Abstract
Stevia rebaudiana Bertoni is a perennial medicinal herb indigenous to tropical regions of South America. The present study evaluated some physiological changes of the plant under cold in the absence and the presence of polyamine conditions. The results showed photosynthetic pigments and photochemical efficiency of PSII reduced in cold treatment conditions than control, significantly. However, a considerable increase in the parameters was observed in all of polyamine treated goups. Leaf compatible solutes such as proline and glycine betaine that were not shown significantly increasing at cold treatment groups, elevated in the 2 days cold-polyamine-treated group. The similar results were seen for free amino acid and total protein contents of the treated plants. It was also revealed that specific activity of some antioxidant enzymes such as catalase and ascorbate peroxidase increased in cold-treated or cold + polyamine-treated groups than related controls. It caused a significant decrease in H2O2 and malondialdehyde contents of the treated plants. The results indicated an increasing in saturated long chain fatty acids after 4 days of cold at 4 °C. It was concluded that the polyamine supplement can induced a considerable tolerance in stevia plants at cold condition.
Keywords:
Classification:
Introduction
Stevia rebaudiana Bertoni (Asteraceae family) is a well-known medicinal plant with perennial habit. It has been used in many countries for prevention of hyperglycemia. This pharmacological effect is attributed to the presence of certain diterpenic glycosides with the very sweet test. These substances were named as steviol glycosides and can be used for diabetes or PKU patients without any side effects in comparison with artificial sweeteners. Hence, the plant cultivation is considered in many parts of the world. The plant is indigenous to tropical and subtropical regions of South America (Lemus-Mondaca et al., Citation2012). However, there are a few studies subjecting to stevia resistance to cold stress or hardening before the cultivation of this plant in regions with a risk of chilling or frost (Moraes et al., Citation2013; Soufi et al., Citation2016). Cold stress is regarded as main limiting factor for stevia cultivation, especially in climates with severe cold winters. Polyamines are molecules with protective direct effect and indirect regulatory functions on plants under abiotic stress conditions (Pál et al., Citation2015). Stress induced or exogenously applied polyamines can enhance plant tolerance to the different abiotic stress conditions (Groppa & Benavides, Citation2008).
Because stevia plant is native to the tropical region, further investigations are needed to evaluate the plant responses and yields at cold condition. There are few reports in the literature on effect of polyamines on stevia. The synergistic effects of polyamines with plant hormones on the increase of phenolic, antioxidant activity, and steviol glycosides have been previously described (Khalil et al., Citation2016). The ameliorating effect of polyamines on the effects of heavy metal stress in the stevia plant has also been reported (Prasann et al., Citation2012). This study aims to physiological and biochemical response of stevia at cold condition (4 °C) and the effects of the polyamine supplement to modify the response to combat the cold stress. It may be tended to cultivation possibility of the plant in the highlands and cold regions. In the present work, some physiological and biochemical parameters that were changeable in plants at cold condition such as osmotic compatible solutes, Ros, and antioxidant enzymes activity were mesured.
Material and methods
Stevia growth condition and treatments
Tow month old stevia plants were purchased from Nahal Gostar Royan plant nursery (Qazvin, Iran). The plants were grown at 25 °C under 16/8 h (L/D) photoperiod with 85 μmol m−2 s−1 light intensity. The cold room temperature was reduced 5 °C per hour to reach 4 °C. All of experiment carried out in a completely randomized design with three replications in 6 groups as: control group (before of cold; 25 °C), 48 and 96 h of cold treatment groups and polyamine supplement-treated groups before cold and after 48 and 96 h of cold treatments. Liquid polyamine supplement (Baspar Danesh Sabz Co.-DUPINIX™ Galaxy A4 45%, Iran) that composed of synthetic polyamines ‘diamino hydroxyl ethyl phospho mineral and diamino hexanoic mineral amino ethanoic acid’, was applied twice with four-day interval at a concentration of 4 ml L−1at the vegetative stage of the plants. The supplement was dispersed in water using 100 μL of tween 20. Three days after second polyamine application leaves were harvested and held in −80 °C freezer for the analyses.
Photosynthetic parameters
Relative chlorophyll content (SPAD chlorophyll) and chlorophyll fluorescence parameters were measured using a chlorophyll meter (CL-01model, Hansatech instrument, UK) and Chlorophyll Fluorimeter (PEA model, Hansatech instrument, UK), respectively. Photosynthetic pigments including Chl a, Chl b and carotenoids were measured according Arnon (Citation1949) method. For this purpose, acetone (80%) extract of samples was centrifuged (6440 g) for 10 min. The supernatant was used for measuring of absorbance at 663, 645, and 470 nm to determine pigment content (mg g−1 FW).
