Abstract
To identify differences in root plasticity patterns of two upland New Rice for Africa (NERICA) varieties, NERICA 1 and 4, in response to drought under conditions with contrasting soil profile characteristics, soil moisture gradients were imposed using a sloping bed system with depths ranging 30–65 cm and a line-source sprinkler system with a uniformly shallow soil layer of 20 cm depth. Varietal differences in shoot and root growths were identified only under moderate drought conditions, 11–18% v/v soil moisture content. Further, under moderate drought soil conditions where roots could penetrate into the deep soil layer, deep root development was greater in NERICA 4 than in NERICA 1, which contributed to maintaining dry matter production. However, under soil conditions with underground impediment to deep root development, higher shoot dry weight was noted for NERICA 1 than for NERICA 4 at 11–18% v/v soil moisture content, which was attributed to increased lateral root development in the shallow soil layer in NERICA 1. Enhanced lateral root development in the 0–20-cm soil layer was identified in NERICA 1 even under soil conditions without an impediment to deep root development; however, this did not contribute to maintaining dry matter production in upland rice. Thus, we show different root developmental traits associated with drought avoidance in the two NERICA varieties, and that desirable root traits for upland rice cultivation vary depending on the target soil environment, such as the distribution of soil moisture and root penetration resistance.
Introduction
In sub-Saharan Africa, where rice consumption has increased rapidly in recent years, the major ecosystem for rice cultivation is rainfed uplands which account for approximately 40% of sub-Saharan Africa’s total rice area (IRRI, Citation2013). This is more than double the overall global proportion of rice crops that are rainfed upland rice, which is 15% or approximately 19 million hectares (Thanh et al., Citation1999). Yields of traditional upland rice in sub-Saharan Africa average about 1 t ha−1 (Kijima et al., Citation2006) and tend to be unstable because of erratic and unpredictable rainfall (Serraj et al., Citation2011), frequent droughts, low soil water holding capacity, and, to a greater extent, shallow root system of the rice crop (Mambani & Lal, Citation1983). A pressing issue is the improvement of upland rice productivity in Africa.
The upland New Rice for Africa (NERICA) varieties, which are inter-specific hybrids between Oryza sativa and Oryza glaberrima developed by the Africa Rice Center (Balasubramanian et al., Citation2007; Jones et al., Citation1997), have potential yields of 4–7 t ha−1 (Sekiya et al., Citation2013). There are currently 18 varieties suited for upland growth conditions (NERICA 1 to NERICA 18) (Wainaina et al., Citation2015). The use of these improved rice varieties is expected to achieve remarkable yield improvements (Kijima et al., Citation2006) and therefore catalyze a rice green revolution in sub-Saharan Africa (Matsumoto et al., Citation2014).
Among the 18 upland NERICA varieties, NERICA 4 and NERICA 1 have been adopted or certified in 14 and 12 countries, respectively, making them the most widely cultivated among sub-Saharan Africa countries, and covering the most total area among NERICA varieties in different countries (Diagne et al., Citation2010). However, inconsistent results have been reported for the yield of NERICA 1 and NERICA 4 from various upland cultivation trials conducted under rainfed conditions in some locations in sub-Saharan African countries. For instance, in western Kenya (Atera et al., Citation2011) and multiple locations in Tanzania (Sekiya et al., Citation2013), NERICA 1 had a higher yield than NERICA 4. On the other hand, farmers in the semi-arid Kerio Valley of Kenya (Kinyumu, Citation2009), multiple locations in Uganda (Onaga et al., Citation2012), and parts of northern Nigeria (Kamara et al., Citation2010) selected NERICA 4 because of its higher yields than NERICA 1. The inconsistencies in yield performance of NERICA 1 and NERICA 4, which have been observed even under the same climatic conditions, could be due to the differences between the two varieties in drought avoidance mechanisms and their expression in different soil environments.
There is extensive evidence in the literature that a deep root system with thick roots contributes to drought avoidance in rice under upland field conditions (Araki et al., Citation2002; Kato et al., Citation2006; Lilley & Fukai, Citation1994; Yoshida & Hasegawa, Citation1982). Although deep roots give the rice plant access to water at depth (Price et al., Citation2002), this advantage may depend on the duration of the drought period, the water-holding capacity of the soil (Kamoshita et al., Citation2004), and the soil penetration resistance (Cairns et al., Citation2004; Samson et al., Citation2002). Furthermore, Kano et al. (Citation2011) suggested that under field conditions these traits may not be functional or fully expressed due to strong interactions with the soil environment.
Upland rice cultivation environments in sub-Saharan Africa have highly heterogeneous soil physical characteristics; large variations in whether penetration resistance is sufficient to impede vertical root growth into deep soil profile have been reported across upland sites used for drought experiments at the West African Rice Development Association (WARDA, now Africa Rice Centre) (Cairns et al., Citation2011). Under such conditions, the ability of plant roots to change their morphology (e.g. increased total root length and lateral root elongation) (O’Toole & Bland, Citation1987) and physiological functions (e.g. alterations in water and nutrient uptake capacity) (Hodge, Citation2003) as environmental conditions change is known as phenotypic plasticity. Phenotypic plasticity is a key trait for the adaptation of rice plants to drought conditions (Kamoshita et al., Citation2008; Kano et al., Citation2011; Niones et al., Citation2012; Tran et al., Citation2014a). Tran et al. (Citation2014b) showed that root plasticity triggered by drought was clearly expressed under relatively compacted soils, while Kameoka et al. (Citation2015) showed that rice varieties expressed root plasticity either as lateral root development on the soil surface or dry matter allocation to deep root development. According to Tran et al. (Citation2015), such plastic changes in the rice root were triggered specifically by water-deficit conditions, and not by changes in the forms of nitrogen available in the soil.
Thus, in this study, we hypothesized that the differences between NERICA 1 and NERICA 4 under upland field conditions were because of differences in the drought avoidance mechanisms that are related with root traits. The root traits we considered included plasticity in deep root development, and in lateral root development in the shallow soil layer in accordance with soil heterogeneous rooting depth environments for upland rice cultivation in sub-Saharan Africa. Plasticity in deep root development is expected to be beneficial for upland rice cultivation; however, its functional expression can be affected by underground impediments to root penetration. On the other hand, plasticity in lateral root development in the shallow soil layer can be useful for sustaining dry matter production under field conditions with high soil penetration resistance. We expected that NERICA 1 and NERICA 4 would have different root traits with regard to drought avoidance, so their shoot growth response would differ under various intensities of drought conditions and in different soil environments.
