 but lower than that in W3 (Table ). However, RWD in W2 was the highest, particularly in the 60–80- and 80–100-cm soil layers (Figure ). The RWD associated with the W1 treatment was the lowest in the 0–100-cm soil layer. The results suggest that the amount of irrigation determined by measuring the moisture of the 0–40-cm soil layer facilitate root growth in the 60–100-cm soil layer because of water and nutrient absorption (Merrill et al., Citation2002Qi et al., Citation2012).
Water stress and excessive water supply would limit root system and antioxidant enzyme activities (Li et al., Citation2006; Rasse & Smucker, Citation1998; Shi et al., Citation1998; Tang et al., Citation2014; Yang et al., Citation2010). Zuo et al. (Citation2010) reported that after drought stress treatment, root activities decreased by 28.5% at 12 DAA and 36.3% at 24 DAA compared with normal irrigation treatment. Wang et al. (Citation2012) found that appropriate soil water conditions (70–75% FC) could effectively promote root activity and increase the grain yield. It has been reported that SP concentrations and CAT and SOD activities decreased and MDA concentration increased with the water stress, thus accelerating plant senescence (Shi et al., Citation1998; Tang et al., Citation2014). The water content in the 0–40-cm soil layer was 63.4–63.5% FC at jointing and 68.2–70.3% FC at anthesis after SI in W40, which had the highest TTC reduction activity (45.8 mg g−1 h−1) (Figure ). Root SP concentrations and CAT and SOD activities in W40 were the highest, particularly in the 40–60-cm soil layer (Figure ). This suggests that SI, based on the 0–40-cm soil layer moisture, enhances the antioxidant enzyme activities and improves the root system activities, particularly in the 40–60-cm soil layer at the late growing stages of winter wheat.
Root growth in the soil layer is critical to the stored soil water uptake, particularly under water deficit conditions (Huang et al., Citation2012; Li et al., Citation2010; Shao et al., Citation2009). Xue et al. (Citation2003) showed that the water uptake rate in 0–100-cm soil layers was significantly lower in rainfed than in irrigation treatments because of low root density. Li et al. (Citation2010) reported that under restricted irrigation regimes, irrigation of 120 mm only at jointing results in the highest root length density, leading to the highest SWU in 0–160-cm soil layers. We found that the mean RWD in the 0–100-cm soil layer, particularly in the 60–100-cm layer, was the highest for W40 (Figure ). Moreover, SWC was the lowest in the 60–100-cm soil layer at 20 DAA (Figure ), indicating that the higher SWU of W2 in the 60–100-cm soil layer is due to the high RWD (Figure ).
Wheat grain yield and WUE are closely related to the growth and development of the root system in the soil (Li et al., Citation2006; Shi et al., Citation1998; Wang et al., Citation2014). Increased RWD was found to be associated with improved water use efficiency and grain yield of wheat in Mediterranean environments (Liao et al., Citation2004). Zhang et al. (Citation2009) reported that greater root biomass is significantly associated with greater aboveground biomass, which contributes to a higher grain yield. Li et al. (Citation2010) observed that irrigation of 60 mm each at jointing and heading increased the root length density in the 60–100-cm soil layers, resulting in the highest 1000-grain weight, grain yield, and WUE. Wang et al. (Citation2014) concluded that during the late growth stage, grain weight is closely positively correlated with RWD in the 0–100-cm soil layers. In the present study, grain yield and WUE were significantly positively correlated with RWD in all soil layers; a higher RWD, particularly in the 40–100-cm soil layers, can significantly improve grain yield and WUE (Table ). The study also showed that grain yield and WUE had a significant positive relationship with root TTC reduction activity, CAT and SOD activities, and SP concentration (Table ). Suitable irrigation at jointing and anthesis appears to increase RWD, root TTC reduction activity, and root antioxidant enzyme activity at the later stages of grain filling, which may be the reason why W40 achieved a high soil water absorption, grain yield, and WUE.
