Abstract
Saline soils hamper various physiological functions in soybean [Glycine max (L.) Merr.]. One example is the reduction in nitrogen (N) uptake capacity, a major dysfunction that limits soybean growth and yield under saline conditions. Previous studies have revealed that tolerance to salinity varies with cultivar; however, the cultivars used in these studies were selected solely based on agro-morphological traits. In this study, we examined genotypic variation in salinity tolerance among 85 soybean genotypes which were selected based on an assessment of both single nucleotide polymorphisms (SNP) markers and agro-morphological traits. Additionally, we examined whether salt tolerance is associated with nodulation and N uptake. We used a subset of the world soybean mini-core collection (80 cultivars) and an additional five cultivars/genetic lines (NILs72-T, NILs72-S, Enrei, En-b0-1, and En1282). All plants were grown in pots and treated with saline (final concentration of 150 mM NaCl) during the vegetative growth stage. To evaluate salinity tolerance, we used the ratio of saline-treated (S) to control (C) plant total dry weight [DW (S/C)]. The ratio differed markedly according to genotype. Furthermore, salinity-tolerant genotypes exhibited superior nodulation, leaf greenness, and N uptake under saline conditions. These results indicate that there is a marked genotypic variation in salinity tolerance, and that the tolerant genotypes exhibit greater nodulation and N uptake, although further studies are needed to clarify whether the superior nodulation and N uptake of salinity-tolerant genotypes are responsible for the observed tolerance.
Classification:
Soil salinity is the primary environmental factor limiting crop production in arid and semi-arid regions (Neumann, Citation1997), with high salinity adversely affecting approximately 10% of the world’s cropland (Shannon, Citation1997). Affected soils contain high concentrations of soluble salts, particularly sodium and chloride, causing growth and yield reductions in most plant species (Flowers & Flowers, Citation2005; Niknam & McComb, Citation2000; Tuyen et al., Citation2010). Saline soil impairs physiological functions through multiple mechanisms, including water stress, specific ion toxicity, ion imbalance stress, induced nutrient deficiency, or a combination of these factors (Jones, Citation1981; Munns & Tester, Citation2008; Shannon, Citation1997; Zhang et al., Citation2010).
Genetic analyses using recombinant inbred lines (RIL) derived from crosses between salt-tolerant and susceptible genotypes have indicated that salt tolerance in soybean is linked to quantitative trait loci (QTLs) (Chen et al., Citation2008; Hamwieh & Xu, Citation2008; Hamwieh et al., Citation2011; Lee et al., Citation2004; Xu & Tuyen, Citation2012). Using a population derived from the salt-tolerant cultivar Tiefeng 8 and the salt-sensitive cultivar 85-140, Guan et al. (Citation2014a) mapped a salt tolerance gene (GmSALT3) from Tiefeng 8, which is a dominant gene associated with limiting the accumulation of Na+ in shoots. In their subsequent study, they showed that salinity tolerance in soybean is modulated by natural variation in the GmSALT3 gene (Guan et al., Citation2014b). Recently, Do et al. (Citation2016) isolated a gene (Ncl) that regulates ion (Na+, K+, Cl−) transport and accumulation, and demonstrated that higher expression of Ncl in the root led to lower ion accumulation in the shoot. Furthermore, when Ncl was incorporated into the ‘Kariyutaka’ soybean cultivar, the transformed genotype significantly enhanced salt tolerance. In another study, using an RIL population of a Glycine soja accession, Qi et al. (Citation2014) identified the salt tolerance gene CHX1, which conferred the capacity of lowering the Na+ content in leaves. Using this gene, a subsequent study developed high-throughput SNP markers for salinity tolerance in soybean (Patil et al., Citation2016). These studies indicate that it is feasible to screen for or breed genotypes with an improved capacity of regulating the specific ions responsible for salt tolerance.
Saline conditions hamper nodule formation in soybean (Singleton & Bohlool, Citation1984; Yasuta & Kokubun, Citation2014), significantly reducing growth (Abel & MacKenzie, Citation1964; Wang & Shannon, Citation1999) and seed yield (Parker et al., Citation1983; Yang & Blanchar, Citation1993). Salt-induced limitation of nodulation in legumes appears to reduce growth and yield through reducing N uptake (Balasubramanian & Sinha, Citation1976; Lakshmi-Kumari et al., Citation1974; Lauter et al., Citation1981; Singleton & Bohlool, Citation1984; Tu, Citation1981; Wilson, Citation1970). In our previous study, we observed that the significantly improved nodulation of En-b0-1 (a super-nodulating genotype) over its normal parent cultivar was associated with greater tolerance under saline conditions (Yasuta & Kokubun, Citation2014).