Enzyme activity assay
A spectrophotometric method was used for the measurement of enzyme-specific activity. Protease activity was assayed according to Penner and Aston (Citation1969) method via reduction of casein solution after the proteolytic activity of enzyme extraction. Catalase specific activity was conducted according method of Aebi (Citation1984). Ascorbate peroxidase and polyphenol oxidase were done according to Nakano and Asada (Citation1981) and Raymond et al. (Citation1993) methods respectively.
Measurement of H2O2 content
H2O2 content was determined according to Velikova et al. (Citation2000). Half of a gram fresh leaf tissue was homogenized in ice bath with 5-ml trichloroacetic acid (TCA) .1% (w/v). After centrifuging (4472 g for 15 min), .5 ml of supernatant was mixed with .5 ml of 10 mM phosphate buffer (pH: 7) and 1 ml of 1 M KI. Then mixture absorbance at 390 nm was measured. Serial dilutions of H2O2 concentration were used for standard calibration curve.
Malondialdehyde determination
Lipid peroxidation was determined according to Heath and Packer (Citation1968) method. According of this method, 250 mg of fresh leaves homogenized in mortar and pestle with 5 ml of TCA (.1%). Homogenate was centrifuged 40,248 g for 15 min. One milliliter of supernatant was mixed with 3 ml of .5% thiobarbituric acid (TBA) in 20% TCA and incubate in water bath at 100 °C for 30 min. Tubes were placed in an ice bath for termination of the reaction. Sample absorptions were measured at 532 nm. Nonspecific absorption of samples at 600 nm were subtracted from 532 nm absorption. The malondialdehyde (MDA) content in samples were calculated from the extinction coefficient of 155 mM−1 cm−1 for MDA–TBA abduct.
Total protein and free amino acid extraction
Total protein was extracted and quantified by the method of Bradford (Citation1976) using bovine serum albumin as the standard protein. Free amino acid was measured by Hwang and Grace (Citation1975) method. About .1 g of sample homogenized in 5 ml of 50 mM phosphate buffer (pH: 6.8) and centrifuged at 4472 g for 15 min. Supernatant was mixed with 1 ml of ninhydrin reagent. After 10 min incubation in 70 °C water bath, absorbance of samples was measured at 570 nm. The amount of free amino acids was calculated using glycine standard curve.
Extraction of steviol glycosides
Hundred milligrams of dried leaves were grounded and boiled in 5 ml of distilled water and filtered through Whatman filter paper then decolorized by activated charcoal. Then samples were measured at 210-nm wavelength by spectrophotometer. The Rebaudioside A (Sigma) standard prepared to draw the calibration curves (Rajab et al., Citation2009).
Proline determination
For determination of free proline content, the method of Bates et al. (Citation1973) was used. About .5 g of leaf samples were homogenized in 3% aqueous sulphosalycylic acid, then the homogenate filtered through Whatman filter paper. Two ml of filtrate was mixed with 2 ml of ninhydrin reagent and 2 ml of acetic acid in a test tube and incubate for 60 min at 100 °C. The reaction terminated by ice-cold bath then the mixture was vortexed with 4-ml toluene. Toluene phase was used for proline assay at 520 nm. Proline concentration was determined using calibration curve and expressed as μmol proline g−1 fresh weight (FW).
Glycine betaine determination
Two hundred and fifty milligrams of leaf fresh tissue were grounded in mortar and pestle with liquid N2 and shaken with 4 ml of distilled water for 24 h at 25 °C. Samples after filtration were mixed 1:1 with 2 N sulfuric acid. About .5 ml of the aliquots were incubated in ice bath for 1 h and .1 ml of KI3 reagent (7.85 g of I2 with10 g of KI in 50-ml water) was added to the tubes and incubate at 4 °C for 24 h. Tubes were centrifuged at 4472 g at 4 °C for 30 min. Precipitates were dissolved in 7 ml of 1,2-dichloroethane. Then absorption of samples at 365 nm were measured (Grieve & Grattan, Citation1983). The amount of betaine in samples quantify using of betaine standard curve.
Fatty acid assay
Methanolic (75%) extract of 300 mg of stevia leaves after homogenization with liquid nitrogen was sonicated at 70 °C for 60 min. Samples were centrifuged at 12,000 g for 10 min. Then 2 ml of supernatant was lyophilized. For silylation reaction, 90 μL BSTFA reagent (with TMCS 1%) with 100-μL hexane were added to lyophilized samples. Then, the vials were tightened by caps and heated for 45 min at 75 °C. Ten microliters of samples were injected to GC Ms. For quantification, decanoic acid (capric acid) as internal standard was added to the samples.
