Abstract
Drought stress which often occurs during early growth stage is one constraint in sugarcane production. In this study, the response of sugarcane to drought and nitrogen application for physiological and agronomical characteristics was investigated. Two water regimes (well-watered and drought stress from 60 to 120 day after transplanting) and four nitrogen levels (0, 4.4, 8.8 and 13.2 g pot−1 equivalent to 0, 90, 180 and 270 kg ha−1, respectively) were assigned in a Split-plot design with three replications. The results showed that photosynthetic responses to light intensity and intercellular CO2 concentrations of sugarcane were different between fertilized and non-fertilized treatments. Photosynthetic rates of 180 and 270 N treatments, normally, were significantly higher than that of 90 N, but not significant at drought conditions. Photosynthetic rates of 0 N treatment were the lowest under both conditions. Higher nitrogen application supported higher photosynthetic rate, stomatal conductance, and chlorophyll content because of higher nitrogen concentration accumulated into the leaf. Drought significantly reduced the potential photosynthetic rate, stomatal conductance, SPAD, leaf area, and biomass production. Higher nitrogen applications with larger root system could support higher photosynthetic activities to accumulate more dry mass. Strong positive coefficient between photosynthetic and biomass nitrogen use efficiency and drought tolerance index may suggest that higher nitrogen use efficiency could help plants have higher ability to tolerate drought stress.
Classification:
Abbreviations:
- A; photosynthetic rate
- Amax; potential photosynthetic rate
- Ca; ambient CO2 concentration
- Ci; intercellular CO2 concentration
- DAT; days after transplanting
- DTI; drought tolerant index
- gs; stomatal conductance
- IS; initial slope of A/Ci curve
- NL; specific leaf nitrogen content
- NU; Enitrogen use efficiency
- PEP; Casephosphoenolpyruvate carboxylase
- PFD; photon flux density
- PNUE; photosynthetic nitrogen use efficiency
- PWUE; photosynthetic water use efficiency; Rubisco, ribulose bisphosphate carboxylase oxygenase
Drought stress is one of the main factors constraining sugarcane production in over the world. Water deficit which restricts growth and photosynthetic activity (Barbosa et al., Citation2015; Graça et al., Citation2010; Jangpromma et al., Citation2010) is responsible for the reduction in biomass and cane yield (Ramesh, Citation2000; Zhao & Li, Citation2015). However, the effects of drought stress were varying according to crop growth stages. Drought stress at early and mid-season stages reduced growth, cane and sugar yield, whereas cane and sugar yield produced when water stress was imposed at the late season stage was not significantly from yields obtained at full irrigation (Ethan et al., Citation2016). Tillering and grand growth phases were critical stages of drought sensitivity due to the high water requirement for sustainable growth in sugarcane (Zingaretti et al., Citation2012). Although grand growth stage is the longest stage with highest water requirement, by crop season management it is often fixed closely during rainy season, hence, the effect of drought stress is diminished. Therefore, water stress at tillering and early grand growth (early growth stage) become more frequently.
Nitrogen plays an important role in sugarcane crops. It is involved in many critical processes such as plant growth, expansion of green leaves, and tiller or sucker production, especially in the formation of plant protein which is essential for photosynthesis such as PEPCase or Rubisco. The growth and yield of sugarcane decrease due to nitrogen deficiency, but excess nitrogen can lead to prolonged vegetative growth and reduced sugar yield and quality (Bell, Citation2014). Higher NUE can support better growth and higher crop yield. For instance, increase nitrogen uptake and NUE in rice contributed to the increase in grain yield (Zhu et al., Citation2016). In sugarcane, positive association was found between NUE traits with sugar yield and cane yield (Acreche, Citation2017). Similarly, Calif and Edgecombe (Citation2015) showed remarkable improvements in plant growth and biomass production in sugarcane lines incorporating NUE traits.
Drought stress restricted nutrient uptake may cause a deficiency of nutrient as well as nitrogen (Dinh et al., Citation2014). Unfortunately, nitrogen is often applied at early stage and finished before plant elongating. Therefore, uptake of nitrogen and NUE may be fallen due to water stress during early growth stage. In maize, drought tolerant cultivars produced consistently higher yields because that these cultivars had either high nitrogen uptake and nitrogen utilization efficiency (Kamara et al., Citation2014). In sweet sorghum, improved water and nitrogen use efficiencies under water stress may both contribute to the high degree of physiological acclimation to drought (Wang et al., Citation2014). Therefore, higher NUE should be a strategy to confront with the compromise from a shortage of water source. However, still no information has been reported on NUE under drought period in sugarcane.
The present study focuses on the photosynthetic and agronomical responses of sugarcane to drought stress conditions under different nitrogen applications, and the relationship between NUE under drought condition with drought tolerant index to get a better understanding of the mechanism of drought stress tolerance in sugarcane.