Although substantial research has demonstrated that soybean exhibits considerable genotypic differences in salt tolerance (Ghassemi-Golezani et al., Citation2009; Hakeem et al., Citation2012; Karim et al., Citation2012; Lee et al., Citation2008; Mannan et al., Citation2012; Parker et al., Citation1983; Yang & Blanchar, Citation1993), the cultivars used in these studies were selected solely based on agro-morphological traits. In the present study, we examined genotypic variation in salinity tolerance among 85 soybean genotypes which were selected based on an assessment of both single nucleotide polymorphisms (SNP) markers and agro-morphological traits, and may thus provide useful information for the genetic improvement of salt tolerance (Kaga et al., Citation2012).
Therefore, in this study, we aimed to examine the genotypic variation in salinity tolerance among 85 representative soybean genotypes, and to identify whether differences in salt tolerance are linked to corresponding differences in nodulation and N uptake.
Materials and methods
Plant materials
For the purposes of this study, we used a subset of the world soybean mini-core collection (80 cultivars). This collection was developed by NIAS (National Institute of Agrobiological Sciences, Tsukuba, Japan), based on agro-morphological and SNP data from a global soybean collection maintained at the NIAS Genebank (Kaga et al., Citation2012). This sub-set has various seed traits, including color (yellow, green, brown, and black), size, and shape (round and oval). For comparison, we added a further five genotypes: Enrei, En-b0-1, En1282, NILs72-T, and NILs72-S. Enrei is among the major cultivars grown in the eastern part of Japan. En-b0-1 and En1282 are super-nodulating and non-nodulating genotypes, respectively, and are the progeny of Enrei × En6500, an ethyl methylsulfonate-induced Enrei mutant (Akao & Kouchi, Citation1992). Even under saline conditions, En-b0-1 forms significantly more nodules and thus exhibits higher N2 fixation activity than Enrei, traits that partly contribute to its higher salinity tolerance (Yasuta & Kokubun, Citation2014). Finally, NILs72-T and NILs72-S are salt-tolerant and -sensitive progenies, respectively, of an RIL population resulting from a cross between salt-tolerant FT-Abyara and salt-sensitive C01 (Do et al., Citation2016; Hamwieh et al., Citation2011).
The experiments were conducted at the Graduate School of Agricultural Science, Tohoku University (38°1′6 N, 140°50′E). Experiments were performed in 2013 and 2015, with the only difference being a 17-day shift in sowing time between the two years. Plants were potted in a greenhouse with four open sides. Prior to sowing, fertilizer was applied at fixed rates and mixed with soil: 0.05 g of N, 0.15 g of P2O5, 0.2 g of K2O, 1 g of fused phosphate, 2 g of superphosphate of lime, and 2 g of slaked lime per liter of low-humic Andosol field soil [N: 0.55%, cation-exchange capacity (CEC): 44.9 cmol kg−1]. The seeds were inoculated with J1065, a strain of Bradyrhizobium japonicum obtained from Tokachi Nokyoren (Obihiro, Japan). On 16 May 2013 and 2 June 2015, 7–10 seeds per genotype were sown in paper pots (3.5 cm length × 3.5 cm width × 3.8 cm height), at a rate of one seed per pot. At the first trifoliate stage, medium-sized plants from each genotype were transplanted to plastic pots (10 cm length × 10 cm width × 15 cm height) for saline treatment, at a rate of one plant per pot. A total of 510 pots (3 plants × 85 genotypes × 2 treatments) were employed in each year. When not undergoing saline treatment, plants were regularly irrigated with tap water. Preventative insecticides were applied as needed during plant culture.
