Abstract
Mixed cropping is a cultivation method widely practiced in tropical regions. The newly developed close mixed planting technique mitigates the flood stress of drought-adapted upland cereal species by co-growing rice (Oryza sativa) plants under field flood conditions. We tested the hypothesis that O2 was transferred from rice to upland crops using the model system of hydroponic culture. To confirm the hypothesis, the phenomena of O2 absorption and release by plants were evaluated in a water culture condition without soil. Experiments were conducted in a climate chamber to estimate the amount of O2 released from the roots of rice and pearl millet (Pennisetum glaucum) under both O2-rich (20.0 ± .0% conc. in phase I) and O2-free dark (.8 ± .0% conc. in phase II) conditions. The total O2 change (between the two phases) in a single planting of rice and pearl millet was significantly higher than that of the mixed planting of rice and pearl millet, which indicated that O2 was transferred from rice to pearl millet under a water culture condition. The result indicated that approximately 7 μM O2 g fresh root weight−1 h−1 was transferred between the two plant species. O2 transfer was confirmed between the two plant species in a mix cultured in water, implying its contribution to the phenomenon that improved the physiological status of drought-adapted upland crops under field flood conditions.
Classification:
Introduction
Mixed cropping is a major cultivation technique that is used to grow two or more plant species in the same field in the same year, and it is widely practiced in tropical regions (Ramert et al., Citation2002; Vandermeer, Citation1989). Although mixed cropping generally induces competition for light, moisture, and nutrients among the co-growing plants (Lithourgidis et al., Citation2011), it is a risk management practice against crop losses (Brooker et al., Citation2015; Wolfe, Citation2000). Moreover, it often exhibits compensatory interactions such as the supply of nitrogen nutrition from legumes to non-leguminous crops (Ehrmann & Ritz, Citation2014; Ramirez-Garcia et al., Citation2015). Close mixed planting is a newly proposed mixed cropping technique that enhances the flood stress tolerance of co-growing drought-adapted upland cereal crops (Awala et al., Citation2016; Iijima et al., Citation2016). Growing flood- and drought-adapted crops at the same time in the same field, as in close mixed planting, will be a new global challenge to overcome the effects of climate change. For example, close mixed planting with rice (Oryza sativa) improved the photosynthetic rate, transpiration rate, and biomass of co-growing upland crops grown under O2-deficient solution culture conditions. In addition, the O2 concentration in the solution culture of upland crops was significantly increased by co-growing rice (Iijima et al., Citation2016). Even in field conditions, close mixed planting with rice mitigated the flood stress of upland cereals in terms of plant survival and grain production (Awala et al., Citation2016). These results suggest that the flood stress of upland crops was mitigated by utilizing rhizosphere O2 that was released from rice roots. However, the O2 transfer between different plant species has never been analyzed before, even though mixed cropping is widely practiced.
Aerenchyma formation is poorly developed in upland crops, and plant survival over longer periods under flood conditions is difficult. On the other hand, flood-adapted cereal crops such as rice have a fully developed aerenchyma, and the O2 received from the stomata of leaves and stems is transported to the roots (Armstrong, Citation1979). Because the barrier to radial oxygen loss (ROL) at the root surface is not formed near the root apex, O2 from the shoot system leaks into the rhizosphere (Colmer, Citation2003; Yamauchi et al., Citation2013). Oxygen release from the root apex affects soil biological environments under field conditions. For example, the O2 release from rice made the rhizosphere with high specific rates of various microbial transformations under field condition (Revsbech et al., Citation1999). In a real rice paddy field, these microbial activities will hinder the estimation of oxygen release from crop species, and therefore, the measurement of O2 release will be usually conducted under the laboratory conditions. Exposing the aboveground part of a plant to atmospheric N2 prevents O2 transport from the atmosphere to the roots through the aerenchyma, generating a condition where ROL does not occur (Lee, Citation2003). Maricle and Lee (Citation2007) applied the method to evaluate internal O2 transport by measuring the difference between oxygen absorption when ROL did or did not occur. Their method enabled us to assess a possible O2 transfer phenomenon that could mitigate the flood stress of drought-adapted upland crops.