To prove this hypothesis, two experimental upland rice cultivation systems simulating different soil environments were used in this study to expose plants to various intensities of drought stress. One is a sloping bed system with a gradient of soil depths from 30 to 65 cm to allow for the expression of deep root traits in response to the lowering of available soil moisture to deeper soil layers. Kameoka et al. (Citation2015) revealed that a sloping bed can effectively create a gradient of available water both along the soil surface and along the vertical soil profile by manipulating the ground water level. The other experimental system is a line-source sprinkler system with a soil depth of approximately 20 cm, which is expected to eliminate the advantageous effects of the deep root trait. The line-source sprinkler system was previously used to evaluate the plasticity in lateral root development in the shallow soil layer in response to different intensities of drought stress (Kano et al., Citation2011; Tran et al., Citation2014a). A comparative analysis of root and shoot growth responses to various intensities of drought stress in the two systems should clarify the genotypic differences in drought avoidance-related root traits. Thus, in this study, we aimed to evaluate the genotypic differences between NERICA 1 and NERICA 4 in their plasticity in deep root development, and lateral root development in the shallow soil layer, under different intensities of drought stress using the sloping bed and line-source sprinkler systems, respectively.
Materials and methods
Field experiments
Two field experiments were carried out under a rainout shelter with a sloping bed system (Experiment 1) and a line-source sprinkler system (Experiment 2) at the Togo Field, Field Science Center, Graduate School of Bioagricultural Sciences, Nagoya University, Togo-cho, Aichi, Japan (35°6′42″N, 137°4′57″E) in 2010 and 2011. The monthly mean maximum temperature experienced inside the rainout shelter during the experiment was 29–35 °C in 2010 and 27–33 °C in 2011, while the monthly mean minimum temperature was 22–27 °C in 2010 and 21–25 °C in 2011. The soil used for both experiments was classified as sandy loam (72.3% sand, 20.9% silt, 6.8% clay) with a field capacity of 27.7% w/w (gravimetric), wilting point of 10.6% w/w, pH (H2O) of 5.5, electrical conductivity of 3.3 mS m−1, cation exchange capacity of 9.5 cmol kg−1, available K of 2.1 g kg−1, and a total nitrogen content of 1.3 g kg−1. Two upland NERICA varieties, NERICA 1 and NERICA 4, were used in both experiments.
Experiment 1. Evaluation of plasticity in deep root development
To evaluate the plasticity in deep root development of NERICA 1 and NERICA 4, a soil moisture gradient was created using the sloping bed system. Lemont was used in 2010, and IRAT109 in 2011 as check varieties. Lemont, which is an improved irrigated lowland variety that can adapt to the upland ecosystem, has thick nodal roots and deep root characteristics (Kato et al., Citation2006). On the other hand, IRAT109, which is an upland japonica variety originally developed in the Ivory Coast, has a deep rooting trait and is often used as a drought-resistant donor in breeding programs (Ji et al., Citation2012; Nemoto et al., Citation1998).
The design and soil moisture manipulation of the sloping bed system were the same as those described by Kameoka et al. (Citation2015). Briefly, the sloping bed was established using removable aluminum panes in a water tight pool with an underlying impermeable plastic sheet at an inclination angle of 15°. The length and minimum and maximum heights of the experimental bed were 200, 30, and 80 cm, respectively.
Seeds of the four varieties were sterilized using a fungicide (Benlate; Sumitomo Chemical Garden Products Inc. Tokyo, 0.15% w/v) for 24 h, and then soaked in running water and incubated in a seed germinator at a constant temperature of 30 °C for 48 h. The pre-germinated seeds were then sown in plastic trays with soil under well-watered conditions. Twenty-one-day-old seedlings of each variety, with an intact root system, were transplanted into three unpuddled and well-watered plots in rows along the slope on 4 June 2010 and on 3 June 2011, at a rate of one seedling per hill. The experimental design for the sloping bed system was regarded as a split-plot design in which a soil moisture gradient, created as a result of increasing soil depths from the water table, was the main plot. Rows containing seven plants of a specific variety, grown across the gradient, were the subplot. Thus, each three-row plot contained three varieties, with plant spacing of 28 cm between hills of the same variety and 50 cm between randomized rows. After transplanting, the field was kept well-watered with sprinkler irrigation. Different intensities of drought stress were imposed on the soil surface at 14 d after transplanting (DAT) by withholding sprinkler irrigation and decreasing the ground water level. In 2010, the ground water level was maintained at 15 cm from the bottom until 39 DAT and then lowered to and maintained at 5 cm until sampling. In 2011, the ground water level was maintained at 5 cm from the bottom throughout the growth period. Chemical fertilizer was applied at a rate of 100 kg N ha−1, 100 kg P ha−1, and 100 kg K ha−1 in the form of a compound fertilizer split twice; first as a basal application at 14 DAT, and then as a top dressing at 50 DAT. This fertilizer application rate was determined to be appropriate for this study based on the results of field studies examining the growth of upland rice in response to nitrogen (Jones et al., Citation1997), phosphorus (Sahrawat et al., Citation1995), and potassium (Fageria et al., Citation1990). Preventive measures were taken to preclude damage from disease and insects.
Shoot samples were collected at 123 DAT in 2010 and 102 DAT in 2011. Thus, the total soil drying duration was 109 d in 2010 and 88 d in 2011. In 2011, roots for each plant along the soil moisture gradient and increasing soil depths were sampled at 20-cm depth increments from the soil surface using a stainless steel cylinder measuring 15 cm in diameter (Kang et al., Citation1994). Deep roots were defined as roots that developed below 20-cm soil depth in this study.
Experiment 2. Evaluation of plasticity in lateral root development
To evaluate the plasticity in lateral root development of NERICA 1 and NERICA 4, a line-source sprinkler was used to create a soil moisture gradient. Milyang 23 was used in 2010, and Lemont in 2011 as check varieties. Milyang 23 is a lowland Indica/Japonica derivative that is cultivated in Korea and known to be high yielding (Cho et al., Citation2010). The dimensions of the experimental field were 23.0 m by 3.6 m (L × W). The field was made watertight with an underlying polyvinyl chloride (PVC) sheet at an average depth of 20 cm below the soil surface. A line-source sprinkler was established at the midpoint along the field at 15 cm above the ground. The sprinkler system comprised a 4-cm-diameter PVC pipe with nozzles through which water was released in the form of a mist across both sides of the field and a drainage system. The drainage system was operated manually. We continuously confirmed that the drainage system could open to release excess water and/or close to prevent water and nutrient drainage as described by Kano et al. (Citation2011). An automated system was used to regulate the timing and intensity of irrigation to create a soil moisture gradient from wet to dry. To precisely control the amount and duration of irrigation and thus create a smooth moisture gradient from wet to dry, soil moisture content was measured every hour using soil moisture sensors (EC-5 Decagon, Utah, USA). The sensors were placed at a depth of 12 cm at five points 30 cm apart on both sides of the line-source sprinkler (total 10 sensors).