Conclusions
SI at jointing and anthesis, determined by measuring the moisture of the 0–40 cm soil layer, improves RWD in the 0–100-cm soil layer and particularly in the 60–80- and 80–100-cm soil layers compared with other treatments, which are likely the reasons of the high SWU in these soil layers. It also increases the root TTC reduction activity, root SP and CAT and SOD activities at the late stages of grain filling. Maximal grain yield, WUE and IWP were obtained at suitable SWC (65% FC at jointing and 70% FC at anthesis) with the W2 treatment. Moreover, significant positive correlation among RWD, root TTC reduction activity, SP concentrations, CAT and SOD activities, SWU, grain yield and WUE was observed.
Disclosure statement
No potential conflict of interest was reported by the authors.
Funding
This work was supported by the National Natural Science Foundation of China [31401334] and [31171498]; the National Agriculture Technology Research System of China [CARS-3-1-19].
References
- Abourached, C. G., Yau, S. K., Nimah, M. N., & Bashour, I. I. (2008). Deficit irrigation and split N fertilization on wheat and barley yields in a semi-arid, cool Mediterranean area. The Open Agriculture Journal, 2, 28–34.10.2174/1874331500802010028
- Ali, M. H., Hoque, M. R., Hassan, A. A., & Khair, A. (2007). Effects of deficit irrigation on yield, water productivity, and economic returns of wheat. Agricultural Water Management, 92, 151–161.10.1016/j.agwat.2007.05.010
- Chattaraj, S., Chakraborty, D., Garg, R. N., Singh, G. P., Gupta, V. K., Singh, S., & Singh, R. (2013). Hyperspectral remote sensing for growth-stage-specific water use in wheat. Field Crops Research, 144, 179–191.10.1016/j.fcr.2012.12.009
- Chen, C., Wang, E., & Yu, Q. (2009). Modeling wheat and maize productivity as affected by climate variation and irrigation supply in north China plain. Agronomy Journal, 102, 1037–1049.
- Chen, Y. L., Palta, J., Clements, J., Buirchell, B., Siddique, K. H. M., & Rengel, Z. (2014). Root architecture alteration of narrow-leafed lupin and wheat in response to soil compaction. Field Crops Research, 165, 61–70.10.1016/j.fcr.2014.04.007
- Cooperative Research Group on Chinese Soil Taxonomy. (2001). Chinese Soil Taxonomy. Beijing: Science Press.
- Cuenca, R. H. (1989). Irrigation system design, an engineering approach. Englewood Cliffs, NJ: Prentice Hall.
- Garciay Garcia, A., Larry, C. G., & Gerrit, H. (2009). Water use and water use efficiency of sweet corn under different weather conditions and soil moisture regimes. Agricultural Water Management, 96, 1369–1376.10.1016/j.agwat.2009.04.022
- Gardner, W. H. (1986). Water content. In A. Klute (Ed.), Methods of soil analysis, Part 1. Agron. Monogr. 9 (2nd ed., pp. 493–544). Madison (Wisconsin): Society of Agronomy and Soil Science Society of America.
- Giannopolitis, C. N., & Ries, S. K. (1977). Superoxide dismutase. I. Occurrence in higher plants. Plant Physiology, 59, 309–314.