The objective of this study was to confirm whether O2 can be transferred from rice to upland crops through their roots at the stage examined using the model system of hydroponic culture. For this purpose, the experiment was conducted in a climate chamber to estimate the amount of O2 transferred between rice and pearl millet (Pennisetum glaucum) roots. We verified the O2 transfer between rice and pearl millet at least in our experimental system based on differences in O2 concentration between single and mixed planting measured by a fiber optic O2-sensing probe.
Materials and methods
Rice (O. sativa cv. Nipponbare) was used as the flood tolerant crop, and it is extensively used as an experimental material in Japan. Pearl millet (P. glaucum cv. Okashana 2) was used as the drought-adapted crop, which is the recommended cultivar in semiarid southern African countries. Rice and pearl millet seeds were surface sterilized with 2.5% (v/v) sodium hypochlorite for 5 min and rinsed in running water for 20 min. Rice seeds were soaked in distilled water, whereas pearl millet seeds were sown on paper towels in 23 × 23 cm Petri dishes. Seeds were pre-germinated in a dark incubator at 30 °C for 14 and 48 h for pearl millet and rice, respectively.
The O2 transfer between rice and pearl millet was analyzed by growing the seedlings in a nutrient solution (Miyamoto et al., Citation2001) without soil that might disturb root respiration measurements. The nutrient solution contained .09 mM (NH4)2SO4, .05 mM KH2PO4, .05 mM KNO3, .03 mM K2SO4, .06 mM Ca(NO3)2, .07 mM MgSO4, .11 mM Fe-EDTA, 4.6 μM H3BO3, 1.8 μM MnSO4, .3 μM ZnSO4, .3 μM CuSO4 (pH 5.5–6.0). The seedlings were grown in a plant growth room with a 28/23 °C day/night temperature, a 14 h photoperiod, and a 318 ± 2 μmol m−2 s−1 photosynthetically active radiation (PAR) on the top of the canopy. The PAR value is almost same as those in previous reports to evaluate oxygen release (root respiration) in the laboratories for growing Phragmites australis (Armstrong et al., Citation2000), O. sativa (Rubinigg et al., Citation2002), and Zizania latifolia (Nakamura et al., Citation2013). Pre-germinated rice seeds were sown on a screen mesh and placed on the bottom of each plastic seedling tray compartment (37 × 37 × 46 mm, length × width × height) and then covered with a thin plastic filling to avoid seed drying and light penetration into the root system. They were then placed in a plastic container (300 × 210 × 50 mm for 0–7 d after sowing (DAS); 410 × 290 × 60 for 7–14 DAS) filled with aerated deionized water. The averaged day water temperature was 30.5 °C and the night water temperature was 27.5 °C during the plant growth period. From 5 DAS, the seedlings were grown in the nutrient solution dissolved in deionized water. At 14 DAS, the seedlings were transferred to another plastic container (295 × 130 × 240 mm) and grown until the measurement. The solution was renewed every 2–3 d, and the pH was adjusted to 6.0. The 4- and 3-week-old rice and pearl millet were sampled, and their shoot bases were fixed tightly in silicon lids on top of individual root chambers made of glass (Figure ; 118 × 47 mm, height × diameter) and placed inside a gas exchange chamber (Figure ; 750 × 450 × 450 mm). The root growth chamber was filled with 150 mL of distilled water. This chamber was equipped with a fiber optic O2-sensing probe (FireSting O2 Fiber-Optic O2 Meter with the OXROB3, PyroScience GmbH, Germany) and a temperature sensor (K320, Takeyama Kagaku, Japan) which was placed at about 5 mm below the root system. Further, an air-bubbling inlet tube and an exhaust tube were placed to relieve the pressure during air-bubbling. The distilled water was continuously stirred using a magnetic stirrer. The water temperature inside the root chamber was precisely controlled using a water bath equipped with a cooler connected to a pump (9 L min−1), and the water was circulated using four mini-pumps at 4 L min−1 (Table ). Air in the gas exchange chamber was mixed continuously by two fans (Table ). The CO2 concentration was monitored using a portable photosynthesis analyzer (LCpro SD, ADC BioScientific, UK). The O2 release from the plant roots was evaluated during the following two continuous phases (Lee, Citation2003; Maricle & Lee, Citation2007):
Figure 1. Schematic diagram of the root chamber. For root chamber, an air-bubbling inlet tube and an exhaust tube were placed to relieve the pressure during air-bubbling. The distilled water was continuously stirred using a magnetic stirrer.