Air temperature around the canopy of the plants was monitored every hour, using a thermorecorder (TR-52S; T and D, Matsumoto, Japan). The daily mean air temperature during the experiments (from sowing to harvest) was higher in 2015 (22.7 °C) than in 2013 (19.9 °C). Data regarding solar radiation during the experiments were obtained from the website of Sendai Regional Headquarters, Japan Meteorological Agency, which is located approximately 3 km apart from the experimental site. The daily mean solar radiation was 18.3 and 17.4 MJm−2 in 2013 and 2015, respectively. Solar radiation inside the greenhouse was 10% lower than outside the house.
Saline treatment
Pots containing plants were placed in quadrilateral boxes (135 cm length × 91 cm width × 20 cm depth) containing saline water of 10 cm depth, which allowed plants to take up water through the hole at the bottom of each pot. Saline treatments were initiated at the second trifoliate compound leaf expansion stage. During the first week, 50 mM NaCl was added, followed by 100 mM in the second week, and 150 mM in the third week. The final NaCl concentration was determined based on previous studies that clearly distinguished cultivar differences in salinity tolerance after treatments with 100–150 mM NaCl (Lee et al., Citation2008; Valencia et al., Citation2008; Yasuta & Kokubun, Citation2014). Control plants were supplied with tap water using the same box setup as the saline treatments. Saline and tap water were renewed every few days.
Measurements and data analyses
Leaf chlorophyll content of the last expanded leaf was measured at several-day intervals during the saline treatment, using a chlorophyll meter (SPAD 502; Minolta Inc., Tokyo, Japan). After the 150 mM NaCl treatment, three or four plants per genotype were sampled, and separated into shoots and roots + nodules. Samples were oven-dried at 80 °C for 3 days, and then weighed. Subsequently, nodules were detached from roots, counted, and weighed. Dried samples of shoots were ground in a mill and N content was analyzed using an automated CN analyzer (JM1000CN; J-Science Lab Co., Ltd., Kyoto, Japan).
To evaluate salinity tolerance, we used the ratio of saline-treated (S) to control (C) plant total dry weight [DW(S/C)]. Correlations between total DW(S/C) and other traits associated with nodulation and N uptake (nodule number, nodule weight per plant/nodule, root weight) were determined.
Significant differences between genotypes, saline treatments, and years in traits were tested with ANOVA in JMP Statistical Discovery software (SAS Institute, Cary, NC, U.S.A.); individual means were compared with the Tukey’s test. The genotypic ranking for total DW(S/C) between the two years was determined with Spearman’s rank correlations.
Results
Genotypic variation in salinity tolerance
Analysis of variance (ANOVA) revealed that genotype, saline treatment, and year had a significant effect on total DW and the traits associated with nodulation (nodule number, nodule weight per plant/nodule) (Table ), indicating that there exists a substantial genotypic variation among the diverse genetic resources in the responses of these traits responding to saline treatment. In addition, the analysis revealed interactive effects (genotype × treatment or year) on these traits, suggesting that the magnitude and manner of the genotypic variations are affected by saline condition and year.
Table 1. Summary of ANOVA for total dry weight (DW) and traits associated with nodulation of 85 soybean genotypes.
A marked genotypic variation was observed in the total DW(S/C) in both years (2013, 2015). The data for the two years showed similar frequency distributions, but the values were generally greater in 2013 than in 2015 (Figure ). Salinity tolerance rankings for genotypes, evaluated by total DW(S/C), were stable across years, in which sowing times and climatic conditions differed (Spearman’s rank correlations; p < 0.01).
Figure 1. Genotypic variation in salinity tolerance among 85 soybean genotypes subjected to saline conditions (final concentration of 150 mM NaCl). Salinity tolerance was evaluated as the ratio of saline-treated (S) to control (C) plant total dry weight [DW (S/C)]. The number of genotypes vs. total DW (S/C) for two years (2013, 2015) is shown.
![Figure 1. Genotypic variation in salinity tolerance among 85 soybean genotypes subjected to saline conditions (final concentration of 150 mM NaCl). Salinity tolerance was evaluated as the ratio of saline-treated (S) to control (C) plant total dry weight [DW (S/C)]. The number of genotypes vs. total DW (S/C) for two years (2013, 2015) is shown.](/cms/asset/54be16d0-db34-470a-b56b-cc02788d37fd/tpps_a_1360140_f0001_b.gif)
Table lists all the genotypes examined in order of total DW(S/C) with their geological origin. We found that genotypes with both superior and inferior total DW(S/C) originated from vast geographical regions, including India, China, South-East Asia, and eastern Asia (Table ). The ranking for NILs72-T, which was previously evaluated as salt-tolerant, was 9th, whereas that of NILs72-S was 46th. When comparing the morphology of tolerant versus sensitive genotypes under saline conditions, leaf wilting, and plant growth retardation were more pronounced in the latter (Figure ).