Seed sowing, seedling management, transplanting dates, and fertilizer management were done in a manner similar to Experiment 1. Twenty-one-day-old seedlings of each variety, with an intact root system, were transplanted into three unpuddled plots, in rows perpendicular to the line-source sprinkler, at a rate of one seedling per hill. As in Experiment 1, the experimental design for the line-source sprinkler system was regarded as a split-plot design with three replications. Each plot contained three rows, in which each row contained eight plants of a specific variety that were exposed to a soil moisture gradient created by a line-source sprinkler. Plant spacing was 20 cm between hills of the same variety and 40 cm between randomized rows. Fourteen days after transplanting, drought stress was imposed by a sprinkler set to automatically release a mist of water for a distance of up to 80 cm three times a day (at 06:00, 11:00, and 18:00) for 1 min. Thus, we were able to create and maintain a moisture gradient across the field such that the soil was drier with increasing distance from the sprinkler. Shoot samples were collected at 93 DAT and 66 DAT in 2010 and 2011, respectively. Hence, the total soil drying duration was 79 d in 2010 and 52 d in 2011. Roots were sampled on the same day as shoot sampling using a stainless steel cylinder that was 15 cm diameter. Lateral root length was used to evaluate the differences between varieties in their plasticity in lateral root development.
Measurements
The shoot dry weight of each plant was determined after oven-drying for 3 d at 72 °C. Soil moisture content at 0–12-cm soil depth was monitored using a time-domain reflectometry probe (TDR; Tektronix Inc., Wilsonville, OR, USA) (Ledieu et al., Citation1986). The soil moisture content for each individual plant was obtained by averaging soil moisture measurements obtained 10 cm from opposite sides of the plant. We determined the relationship between soil moisture content and the equivalent soil water potential values in three plastic pots (170 mm diameter × 190 mm height) that were filled with 2.5 kg of the same soil used in this experiment. Two stainless steel nails for obtaining soil moisture content readings using TDR and a tensiometer were inserted to a depth of 12 cm in each pot, which was then flooded and gradually drained to −80 kPa. Gradual changes in soil moisture and the corresponding soil water potential were regularly recorded. As the result, we generated an equation: y = −0.11x2 + 7.4x − 115 (n = 90, R2 = 0.797). When x (TDR value, % v/v) was 0; y (tensiometer reading, kPa) was −115 kPa. Thus, soil moisture readings provided by TDR for each plant in Experiment 1 and 2 were converted into soil water potential values using the calibrated equation.
To statistically evaluate the effects of different intensities of drought stress on shoot and root growths, we followed the procedure described by Kano et al. (Citation2011). In this study, soil moisture content values were divided into four ranges: well-watered, >28% v/v (>−3 kPa), mild drought stress, 18–28% v/v (−18 to −3 kPa); moderate drought stress, 11–18% v/v (−48 to −18 kPa), and severe drought stress, <11% v/v (<−48 kPa). For well-watered conditions, the reference 28% v/v soil moisture content (−3 kPa) was near saturation level. The boundary between mild and moderate drought stress was at 18% v/v soil moisture content (−18 kPa), and beyond −20 kPa stomatal conductance was reported to be inevitably reduced compared to saturated soil conditions (Kato & Okami, Citation2011). For severe drought stress conditions, the reference 11% v/v soil moisture content was close to the critical soil moisture content (i.e. 14% v/v) when most legumes and cereals start showing signs of wilting (Suralta et al., Citation2010). In our experiments, the effects of different intensities of drought stress were confirmed during the growing period by observation. For example, under conditions of severe drought in Experiment 2, we observed leaf rolling, reduced leaf elongation, and dead leaves for all varieties. However, in Experiment 1, the soil moisture gradient did not reduce to reach the levels of severe drought. All plants in each soil moisture range were sampled from the three replicate groups in order to identify varietal differences in shoot dry weight and total root length. Average values were computed from three replicates to perform statistical analyses in 2011. In 2010, the experiment was conducted with one replication, thus not adequate to perform statistical analyses.
Root trait analysis
The whole root system for each plant was cut into approximately 1-cm segments and spread in a thin film of water on transparent plastic sheets with minimal overlapping. Digital tiff files were then taken using an EPSON scanner (ES2200, Seiko Epson Corporation, Japan) with a transparency adapter at 300 dpi resolution. In 2010, total root length was determined using a macro program developed by Kimura and Yamasaki (Citation2001) on the NIH image software version 1.60 a public domain released by the National Institute of Health, USA. In 2011, total root length was determined using WinRhizo software v. 2007 d (Regent Instruments Inc., Saint-Foy, Canada) (Bouma et al., Citation2000).
In Experiment 1, branching index in the shallow soil layer (0–20 cm) was calculated by dividing lateral root length by nodal root length (Morita & Collins, Citation1990). Root length density was calculated as the ratio of the root length in a certain soil layer to the soil volume from which the roots were obtained. Deep root length ratio was calculated as the ratio of root length below 20-cm soil depth to total root length.
In Experiment 2, lateral root length values were obtained by subtracting the nodal root length value from the total root length value for each plant. The nodal root length for each plant was calculated as the sum of each nodal root length measured manually using a ruler.
Statistical analysis
Statistical analyses included a multiple regression analysis to examine fitted curves of the relationships between soil moisture content and (a) total root length and (b) shoot dry weight. Statistical analyses were performed using SPSS statistical software (version17.0) (SPSS Inc., Chicago, Illinois, USA). To test the effects of soil moisture, variety, and their interaction on total root length and shoot dry weight, an analysis of variance was performed using the general linear model (GLM) procedure of the Statistical Analysis System (version 9.0) (SAS Institute Inc., Cary, NC, USA), where soil moisture content was the main plot and the varieties were the split plot factor. Means of shoot and root traits across soil moisture treatments were separated by the least significant difference (LSD) test at p < 0.05.
Results
Experiment 1. Shoot and root responses when roots are allowed to penetrate into the deep soil layer
There was a significant interaction between the effect of soil moisture and variety on shoot dry weight but not total root length (Table ). Figure shows the relationship between soil moisture content and shoot dry weight for plants grown under field conditions with a sloping bed system in Experiment 1. Soil moisture content at 0–12 cm depth from the soil surface ranged from 16 to 44% v/v in 2010 and from 11 to 44% v/v in 2011. NERICA 1 started to reduce its shoot dry weight as the soil moisture content at 0–12 cm soil depth decreased below 20% v/v. On the other hand, the shoot dry weight of NERICA 4, Lemont, and IRAT109 was not affected by the soil moisture content in the shallow soil. Based on the regression curve equations, the shoot dry weight of NERICA 1 peaked at 28.7 and 30.1% v/v in 2010 and 2011, respectively (Figure ).
Table 1. Shoot dry weight (SDW), total root length (TRL), and branching index (BI) in the 0–20-cm soil layer of rice varieties grown under different soil moisture content (SMC) levels in a field with a sloping bed system in Experiment 1 in 2010 and 2011.