Materials and methods
The experiment was conducted under glasshouse conditions at the University of the Ryukyus, Okinawa, Japan (26°25′ N, 127°45′ E; altitude 126 m) from May to September 2016. Two months old seedlings of commercial cultivar NiF8 were transplanted into Wagner pots (1/2000a) filled with 10 kg substrate of red soil: sea sand: peat moss (1:1:1, v v−1) at 12% of soil moisture content. The substrate properties were analyzed before transplanting with pH (7.1), electronic conductivity (153.1 mS m−1), total N (.07%), P (.1 ppm) and K (12.2 ppm).
A Split-plot design was used with three replications. Two soil water regimes including well-watered (WW) at field capacity and drought stress (DS) at 1/3 of available water for 60 days were assigned in main plots. In subplots, four nitrogen levels including 0, 4.4, 8.8 and 13.2 gN pot−1 (equivalent to 0, 90,180 and 270 kg N ha−1 in field conditions, respectively) were designed.
Experimental pots were arranged in distance 40 × 90 cm of each pot and row. During experiment period, all tillers were removed immediately after emergence. At 7 DAT, superphosphate at rate 5.2 g pot−1 was applied. Potassium chloride (2.1 g pot−1) and ammonium sulfate were fertilized at the same ratio of 1:1.5:1:1.5 at 7, 30, 60 and 90 DAT, respectively.
After transplanting, irrigation with full available water was practiced until 60 DAT. After that at the moisture stress plot, water was applied by just 50% of water loss until soil moisture content reaching to 1/3 available water (it took 10 days), then by full available water loss of this treatment for another 50 days. Pot weight of each treatment was determined every day using a gravimetric balance to calculated amounts of water loss. Soil moisture content (37.19% of field capacity and 28.36% of 1/3 available water) was monitored by using Hydra probe soil sensors (Steven Water Monitoring Systems, Inc., Portland, OR) at 10 cm of depth with corresponding values for field capacity and 1/3 available water were .40 and .29 m3 m−3 (Figure ).
Figure 1. Weather conditions (a) and soil moisture content (b) at well-watered (WW) and drought stress (DS) treatments during the experimental period.
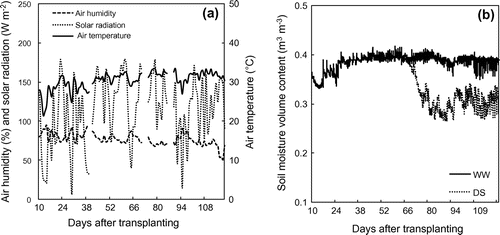
Data collections
An open gas exchange system, in detail described by Kawamitsu et al. (Citation2002), were used to determine photosynthetic light response curve at PFD (50–1500 μmol m−2 s−1, at 400 μmol mol−1 CO2) and A/Ci curve at various CO2 concentration (30–1000 μmol mol−1 CO2, at 1500 μmol m−2 s−1 of PFD). At one week before the start and complete of drought stress period, one sample plant of each treatment was taken randomly. The first fully expanded leaf from the top of each sampled plant was set in a flexible chamber (26 × 30 × 9 cm). Leaf temperature was controlled at 30 ± 1 °C.
From 5 DAT, the growth parameters including plant height and total leaves number of each treatment were collected at each one-week interval. At one day before the start and complete of drought treatment, the first fully expanded leaves of sample plants of each treatment in all replications were taken to determine potential photosynthetic rate (Amax), stomatal conductance (gs) and transpiration rate by using Li-6400 portable photosynthesis system (Li-COR, Lincoln, Nebraska, USA) equipped with a 2 × 3 cm2 LED chamber between 0900 to 1500 at a PFD of 2000 μmol m−2 s−1, leaf temperature of 31 ± 2 °C, CO2 concentration of 400 ± 5 μmol mol−1. After photosynthesis measurement, SPAD values were recorded at the same positions using a SPAD meter (SPAD-502, Minolta, Japan).
The first leaf of each sampled plant was cut to determine leaf area by using Li-3100 portable leaf area meter (Li-COR, Lincoln, Nebraska, USA), then oven-dried at 80 °C for 48 h to determine dry weight. After grinding by TI-100 vibrating sample mill (CMT, Tokyo, Japan), 25 mg of dry leaf sample was taken to determine specific leaf nitrogen content (NL) by using an N/C analyzer (NC-90A, Shimadzu, Japan).
Photosynthetic nitrogen use efficiency (PNUE) and photosynthetic water use efficiency (PWUE) was then calculated by the following formula:
At 120 DAT when drought stress treatment was completed, the whole plant was cut by separating leaves, stem, and root. The stem (after squeezing) and leaves (after scanning by Li-3100) were dried at 80 °C for 48h to determine shoot dry weight. The root was cleaned by tap water, then dried at 80 °C for 48 h to determine root mass. Total biomass was calculated by the sum of the shoot and root dry weight. Then, biomass nitrogen use efficiency (NUE) was calculated by following:
Drought tolerant index (DTI) was determined as follows:
Statistical analysis
The data were subjected to analysis of variance according to a Split-plot and Randomized Complete Block Design using Statistix 8.0 package. Tukey test was used to compare the means. Correlation coefficients among photosynthetic traits and biomass were calculated to assess the relationships.
