Abstract
γ-Oryzanol is a main oleophilic component in rice bran oil and has been well recognized as a good dietary supplement for human health, as well as having uses in industrial materials. japonica-type rice cultivars generally showed significantly higher contents of total γ-oryzanol in brown rice compared with indica-type cultivars, although within-group variation was significant. The objective of this study was to explore quantitative trait loci (QTLs) responsible for the difference in the γ-oryzanol content between japonica-type and indica-type rice cultivars, using recombinant inbred lines (RILs), backcross inbred lines (BILs), and corresponding chromosome segment substitution lines (CSSLs) derived from crosses between japonica-type and indica-type. Results from RILs and BILs showed that eight QTLs were detected with R2 from .09 to .16. Nine candidate regions for QTL were also suggested from corresponding CSSLs. These QTLs from RILs and BILs and the candidate regions from CSSLs were not overlapped, although one QTLs was mapped near the boundaries of the respective candidate region. At four QTLs and three candidate regions, alleles or segments from japonica-type caused higher contents than those from indica-type. On the other hand, at the other four QTLs and six candidate regions, alleles or segments from indica-type caused higher contents than those from japonica-type, which is a reverse result to the parental differences. This result strongly suggested that alleles with increasing effects on γ-oryzanol content could be accumulated not only from japonica-type but also from indica-type, leading to a potential for increase in γ-oryzanol content in future breeding programs.
Classification:
Introduction
γ-Oryzanol is a mixture of several organic compounds in rice (Oryza sativa L.) bran, consisting mainly of cycloartenyl ferulate, 24-methylenecycloartanyl ferulate, campesteryl ferulate, and β-sitosteryl ferulate, all of which are ferulate esters of phytosterols (Fang et al., Citation2003; Piironen et al., Citation2000). γ-Oryzanol is well known as an important and relatively stable oleophilic component of rice bran oil, and is recognized as a good dietary supplement and a pharmaceutical material for the maintenance and enhancement of human health (Heinemann et al., Citation2008; Shin et al., Citation1997). In addition, γ-oryzanol can be utilized industrially as a heat-resistant antioxidant against auto-oxidation of edible oil (Kanno et al., Citation1985).
Several studies have shown that the content of total γ-oryzanol in rice grains including bran exhibited a wide genetic variation among cultivars from the southern USA (Chen & Bergman, Citation2005), European countries (Miller & Engel, Citation2006), Brazil (Heinemann et al., Citation2008), Taiwan (Huang & Ng, Citation2011), and Korea (Kim et al., Citation2015), as well as non-genetic fluctuation due to environmental factors (Britz et al., Citation2007) and temporal changes in the course of the grain filling process after fertilization and germination (Kimura et al., Citation2008). Kato (Citation2010) also examined the variation in total γ-oryzanol content in brown rice among 27 rice cultivars including japonica-type and indica-type. In these cultivars, japonica-type cultivars as a whole showed significantly higher total γ-oryzanol content compared with indica-type cultivars, while within-group variation was also significant. The same trends for the higher contents in japonica-type cultivars compared with indica-type cultivars were also reported in several other cultivar populations, though the significant tests between the two types were not performed (Heinemann et al., Citation2008; Huang & Ng, Citation2011). Such genetic variation should be essential for breeding programs to increase γ-oryzanol content. However, no information is available on the genetic factors underlying the variation in γ-oryzanol content in rice.
This study was conducted to explore the quantitative trait loci (QTLs) responsible for the difference in γ-oryzanol content between japonica-type and indica-type cultivars. For this purpose, we used several populations of recombinant inbred lines (RILs), backcross inbred lines (BILs), and chromosome segment substitution lines (CSSLs) with different genetic backgrounds. The results from this study will provide novel information for further fine investigations of QTLs for this character and facilitate related breeding programs.