- Hayashi, T., Yoshida, T., Fujii, K., Mitsuya, S., Tsuji, T., Okada, Y., … Yamauchi, A. (2013). Maintained root length density contributes to the waterlogging tolerance in common wheat (Triticum aestivum L.). Field Crops Research, 152, 27–35.10.1016/j.fcr.2013.03.020
- Huang, G. B., Chai, Q., Feng, F. X., & Yu, A. Z. (2012). Effects of different tillage systems on soil properties, root growth, grain yield, and water use efficiency of winter wheat (Triticum aestivum L.) in arid northwest China. Journal of Integrative Agriculture, 11, 1286–1296.10.1016/S2095-3119(12)60125-7
- Huseynova, I. M. (2012). Photosynthetic characteristics and enzymatic antioxidant capacity of leaves from wheat cultivars exposed to drought. Biochimica et Biophysica Acta (BBA) – Bioenergetics, 1817, 1516–1523.10.1016/j.bbabio.2012.02.037
- Jalilian, J., Modarres-Sanavy, S. A. M., Saberali, S. F., & Sadat-Asilan, K. (2012). Effects of the combination of beneficial microbes and nitrogen on sunflower seed yields and seed quality traits under different irrigation regimes. Field Crops Research, 127, 26–34.10.1016/j.fcr.2011.11.001
- Kang, S. Z., Hu, X. T., Goodwin, I., & Jerie, P. (2002). Soil water distribution, water use, and yield response to partial root zone drying under a shallow groundwater table condition in a pear orchard. Scientia Horticulturae, 92, 277–291.10.1016/S0304-4238(01)00300-4
- Karrou, M., & Oweis, T. (2012). Water and land productivities of wheat and food legumes with deficit supplemental irrigation in a Mediterranean environment. Agricultural Water Management, 107, 94–103.10.1016/j.agwat.2012.01.014
- Kato, M., & Shimizu, S. (1987). Chlorophyll metabolism in higher plants. VII. Chlorophyll degradation in senescing tobacco leaves, phenolic-dependent peroxidative degradation. Canadian Journal of Botany, 65, 729–735.10.1139/b87-097
- Kong, L. A., Wang, F. H., Feng, B., Li, S. D., Si, J. S., & Zhang, B. (2010). A root-zone soil regime of wheat: physiological and growth responses to furrow irrigation in raised bed planting in northern China. Agronomy Journal, 102, 154–162.10.2134/agronj2009.0288
- Lindström, A., & Nyström, C. (1987). Seasonal variation in root hardiness in container grown Scots pine, Norway spruce, and Lodgepole pine seedlings. Canadian Journal of Forest Research, 17, 787–793.10.1139/x87-126
- Li, J. C., Wei, F. Z., Wang, C. Y., & Yin, J. (2006). Effects of waterlogging on senescence of root system at booting stage in winter wheat. Acta Agronomica Sinica, 32, 1355–1360 (In Chinese).
- Li, Q. Q., Dong, B. D., Qiao, Y. Z., Liu, M. Y., & Zhang, J. W. (2010). Root growth, available soil water, and water-use efficiency of winter wheat under different irrigation regimes applied at different growth stages in North China. Agricultural Water Management, 97, 1676–1682.10.1016/j.agwat.2010.05.025
- Li, Z. J., Xie, X. Y., Zhang, S. Q., & Liang, Y. C. (2011). Negative effects of oxytetracycline on wheat (Triticum aestivum L.) growth, root activity, photosynthesis, and chlorophyll contents. Agricultural Sciences in China, 10, 1545–1553.10.1016/S1671-2927(11)60150-8
- Liao, M., Fillery, I. R. P., & Palta, J. A. (2004). Early vigorous growth is a major factor influencing nitrogen uptake in wheat. Functional Plant Biology, 31, 121–129.10.1071/FP03060
- Liu, H. J., Yu, L. P., Luo, Y., Wang, X. P., & Huang, G. H. (2011). Responses of winter wheat (Triticum aestivum L.) evapotranspiration and yield to sprinkler irrigation regimes. Agricultural Water Management, 98, 483–492.10.1016/j.agwat.2010.09.006
- Lu, R. K. (2000). Analysis methods of soil agro-chemistrical. Beijing: China Agriculture and Technology Press, pp. 109, 147, 150, 180, 194 (In Chinese).
- Lv, G., Kang, Y., Li, L., & Wan, S. (2010). Effect of irrigation methods on root development and profile soil water uptake in winter wheat. Irrigation Science, 28, 387–398.10.1007/s00271-009-0200-1
- Lv, L. H., Wang, H. J., Jia, X. L., & Wang, Z. M. (2011). Analysis on water requirement and water-saving amount of wheat and corn in typical regions of the North China Plain. Frontiers of Agriculture in China, 5, 556–562.10.1007/s11703-011-1149-4
- Ma, Y. X., Wang, C. Y., & He, D. X. (1999). Root of wheat. China Agriculture Press, Beijing, pp. 1–10 (In Chinese).