Table 2. The ratio of saline-treated (S) to control (C) plant total dry weight [DW (S/C)] of all the genotypes subjected to saline treatment (final concentration of 150 mM NaCl) for two years (2013, 2015) and their country of origin.
Relationship between salinity tolerance and traits involved in nodulation and N uptake
Across both years, the ratios of nodule number and nodule dry weight per plant/nodule (S/C) were positively correlated with total DW(S/C), except for nodule number in 2013 (Figure ). Furthermore, root DW (S/C) was positively correlated with shoot DW(S/C) across both years (Figure ).
Figure 3. Relationship between total dry weight [DW (S/C)] and nodule number, nodule weight per plant/nodule, among 85 soybean genotypes subjected to saline conditions. All the data are expressed as the ratio of saline-treated (S) to control (C) plants (S/C). Diagonal lines indicate the regressions for each year (2013, 2015).
![Figure 3. Relationship between total dry weight [DW (S/C)] and nodule number, nodule weight per plant/nodule, among 85 soybean genotypes subjected to saline conditions. All the data are expressed as the ratio of saline-treated (S) to control (C) plants (S/C). Diagonal lines indicate the regressions for each year (2013, 2015).](/cms/asset/97aa7554-45df-4b9d-9bca-2ff4bdb766cc/tpps_a_1360140_f0003_b.gif)
Figure 4. Relationship between shoot DW (S/C) and root DW (S/C) among 85 soybean genotypes subjected to saline conditions. All the data are expressed as the ratio of saline-treated (S) to control (C) plants (S/C). Diagonal lines indicate the regressions for each year (2013, 2015).
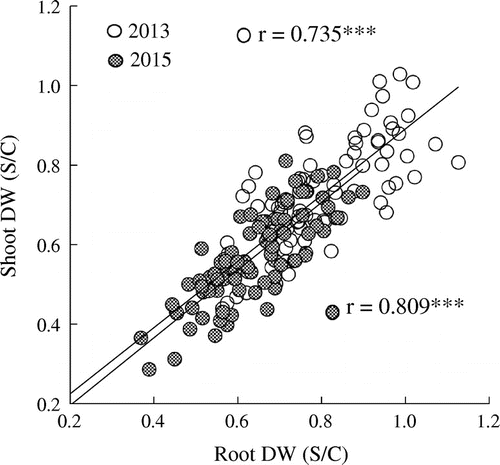
Regarding N uptake, total DW (actual value) was negatively correlated with shoot N concentration (actual value), but positively correlated with shoot N content (actual value) under both control and saline conditions (Figure ). Significant correlations were observed regardless of treatment. Leaf N content (S/C) was positively correlated with SPAD reading (S/C) (Figure ). Table compares total DW and shoot N content [actual value and its ratio (S/C)] between salinity-tolerant and -sensitive genotypes, which ranked among the top 20 and bottom 20 in total DW(S/C) for the two years (refer to Table ). Under saline conditions, salt-tolerant genotypes exhibited superior shoot N content [in both actual value and its ratio (S/C)].
Figure 5. Relationship between total dry weight (DW) and shoot nitrogen (N) concentration, and shoot N content among 85 soybean genotypes. All the data are actual values of the plants grown under the respective conditions (Control, Saline) in 2015. Diagonal lines indicate the regressions for each relationship.
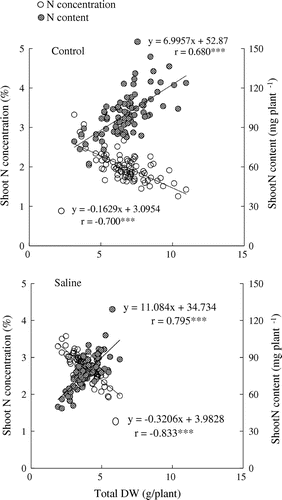
Figure 6. Relationship between leaf N content (S/C) and leaf SPAD reading (S/C) among 85 soybean genotypes grown under saline conditions. All the data are expressed as the ratio of saline-treated (S) to control (C) plants (S/C) in 2015. Diagonal line indicates the regression.