Figure 1. Relationships between soil moisture content at 0–12 cm depth and shoot dry weight for NERICA 1 (●—), NERICA 4 (○), Lemont (▲), and IRAT109 (×) plants grown in a field with a sloping bed system in Experiment 1. Shoot dry weight was determined at 123 d in 2010 and 102 d after transplanting in 2011. The regression equations for the curves were y = −0.08x2 + 4.6x + 4 (R2 = 0.893*) for NERICA 1, y = −0.01x2 − 0.3x + 72 (R2 = 0.458 ns) for NERICA 4, and y = 0.03x2 + 1.8x + 111 (R2 = 0.138 ns) for Lemont in 2010; and y = −0.10x2 + 6.1x + 9 (R2 = 0.590*) for NERICA 1, y = 0.01x2 − 0.4x + 94 (R2 = 0.045 ns) for NERICA 4, and y = −0.02x2 − 1.5x + 129 (R2 = 0.294 ns) for IRAT109 in 2011. *Indicates significance at p < 0.05, ns indicates not significant.
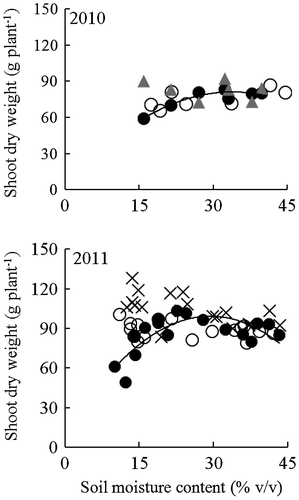
In 2010, the shoot dry weight of NERICA 4 tended to be higher than NERICA 1, and Lemont accumulated 53 and 28% higher shoot dry weight than did NERICA 1 and NERICA 4, respectively, under moderate drought conditions (11–18% v/v) (Table ). In 2011, the shoot dry weight of NERICA 4 was significantly higher than that of NERICA 1, and the shoot dry weight of IRAT109 was higher than that of the two NERICA varieties under moderate drought conditions. However, under mild drought and well-watered conditions, there were no significant differences between the two NERICA varieties. The shoot dry weight of IRAT109 was higher than that of NERICA 4 under mild drought conditions.
Total root length, which ranged from 76.9 to 231.5 m per plant across soil moisture content levels in 2011, was not affected by the soil moisture content at 0–12-cm soil depth for any variety (Figure ). Furthermore, there were no significant differences in the total root length among the varieties in any of the soil moisture regimes in 2011 (Table ). Branching index did not differ for all varieties under well-watered conditions, however, that of NERICA 1 was higher than that of NERICA 4 under mild and moderate drought conditions. Under moderate drought conditions, the branching index of NERICA 1 was significantly higher than that under well-watered conditions, while that of NERICA 4 and IRAT109 was comparable between the two soil moisture regimes (Table ).
Figure 2. Relationships between soil moisture content at 0–12 cm depth and total root length for NERICA 1 (●), NERICA 4 (○), and IRAT109 (×) plants grown in a field with a sloping bed system in Experiment 1 in 2011. Total root length was determined at 102 d after transplanting. The regression equations for the curves were y = 0.06x2 − 3.8x + 188 (R2 = 0.056 ns) for NERICA 1, y = −0.10x2 + 3.7x + 108 (R2 = 0.196 ns) for NERICA 4, and y = −0.05x2 + 1.8x + 134 (R2 = 0.0381 ns) for IRAT109. ns indicates not significant.
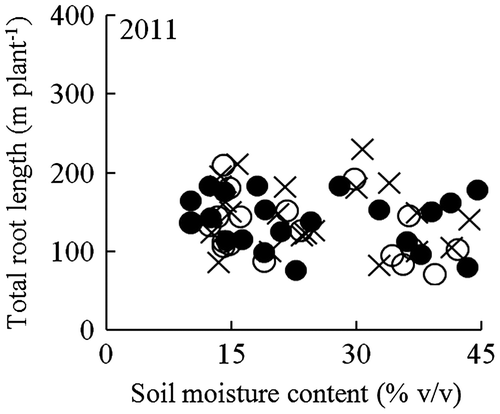
Since genotypic differences in shoot dry weight were observed only under moderate drought conditions, we compared root length densities to evaluate expression of a deep root trait under moderate drought conditions. The root length density of NERICA 4 was significantly lower than that of NERICA 1 and IRAT109 in the 0–20-cm soil layer (Table ). Below the 20-cm soil layer, the root length density of NERICA 4 and IRAT109 was significantly higher than that of NERICA 1. On the other hand, deep root length ratio was significantly higher in NERICA 4 than in NERICA 1 and IRAT109.
Table 2. Root length density (RLD) at 0–20 and 20–60-cm soil depth, and deep root length ratio (DRR) for the three rice varieties grown under 11–18% v/v soil moisture content in a field with a sloping bed system in Experiment 1 in 2011.
There was no significant correlation between total root length in the 0–20 cm soil layer and the shoot dry weight in any variety (Figure a). On the other hand, total root length below 20-cm soil depth was positively and significantly correlated with shoot dry weight for NERICA 4 (r = 0.952*) and IRAT109 (r = 0.943*), while there was no significant correlation for NERICA 1 (r = 0.801 ns) (Figure b).
Figure 3. Relationship between total root length (TRL) at 0–20-cm soil depth and shoot dry weight (a), and TRL below 20-cm soil depth and shoot dry weight (b) under 11–18% v/v soil moisture content for NERICA 1 (●), NERICA 4 (○—), and IRAT109 (×– –) plants grown in a field with a sloping bed system in Experiment 1 in 2011. Total root length and shoot dry weight were determined at 102 d after transplanting. *Indicates significance at p < 0.05.
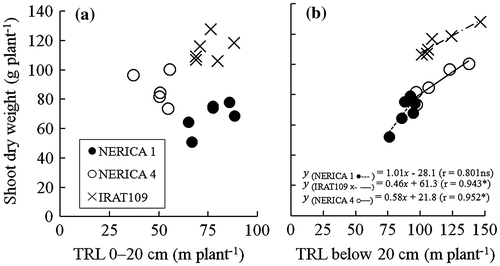
Experiment 2. Shoot and root responses when the advantageous effect of the deep root trait is eliminated
A two-way ANOVA revealed that the effect of soil moisture content and variety on shoot dry weight and total root length was significant at p < 0.05 (Table ). The multiple regression analysis for the fitted curves revealed that the relationship between soil moisture content and shoot dry weight was significant for all varieties in both years (Figure ). The shoot dry weight of Milyang 23 increased exponentially as soil moisture content increased, while the shoot dry weight of Lemont, NERICA 1, and NERICA 4 peaked at 24–34% v/v soil moisture content. Under well-watered, severe drought, and mild drought conditions, there were no significant differences in shoot dry weight among the varieties in 2011 (Table ). Under moderate drought conditions, however, NERICA 1 tended to be higher in shoot dry weight than NERICA 4. Under severe, moderate, and mild drought conditions, the shoot dry weights of Milyang 23 were consistently lower than those of the two NERICA varieties; however, it was threefold greater than those of the two NERICA varieties under well-watered conditions.
Table 3. Shoot dry weight (SDW) and total root length (TRL) of rice varieties grown under different soil moisture content levels (SMC) in a field with a line-source sprinkler system in Experiment 2 in 2010 and 2011.