- Merrill, S. D., Tanaka, D. L., & Hanson, J. D. (2002). Root length growth of eight crop species in haplustoll soils. Soil Science Society of America Journal, 66, 913–923.10.2136/sssaj2002.9130
- Monti, A., & Zatta, A. (2009). Root distribution and soil moisture retrieval in perennial and annual energy crops in Northern Italy. Agriculture, Ecosystems & Environment, 132, 252–259.10.1016/j.agee.2009.04.007
- National Bureau of Statistics of China. (2013). China statistical yearbook (Vol. 449, pp. 1593). Beijing: China Statistics Press (In Chinese).
- Oweis, T., & Hachum, A. (2006). Water harvesting and supplemental irrigation for improved water productivity of dry farming systems in West Asia and North Africa. Agricultural Water Management, 80, 57–73.10.1016/j.agwat.2005.07.004
- Qi, W. Z., Liu, H. H., Liu, P., Dong, S. T., Zhao, B. Q., So, H. B., … Zhao, B. (2012). Morphological and physiological characteristics of corn (Zea mays L.) roots from cultivars with different yield potentials. European Journal of Agronomy, 38, 54–63.10.1016/j.eja.2011.12.003
- Quan, R. D., Shang, M., Zhang, H., C, Y. X., & Zhang, J. R. (2004). Improved chilling tolerance by transformation with betA gene for the enhancement of glycinebetaine synthesis in maize. Plant Science, 166, 141–149.10.1016/j.plantsci.2003.08.018
- Ram, H., Dadhwal, V., Vashist, K. K., & Kaur, H. (2013). Grain yield and water use efficiency of wheat (Triticum aestivum L.) in relation to irrigation levels and rice straw mulching in North West India. Agricultural Water Management, 128, 92–101.10.1016/j.agwat.2013.06.011
- Rasse, D. P., & Smucker, A. (1998). Root recolonization of previous root channels in corn and alfalfa rotations. Plant and Soil, 204, 203–212.10.1023/A:1004343122448
- Read, S. M., & Northcote, D. H. (1981). Minimization of variation in the response to different protein of the Coomassic blue G dye-binding assay for protein. Analytical Biochemistry, 116, 53–64.10.1016/0003-2697(81)90321-3
- Shao, L., Zhang, X., Chen, S., Sun, H., & Wang, Z. (2009). Effects of irrigation frequency under limited irrigation on root water uptake, yield and water use efficiency of winter wheat. Irrigation and Drainage, 58, 393–405.10.1002/ird.v58:4
- Shi, Y., Yu, Z. W., Wei, D. B., & Yu, S. L. (1998). Effects of soil water stress on the senescence of root system and flag leaves in wheat. Acta Botany Boreal-Occident Sinica, 18, 196–201 (In Chinese).
- Sun, H. Y., Liu, C. M., Zhang, X. Y., Shen, Y. J., & Zhang, Y. Q. (2006). Effects of irrigation on water balance, yield and WUE of winter wheat in the North China Plain. Agricultural Water Management, 85, 211–218.10.1016/j.agwat.2006.04.008
- Sun, Q. P., Krobel, R., Muller, T., Romheld, V., Cui, Z. L., Zhang, F. S., … Chen, X. P. (2011). Optimization of yield and water-use of different cropping systems for sustainable groundwater use in North China Plain. Agricultural Water Management, 98, 808–814.10.1016/j.agwat.2010.12.007
- Tang, Y. J., Ma, M., Deng, X. P., Tang, C. Q., Deng, R., & Yang, S. S. (2014). Relationships between drought resistance and root characteristics of wheat under drought stress. Journal of Northwest A&F University, 42: 48–53, 60 (In Chinese).