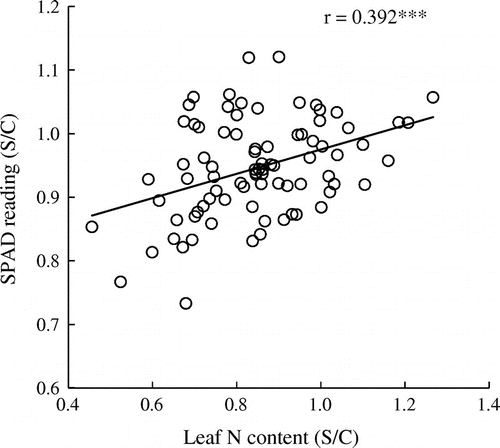
Table 3. Total dry weight (DW) and shoot nitrogen content (NC) of salinity-tolerant and -sensitive genotypes subjected to saline conditions.
Discussion
That saline stress during the vegetative growth stage can markedly reduce dry matter production and yield in soybean has been well documented (Abel & MacKenzie, Citation1964; Parker et al., Citation1983; Wang & Shannon, Citation1999; Yang & Blanchar, Citation1993). In this study, we therefore evaluated salinity tolerance via the ratio of saline-treated to control plant total dry weight [DW(S/C)] during the vegetative growth stage.
The primary objective of this study was to evaluate the genotypic variation in biomass production among diverse soybean genotypes under saline conditions. The results revealed a marked genotypic variation in the total DW(S/C), with the ratios of the top several tolerant genotypes being more than 0.8, whereas those of the most sensitive genotypes were less than 0.5 (Figure , Table ). The tolerant genotypes screened in the present study will have applications as genetic resources for breeding programs, whereas both tolerant and sensitive genotypes could be used as experimental materials in future physiological studies on salinity tolerance. Importantly, we were able to identify several genotypes more tolerant than NILs72-T and En-b0-1 (Table ), both of which were previously identified as salt-tolerant (Do et al., Citation2016; Hamwieh et al., Citation2011; Yasuta & Kokubun, Citation2014), suggesting the possibility of identifying further tolerant cultivars.
We had expected that tolerant genotypes would originate from regions where salinity is a serious issue hampering soybean production. However, there was a considerable overlap in the countries of origin of tolerant and sensitive genotypes, ranging from India, through several South-Eastern Asian nations, to Korea. In addition, the agro-morphological traits (e.g. growth habit, seed shape/color/size, and maturity group) of both tolerant and sensitive genotypes were diverse.
The other objective of this study was to clarify whether nodulation and N uptake are associated with the genotypic variation in salinity tolerance. Here, we observed a marked genotypic difference [associated with the variation in DW(S/C)] in the nodule number and nodule dry weight per plant/nodule, all parameters associated with N2-fixation capacity (Figure ). In addition, a positive correlation was observed between SPAD reading (S/C) and leaf N content (S/C) among genotypes (Figure ). The positive correlation between SPAD reading (S/C) and leaf N content (S/C), along with the greater greenness of salt-tolerant genotypes under saline conditions (Figure ), indicates the possibility that a leaf chlorophyll meter could be used for an instantaneous assessment of the salinity tolerance of soybean genotypes.
In our previous study, we found that En-b0-1, a super-nodulating genotype, exhibits both greater N2-fixing capacity and better salinity tolerance than its parental genotype Enrei, suggesting that nodulation capacity is partly responsible for salinity tolerance in soybean (Yasuta & Kokubun, Citation2014). In the present study, the non-nodulating genotype En1282 ranked higher than the super-nodulating genotype En-b0-1 and the normal nodulating genotype (Enrei). The reason for the inconsistency between the results of our present and previous studies is currently not clear.
Root and shoot DW(S/C) were closely correlated among genotypes (Figure ), suggesting that non-symbiotic N uptake by the root system might contribute to the greater total DW(S/C). Thus, our data do not allow us to conclude whether symbiotic N2 fixation or non-symbiotic N2 absorption is more important for the enhanced N uptake of salt-tolerant cultivars. Nonetheless, the close correlation between total DW and shoot N content under saline conditions (Figure ) and the greater shoot N content (actual value and S/C) of salt-tolerant genotypes (Table ) suggest that superior N uptake is crucial to better dry matter production under saline conditions. However, there is a possibility that salt tolerance resulted in superior N uptake, rather than superior N uptake being responsible for the tolerance. Thus, on the basis of the results of the present study, it is not possible to conclusively determine the causal relationship between salt tolerance and N uptake in soybean.