Materials and methods
Plant materials and cultivation
Five kinds of segregating populations with different genetic backgrounds were used: 66 RILs derived from Asominori/IR24 (AIRIL), 41 CSSLs of IR24 segments in Asominori background (AIS) and their reciprocal 30 CSSLs (IAS), 87 BILs from Habataki/Sasanishiki//Sasanishiki (SHBIL), and 38 CSSLs of Habataki segments in Sasanishiki background (SL). Of these cultivars, the parents of Asominori and Sasanishiki are japonica-types, and those of IR24 and Habataki are indica-types. AIRIL, AIS, IAS, and their parents, Asominori and IR24, were provided from Prof. A. Yoshimura, Graduate School of Kyushu University, Japan, and SHBIL, SL, and their parents, Sasanishiki and Habataki, were provided by the NIAS GeneBank, Japan.
AIRIL, AIS, and IAS were grown in 2012, and SHBIL and SL were in 2013, together with the respective parents. They were sown in nursery boxes on 15 May 2012 and 2013, grown in a greenhouse, and transplanted into experimental paddy fields of the Faculty of Biology-Oriented Science and Technology, Kindai University, Kinokawa, Wakayama, Japan, on 13 June 2012 or 11 June 2013. Each line consisted of six plants, and planted as a single row at the density of 30 cm inter-row and 15 cm inter-hill, with a single plant per hill. Fertilizer was applied as basal dressing at a rate of 6:6:6 g m−2 for N:P2O5:K2O. Standard cultivation practices, including irrigation control, pest management, etc., were applied.
Extraction and quantification of total γ-oryzanol from brown rice
At maturing stage (approximately 45–50 days after heading), several panicles on a single plant in each line and cultivar were harvested and air-dried. Grains on these panicles were threshed and de-husked to obtain brown rice. The brown rice was immediately ground into fine powder using a mixer mill (MM 301, RETSCH GmbH, Haan, Germany). The present methods of extraction and quantification of γ-oryzanol essentially followed the method of Chen and Bergman (Citation2005). Immediately after grinding, precisely weighed powder of around 250 mg was immersed with 1 mL of methanol (Nacalai Tesque, Inc., Kyoto, Japan), homogenized well using a vortex, and stored at approximately 4 °C for 24 h. After centrifuged at 850 × g for 10 min at room temperature, supernatant was filtered using a disposable filter of .45 μm pore size (Whatman, GE Healthcare UK Ltd, Buckinghamshire, UK). The resulting samples were applied to high-performance liquid chromatography (HPLC). This extraction was performed three times for each line and cultivar as three replicates.
The HPLC system used in the present experiment consisted of an auto-injector (AS-8010, Tosoh Corp., Tokyo, Japan), a single pump (LC-10AS, SHIMADZU Corp., Kyoto, Japan), a reverse-phase column (Inertsil ODS-3, 5 μm 4.6 × 150 mm, GL Sciences Inc., Tokyo, Japan) in a column oven (CTO-10A, SHIMADZU Corp., Kyoto, Japan) set at 40 °C, and UV-detector (SPD-10A, SHIMADZU Corp., Kyoto, Japan). The mobile phase in the HPLC consisted of .70 L L−1 methanol, .25 L L−1 acetonitrile and .05 L L−1 2-propanol (all were from Nacalai Tesque, Inc.). A 10 μL volume of each sample was injected, run at a flow rate of .8 mL min−1, and absorbance was monitored at 325 nm. As described later, there were four major peaks from retention times of approximately 15–25 min (Figure ). The concentration of total γ-oryzanol in a sample was estimated from the integrated area of the four peaks (using a SmartChrom software, KYA TECH Corp., Tokyo, Japan). Cycloartenyl ferulate (CA, Wako Pure Chemical Industries, Ltd, Osaka, Japan) 2.5 and 5.0 μg mL−1 in methanol were used as an authentic standard, because CA is the only component commercially available. The content of total γ-oryzanol in a sample was recorded as ng CA equivalent mg dry weight−1.