Figure 4. Relationships between soil moisture content at 0–12-cm soil depth and shoot dry weight for NERICA 1 (●—), NERICA 4 (○∙∙∙∙), Milyang 23 (△– – –), and Lemont (▲– – –) plants grown in a field with a line-source sprinkler system in Experiment 2. Shoot dry weight was determined at 93 d in 2010 and 66 d after transplanting in 2011. The regression equations for the curves were y = −0.23x2 + 11.0x − 58 (R2 = 0.961***) for NERICA 1, y = −0.06x2 + 4.0x − 10 (R2 = 0.909**) for NERICA 4, and y = 0.22x2 − 4.0x + 33 (R2 = 0.905**) for Milyang 23 in 2010; and y = −0.08x2 + 4.2x + 6 (R2 = 0. 690**) for NERICA 1, y = −0.06x2 + 3.8x + 0.1 (R2 = 0.781**) for NERICA 4, and y = −0.07x2 + 3.8x + 10 (R2 = 0.660**) for Lemont in 2011. *, ** and ***Indicate significance at p < 0.05, p < 0.01 and p < 0.001, respectively. ns indicates not significant.
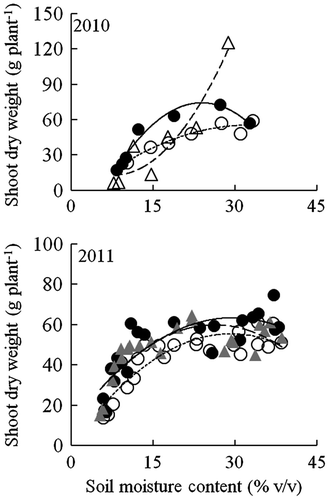
Figure shows the relationship between soil moisture content and total root length for plants grown in a field with a line-source sprinkler system in 2010 and 2011. Multiple regression analysis revealed a significant relationship between soil moisture content and total root length in all varieties. The total root length of the two NERICA varieties and Lemont peaked under mild drought conditions (23–25% v/v soil moisture content) in 2011, in which total root length of NERICA 1, NERICA 4, and Lemont was 44, 61, and 68% higher than that under well-watered conditions, respectively. In contrast, Milyang 23 peaked under well-watered conditions (44% v/v soil moisture content) in 2010 (Figure ). Total root lengths of NERICA 1, NERICA 4, and Lemont were comparable under all soil moisture regimes except moderate drought conditions in 2011 (Table ). Under moderate drought conditions, the total root length of NERICA 1 increased by 56–68% compared to that under well-watered conditions; that of NERICA 4 increased by 16% in 2011, but was reduced in 2010, while that of Lemont increased by 20%. Consequently, the total root length of NERICA 1 was significantly higher than that of NERICA 4 and Lemont under moderate drought conditions in 2011. Although not significant, in 2010, NERICA 1 had about 2.4 times greater total root length than did NERICA 4 under moderate drought. On the other hand, under severe drought conditions, the total root length was reduced by 29–39% for NERICA 1, 44–49% for NERICA 4, and 56–59% for Lemont compared to that under well-watered conditions in 2010 and 2011.
Figure 5. Relationships between soil moisture content and total root length for NERICA 1 (●—), NERICA 4 (○∙∙∙∙), Milyang 23 (△– – –), and Lemont (▲– – –) plants grown in a field with a line-source sprinkler system in Experiment 2. Total root length was determined at 93 d in 2010 and 66 d after transplanting in 2011. The regression equations for the curves were y = −3.6x2 + 163.1x − 116 (R2 = 0.922*) for NERICA 1, y = −0.36x2 + 30.6x − 16 (R2 = 0.933**) for NERICA 4, and y = −1.3x2 + 67.4x − 247 (R2 = 0.933**) for Milyang 23 in 2010; and y = −1.04x2 + 47.8x − 143 (R2 = 0.636***) for NERICA 1, y = −0.99x2 + 46.7x − 199 (R2 = 0.726***) for NERICA 4, and y = −0.77x2 + 35.5x − 62.6 (R2 = 0.561***) for Lemont in 2011. *, ** and ***Indicate significance at p < 0.05, p < 0.01 and p < 0.01, respectively.
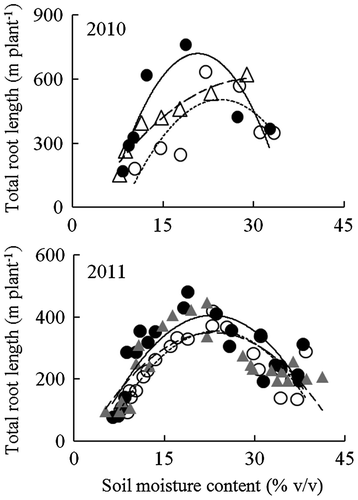
Since genotypic differences in shoot dry weight and total root length were observed only under moderate drought conditions, root traits expressed under moderate drought conditions are analyzed below. NERICA 1 had the largest lateral root length in both years (Table ); on the other hand, there was no significant difference among the varieties in the nodal root length in 2011. The lateral root length of plants grown under moderate drought conditions was positively and significantly correlated with shoot dry weight in NERICA 1 (r = 0.882*), while, for NERICA 4 (r = 0.672 ns) and Lemont (0.075 ns), this relationship was not significant in 2011 (Figure ). For all varieties, the relationship between lateral root length and shoot dry weight was not significant under well-watered, mild drought, and severe drought conditions (data not shown)
Table 4. Lateral root length (LRL) and nodal root length (NRL) of rice varieties grown under 11–18% v/v soil moisture content in a field with a line-source sprinkler system in Experiment 2 in 2010 and 2011.
Figure 6. Relationship between lateral root length and shoot dry weight in 11–18% v/v soil moisture content for NERICA 1 (●—), NERICA 4 (○), and Lemont (×) plants grown in a field with a line-source sprinkler system in Experiment 2 in 2011. Lateral root length and shoot dry weight were determined at 66 d after transplanting. *Indicates significance at p < 0.05.
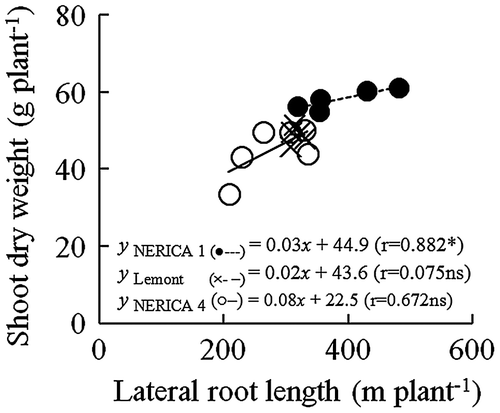
Discussion
Soil moisture content in two experimental systems
In the rainfed upland rice ecosystem, the soil starts drying from the surface, but the deep soil horizon may remain wet and able to supply water to roots (Yoshida & Hasegawa, Citation1982). However, if there is an underground impediment to root penetration such as hardpan, the advantageous effect of deep rooting will be eliminated (Cairns et al., Citation2011; Wade et al., Citation1999). In this study, we used the sloping bed and the line-source sprinkler systems to mimic such drought conditions. In the sloping bed system, soil moisture gradients in the 0–12cm soil layer and the vertical soil profile were created (Figure ). On the other hand, the line-source sprinkler system successfully created a soil moisture gradient in the 0–12-cm soil layer with root systems confined to a soil depth of 20 cm (Figure ). These results indicated that the sloping bed and line-source sprinkler systems are practical for simulating drought conditions under soil environments where the soil starts drying from the surface while maintaining wet conditions in deep soil layers and soil environments where deep root penetration to the subsoil strata is inhibited due to the presence of a hard soil layer, respectively. Thus, by using the two systems, we were able to compare the root plasticity of rice varieties that were expected to express root plasticity differently according to the soil environments.