- Wang, C. Y., Liu, W. X., Li, Q. X., Ma, D. Y., Lu, H. F., Feng, W., … Guo, T. C. (2014). Effects of different irrigation and nitrogen regimes on root growth and its correlation with above-ground plant parts in high-yielding wheat under field conditions. Field Crops Research, 165, 138–149.10.1016/j.fcr.2014.04.011
- Wang, D., Yu, Z., & White, P. J. (2013). The effect of supplemental irrigation after jointing on leaf senescence and grain filling in wheat. Field Crops Research, 151, 35–44.10.1016/j.fcr.2013.07.009
- Wang, F. H., Wang, X. Q., & Ken, S. (2004). Comparison of conventional, flood irrigated, flat planting with furrow irrigated, raised bed planting for winter wheat in China. Field Crops Research, 87, 35–42.
- Wang, J., Liu, W. Z., & Dang, T. H. (2011). Responses of soil water balance and precipitation storage efficiency to increased fertilizer application in winter wheat. Plant and Soil, 347, 41–51.10.1007/s11104-011-0764-4
- Wang, J. C., Xu, Y. L., Gao, S., Han, X. F., Xu, C. L., & Qiao, J. J. (2012). The physiological characteristics and root spatial distribution of spring wheat in drip irrigation field. Acta Agriculturae Boreali-Occidentalis Sinica, 21, 65–70.
- Wang, S. F., Zhang, X. Y., & Pei, D. (2006). Impacts of different water supplied conditions on root distribution, yield and water utilization efficiency of winter wheat. Transaction of the Chinese Society Agricultural Engineering, 22, 27–32 (In Chinese).
- Xue, L. H., Duan, J. J., Wang, Z. M., Guo, Z. W., & Lu, L. Q. (2010). Effects of different irrigation regimes on spatial-temporal distribution of roots, soil water use and yield in winter wheat. Acta Ecologica Sinica, 30, 5296–5305 (In Chinese).
- Xue, Q., Zhu, Z., Musick, J. T., Stewart, B. A., & Dusek, D. A. (2003). Root growth and water uptake in winter wheat under deficit irrigation. Plant and Soil, 257, 151–161.10.1023/A:1026230527597
- Yang, S. M., Li, B. G., Qi, G. H., Guo, S. P., & Hu, Z. W. (2010). Effects of alternate partial root zone irrigation on roots activity, stem sap flow and fruit of apple. Transaction of the Chinese Society Agricultural Engineering, 26, 73–79 (In Chinese).
- Zadoks, J. C., Chang, T. T., & Konzak, C. F. (1974). A decimal code for the growth stages of cereals. Weed Research, 6, 415–421.10.1111/wre.1974.14.issue-6
- Zhang, H., Wang, X., You, M., & Liu, C. (1999). Water-yield relations and water-use efficiency of winter wheat in the North China Plain. Irrigation Science, 19, 37–45.10.1007/s002710050069
- Zhang, X. Y., Pei, D., & Chen, S. Y. (2004). Root growth and soil water utilization of winter wheat in the North China Plain. Hydrological Processes, 18, 2275–2287.10.1002/(ISSN)1099-1085
- Zhang, X. Y., Chen, S. Y., Sun, H. Y., Wang, Y. M., & Shao, L. W. (2009). Root size, distribution and soil water depletion as affected by cultivars and environmental factors. Field Crops Research, 114, 75–83.10.1016/j.fcr.2009.07.006
- Zhang, X., Chen, S., Sun, H., Shao, L., & Wang, Y. (2011). Changes in evapotranspiration over irrigated winter wheat and maize in North China Plain over three decades. Agricultural Water Management, 98, 1097–1104.10.1016/j.agwat.2011.02.003
- Zuo, W. B., Wu, J. L., Yang, Q., Zhang, J. N., & Liu, G. R. 2010. Study on the influence of root of different wheat varieties under drought stress. Acta Agriculturae Boreali Sinica, 25: 191–193 (In Chinese).