The silylated samples were analyzed using an Agilent 7890B series GC with a fused methyl silicon HP-5 MS column (30 m × .25 mm i.d., .25 μm film thickness) and fitted with Agilent 5977 A Series MS system. Helium was used as carrier gas at a flow rate of 1 mL/min. The programmed temperature was increasing from 50 to 320 °C at a rate of 4 °C/min and finally held for 10 min. The injector temperature was 320 °C with split less condition. The mass spectral (MS) data were obtained at the following conditions: ionization potential 70 eV; ion source temperature 200 °C; quadrupole temperature 100 °C; solvent delay 3 min; scanning rate of .4sl mass range of 40–460 amu. EM voltage 3000 volts. Identification of compounds was based on direct comparison of MS data with those for standard compounds, and computer matching with the NIST NBS54 K Library and Wiley Library.
Statistical analyses
All of experiments were conducted at three replicates. All data were statistically analyzed with SPSS software (ver. 16). Mean comparison was performed using Duncan’s multiple range test at the P ≤ .05 level of significance.
Results
Our observations indicated that there was no sign of wilting or necrosis spots on the stevia leaves after continuous cold stress for 2 and 4 days. However, application of polyamine supplement accompanied with cold stress caused a better growth condition in stevia plants (Figure S1, S2, and S3). The results also showed, whereas photosynthetic pigments were reduced in cold-treated groups, the pigments were significantly enhanced in all of polyamine treated groups than related controls (p ≤ .05). In the cold-treated stevia groups, chl a and chl b contents undergone 6–7% reduction than the control. However, 2 days cold along with polyamine significantly caused an increasing in photosynthetic pigments by about 71.4, 63.3, and 50% for chl a, chl b, and carotenoids in comparison with cold control (Figure A). Similarly, SPAD chlorophyll values increased in the same group to 53% (Figure B). The same manner was obvious in treated groups with 4 days of cold and polyamines.
Figure 1. Changes in photosynthetic pigments (A), leaf SPAD chlorophyll (B) and chlorophyll fluorescence (C) under cold (48 and 96 h) and cold-polyamine treatments.
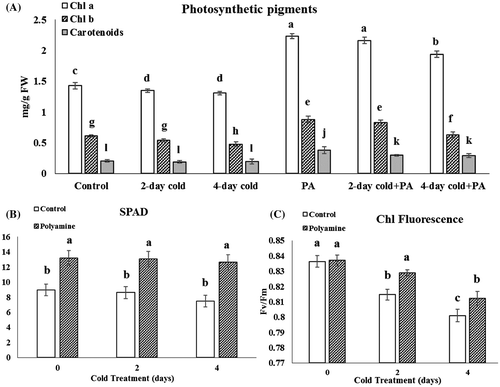
At the cold condition, the maximum photochemical efficiency of PSII (FV/Fm value) significantly reduced to .81 and .80 during 2 and 4 days, respectively. Polyamine enhanced the value to .83 and .81 at same cold courses (Figure C).
Our finding showed that cold treatment had no considerable effects on the hydrogen peroxide content of the stevia leaves, but a significant decrease in this parameter was measured in all polyamine-treated groups than related control at P ≤ .05. So that in the groups treated with cold accompanied by polyamine, hydrogen peroxide reduced to 62 and 74% at 2 and 4 days of cold courses, respectively (Figure A). The measurement of malondialdehyde of stevia samples indicated that cold stress for 2 and 4 days promote malondialdehyde synthesis by to 42 and 50% compared with the control, respectively. The treated groups with polyamines under same cold conditions exhibited reduced malondialdehyde content to 25 and 62% compared to net cold-treated group (Figure B).
Figure 2. Changes in H2O2 (A), malondialdehyde (B), content and catalase (C); polyphenol oxidase (D) and ascorbate peroxidase (E) under cold (48 and 96 h) and cold-polyamine treatments.