Results and discussion
Methodological conditions and soil moisture content
Methodological conditions and soil moisture volume fraction in the experimental site were shown in Figure . During the experimental time, the air temperature and air humidity in glass house ranging from 20.0 to 42.0 °C and from 86.6 to 98.3% were higher at 2.04 ± .7 °C and 12 ± 2.5% than those outside, respectively. Average daily solar radiation ranged from 10 to 180 W m−2, which was as .53 times as this parameter at outside condition. Soil moisture content of control treatment fluctuated around .38 m3 m−3. Meanwhile, in stress treatments, soil moisture content stabled around .38 m3 m−3 until 60 DAT (before stress treatment), then reduced rapidly and changed around .29 m3 m−3 from 70 to 120 DAT. During late period of drought stress, soil moisture was more fluctuated in both control and water stress treatments because of higher air temperature but lower air humidity, and also since higher water requirement to compensate for water loss from larger growth plant.
Photosynthetic responses of sugarcane to light intensity and CO2 concentration
An initial slope of photosynthetic light response curve showed that photosynthesis is limited by the concentration of chlorophyll and the efficiency of light dependent reactions, but in higher levels, it is limited by the efficiency of Rubisco and the availability of CO2. As can be seen from Figure , there were upward trends in A when light intensity increased at all nitrogen levels and both water regimes. In fact, at fertilized treatment, A rose rapidly, then slowly when PFD reached to approximately 1000 μmol m−2 s−1. The same trends were found in previous studies in another C4 plant Amaranthus retroflexus (Sage & Pearcy, Citation1987), and sugarcane (Allison et al., Citation1997; Sage, Citation2014) under normal and mild stress conditions (Zhao et al., Citation2013).
Figure 2. Light and A/Ci response curves at 60 DAT (a, b); and at 120 DAT under well-watered (closed shapes) (c, d) and drought stress (opened shapes) (e, f) conditions with different nitrogen application levels.
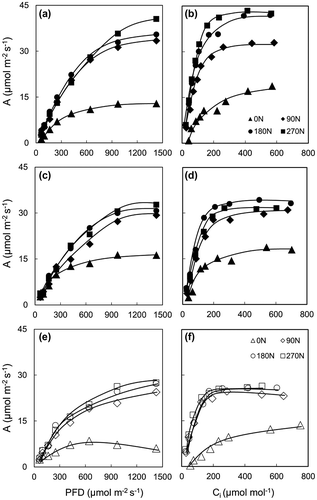
An A/Ci curve shows the photosynthetic response of leaf tissue to varying intercellular CO2 concentrations. The shape of photosynthesis is limited by Rubisco capacity on the initial slope and by RuBP regeneration capacity (C3 plant) at saturated CO2 concentration; whereas at C4 plant it is limited by PEPCase on the initial slope, but very complex at CO2 saturation with effects by Rubisco, RuBP generation or PEP regeneration capacity (Sage, Citation2014). A/Ci curve shown in Figure illustrated that A increased when Ci increased at all nitrogen levels and both water regimes. In fact, A increased rapidly then became stable when Ci reached to approximately 200 μmol mol−1. However, 200 μmol mol−1 seems to be the saturated point of Ci just in case of the photosynthetic rate of sugarcane grown under well-watered conditions and fertilized drought stress conditions. This finding is in line with other previous studies in sugarcane (Du et al., Citation1996; Sage, Citation2014), sorghum (Zhao et al., Citation2005) or another C4 plant, Cenchrus ciliaris and Panicum coloratum (Ghannoum, Citation2009), Tidestromia oblongifolia (Berry & Downton, Citation1982).
Figure 3. The correlation between leaf nitrogen content and initial slope of A/Ci curve (a); and between initial slope of A/Ci curve and potential photosynthetic rate (b) at 60 DAT; and at 120 DAT (c, d, respectively) under well-watered (closed shape) and drought stress (opened shape) conditions.
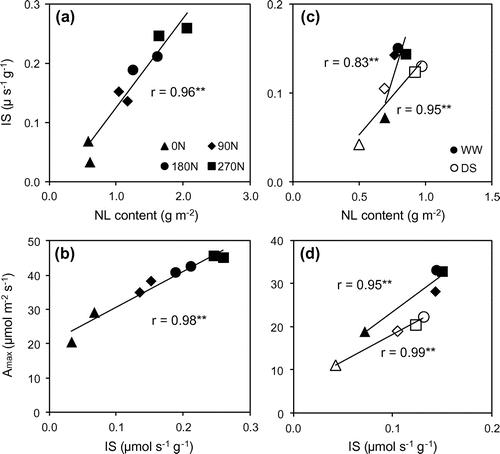
In 0 N treatment, the photosynthetic response was a little different with fertilized treatments. Under normal condition, A increased slowly then became stable from 1000 μmol m−2 s−1 of PFD. This photosynthetic response was also shown by Sage and Pearcy (Citation1987) with Amaranthus retroflexus at lower levels of nitrogen, and Zhao et al. (Citation2013) with sugarcane under severe drought stress. Under drought condition, A even decreased after reaching a peak at around 600 μmol m−2 s−1 of PFD, whereas it did not cease after Ci reached to 200 μmol mol−1. It seems to be that photosynthetic activity of sugarcane, a C4 plant to be lessening with a response as close as a C3 plant or a C3–C4 intermediate species (as shown by Monson, Citation1999) when plants adverse to severe stress from nitrogen and/or moisture deficient. It may lead to lower PNUE of 0 N treatment compared to fertilized treatment because of PNUE of C4 plant is generally higher than C3 plant (Sage & Pearcy, Citation1987).