Previous studies on Glycine salinity tolerance have identified inter-specific differences that are associated with the capacity of tolerant species to prevent excessive Na or Cl accumulation in leaves (Kao et al., Citation2003, 2006; Lenis et al., Citation2011; Luo et al., Citation2005). Similarly, our previous study revealed that improved nodulation was able to prevent excessive ion accumulation in shoots, thereby contributing to greater salinity tolerance (Yasuta & Kokubun, Citation2014). Thus, the salinity tolerance of both wild and cultivated soybean is largely dependent on the ability to control ion (i.e. Na+ and Cl−) distribution in plant parts. Regarding the mechanisms regulating Na/Cl distribution in plants, Do et al. (Citation2016) isolated a gene (Ncl) from a salt-tolerant soybean cultivar (FT-Abyara), which synchronously regulates ion (Na+, K+, and Cl−) transport and accumulation. They showed that higher Ncl expression in the root decreased ion accumulation in the shoot under saline conditions. We are currently investigating whether this Ncl mechanism is associated with the genotypic variation in salinity tolerance among our studied genotypes.
Another physiological process that is probably involved in soybean salt tolerance is osmotic adjustment capacity under saline conditions. Under saline treatment, the salt tolerant AGS313, screened from 170 cultivars/lines, was able to maintain higher leaf water potential than other genotypes (Karim et al., Citation2012; Mannan et al., Citation2012). This cultivar also produced more biomass under saline conditions, and the authors ascribed its superior biomass production to the plant’s enhanced osmotic adjustment, which may enable the maintenance of higher photosynthetic activity. Thus, the mechanisms regulating soybean salinity tolerance are multifaceted. Although further studies are needed to clarify the overall processes underlining soybean salinity tolerance, this study has uncovered multiple salt-tolerant genotypes that can be employed as suitable materials for both breeding programs and future research.
Disclosure statement
No potential conflict of interest was reported by the authors.
Funding
This study was partly supported by a Grant-in-Aid for Scientific Research (No. 24405021) from Japan Society for the Promotion of Science (JSPS).
References
- Abel, G. H., & MacKenzie, A. J. (1964). Salt tolerance of soybean varieties (Glycine max L. Merrill) during germination and later growth. Crop Science, 4, 157–161.10.2135/cropsci1964.0011183X000400020010x
- Akao, S., & Kouchi, H. (1992). A super-nodulating mutant isolated from soybean cultivar Enrei. Soil Science and Plant Nutrition, 38, 183–187.10.1080/00380768.1992.10416966
- Balasubramanian, V., & Sinha, S. K. (1976). Effects of salt stress on growth, nodulation and nitrogen fixation in cowpea and mung beans. Physiologia Plantarum, 36, 197–200.10.1111/ppl.1976.36.issue-2
- Chen, H. T., Cui, S. Y., Fu, S. X., Gai, J. Y., & Yu, D. Y. (2008). Identification of quantitative trait loci associated with salt tolerance during seedling growth in soybean (Glycine max L.). Australian Journal of Agricultural Research, 59, 1086–1091.10.1071/AR08104
- Do, T. D., Chen, H., Vu, V. T. T., Hamwieh, A., Yamada, T., Sato, T., … Xu, D. (2016). Ncl synchronously regulates Na+, K+, and Cl − in soybean and greatly increases the grain yield in saline field conditions. Scientific Reports, 6, 157. doi:10.1038/srep19147
- Flowers, T. J., & Flowers, S. A. (2005). Why does salinity pose such a difficult problem for plant breeders? Agricultural Water Management, 78, 15–24.10.1016/j.agwat.2005.04.015
- Ghassemi-Golezani, K., Taifeh-Noori, M., Oustan, S., & Moghaddam, M. (2009). Responses of soybean cultivars to salinity stress. Journal of Food, Agriculture and Environment, 7, 401–404.