QTL and statistical analyses
For AIRIL and SHBIL, the information of 375 and 236 DNA markers, respectively, were already available (Nagata et al., Citation2002; Tsunematsu et al., Citation1996). Each linkage map was constructed for AIRIL and SHBIL using MAPMAKER/EXP 3.0 (Lander et al., Citation1987), based on the above markers. QTL analyses were conducted for total γ-oryzanol content of AIRIL and SHBIL using Windows QTL Cartographer ver. 2.5 (Wang et al., Citation2007), based on the respective linkage maps. LOD scores were set at 3.1 and 2.6 for AIRIL and SHBIL, respectively, corresponding to the significance of .01 probability level estimated from permutation test of 1000 times. Positions (cM) of detected QTLs were converted from the positions on the original maps of AIRIL and SHBIL to those on the High-Density Rice Genetic Map (Harushima et al., Citation1998) by intrapolation between the nearest flanking markers.
For the three populations of CSSLs, the means of total γ-oryzanol contents for CSSLs were compared with those of their respective parents based on the least significant differences at the .001 probability level. The distributions of chromosome segments in each CSSLs were estimated from published marker genotypes (https://www.shigen.nig.ac.jp/rice/oryzabase/strain/geneticMarker/about#AIS for AIS, https://www.shigen.nig.ac.jp/rice/oryzabase/strain/geneticMarker/about#IAS for IAS and https://www.rgrc.dna.affrc.go.jp/jp/stock.html for SL).
Results
Figure shows frequency distributions for the total γ-oryzanol content in brown rice in AIRIL and SHBIL populations, together with their parents. Wide segregations in this trait were found in both populations. In SHBIL, many lines showed a transgressive segregation in both directions beyond the range of parents, while in AIRIL a number of lines deviated only to lower direction than a lower parent, IR24.
Figure 2. Frequency distributions of total γ-oryzanol content of brown rice among (A) recombinant inbred lines from Asominori/IR24 and (B) backcross inbred lines from Sasanishiki/Habataki//Sasanishiki. Horizontal bar indicates SD.
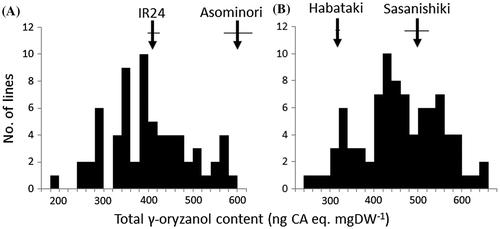
Table shows the results of QTL analyses in AIRIL and SHBIL for the total γ-oryzanol content in brown rice. Total of three and five QTLs in AIRIL (designated as qOZ1c, qOZ5a and qOZ9) and SHBIL (qOZ1a, qOZ1b, qOZ5b, qOZ7 and qOZ12), respectively, were detected. The three QTLs in chromosome 1 and two QTLs in chromosome 5 might be different ones to each other in the respective chromosomes, because of the difference in their estimated map positions. Ratios of variation explained by the respective QTLs to the total variation (R2) were similar among QTLs ranged from .09 to .16. Interestingly, several alleles derived from japonica-type parents at the all QTLs on chromosomes 1 and 9 showed negative values of additive effects, indicating that these alleles from japonica-type parents decreased the total γ-oryzanol content, while the alleles from indica-type parents increased at these QTLs.
Table 1. QTLs for total γ-oryzanol content in brown rice detected from a set of recombinant inbred lines from Asominori/IR24 and that of backcross inbred lines from Sasanishiki/Habataki//Sasanishiki.
Figures and show the results from AIS with IAS and SL, respectively, as well as their parents, for the same trait. The japonica-type parents, Asominori and Sasanishiki, showed significantly higher total γ-oryzanol content than the respective indica-type parents, IR24 and Habataki, at the .001 probability level. Many CSSLs in every population showed significant differences in this trait from the respective background parents.