Effect of drought stress and nitrogen application on photosynthesis of sugarcane
A reduced from 60 DAT to 120 DAT at all nitrogen applied treatments (Tables and ). Consuming from earlier research studies, McCormick et al. (Citation2006) reported that A of sugarcane has been related to plant age which young plants assimilated higher rates than older ones. Inman-Bamber et al. (Citation2011) also found that A of whole plant and of single leaves decreased up to 60% with crop development in some cases. Lower A at later period might occur because of lower NL in comparison with earlier period (Tables and ). Linking to specific leaf nitrogen content, Allison et al. (Citation1997) also agreed A increased linearly to specific leaf nitrogen content from less than 1.0 to 1.7 g m−2.
Table 1a. Initial slope of A/Ci curve (IS), potential photosynthetic rate (Amax), stomatal conductance (gs), photosynthetic nitrogen use efficiency (PNUE), photosynthetic water use efficiency (PWUE), SPAD and leaf nitrogen content (NL) across water regimes with different nitrogen applied levels at 60 DAT.
Table 1b. Initial slope of A/Ci curve (IS), potential photosynthetic rate (Amax), stomatal conductance (gs), photosynthetic nitrogen use efficiency (PNUE), photosynthetic water use efficiency (PWUE), SPAD and leaf nitrogen content (NL) under well-watered and drought stress conditions with different nitrogen applied levels at 120DAT.
Table 2. The correlations between photosynthetic traits and biomass production under well-watered and drought stress conditions.
Photosynthetic traits including IS, A, gs, SPAD and NL increased with increase of nitrogen application (Tables and ). In fact, A of 180 and 270 N treatments, in normal, were significantly higher than that of 90 N, but not significant when plant subjected to drought conditions. In contrast, for gs, SPAD and NL, the significant differences were just found at 60 DAT and in NL under drought conditions at 120 DAT. Meanwhile, 0 N had the lower values for all traits comparing to fertilized treatments under both conditions. Higher NL was a result of higher nitrate reductase activity by increasing nitrogen supply (Abayomi, Citation2001; Abayomi, Etejere, & Fadayomi, Citation1988; Wang et al., Citation2014). Due to a positive correlation between NL with both Rubisco and PEPCase activity (Meinzer & Zhu, Citation1998), higher IS were found in higher nitrogen application treatments which enhanced a higher A than lower applied treatments. This was demonstrated by the positive correlation between NL and IS, between IS and Amax (Figure ). Furthermore, the correlation of NL and Amax, gs and SPAD were positive significant (Figure ). These results suggest that higher nitrogen application supported higher A, gs and chlorophyll content (or SPAD) because of higher nitrogen concentration accumulated into the leaf.
Figure 4. The correlation between SPAD, stomatal conductance (gs), potential photosynthetic rate and leaf nitrogen (NL) content at 60 DAT (a, b, c) and at 120 DAT (d, e, f) under well-watered (closed shape) and drought stress conditions (opened shape), respectively. RP2 and RL2 are R square value on chart of linear and polynomial regression types, respectively.
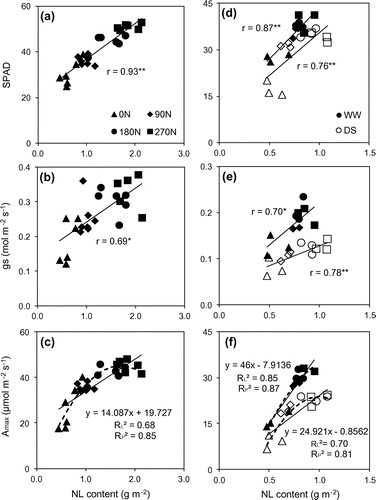
In earlier period (at 60 DAT), PNUE reduced with increasing dose of nitrogen application (Table ). Nevertheless, at 120 DAT, PNUE increased significantly from 0 to 90 N, then slightly climb under well-water condition but decreased under drought stress condition (Table ). At both periods, PWUE of fertilized treatments were not different and higher than that of 0 N treatment. Under field condition at 300 days after planting, Oliveira et al. (Citation2016) also found that sugarcane added nitrogen in irrigation system induced an increase of 14.75% of PWUE in comparison with those did not receive nitrogen. A increased with a polynomial regression rather than linear type with increase of NL (Figure ; Meinzer & Zhu, Citation1998). As can be seen from Figure , A reduced when NL reached approximately 1.71 g m−2 at 60 DAT and 1.0 g m−2 at 120 DAT, respectively. This is along with Allison et al. (Citation1997) that A and NL had linear regression just in case of NL less than 1.7 g m−2. Furthermore, very high NL in high nitrogen fertilized treatment as high as five times as 0 N treatment were found at 60 DAT, whereas these were just two times at 120 DAT might be the reason for the decrease of PNUE when nitrogen was applied at earlier stage (Figure ). Wang et al. (Citation2014) found the same point with a significant reduction of PNUE in a nonlinear relationship with NL of sweet sorghum seedlings.