- Guan, R., Chen, J., Jiang, J., Liu, G., Liu, Y., Tian, L., … Qiu, L. (2014a). Mapping and validation of a dominant salt tolerance gene in the cultivated soybean (Glycine max) variety Tiefeng 8. The Crop Journal, 2, 358–365.10.1016/j.cj.2014.09.001
- Guan, R., Qu, Y., Guo, Y., Yu, L., Liu, Y., Jian, J., … Qiu, L. (2014b). Salinity tolerance in soybean is modulated by natural variation in GmSALT3. The Plant Journal, 80, 937–950.10.1111/tpj.12695
- Hakeem, K. R., Khan, F., Chandna, R., & Siddiqui, T. O. M. (2012). Genotypic variability among soybean genotypes under NaCl stress and proteome analysis of salt-tolerant genotypes. Applied Biochemistry and Biotechnology, 168, 2309–2329.10.1007/s12010-012-9939-6
- Hamwieh, A., & Xu, DH. (2008). Conserved salt tolerance quantitative trait locus (QTL) in wild and cultivated soybeans. Breeding Science, 58, 355–359.10.1270/jsbbs.58.355
- Hamwieh, A., Tuyen, D. D., Cong, H., Benitez, E. R., Takahashi, R., & Xu, D. H. (2011). Identification and validation of a major QTL for salt tolerance in soybean. Euphytica, 179, 451–459.10.1007/s10681-011-0347-8
- Jones, R. G. W. (1981). Salt tolerance. In C. B. Johnson (Ed.), Physiological processes limiting plant physiology (pp. 271–292). London: Butterworths.
- Kaga, A., Shimizu, T., Watanabe, S., Tsubokura, Y., Katayose, Y., Harada, K., … Tomooka, N. (2012). Evaluation of soybean germplasm conserved in NIAS genebank and development of mini core collections. Breeding Science, 61, 566–592.10.1270/jsbbs.61.566
- Kao, W. Y., Tsai, T. T., & Shih, C. N. (2003). Photosynthetic gas exchange and chlorophyll a fluorescence of three wild soybean species in response to NaCl treatments. Photosynthetica, 41, 415–419.10.1023/B:PHOT.0000015466.22288.23
- Kao, W. Y., Tsai, T. T., Tsai, H. C., & Shih, C. N. (2006). Responses of three Glycine species to salt stress. Environmental and Experimental Botany, 56, 120–125.10.1016/j.envexpbot.2005.01.009
- Karim, M. A., Kondo, T., Ueda, K., Higuchi, H., & Nawata, E. (2012). Effect of NaCl treatment on growth and some physiological characteristics of a salt-tolerant soybean genotype AGS 313 bred in Bangladesh. Tropical Agriculture and Development, 56, 139–142.
- Lakshmi-Kumari, M., Singh, C. S., & Subba-Rao, N. S. (1974). Root hair infection and nodulation in lucerne (Medicago sativa L.) as influenced by salinity and alkalinity. Plant and Soil, 40, 261-268.10.1007/BF00011509
- Lauter, D. J., Munns, D. N., & Clarkin, K. L. (1981). Salt response of chickpea as influenced by N supply. Agronomy Journal, 73, 961–966.10.2134/agronj1981.00021962007300060013x
- Lee, G. J., Boerma, H. R., Villagarcia, M. R., Zhou, X., Carter, Jr., T. E., Li, Z., & Gibbs, MO. (2004). A major QTL conditioning salt tolerance in S-100 soybean and descendent cultivars. Theoretical and Applied Genetics, 109, 1610–1619.10.1007/s00122-004-1783-9
- Lee, J. D., Smothers, S. L., Dunn, D., Villagarcia, M., Shumway, C. R., Carter, T. E., & Shannon, J. G. (2008). Evaluation of a simple method to screen soybean genotypes for salt tolerance. Crop Science, 48, 2194–2200.10.2135/cropsci2008.02.0090
- Lenis, J. M., Ellersieck, M., Blevins, D. G., Sleper, D. A., Nguyen, H. T., Dunn, D., … Shannon, J. G. (2011). Differences in ion accumulation and salt tolerance among glycine accessions. Journal of Agronomy and Crop Science, 197, 302–310.10.1111/jac.2011.197.issue-4
- Luo, Q., Yu, B., & Liu, Y. (2005). Differential sensitivity to chloride and sodium ions in seedlings of Glycine max and G. soja under NaCl stress. Journal of Plant Physiology, 162, 1003–1012.10.1016/j.jplph.2004.11.008
- Mannan, M. A., Karim, M. A., Haque, M. M., Khaliq, Q. A., Higuchi, H., & Nawata, E. (2012). Response of soybean to salinity: I. Genotypic variations in salt tolerance at the vegetative stage. Tropical Agriculture and Development, 56, 117-122.