Figure 3. Total γ-oryzanol content of brown rice in chromosome segment substitution lines (CSSLs) with IR24 segments in Asominori background (AIS), and those with Asominori segments in IR24 background (IAS), together with their parents. White, black and hatched bars indicate CSSLs with no significant differences from Asominori, from IR24 and with significant difference from the respective background parents (p < .001), respectively.
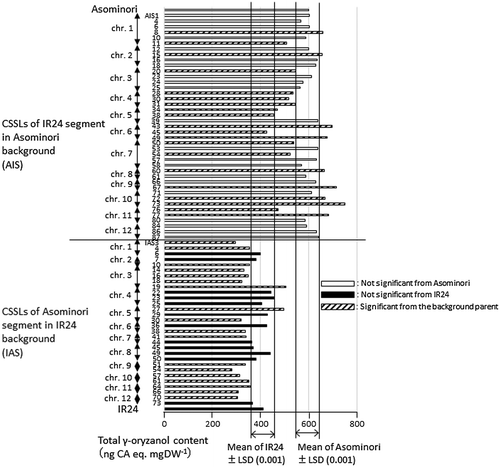
Figure 4. Total γ-oryzanol contents of brown rice in chromosome segment substitution lines (CSSLs) with Habataki segments in Sasanishiki background (SL), together with their parents. White, black and hatched bars indicate CSSLs with no significant differences from Sasanishiki, from Habataki and with significant difference from background parent, Sasanishiki (p < .001), respectively.
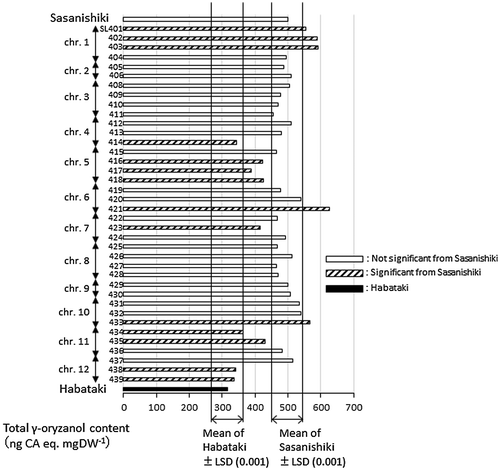
Thus for individual chromosomes, the distributions of chromosome segments from different parents and the performances of CSSLs in this trait were examined in Supplementary Figures S1 and S2 to explore candidate regions of QTLs for total γ-oryzanol content in brown rice. Table represents expected performance of a CSSL involving a QTL for this trait. When a CSSL has a chromosome segment which really had an increasing allele from parent A at a QTL, this CSSL is expected to show similar content to parent A in the background of this parent A, while under the background of another parent B, the CSSL having the same allele from parent A is expected to show higher content than parent B, etc. Moreover, when a CSSL involves no QTLs for this trait, this CSSL is expected to show similar content to that of the respective background parent. For this reason, CSSLs in SL for chromosomes 2, 3, 8 and 9 in SL were not examined.
Table 2. Expected performance of a chromosome segment substitution line (CSSL) involving a QTL for total γ-oryzanol content in brown rice.
Candidate regions involving QTLs for this trait were deduced as regions where the existence of the QTLs could not completely be rejected from the results of CSSLs. Consequently, total of three candidate regions (in chromosomes 9, 10, and 12) in AIS and IAS, and six regions (in chromosomes 1, 4, 6, 10, 11, and 12) in SL were suggested (Table ). Thus, there might be putative QTLs in these candidate regions for this trait, in addition to those detected from QTL analysis in Table .
Table 3. Candidate regions of QTL for total γ-oryzanol content in brown rice suggested from three sets of chromosome segment substitution lines.