Figure 5. The correlation between leaf nitrogen content and photosynthetic nitrogen use efficiency at 60 DAT (a); and at 120 DAT (b) under well-watered (closed shape) and drought stress (opened shape) conditions.
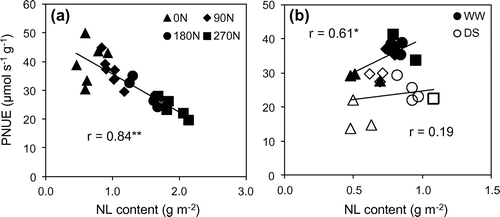
Excepting for PWUE and NL, drought reduced IS, A, gs, PNUE and SPAD (Table ). Jangpromma et al. (Citation2012) showed an insignificant effect of water stress on WUE of 10 sugarcane cultivar in Thailand. At 300 day after planting, different water replacements did not significantly alter PWUE (Oliveira et al., Citation2016). Reduction of nitrate reductase activity under water stress conditions (Abayomi et al., Citation1988) might be responsible for the decline of NL (Wang et al., Citation2014). However, the depressive effect of moisture stress was found in three of six cultivars, whereas another cultivar NL did not differ or even higher at rain-fed treatment compared to irrigated treatment (Ludlow et al., Citation1991). In fact, Abayomi et al. (Citation1988) also found the reduction in nitrate reductase activity by the effect of drought when increasing nitrogen application in Co 957 cultivar, whilst nitrate reductase activity still increased with nitrogen supply in Co997. In our study, the redundancy of nitrogen from former in company with smaller leaf area (Table ), might be the reason for later higher NL in 180 and 270 N treatment. In opposite way, the deficiency in lower nitrogen application treatment might cause a decrease of NL. Many previous studies discovered the same effects of drought stress on A, gs, and chlorophyll content or SPAD value of sugarcane (Barbosa et al., Citation2015; Du et al., Citation1996; Graça et al., Citation2010; Jangpromma et al., Citation2010; Koonjah et al., Citation2006; Silva et al., Citation2007, 2011). In the C4 plant, the initial slope of photosynthetic response shows the activity of PEPCase and effect of chlorophyll content in leaves, whereas at higher levels of light and A/Ci curve, A maybe affected by Rubisco activity. Rubisco and PEPCase activity, main limiting factors for photosynthesis, decreased when sugarcane is subjected to drought stress (Du et al., Citation1996), as a result it caused reduction in IS and A. Previous studies used the slope value of line regression in the relationship between Amax and NL as PNUE (Sage, Citation2014; Sage & Pearcy, Citation1987). By this way, drought stress also caused reductions in PNUE of sugarcane because of the slopes values were 46.0 and 24.9 (Figure ) under well-watered and stress conditions, respectively. This result was consistent with lower PNUE values of drought stress treatment in Table .
Effects of drought and nitrogen application on agronomic traits of sugarcane
As can be seen from Figure , there was an obvious difference in plant height and total leaves number of fertilized treatments with 0 N treatment starting from 14DAT. Under full irrigating condition, among fertilized treatments, the difference in plant height and total leaves number became clearly from 63DAT with higher values belonging to higher nitrogen supplied treatments. However, there were not significant differences in these values between 180 and 270 N treatments. From 63DAT under effect of drought stress, increasing rates of plant height and total leaves number were decreased, especially from 77DAT they did not virtually increase when water stress became more severe.
Figure 6. Plant height and total leaves number under well-watered (closed shapes) and drought stress (opened shapes) conditions with different nitrogen application levels.