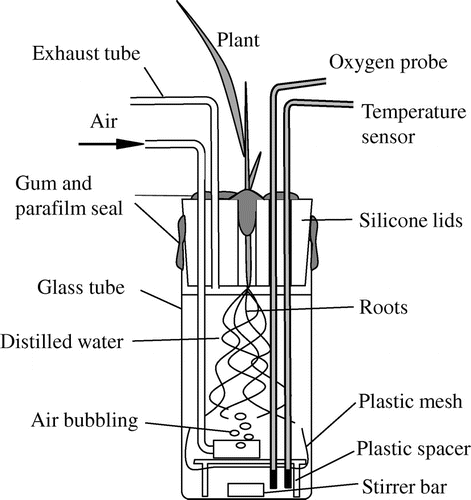
Figure 2. Schematic diagram of the gas exchange chamber. The water temperature inside the root chamber was precisely controlled using a water bath, and the water was circulated using four mini-pumps at 4 L min−1. Air in the gas exchange chamber was mixed continuously by two fans.
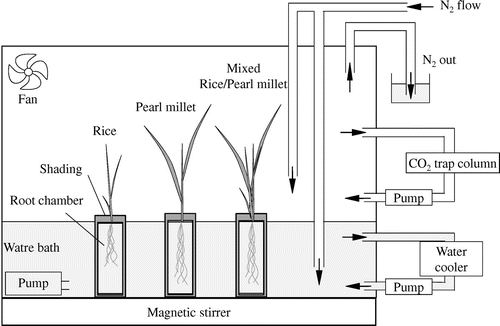
Table 1. Air temperature in a gas exchange chamber and water temperature in a root chamber.
Phase I–Light (photosynthetically active radiation of 168.9 ± 1.6 μmol m−2 s−1) and ambient air conditions were maintained in a normal state to allow photosynthesis for 30 min. Distilled water inside the root chamber was continuously air-bubbled, starting from setting up the root system until the beginning of phase I, and the dissolved O2 concentration was then measured continuously for 30 min (Figure ). In all of the measurements, phase I started at 1300 h of the day. Phase II–The gas exchange chamber O2 level was reduced to less than .8% by pumping N2 into the chamber at 50 L min−1 for 10 min before the start of phase II at 1400 h of the day (Figure ); subsequently, the flow rate was reduced to 5 L min−1 for the entire phase. Photosynthesis was stopped by creating darkness by covering the chamber with plastic shedding (photosynthetically active radiation of .1 ± .0 μmol m−2 s−1), and CO2 was removed continuously from the column. Other conditions were the same as in phase I. After the O2 transfer measurements, fresh weight of shoots and roots, number of leaves, and tillering number of the plants were measured. Several investigators including Colmer et al. (Citation1998) used root surface area-based concentration; however, they used only single root axis not whole root system for the estimation of root surface area. The majorities of studies dealing with whole root system used root fresh/dry weight-based concentrations, and thus, the root surface area was not measured. All statistical analyses were performed using Excel Statistics Version 2012 software (SSRI).