These nine candidate regions did not overlap apparently in their positions to the QTLs detected in Table . However, qOZ9 was mapped between one of the boundaries of the candidate region in chromosomes 9 in AIS and IAS, R2638, and its nearest flanking marker C609 (Figure S1-(I)). Therefore, it could not be concluded completely that qOZ9 is not involved in the respective candidate region, since the boundary between R2638 and C609 cannot be determined definitely with the present map. In the case of SHBIL and SL, the estimated positions of two QTLs (qOZ1a and qOZ1b) in chromosome 1, and one QTL (qOZ12) in chromosome 12 were obviously out of the respective candidate region (Figures S2-(A) and S2-(H)).
Similar to the QTLs in Table , in six candidate regions out of nine, alleles with increasing effect on total γ-oryzanol content at the putative QTLs were derived from indica-type parents, IR24 in AIS and IAS or Habataki in SL. For the candidate regions in chromosome 10 suggested from different populations, the right boundary marker C405 in AIS and IAS (physical map position of 23,558,190 in RAP-DB Build5) is mapped at the downstream of the left boundary marker RM3773 in SL (20,463,948), although no data of physical map positions for the remaining two markers were found. Therefore, these two candidate regions are apparently overlapped. In addition, both segments might involve alleles with increasing effect on total γ-oryzanol content from indica-type parents. This implied that the putative QTLs in these chromosome segments were the same.
Discussion
From the present HPLC analysis, a common profile was obtained from all samples, as shown in Figure . Four major peaks (A–D in Figure ) appeared from approximately 15–25 min of retention time. The same four peaks have been reported in other HPLC analyses of γ-oryzanol in different rice samples from different procedures. Of these reports, Rogers et al. (Citation1993), Xu and Godber (Citation1999), Fang et al. (Citation2003), Huang and Ng (Citation2011), Miller and Engel (Citation2006), and Kim et al. (Citation2015) assigned the components of γ-oryzanol to the respective peaks. Most of these reports confirmed that the major four peaks of A, B, C, and D in Figure corresponded to cycloartenyl ferulate, 24-methylenecycloartanyl ferulate, campesteryl ferulate and β-sitosteryl ferulate, respectively, with means of mass spectrometry. This result strongly suggested that the present four peaks should be the same respective compounds, indicating that the total peak area of these four can represent the total γ-oryzanol content.
The present QTL analysis detected eight QTLs for total γ-oryzanol content in brown rice, in different segregating populations. In addition, nine candidate regions of QTLs for the same trait were also suggested from the analysis of CSSLs derived from the same cross combinations in the above QTL analysis, while two regions out of the nine from different cross combinations might involve the same QTL. These QTLs and candidate regions could explain the differences between Asominori and IR24, and Sasanishiki and Habataki, i.e. between japonica-type and indica-type parents of the present mapping populations. In each of the cross combination, Asominori and IR24, or Sasanishiki and Habataki, no QTLs detected from QTL analysis could be mapped in the candidate regions of QTLs from CSSLs, although one QTLs (qOZ9) could not be concluded completely for their exclusion from the respective candidate regions. This inconsistency might be attributed to interactions of QTLs and genetic backgrounds, environmental effects, or relatively moderate R2 of the detected QTLs, etc. Further studies will be needed to confirm the existence of the detected QTLs and additional putative QTLs in the candidate regions deduced in CSSLs, and also to make fine mapping of them.
Notable results from this study were: (1) detected QTLs, and also candidate regions, for total γ-oryzanol content were different depending on the cross combinations of segregating populations used, while two candidate regions might involve the same QTL, and (2) RILs and BILs with indica-type alleles and also CSSLs with segments from indica-type parents showed higher total γ-oryzanol content than those with japonica-type alleles or segments, whereas indica-type parents significantly showed lower contents than japonica-type parents. These results might be one of the causes for a wide within-group variation (within indica-types and within japonica-types) for this trait (Kato, Citation2010). The former result could indicate that much more loci exist along rice genome for this trait, than those detected in this study. The latter result strongly suggested that total γ-oryzanol content should be increased beyond the levels of japonica-type cultivars through the accumulation of alleles derived not only from japonica-types but also from indica-types.