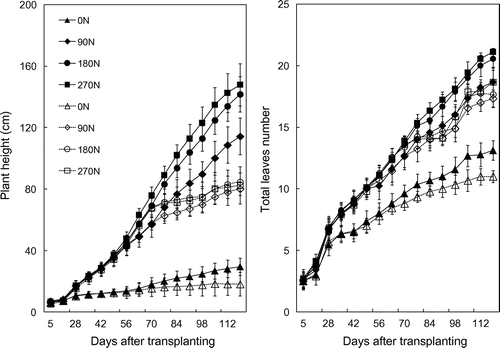
Leaf area was significantly different between nitrogen application treatments (Figure ). Actually, it augmented significantly when nitrogen level was changed from 0 to 180 N, but not as up to 270 N. Increasing nitrogen application was just significant for biomass traits under sufficient water supply. Under stress conditions, the increase was just in case of increasing nitrogen from 0 to 90 N, after that biomass became similar, especially at higher nitrogen supplied treatments. Drought stress reduced noticeably leaf area, shoot, root, and total biomass of sugarcane (Figure ). Earlier studies demonstrated positive effects of nitrogen application as well as negative effects of drought stress (Cha-um & Kirdmanee, Citation2008; Jangpromma et al., Citation2012; Kumara & Bandara, Citation2001; Oliveira et al., Citation2016; Ramesh, Citation2000; Robertson et al., Citation1999; Saleem et al., Citation2012) on leaf area, and shoot, root and total biomass of sugarcane. The positive significant correlations between photosynthetic traits (Amax, NL and SPAD) with biomass traits under both normal and drought stress conditions (Table 2) suggest that higher nitrogen applications could support higher photosynthesis activities to accumulate more dry mass. On the other hand, according to Bell (Citation2014) early shoot and root vigor, and higher root length density could support the greater uptake of nitrate and yield in soils with high nitrogen leaching potential. In our study, at higher doses of nitrogen application, the plants had faster growth rate (higher plant height and the number of leaves) than lower ones (Figure ). Furthermore, larger root with higher dry mass and number of roots (data not shown) also found in higher applied nitrogen treatments. It seems to be that adding nitrogen was advance to create earlier a larger root system for absorbing more nutrient (rather than nitrogen). Root dry weight had significant correlations with shoot dry weight and total biomass under both conditions (Table 2). The positive correlation between root dry weight with leaf, shoot dry weight and total biomass was also presented by Jangpromma et al. (Citation2012) and Jackson et al. (Citation2016). These findings raise a point of view that larger root system by adding nitrogen at early stage helps plant uptake more nutrient to create more shoot dry weight, as a result increasing total biomass under full irrigation as well as drought stress conditions.
Figure 7. Shoot dry weight (a), root dry weight (b), total biomass (c) and leaf area (d) at 120 DAT under well-watered (closed shape) and drought stress (opened shape) conditions with different nitrogen applied levels.
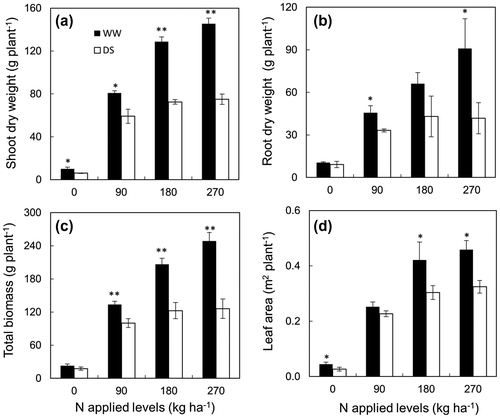
There are several investigations in the relationship between NUE and drought tolerance ability of cereal crops. In winter wheat, NUE in a line with high drought tolerance was higher than that of the line with low drought stress tolerance (Fan & Li, Citation2001). In maize, down to having either high nitrogen uptake and nitrogen utilization efficiency, drought tolerant cultivars produced consistently higher yields (Kamara et al., Citation2014). In sweet sorghum, improved water and nitrogen use efficiencies under water stress may both contribute to the high degree of physiological acclimation to drought (Wang et al., Citation2014). In this study, the relationship between PNUE, NUE and DTI were shown in Figure with strong positive coefficients (r = .90** and r = .99**, respectively). This finding highlights that higher PNUE or NUE could help plant have higher ability to tolerate drought stress. However, further studies with more diversity of sugarcane varieties should be conducted to more strongly support these findings. Furthermore, although higher nitrogen application levels had better performance (not significant especially under drought conditions), the lower levels with higher PNUE or NUE could diminish the negative effects of drought stress. In the context of this study, a range of nitrogen application from 90 to 180 kg N ha−1 seems to be the appropriate amount to maintain acceptable growth under water deficit condition. However, to suggest an optimum amount for nitrogen application, a larger scale experiment under field conditions should be investigated in later studies.
Disclosure statement
No potential conflict of interest was reported by the authors.
References
- Abayomi, Y. A. (2001). Nitrogen use efficiency and drought tolerant capacity of two commercial sugarcane cultivars. Journal of Agricultural Science and Technology, 9–11, 9–15.
- Abayomi, Y. A., Etejere, E. O., & Fadayomi, O. (1988). Effect of nitrogen level and water deficit on nitrate reductase activity (NRA) from leaves of two sugarcane cultivars- Co 957 1001. Turrialba, 38, 352–358.