Results and discussion
O2 transfer between mixed plants
Awala et al. (Citation2016) reported that the productivity in drought-adapted crops of close mixed planting with rice was higher than that of normal single planting under flooding condition in the field experiment. The mitigation effects of rice on flood stress for drought-adapted crops would most probably be attributed to the relatively O2 rich environments and/or O2 transfer when pearl millet was closely grown with rice (Iijima et al., Citation2016). To confirm the hypothesis, O2 transfer was evaluated under a water culture condition without soil. Figure illustrates the phenomena of O2 absorption and release by plants in a water culture. O2 normal diffusion (nD) from aboveground to water, O2 reverse diffusion (rD) from water to aboveground, and root respiration (R) are the factors that affect O2 concentration in a water culture. In addition to these factors, O2 transfer (T) between different neighboring species can be considered to exist under mixed planting conditions. Rice roots are known to release O2 from the shoot system via the aerenchyma (Yamauchi et al., Citation2013). Upland crop species also release O2 (Maricle & Lee, Citation2007), although the amount is smaller (Figure , Single pearl millet) compared to rice. The O2 released from rice roots is most probably absorbed by the neighboring upland species due to their higher O2 demand caused by a lower O2 supply from shoots. O2 released by pearl millet may be absorbed by rice as well. In order to accurately compare O2 absorption/release between single planting and mixed planting, we combined the two single-species crops of rice and pearl millet immediately before the experiment (defined as mixed planting in this study). The only difference between single planting and mixed planting was whether the roots of the two different species stayed together during the short period of measurement or not. For this purpose, plants with similar agronomical traits (fresh weight of shoot and root and the number of leaf and tiller) were selected (Table ) for both single- and mixed- planting crops. Thus, the morphological characteristics [e.g. ROL barrier formation near the root tips (Shiono et al., Citation2011)] of mixed planting should be equivalent to those of single planting.
Figure 4. Illustration of O2 absorption and release by rice and pearl millet roots in the root chamber. The solid line and broken line represent the O2 movement in phase I and phase II, respectively. R, respiration; nD, normal diffusion; rD, reverse diffusion; T, transfer.
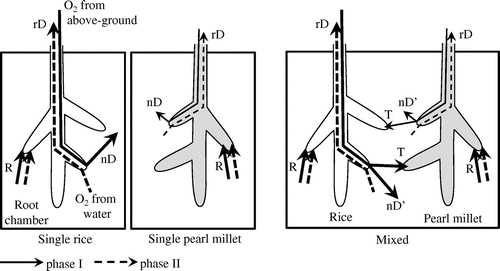
Table 2. Growth traits of plants used for O2 transfer measurements.
The CO2 level was adjusted to 527 ± 6 μmol mol−1 by using a soda lime absorbent column at the start of phase I (Table ). O2 transfer between the two species can be evaluated by measuring O2 changes in the water culture when shoots were grown under O2-rich (20.0 ± .0% conc. in phase I) and O2-free dark (.8 ± .0% conc. in phase II) conditions (Figure ). In phase I, the plants absorb dissolved O2 in the water for root respiration and the roots simultaneously release O2 as normal diffusion from aboveground to water due to the O2-rich condition in the shoot system (Figure ). Thus, O2 changes in phase I in the cultured water can be expressed as follows;
Table 3. O2 and CO2 gas concentrations in a gas exchange chamber.
Figure 5. Dissolved O2 concentration of the distilled water in the root chamber (Figure ) where plant roots were situated (n = 21) during the two 30-min consecutive phases: O2-rich light phase (Phase I) and O2-poor dark phase (Phase II) shoot environment. In order to accurately compare O2 absorption/release between single and mixed, the two single plants of rice and pearl millet were combined immediately before the experiment to measure O2 transfer.
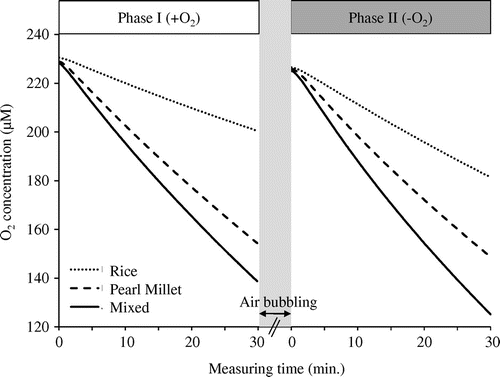
(1)
In phase II, the plants absorb dissolved O2 from the water as was noted in phase I, and the shoots simultaneously release O2 as reverse diffusion from water to aboveground due to the O2-poor conditions in the shoot system (Figure ). According to Lee (Citation2003), the root respiration (R) during Phase I can be assumed to be similar to that in phase II. Thus, the O2 changes in phase II in the cultured water can be described as,
(2)
Therefore, (Equations (Equation1(1) )–(Equation2
(2) )) is,
(3)
The difference in O2 changes between phase I and phase II indicates the sum of normal diffusion and reverse diffusion. Equation (Equation3(3) ) can be expressed by the total single planting of rice and pearl millet (Total single) and mixed planting (Mix), respectively, as follows;
(4)
where r is rice and p is pearl millet.