The detected QTLs and the putative ones in the candidate regions for the content of γ-oryzanol in this study would be enzyme loci related to the biosynthesis pathway of γ-oryzanol components (Piironen et al., Citation2000). Hoa et al. (Citation2003) reported an increase in γ-oryzanol content by transforming several genes involved in the isoprenoid pathway from daffodil and Erwinia uredovara into indica-type cultivars. Tang et al. (Citation2011) tried to increase the stigmasterol content by addition of barley chromosome 3H, which involves the CYP710A8-encoding sterol C-22 desaturase gene, into common wheat. Table shows several loci responsible for enzymes along sterol pathway synthesizing phytosterols and phenylplopanoid pathway synthesizing ferulate (https://plantreactome/gramene.org/PathwayBrowser/), which are located relatively near the detected QTLs in the present study. No these enzyme loci were found within nor near the suggested candidate regions. These enzyme loci could be candidates for these QTLs.
Table 4. Loci for enzymes involved in sterol syntheses located to near the detected QTLs for total γ-oryzanol content in brown rice (within 5 Mbp), searched at https://plantreactome/gramene.org/PathwayBrowser/ (secondary metabolite biosynthesis, sterol biosynthesis, and phenylplopanoid biosynthesis).
Disclosure statement
No potential conflict of interest was reported by the authors.
Supplemental data
Supplemental data for this article can be accessed at https://doi.org/10.1080/1343943X.2017.1372109
TPPS_1372109_Supplementary_material.docx
Download MS Word (850.9 KB)Acknowledgements
The authors wish to thank T. Yako, T. Yamamoto, T. Fujiwara, C. Yamato, K. Yajima, S. Inagaki and Y. Takata, Fac. Biology-Oriented Science and Technology, Kindai University, for their technical assistance. The authors also very appreciate K. Nishimura, Graduate School of Agriculture, Kyoto University, for his conductance of QTL analysis.
References
- Britz, S. J., Prasad, P. V. V., Moreau, R. A., Allen, L. H., Jr., Kremer, D. F., & Boote, K. J. (2007). Influence of growth temperature on the amounts of tocopherols, tocotrienols, and γ-oryzanol in brown rice. Journal of Agricultural and Food Chemistry, 55, 7559–7565. doi:10.1021/jf0637729
- Chen, M. H., & Bergman, C. J. (2005). A rapid procedure for analysing rice bran tocopherol, tocotrienol and γ-oryzanol contents. Journal of Food Composition and Analysis, 18, 139–151. doi:10.1016/j.jfca.2003.09.004
- Fang, N., Yu, S., & Badger, T. M. (2003). Characterization of triterpene alcohol and sterol ferulates in rice bran using LC-MS/MS. Journal of Agricultural and Food Chemistry, 51, 3260–3267. doi:10.1021/jf021162c
- Harushima, Y., Yano, M., Shomura, A., Sato, M., Shimano, T., Kuboki, Y., … Sasaki, T. (1998). A high-density rice genetic linkage map with 2275 markers using a single F2 population. Genetics, 148, 479–494.
- Heinemann, R. J. B., Xu, Z., Godber, J. S., & Lanfer-Marquez, U. M. (2008). Tocopherols, tocotrienols, and γ-oryzanol contents in japonica and indica subspecies of rice (Oryza sativa L.) cultivated in Brazil. Cereal Chemistry, 85, 243–247. doi:10.1094/CCHEM-85-2-0243
- Hoa, T. T. V., Potrykus, I., & Beyer, P. (2003). Increase the level of γ-oryzanol, tocopherols and tocotrienols in rice by isoprenoid-pathway engineering. Omonrice, 11, 28–34.