- Acreche, M. M. (2017). Nitrogen-, water- and radiation-use efficiencies affected by sugarcane breeding in Argentina. Plant Breeding, 136, 174–181. doi:10.1111/pbr.12440
- Allison, J. C. S., Williams, H. T., & Pammenter, N. W. (1997). Effect of specific leaf nitrogen content on photosynthesis of sugarcane. Annals of Applied Biology, 131, 339–350.10.1111/aab.1997.131.issue-2
- Barbosa, A. M., Guidorizi, K. A., Catuchi, T. A., Marques, T. A., Ribeiro, R. V., & Souza, G. M. (2015). Biomass and bioenergy partitioning of sugarcane plants under water deficit. Acta Physiologiae Plantarum, 37, 142. doi:10.1007/s11738-015-1887-7
- Bell, M. J., Moody, P., Salter, B., Connellan, J., & Garside, A. L. (2014). Agronomy and physiology of nitrogen use in Australian sugarcane crops. In M. J. Bell (Ed.), A review of nitrogen use efficiency in sugarcane: Sugar Research Australia . eLibary. Completed projects final reports. Retrieved from https://elibrary.sugarresearch.com.au
- Berry, J. A., & Downton, W. J. S. (1982). Environmental regulation of photosynthesis. In U. Govindjee (Ed.), Photosynthesis volume II. Development, carbon metabolism, and plant productivity (pp. 263–344). New York, NY: Academic Press. A subsidiary of Harcourt Brace Jovanovich,.
- Calif, D., & Edgecombe, M. (2015). Study shows nitrogen use efficiency trait increase biomass of sugarcane. Retrieved from https://finance.yahoo.com/news/study-shows-nitrogen-efficiency-trait-110000424.html
- Cha-um, S., & Kirdmanee, C. (2008). Effect of osmotic stress on proline accumulation, photosynthetic abilities and growth of sugarcane plantlets. Pakistan Journal of Botany, 40, 2541–2552.
- Dinh, T. H., Kaewpradit, W., Jogloy, S., Vorasoot, N., & Patanothai, A. (2014). Nutrient uptake of peanut genotypes with different levels of drought tolerance under midseason drought. Turkish Journal of Agriculture and Forestry, 38, 495–505.10.3906/tar-1309-45
- Du, Y. C., Kawamitsu, Y., Nose, A., Hiyane, S., Murayama, S., Wasano, K., & Uchida, Y. (1996). Effect of water stress on carbon exchange rate and activities of photosynthetic enzymes in leaves of sugarcane (Saccharum sp.). Australian Journal of Plant Physiology, 23, 719–726.10.1071/PP9960719
- Ethan, S., Olagoke, O., & Yunusa, A. (2016). Effect of deficit irrigation on growth and yield of sugarcane. Direct Research Journal of Agriculture and Food Science, 4, 122–126.
- Fan, X. L., & Li, Y. K. (2001). Effect of drought stress and drought tolerance heredity on nitrogen efficiency of winter wheat (abstract). In W. J. Horst, M. K. Schenk, A. Bürkert, N. Claassen, H. Flessa, W. B. Frommer, … L. Wittenmayer (Eds.), Plant nutrition. Developments in plant and soil sciences (Vol. 92, pp. 62–63). Dordrecht: Springer.
- Ghannoum, O. (2009). C4 photosynthesis and water stress. Annals of Botany, 103, 635–644. doi:10.1093/aob/mcn093
- Graça, J. P., Rodrigues, F. A., Farias, J. R. B., Oliveira, M. C. N., Hoffmann-Campo, C. B., & Zingaretti, S. M. (2010). Physiological parameter in sugarcane cultivars submitted to water deficit. Brazilian Journal of Plant Physiology, 22, 189–197.
- Inman-Bamber, N. G., Jackson, P. A., & Hewitt, M. (2011). Sucrose accumulation in sugarcane stalks does not limit photosynthesis and biomass production. Crop and Pasture Science, 62, 848–858.10.1071/CP11128
- Jackson, P., Basnayake, J., Inman-Bamber, N. G., Lakshmanan, P., Natarajan, S., & Stokes, C. (2016). Genetic variation in transpiration efficiency and relationships between whole plant and leaf gas exchange measurements in Saccharum spp. and related germplasm. Journal of Experimental Botany, 67, 861–871.10.1093/jxb/erv505
- Jangpromma, N., Songrsi, P., Thammasiririak, S., & Jaisil, P. (2010). Rapid assessment of chlorophyll content in sugarcane using a SPAD chlorophyll meter across different water stress conditions. Asian Journal of Plant Science, 9, 368–374.
- Jangpromma, N., Thammasirirak, S., Jaisil, P., & Songsri, P. (2012). Effects of drought and recovery from drought stress on above ground and root growth, and water use efficiency in sugarcane (Saccharum officinarum L.). Australian Journal of Crop Science, 6, 1298–1304.
- Kamara, A. Y., Ewanshia, S. U., & Menkir, A. (2014). Assessment of nitrogen uptake and utilization in drought tolerant and Striga resistant tropical maize varieties (abstract). Archives of Agronomy and Soil Science, 60, 195–207. doi: 10.1080/03650340.2013.783204
- Kawamitsu, Y., Hiyane, S., Tamashiro, Y., & Hakoyama, S. (2002). Regulation of photosynthesis and water use efficiency in relation to stomatal frequency and interveinal distance in C3- and C4- grass species. Environmental Control in Biology, 40, 365–374.10.2525/ecb1963.40.365
- Koonjah, S. S., Walker, S., Singels, A., Antwerpen, R. V., & Nayamuth, A. R. (2006). A quantitative study of water stress effect on sugarcane photosynthesis. Proceeding of South African Sugar Technologists’ Association, 80, 140–158.