Shoot and root responses to different intensities of drought stress
It is widely known that rice is an aquatic plant and therefore naturally adapted to high soil moisture environments (Kato & Okami, Citation2010). For Milyang 23, a typical irrigated lowland variety, shoot dry weight increased with increasing in soil moisture content (Figure ). However, the maximum shoot dry weight of the upland NERICA varieties, Lemont, and IRAT109 was not obtained in the wettest soil moisture conditions in either the sloping bed (Figure ) or the line-source sprinkler system (Figure ). This indicates that upland NERICA varieties can adapt to mild to moderate drought as well as aerobic conditions (well-drained, non-puddled, and non-saturated soils).
There were varietal differences in the relationships between soil moisture content and shoot dry weight (Figures and ) in both systems, and total root length (Figure ) in the line-source sprinkler system. Moreover, the varietal differences in shoot dry weight and total root length were identified only under moderate drought conditions in 2011 (Tables and ). These results imply that advantages of drought avoidance-related traits were expressed specifically under moderate drought conditions, which was around 11–18% v/v soil moisture content.
Expression of root plasticity in deep root development
Under moderate drought conditions in the sloping bed system, root length density in the deep soil layer was higher for NERICA 4 and IRAT109 than for NERICA 1 (Table ), although the total root length was not affected by the soil moisture content at 0–12 cm soil depth (Figure ). Furthermore, NERICA 4 had a greater deep root length ratio than both IRAT109 and NERICA 1 (Table ). These results indicate that the root plasticity of NERICA 4 was expressed by developing more roots in the deep soil layers where moisture was more available. Although IRAT109 developed more roots in the deep, wet soil layers than NERICA1, the deep root length ratio of IRAT109 was comparable with that of NERICA 1 and lower than that of NERICA 4. This was because IRAT109, which was used as the deep rooting check variety, developed more roots in the shallow layers than NERICA 4. Moreover, shoot dry weight in NERICA 4 and IRAT109 significantly increased with increasing total root length below 20-cm soil depth (Figure b). Deep root development in rice can contribute to dry matter production under drought through enhanced water uptake (Gowda et al., Citation2011; Henry, Citation2012; Kamoshita et al., Citation2004; Uga et al., Citation2011). Therefore, it can be assumed that NERICA 4 and IRAT109 had access to wet soil at depth from the increased root length density below 20-cm soil depth (Table ). Thus, both varieties maintained shoot dry weight regardless of the soil moisture content in the 0–12-cm soil layer in 2011 (Figure ).
On the other hand, under moderate drought conditions in the line-source sprinkler system, the total root length of NERICA 4 tended to be shorter than that of NERICA 1 and Milyang 23 in 2010, and those of NERICA 4 and Lemont were shorter than that of NERICA 1 in 2011 (Table ). This shows that NERICA 4, the deep rooting variety, was not able to enhance root system when there was an underground impediment to deep root development.
Expression of root plasticity in lateral root development
In the line-source sprinkler system, the total root lengths of NERICA 1, NERICA 4, and Lemont increased by 16–74% compared to well-watered plants under mild and moderate drought conditions (with the exception of NERICA 4 under moderate drought conditions) in 2010. In contrast, total root length of Milyang 23 declined with a decrease in soil moisture content (Table ). This indicates that root plasticity in NERICA 1, NERICA 4, and Lemont was expressed by enhancing root systems in response to mild and moderate drought conditions, whereas that of Milyang 23 was not expressed. Consistent with the results of Kano et al. (Citation2011), under severe drought conditions, total root lengths were reduced in all varieties by 29–57%, suggesting that root plasticity in lateral root development was not expressed due to the insufficient soil moisture content.
Under moderate drought conditions in the line-source sprinkler system, lateral root length tended to be higher for NERICA 1 than for NERICA 4 and Lemont (Table ). This indicates that NERICA 1 has greater root plasticity in lateral root development than NERICA 4 and Lemont under field conditions with an underground impediment to root penetration. Varietal differences in root plasticity in lateral root development have been observed at 21–42% v/v soil moisture content (considered to be mild drought to well-watered conditions in our study) for lowland rice varieties (Kano et al., Citation2011; Tran et al., Citation2014a). Our results imply that varietal differences in root plasticity in lateral root development for upland varieties may be more clearly observed under drier soil moisture conditions than those of lowland varieties.
On the other hand, under moderate drought conditions in the sloping bed system, the branching index of NERICA 1 increased by 39% compared to well-watered conditions, while that of NERICA 4 and IRAT109 increased by 20% and 13%, respectively (Table ). This shows that NERICA 1 expressed greater plasticity in lateral root development in the shallow soil layer than NERICA 4 and IRAT109 even in the sloping bed system where roots were allowed to penetrate into the deep soil layer.
In the line-source sprinkler system, NERICA 1 increased shoot dry weight with increasing lateral root length (Figure ). This indicates that the enhanced root systems contributed to the dry matter production when the advantageous effect of a deep root trait is eliminated. This result is consistent with those reported previously by Kano et al. (Citation2011) and Tran et al. (Citation2014a). In the sloping bed system, however, NERICA 1 reduced shoot dry weight under moderate drought conditions (Figure ), even though lateral root development in the shallow soil layer was enhanced (Table ). Furthermore, in NERICA 1, the total root length in the 0–20-cm soil layer was not correlated with shoot dry weight under moderate drought conditions in the sloping bed system (Figure a). These results are in agreement with those reported by Kameoka et al. (Citation2015) suggesting that root plasticity expressed in soil layers where moisture is less available may not contribute to maintaining dry matter production in upland NERICA varieties.