- Huang, S. H., & Ng, L. T. (2011). Quantification of tocopherols, tocotrienols, and γ-oryzanol contents and their distribution in some commercial rice varieties in Taiwan. Journal of Agricultural and Food Chemistry, 59, 11150–11159. doi:10.1021/jf202884p
- Kanno, H., Usuki, R., & Kaneda, T. (1985). Antioxidative effect of oryzanol on thermal oxidation of oils. Nippon Shokuhin Kogyo Gakkaishi, 32, 170–173**.10.3136/nskkk1962.32.3_170
- Kato, T. (2010). Variation of γ-oryzanol content in brown rice among rice cultivars. Japanese Journal of Crop Science, 79, 154–155*.
- Kim, H. W., Kim, J. B., Cho, S.-M., Cho, I. K., Li, Q. X., Jang, H-.H., … Hwang, K-.A. (2015). Characterization and quantification of γ-oryzanol in grains of 16 Korean rice varieties. International Journal of Food Sciences and Nutrition, 66, 166–174. doi:10.3109/09637486.2014.971226
- Kimura, E., Yoshida, T., Koike, S., Watanabe, A., Kimura, T., & Nishio, T. (2008). Content changes of γ-oryzanol during rice grain filling and germination. Japanese Journal of Crop Science, 77, 134–135*.
- Lander, E., Green, P., Abrahamson, J., Barlow, A., Daly, M. J., Lincoln, S. E., & Newburg, L. (1987). MAPMAKER: An interactive computer package for constructing primary genetic linkage maps of experimental and natural populations. Genomics, 1, 174–181. doi:10.1016/0888-7543(87)90010-3
- Miller, A., & Engel, K. H. (2006). Content of γ-oryzanol and composition of steryl ferulates in brown rice (Oryza sativa L.) of European origin. Journal of Agricultural and Food Chemistry, 54, 8127–8133. doi:10.1021/jf061688n
- Nagata, K., Fukuta, Y., Shimizu, H., Yagi, T., & Terao, T. (2002). Quantitative trait loci for sink size and ripening traits in rice (Oryza sativa L.). Breeding Science, 52, 259–273. doi:10.1270/jsbbs.52.259
- Piironen, V., Lindsay, D. G., & Miettinen, T. A. (2000). Plant sterols: Biosynthesis, biological function and their importance to human nutrition. Journal of the Science of Food and Agriculture, 80, 939–966. doi:10.1002/(SICI)1097-0010(20000515)80:7<939::AID-JSFA644>3.0.CO;2-C
- Rogers, E. J., Rice, S. M., Nicolosi, R. J., Carpenter, D. R., McClelland, C. A., & Romanczyk, L. J., Jr (1993). Identification and quantitation of γ-oryzanol components and simultaneous assessment of tocols in rice bran oil. Journal of the American Oil Chemists’ Society, 70, 301–307. doi:10.1007/BF02545312
- Shin, T. S., Godber, J. S., Martin, D. E., & Wells, J. H. (1997). Hydrolytic stability and changes in E vitamers and oryzanol of extruded rice bran during storage. Journal of Food Science, 62, 704–728. doi:10.1111/j.1365-2621.1997.tb15440.x
- Tang, J., Ohyama, K., Kawaura, K., & Hashinokuchi, H. (2011). A new insight into application for barley chromosome addition lines of common wheat: Achievement of stigmasterol accumulation. Plant Physiology, 157, 1555–1567. doi:10.1104/pp.111.183533
- Tsunematsu, H., Yoshimura, A., Harushima, Y., Nagamura, Y., Kurata, N., Yano, M., … Iwata, N. (1996). RFLP framework map using recombinant inbred lines in rice. Breeding Science, 46, 279–284. doi:10.1270/jsbbs1951.46.279
- Wang, S., Basten, C. J., & Zeng, Z. B. (2007). Windows QTL Cartographer 2.5. Raleigh: Department of Statistics, North Carolina State University.
- Xu, Z., & Godber, J. S. (1999). Purification and identification of components of γ-oryzanol in rice bran oil. Journal of Agricultural and Food Chemistry, 47, 2724–2728. doi:10.1021/jf981175j