(5)
where mix is mixed planting of rice and pearl millet.
As mentioned above, in this experiment, morphological characteristics of mixed planting during the short period of measurement were not quantitatively different from that of single planting. Because the two plants were combined just before the experiment, rD was assumed to be the species characteristic value, and the total rD of single planting rice and pearl millet {rD (r + p)} was not different from that of mixed planting {rD (mix)}. Therefore, (Equations (Equation4(4) )−(Equation5
(5) )) can be expressed as follows;
(6)
Similarly, the total amount of released O2 was also assumed to be the species characteristic value. The O2 released from the roots by mixed planting can be classified into two categories: that remaining in the cultured water (residual component, indicated as nD’ in Figure ) and that transferred to different neighboring species (O2 transfer component, indicated as T in Figure ). On the other hand, the O2 released from the roots by single planting is represented only by normal diffusion. Thus, the sum of O2 released by the two single-species seedlings {nD (r + p)} will be equivalent to the total O2 released by the mixed two seedlings {nD’ (mix) + T (mix)} as follows;
(7)
Equation (Equation7(8) ) is substituted into (Equation6
(7) ) as follows;
(8)
O2 transfer between rice and pearl millet was represented by the difference of O2 changes (phase I−II) between single planting of rice and pearl millet and mixed planting. In this study, the sum of the O2 changes (phase I−II) in single planting of rice and pearl millet was significantly higher than that of mixed planting of rice and pearl millet (Figure ; p < .01), which indicated that O2 was transferred between rice and pearl millet under a water culture condition, at least in our experimental system. These results indicated that approximately 7 μM O2 g fresh root weight−1 h−1 was transferred between the two plant species, and most of them should be transferred from rice to pearl millet.
Figure 6. Changes in O2 concentration in the distilled water in the root chamber (Figure ) where rice and pearl millet roots in single planting or mixed planting were situated (n = 21). An asterisk indicates a significant difference by a Student’s t-test at the p < 0.01 level. The difference between phase I and phase II indicates the sum of diffusion (nD + rD), and those between single total and mixed indicates the oxygen transfer between the two species {T (mix)}.
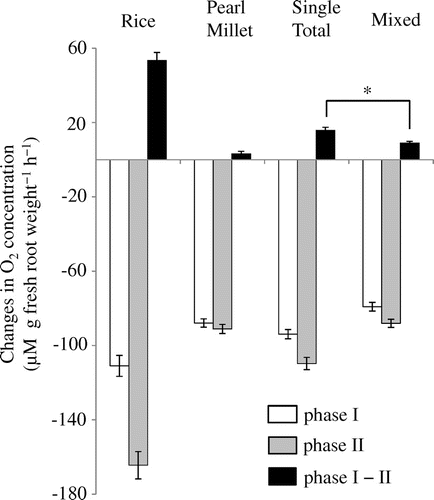
Technical evaluation for O2 transfer
The flood mitigation effects observed in previous studies (Awala et al., Citation2016; Iijima et al., Citation2016) for drought-adapted upland crops could be attributed to the O2 transferred from the roots of flood-adapted to drought-adapted crops. To date, O2 release can be analyzed using several methods (Colmer, Citation2003; Wu et al., Citation2014). This study used a fiber optic O2-sensing probe (Maricle & Lee, Citation2007) to measure the O2 net flux from the whole root system to the O2-rich (228 ± .4 μM) water environment, which has not been used before. So far, low O2 concentrations, i.e. 15 μM (Sorrell & Armstrong, Citation1994), 60–70 μM (Lee, Citation2003), or 120 μM (Maricle & Lee, Citation2007), were used at the start of the experiments; however, the root respiration rates of the drought-adapted upland species used in this study were significantly reduced under such a low O2 concentration range within 30 min. Thus, estimating O2 release at low O2 concentrations was considerably difficult. In this study, seedlings grown in the water culture without a soil medium were used for the estimation of O2 transfer; this might be different from the actual O2 released by a tangled root system grown in soil. In the case of mixed planting, the root surface sorption zone was partly disturbed by the mixing of different species, whereas that of the tangled root systems of the close mixed seedlings grown in soil would be significantly disturbed by the mixed species during growth. For example, the root hairs of epidermal cells of the two species may become entangled with each other and with soil particles. Thus, the living sloughed root cap cells on the root surface during root penetration (Iijima et al., Citation2000, Citation2004) will also interact with those from the mixed neighboring species. Further study using a real soil system is required to confirm this phenomenon in fields.