Conclusions
In this study, we aimed to evaluate the differences in drought avoidance-related root traits between NERICA 1 and NERICA 4, specifically plasticity in deep root development and lateral root development, by using sloping bed and line-source sprinkler systems. The results of this study confirmed that there are genotypic differences in root developmental traits associated with drought avoidance in rice, in accordance with other papers (e.g. Kano et al., Citation2011; Tran et al., Citation2014a). This study revealed that NERICA 4 has greater plasticity in deep root development, while NERICA 1 has greater plasticity in lateral root development, and the varietal differences in root plasticity were observed especially clearly under moderate drought conditions. The expression of these traits seemed to contribute to maintaining dry matter production through enhanced water uptake unless enhancement of lateral root development occurs in soil layers where moisture is less available. Thus, to maintain dry matter production in upland rice under moderate drought conditions, the ability to express root plasticity in soil layers, where moisture is more available, is important. The study suggests that desirable root traits for upland rice cultivation vary depending on the target soil environment, such as the distribution of soil moisture and root penetration resistance. For instance, for field conditions with an underground impediment to root penetration, an appropriate rice variety should have high plasticity in lateral root development in the shallow soil layer in response to drought stress (e.g. NERICA 1). On the other hand, for soil environments without an underground impediment to deep root development, a variety which has an enhanced ability to develop a root system in the deep, wet soil layer is more suitable (e.g. NERICA 4).
Disclosure statement
No potential conflict of interest was reported by the authors.
References
- Araki, H., Morita, S., Tatsumi, J., & Iijima, M. (2002). Physiol-morphological analysis on axile root growth in upland rice. Plant Production Science, 5, 286–293.10.1626/pps.5.286
- Atera, E. A., Onyango, J. C., Azuma, T., Asanuma, S., & Itoh, K. (2011). Field evaluation of selected NERICA rice cultivars in western Kenya. African Journal of Agricultural Research, 6, 60–66.
- Balasubramanian, V., Sie, M., Hijmans, R. J., & Otsuka, K. (2007). Increasing rice production in sub-Saharan Africa: Challenges and opportunities. Advances in Agronomy, 94, 55–133.10.1016/S0065-2113(06)94002-4
- Bouma, T. J., Nielsen, K. L., & Koutstaal, B. (2000). Sample preparation and scanning protocol for computerised analysis of root length and diameter. Plant and Soil, 218, 185–196.10.1023/A:1014905104017
- Cairns, J. E., Audebert, A., Townend, J., Price, A. H., & Mullins, C. E. (2004). Effect of soil mechanical impedance on root growth of two rice varieties under field drought stress. Plant and Soil, 267, 309–318.10.1007/s11104-005-0134-1
- Cairns, J. E., Impa, S. M., O’Toole, J. C., Jagadish, S. V. K., & Price, A. H. (2011). Influence of the soil physical environment on rice (Oryza sativa L.) response to drought stress and its implications for drought research. Field Crops Research, 121, 303–310.10.1016/j.fcr.2011.01.012
- Cho, Y. G., Kang, H. J., Lee, Y. T., Jong, S. K., Eun, M. Y., & McCouch, S. R. (2010). Identification of quantitative trait loci for physical and chemical properties of rice grain. Plant Biotechnology Reports, 4, 61–73.10.1007/s11816-009-0120-9
- Diagne, A., Midingoyi, S. G., Wopereis, M., & Akintayo, I. (2010). The NERICA success story: development, achievements and lessons learned. (online). Retrieved August 18, 2015, from http://siteresources.worldbank.org/AFRICAEXT/Resources/258643-1271798012256/NERICA-Success-Story-11-2010.pdf. World Bank Africacan Archives.
- Fageria, N. K., Wright, R. J., Baligar, V. C., & Carvalho, J. R. P. (1990). Upland rice response to potassium fertilization on a Brazilian oxisol. Fertilizer Research, 21, 141–147.10.1007/BF01087423
- Gowda, V. R. P., Henry, A., Yamauchi, A., Shashidhar, H. E., & Serraj, R. (2011). Root biology and genetic improvement for drought avoidance in rice. Field Crops Research, 122, 1–13.10.1016/j.fcr.2011.03.001
- Henry, A. (2012). IRRI’s drought stress research in rice with emphasis on roots accomplishments over the last 50 years. Plant Root, 7, 92–106.
- Hodge, A. (2003). The plastic plant: root responses to heterogenous supplies of nutrients. New Phytologist, 162, 9–24.
- IRRI. (2013). Ricepedia: The online authority on rice. Retrieved from http://ricepedia.org/rice-around-the-world/africa
- Ji, K., Wang, Y., Sun, W., Lou, Q., Mei, H., Shen, S., & Chen, H. (2012). Drought-responsive mechanisms in rice genotypes with contrasting drought tolerance during reproductive stage. Journal of Plant Physiology, 169, 336–344.10.1016/j.jplph.2011.10.010
- Jones, M. P., Dingkuhn, M., Aluko, K. G., & Semon, M. (1997). Interspecific Oryza sativa L. X O. glaberrima Steud. progenies in upland rice improvement. Euphytica, 92, 237–246.10.1023/A:1002969932224
- Kamara, A. Y., Ekeleme, F., Omoigui, L. O., Oikeh, S. O., Chikoye, D., & Tegbaru, A. (2010). Response of upland rice cultivars to nitrogen fertilizer in the savannas of Nigeria. Agronomy Journal, 102, 333–339.10.2134/agronj2009.0238
- Kameoka, E., Suralta, R. R., Mitsuya, S., & Yamauchi, A. (2015). Matching the expression of root plasticity with soil moisture availability maximizes production of rice plants grown in an experimental sloping bed with soil moisture gradients. Plant Production Science, 18, 267–276.10.1626/pps.18.267
- Kamoshita, A., Rodriguez, R., Yamauchi, A., & Wade, L. J. (2004). Genotypic variation in response of rainfed lowland rice to prolonged drought and rewatering. Plant Production Science, 7, 406–420.10.1626/pps.7.406
- Kamoshita, A., Babu, R. C., Boopathi, N. M., & Fukai, S. (2008). Phenotypic and genotypic analysis of drought-resistance traits for development of rice cultivars adapted to rainfed environments. Field Crops Research, 109, 1–23.10.1016/j.fcr.2008.06.010
- Kang, S. Y., Morita, S., & Yamazaki, K. (1994). Root growth and distribution in some japonica-indica hybrid and japonica type rice cultivars under field conditions. Japanese Journal of Crop Science, 63, 118–124.10.1626/jcs.63.118
- Kano, M., Inukai, Y., Kitano, H., & Yamauchi, A. (2011). Root plasticity as the key root trait for adaptation to various intensities of drought stress in rice. Plant and Soil, 342, 117–128.10.1007/s11104-010-0675-9
- Kato, Y., Abe, J., Kamoshita, A., & Yamagishi, J. (2006). Genotypic variation in root growth angle in rice (Oryza sativa L.) and its association with deep root development in upland fields with different water regimes. Plant and Soil, 287, 117–129.10.1007/s11104-006-9008-4
- Kato, Y., & Okami, M. (2010). Root growth dynamics and stomatal behaviour of rice (Oryza sativa L.) grown under aerobic and flooded conditions. Field Crops Research, 117, 9–17.10.1016/j.fcr.2009.12.003
- Kato, Y., & Okami, M. (2011). Root morphology, hydraulic conductivity and plant water relations of high-yielding rice grown under aerobic conditions. Annals of Botany, 108, 575–583.10.1093/aob/mcr184
- Kijima, Y., Sserunkuuma, D., & Otsuka, K. (2006). How revolutionary is the “NERICA revolution”? Evidence from Uganda. The Developing Economies, 44, 252–267.10.1111/deve.2006.44.issue-2
- Kimura, K., & Yamasaki, S. (2001). Root length and diameter measurement using NIH Image: Application of the line-intercept principle for diameter estimation. Plant and Soil, 234, 37–46.10.1023/A:1010501905868
- Kinyumu, M. D. (2009). Comparative study on the growth and yield of NERICA cultivated with organic and inorganic fertilizers: Participatory on-farm research at Marakwet district in Kenya. Journal of Developments in Sustainable Agriculture, 4, 106–117.