- Munns, R., & Tester, M. (2008). Mechanisms of salinity tolerance. Annual Review of Plant Biology, 59, 651–681.10.1146/annurev.arplant.59.032607.092911
- Neumann, P. (1997). Salinity resistance and plant growth revisited. Plant, Cell & Environment, 20, 1193-1198.10.1046/j.1365-3040.1997.d01-139.x
- Niknam, S. R., & McComb, J. (2000). Salt tolerance screening of selected Australian woody species – A review. Forest Ecology and Management, 139, 1–19.10.1016/S0378-1127(99)00334-5
- Parker, M. B., Gascho, G. J., & Gaines, T. P. (1983). Chloride toxicity of soybeans grown on Atlantic Coast flatwoods soils. Agronomy Journal, 75, 439–443.10.2134/agronj1983.00021962007500030005x
- Patil, G., Do, T., Vuong, T. D., Valliyodan, B., Lee, J., Chaudhary, J., … Nguye, H. (2016). Genomic-assisted haplotype analysis and the development of high-throughput SNP markers for salinity tolerance in soybean. Scientific Reports, 6, 244. doi:10.1038/srep19199
- Qi, X., Li, M., Xie, M., Liu, X., Ni, M., Shao, G., … Lam, H. (2014). Identification of a novel salt tolerance gene in wild soybean by whole-genome sequencing. Nature Communications, 5, 4340. doi:10.1038/ncomms5340
- Shannon, M. C. (1997). Adaptation of plants to salinity. Advances in Agronomy, 60, 75–120.10.1016/S0065-2113(08)60601-X
- Singleton, P. W., & Bohlool, B. B. (1984). Effect of salinity on nodule formation by soybean. Plant Physiology, 74, 72–76.10.1104/pp.74.1.72
- Tu, J. C. (1981). Effect of salinity on rhizobium-root-hair interaction, nodulation and growth of soybean. Canadian Journal of Plant Science, 61, 231–239.10.4141/cjps81-035
- Tuyen, D. D., Lal, S. K., & Xu, D. H. (2010). Identification of a major QTL allele from wild soybean (Glycine soja Sieb. & Zucc.) for increasing alkaline salt tolerance in soybean. Theoretical and Applied Genetics, 121, 229–236.10.1007/s00122-010-1304-y
- Valencia, R., Chen, P., Ishibashi, T., & Conatser, M. (2008). A rapid and effective method for screening salt tolerance in soybean. Crop Science, 48, 1773–1779.10.2135/cropsci2007.12.0666
- Wang, D., & Shannon, M. C. (1999). Emergence and seedling growth of soybean cultivars and maturity groups under salinity. Plant and Soil, 214, 117–124.10.1023/A:1004719420806
- Wilson, J. R. (1970). Response to salinity in Glycine. VI. Some effects of a range of short-term salt stresses on the growth, nodulation, and nitrogen fixation of Glycine wightii. Australian Journal of Agricultural Research, 21, 571–582.10.1071/AR9700571
- Xu, D., & Tuyen, D. D. (2012). Genetic studies on saline and sodic tolerances in soybean. Breeding Science, 61, 559–565.10.1270/jsbbs.61.559
- Yang, J., & Blanchar, R. W. (1993). Differentiating chloride susceptibility in soybean cultivars. Agronomy Journal, 85, 880–885.10.2134/agronj1993.00021962008500040019x
- Yasuta, Y., & Kokubun, M. (2014). Salinity tolerance of super-nodulating soybean genotype En-b0-1. Plant Production Science, 17, 32–40.10.1626/pps.17.32
- Zhang, J. I., Flowers, T. J., & Wang, S. M. (2010). Mechanisms of sodium uptake by roots of higher plants. Plant and Soil, 326, 45–60.10.1007/s11104-009-0076-0