Disclosure statement
No potential conflict of interest was reported by the authors.
Funding
This work was supported by the Japan Science and Technology (JST) and the Japan International Cooperation Agency (JICA).
Acknowledgements
We thank the members of the projects entitled ‘Flood- and Drought-adaptive Cropping Systems to Conserve Water Environments in Semi-arid Regions’ by the framework of the ‘Science and Technology Research Partnership. We also thank all the students of Kindai University for their assistance.
References
- Armstrong, W. (1979). Aeration in higher plants. Advances in Botanical Research, 7, 225–332. doi:10.1016/S0065-2296(08)60089-0
- Armstrong, W., Cousins, D., Armstrong, J., Turner, D. W., & Beckett, P. M. (2000). Oxygen distribution in wetland plant roots and permeability barriers to gas-exchange with the rhizosphere: A microelectrode and modelling study with Phragmites australis. Annals of Botany, 86, 687–703. doi:10.1006/anbo.2000.1236
- Awala, S. K., Yamane, K., Izumi, Y., Fujioka, Y., Watanabe, Y., Wada, K. C., … Iijima, M. (2016). Field evaluation of mixed-seedlings with rice to alleviate flood stress for semi-arid cereals. European Journal of Agronomy, 80, 105–112. doi:10.1016/j.eja.2016.07.003
- Brooker, R. W., Bennett, A. E., Cong, W. F., Daniell, T. J., George, T. S., Hallet, P. D., … White, P. J. (2015). Improving intercropping: A synthesis of research in agronomy, plant physiology and ecology. New Phytologist, 206, 107–117. doi:10.1111/nph.13132
- Colmer, T. D. (2003). Long-distance transport of gases in plants: A perspective on internal aeration and radial oxygen loss from roots. Plant Cell and Environment, 26, 17–36. doi:10.1046/j.1365-3040.2003.00846.x
- Colmer, T. D., Gibberd, M. R., Wiengweera, A., & Tinh, T. K. (1998). The barrier to radial oxygen loss from roots of rice (Oryza sativa L.) is induced by growth in stagnant solution. Journal of Experimental Botany, 49, 1431–1436. doi:10.1093/jxb/49.325.1431
- Ehrmann, J., & Ritz, K. (2014). Plant: Soil interactions in temperate multi-cropping production systems. Plant and Soil, 376, 1–29. doi:10.1007/s11104-013-1921-8
- Iijima, M., Awala, S. K., Watanabe, Y., Kawato, Y., Fujioka, Y., Yamane, K., & Wada, K. C. (2016). Mixed cropping has the potential to enhance flood tolerance of drought-adapted grain crops. Journal of Plant Physiology, 192, 21–25. doi:10.1016/j.jplph.2016.01.004
- Iijima, M., Griffiths, B., & Bengough, A. G. (2000). Sloughing of cap cells and carbon exudation from maize seedling roots in compacted sand. New Phytolgist, 145, 477–482. doi:10.1046/j.1469-8137.2000.00595.x
- Iijima, M., Higuchi, T., & Barlow, P. W. (2004). Contribution of root cap mucilage and presence of an intact root cap in maize (Zea mays) to the reduction of soil mechanical impedance. Annals of Botany, 94, 473–477. doi:10.1093/aob/mch166
- Lee, R. W. (2003). Physiological adaptations of the invasive cordgrass Spartina anglica to reducing sediments: Rhizome metabolic gas fluxes and enhanced O2 and H2S transport. Marine Biology, 143, 9–15. doi:10.1007/s00227-003-1054-3
- Lithourgidis, A. S., Dordas, C. A., Damalas, C. A., & Vlachostergios, D. N. (2011). Annual intercrops: An alternative pathway for sustainable agriculture. Australian Journal of Crop Science, 5, 396–410.