- Kumara, A. D. S., & Bandara, D. C. (2001). Influence of nitrogen application and varietal differences on selected physiological parameters of sugarcane. Tropical Agricultural Research, 13, 220–230.
- Ludlow, M. M., Ferraris, R., & Chapman, L. S. (1991). The interaction between nitrogen and water supply on the photosynthetic rate of sugar cane leaves. Proceedings of Australian Society of Sugar Cane Technologists, 13, 66–72.
- McCormick, A. J., Cramer, M. D., & Watt, D. A. (2006). Sink strength regulates photosynthesis in sugarcane. New Phytologist, 171, 759–770. doi:10.1111/j.1469-8137.200601785
- Meinzer, F. C., & Zhu, J. (1998). Nitrogen stress reduces the efficiency of the C4 the CO2 concentrating system, and therefore quantum yield, in Saccharum (sugarcane) species. Journal of Experimental Botany, 49, 1227–1234.
- Monson, R. K. (1999). The origins of C4 genes and evolutionary pattern in C4 metabolic phenotype. In R. F. Sage & R. K. Monson (Eds.), C4 plant biology (pp. 377–410). Cambridge, MA: Academic Press.
- Oliveira, R. C., Silva, F. B., Teixeira, M. B., Costa, A. C., Soares, F. A. L., & Megguer, C. A. (2016). Response of sugarcane to limitation hydric and nitrogen dose. African Journal of Agricultural Research, 11, 1475–1485.
- Ramesh, P. (2000). Sugarcane breeding institute, Coimbatore, India effect of different levels of drought during the formative phase on growth parameters and its relationship with dry matter accumulation in sugarcane. Journal of Agronomy Crop Science, 185, 83–89.10.1046/j.1439-037x.2000.00404.x
- Robertson, M. J., Inman-Bamber, N. G., Muchow, R. C., & Wood, A. W. (1999). Physiology and productivity of sugarcane with early and mid-season water deficit. Field Crop Research, 64, 211–227.10.1016/S0378-4290(99)00042-8
- Sage, R. F., & Pearcy, R. W. (1987). The nitrogen use efficiency of C3 and C4 plants. II. Leaf nitrogen effects on the gas exchange characteristics of Chenopodium album (L.) and Amaranthus retroroflexus (L.). Plant Physiology, 84, 959–963.10.1104/pp.84.3.959
- Sage, R. F., Peixoto, M. M., & Sage, T. L. (2014). Photosynthesis in sugarcane. In P. H. Moore & F. C. Botha (Eds.), Physiology, biochemistry, and functional biology (1st ed.), pp. 377–410. Hoboken, NJ: Wiley.
- Saleem, M. F., Ghaffar, A., Anjum, S. A., Cheema, M. A., & Bilal, M. F. (2012). Effect of nitrogen on growth and yield of sugarcane. Journal of American Society of Sugar Cane Technologists, 32, 75–93.
- Silva, M. A., Jifon, J. L., Sharma, V., Silva, J. A. G., Caputo, M. M., Damaj, M. B., … Ferro, M. I. T. (2011). Use of physiological parameters in screening drought tolerance in sugarcane genotypes. Sugar Technology, 13, 191–197.10.1007/s12355-011-0087-z
- Silva, M. A., Jifon, J. L., Silva, J. A. G., & Sharma, V. (2007). Use of physiological parameters as fast tools to screen for drought tolerance in sugarcane. Brazilian Journal of Plant Physiology, 19, 193–201.10.1590/S1677-04202007000300003
- Wang, W. F., Zong, Y. Z., & Zhang, S. Q. (2014). Water- and nitrogen-use efficiencies of sweet sorghum seedlings are improved under water stress. International Journal of Agriculture & Biology, 16, 285–292.
- Zhao, D., Barry, G., & Comstock, J. C. (2013). Sugarcane leaf photosynthesis and growth characters during development of water-deficit stress. Crop Science, 53, 1066–1075. doi:10.2135/cropsci2012.09.0554
- Zhao, D., & Li, Y. (2015). Climate change and sugarcane production: Potential impact and mitigation strategies. International Journal of Agronomy, 2015, 10. doi:10.1155/2015/547386
- Zhao, D., Reddy, K. R., Kakani, V. G., & Reddy, V. R. (2005). Nitrogen deficiency effects on plant growth, leaf photosynthesis, and hyperspectral reflectance properties of sorghum. European Journal of Agronomy, 22, 391–403. doi:10.1016/j.eja.2004.06.005
- Zhu, G., Peng, S., Huang, J., Cui, K., Nie, L., & Wang, F. (2016). Genetic improvements in rice yield and concomitant increases in radiation- and nitrogen-use efficiency in middle reaches of Yangtze river. Science Reports, 6, 21049. doi:10.1038/srep21049
- Zingaretti, S. M., Rodrigues, F. A., Graça, J. P., Pereira, L. M., & Lourenço, M. V. (2012). Sugarcane responses at water deficit conditions. In P. M. M. Rahman (Ed.), Water Stress. InTech. doi:10.5772/30986