- Ledieu, J., Deridder, P., Declerck, P., & Dautrebande, S. (1986). A method of measuring soil-moisture by time-domain reflectometry. Journal of Hydrology, 88, 319–328.10.1016/0022-1694(86)90097-1
- Lilley, J. M., & Fukai, S. (1994). Effect of timing and severity of water-deficit on four diverse rice cultivars. 1. Rooting pattern and soil-water extraction. Field Crops Research, 37, 205–213.10.1016/0378-4290(94)90099-X
- Mambani, B., & Lal, R. (1983). Response of upland rice varieties to drought stress. 1. Relation between root-system development and leaf water potential. Plant and Soil, 73, 59–72.10.1007/BF02197757
- Matsumoto, S., Tsuboi, T., Asea, G., Maruyama, A., Kikuchi, M., & Takagaki, M. (2014). Water response of upland rice varieties adopted in sub-Saharan Africa: A water application experiment. Journal of Rice Research, 2, 121. doi:10.4172/jrr.1000121
- Morita, S., & Collins, H. (1990). A method to decribe root branching. Japanese Journal of Crop Science, 59, 580–581.10.1626/jcs.59.580
- Nemoto, H., Suga, R., Ishihara, M., & Okutsu, Y. (1998). Deep rooted rice varieties detected through the observation of root characteristics using the trench method. Breeding Science, 48, 321–324.
- Niones, J. M., Suralta, R. R., Inukai, Y., & Yamauchi, A. (2012). Field evaluation on functional roles of root plastic responses on dry matter production and grain yield of rice under cycles of transient soil moisture stresses using chromosome segment substitution lines. Plant and Soil, 359, 107–120.10.1007/s11104-012-1178-7
- Onaga, G., Asea, G., Lamo, J., Kikafunda, J., & Bigirwa, G. (2012). Comparison of response to nitrogen between upland NERICAs and ITA (Oryza sativa) rice varieties. Journal of Agricultural Science, 4, 197–205.
- O’Toole, J. C., & Bland, W. L. (1987). Genotypic variation in crop plant root systems. Advances in Agronomy, 41, 91–145.10.1016/S0065-2113(08)60803-2
- Price, A.H., Steele, K.A., Gorham, J., Bridges, J.M., Moore, B.J., Evans, J.L., … Jones, R. G. W. (2002). Upland rice grown in soil-filled chambers and exposed to contrasting water-deficit regimes. I. Root distribution, water use and plant water status. Field Crops Research, 76, 11–24.10.1016/S0378-4290(02)00012-6
- Sahrawat, K. L., Jones, M. P., & Diatta, S. (1995). Response of upland rice to phosphorus in an Ultisol in the humid forest zone of West Africa. Fertilizer Research, 41, 11–17.10.1007/BF00749515
- Samson, B. K., Hasan, M., & Wade, L. J. (2002). Penetration of hardpans by rice lines in the rainfed lowlands. Field Crops Research, 76, 175–188.10.1016/S0378-4290(02)00038-2
- Sekiya, N., Khatib, J. K., Makame, S. M., Tomitaka, M., Oizumi, N., & Araki, H. (2013). Performance of a number of NERICA cultivars in Zanzibar, Tanzania: Yield, yield components and grain quality. Plant Production Science, 16, 141–153.10.1626/pps.16.141
- Serraj, R., McNally, K. L., Slamet-Loedin, I., Kohli, A., Haefele, S. M., Atlin, G., & Kumar, A. (2011). Drought resistance improvement in rice: An integrated genetic and resource management strategy. Plant Production Science, 14, 1–14.10.1626/pps.14.1
- Suralta, R. R., Inukai, Y., & Yamauchi, A. (2010). Dry matter production in relation to root plastic development, oxygen transport, and water uptake of rice under transient soil moisture stresses. Plant and Soil, 332, 87–104.10.1007/s11104-009-0275-8
- Thanh, N. D., Zheng, H. G., Dong, N. V., Trinh, L. N., Ali, M. L., & Nguyen, H. T. (1999). Genetic variation in root morphology and microsatellite DNA loci in upland rice (Oryza sativa L.) from Vietnam. Euphytica, 105, 43–51.
- Tran, T. T., Kano-Nakata, M., Suralta, R. R., Menge, D., Mitsuya, S., Inukai, Y., & Yamauchi, A. (2015). Root plasticity and its functional roles were triggered by water deficit but not by the resulting changes in the forms of soil N in rice. Plant and Soil, 386, 65–76.10.1007/s11104-014-2240-4
- Tran, T. T., Nakata-Kano, M., Takeda, M., Menge, D., Mitsuya, S., Inukai, Y., & Yamauchi, A. (2014a). Nitrogen application enhanced the expression of developmental plasticity of root systems triggered by mild drought stress in rice. Plant and Soil, 378, 139–152.10.1007/s11104-013-2013-5
- Tran, T. T., Menge, D., Nakata-Kano, M., Suralta, R. R., Mitsuya, S., Inukai, Y., & Yamauchi, A. (2014b). Effects of soil compaction on the expression of plasticity in root system development triggred by water deficit conditions and nitrogen application and its contribution to dry matter production in rice. Japanese Journal of Crop Science, 83, 230–231.
- Uga, Y., Okuno, K., & Yano, M. (2011). Dro1, a major QTL involved in deep rooting of rice under upland field conditions. Journal of Experimental Botany, 62, 2485–2494.10.1093/jxb/erq429
- Wade, L. J., Fukai, S., Samson, B. K., Ali, A., & Mazid, M. A. (1999). Rainfed lowland rice: Physical environment and cultivar requirements. Field Crops Research, 64, 3–12.10.1016/S0378-4290(99)00047-7
- Wainaina, C.M., Inukai, Y., Masinde, P.W., Ateka, E.M., Murage, H., Kano-Nakata, M., … Makihara, D. (2015). Evaluation of cold tolerance in NERICAs compared with Japanese standard rice varieties at the reproductive stage. Journal of Agronomy and Crop Science, 201, 461–472. doi:10.1111/jac.12125
- Yoshida, S., & Hasegawa, S. (1982). The rice root system: Its development and function. In Drought resistance in crops with emphasis on rice (pp. 97–115). Los Baños: IRRI.