- Maricle, B. R., & Lee, R. W. (2007). Root respiration and oxygen flux in salt marsh grasses from different elevational zones. Marine Biology, 151, 413–423. doi:10.1007/s00227-006-0493-z
- Miyamoto, N., Steudle, E., Hirasawa, T., & Lafitte, R. (2001). Hydraulic conductivity of rice roots. Journal of Experimental Botany, 52, 1835–1846. doi:10.1093/jexbot/52.362.1835
- Nakamura, M., Nakamura, T., Tsuchiya, T., & Noguchi, K. (2013). Functional linkage between N acquisition strategies and aeration capacities of hydrophytes for efficient oxygen consumption in roots. Physiologia Plantarum, 147, 135–146. doi:10.1111/j.1399-3054.2012.01643.x
- Ramert, B., Lennartsson, M., & Davies, G. (2002). The use of mixed species cropping to manage pests and diseases – Theory and practice. In Proceedings of the UK Organic Research 2002 Conference, Organic Centre Wales, Institute of Rural Studies, University of Wales Aberystwyth, 207–210.
- Ramirez-Garcia, J., Martens, H. J., Quemada, M., & Thorup-Kristensen, K. (2015). Intercropping effect on root growth and nitrogen uptake at different nitrogenlevels. Journal of Plant Ecology, 8, 380–389. doi:10.1093/jpe/rtu024
- Revsbech, N. P., Pedersen, O., Reichardt, W., & Briones, A. (1999). Microsensor analysis of oxygen and pH in the rice rhizosphere under field and laboratory conditions. Biology and Fertility of Soils, 29, 379–385. doi:10.1007/s003740050568
- Rubinigg, M., Stulen, I., Elzenga, J. T. M., & Colmer, T. D. (2002). Spatial patterns of radial oxygen loss and nitrate net flux along adventitious roots of rice raised in aerated or stagnant solution. Functional Plant Biology, 29, 1475–1481. doi:10.1071/FP02081
- Shiono, K., Ogawa, S., Yamazaki, S., Isoda, H., Fujimura, T., Nakazono, M., & Colmer, T. D. (2011). Contrasting dynamics of radial O2-loss barrier induction and aerenchyma formation in rice roots of two lengths. Annals of Botany, 107, 89–99. doi:10.1093/aob/mcq221
- Sorrell, B. K., & Armstrong, W. (1994). On the difficulties of measuring oxygen release by root systems of wetland plants. Journal of Ecology, 82, 177–183. doi:10.2307/2261396
- Vandermeer, J. (1989). The ecology of intercropping. Cambridge: Cambridge University Press.10.1017/CBO9780511623523
- Wolfe, M. S. (2000). Crop strength through diversity. Nature, 406, 681–682. doi:10.1038/35021152
- Wu, H., Liu, J., Zhang, J., Li, C., Fan, J., & Xu, X. (2014). Comparative quantification of oxygen release by wetland plants: Electrode technique and oxygen consumption model. Environmental Science and Pollution Research, 21, 1071–1078. doi:10.1007/s11356-013-1984-3
- Yamauchi, T., Shimamura, S., Nakazono, M., & Mochizuki, T. (2013). Aerenchyma formation in crop species: A review. Field Crops Research, 152, 8–16. doi:10.1016/j.fcr.2012.12.008