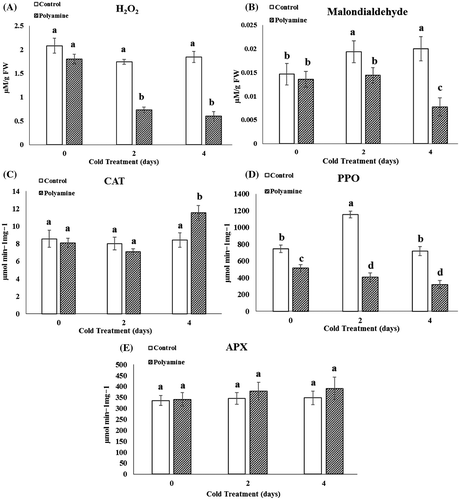
Analysis of the total steviol glycosides revealed that four-day cold treatment increased these substances by about 29% than control. On the other hand, stevia plants treated with cold-polyamines had more increased steviol glycosides at both cold treatment time courses (Figure A). The results indicated that cold stress in both treatment courses did not induced any considerable changes in proline and glycine betaine content of stevia leaves. On the contrary, in polyamine treated group followed with 48 h of cold, a significant increase of 41.1 and 289% was seen for proline and glycine betaine, respectively. Similar increasing was obvious for proline in the group treated with polyamine combined with 4 days of cold course (Figure B, C).
Figure 3. Changes in steviol glycosides (A), proline (B), and glycine betaine (C) content under cold (48 and 96 h) and cold-polyamine treatments.
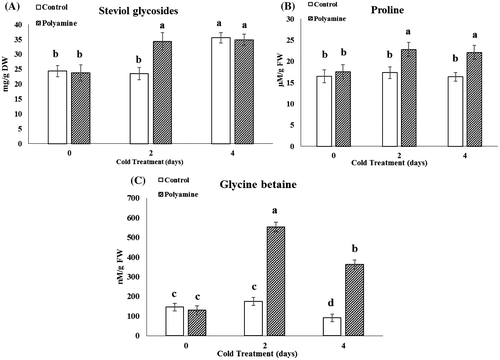
The results also showed activity of some antioxidant or hydrolytic enzymes such as catalase (CAT), ascorbat peroxidase (APX), polyphenol oxidase (PPO), and protease enhanced in the stevia plant groups treated with cold-polyamine. In the polyamine-treated group exposed to 4 days of cold, the specific activity of catalase, ascorbate peroxidase, and protease increased to 29.7, 15.2, and 14.2% compared with related control (Figures C–E and C).
Figure 4. Changes in Bradford total protein (A), free amino acids (B), and protease activity (C) under cold (48 and 96 h) and cold-polyamine treatments.
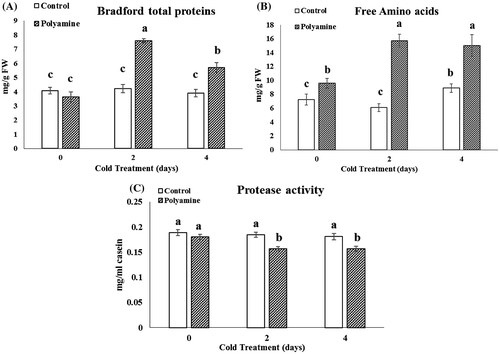
Results of Bradford total proteins assay showed that the combined treatment of polyamine and cold significantly increased protein content by 55 and 40% after 2 and 4 days of cold courses, respectively (Figure A). Cold stress for 4 days accompanied with polyamine significantly increased free amino acids by 15.6% in comparison with alone cold condition (Figure B).
GC-MS analyses revealed that cold stress induced long chain fatty acids such as palmitic, stearic, and myristic acids content in stevia leaves. However, in cold-polyamine- and polyamine-treated groups a significant declining in long chain fatty acids quantity was obvious (Table ).
Table 1. Changes in the fatty acid composition of stevia leaves under cold and polyamine supplement treatment after 96 h of exposure under 4 °C growth condition.
Discussion
Our findings tend to indicate that although Stevia rebaudiana Bertoni is regarded as a tropical herb, cold-treated plants for 2 and 4 days at 4 °C did not demonstrate significant visible damages. This may possibly be due to the fact that the plant might have a degree of tolerance to chilling.
The results herein reported showed that chilling temperature (4 °C) reduced the plants chlorophylls. It may be related to promoting ROS production by cold stress tending to impede some chlorophyll biosynthesis enzymes such as Mg-chelatase, Fe-chelatase, and protoporphyrinogen IX oxidase (Aarti et al., Citation2006). However, the polyamine supplement alleviated cold effects and considerably enhanced chlorophyll contents of cold intact plants. It might be attributed to the polyamines role in attenuating free radical or ROS induced damage (Von Deutsch et al., Citation2005). Our findings implied on severe increasing of hydrogen peroxide as a well-known ROS in stevia plants under chilling stress.
The levels of steviol glycosides after cold-polyamine treatment indicated that polyamine caused an increase due to synthesis and accumulation of the glycosides. It was previously documented that polyamines promote carbohydrate accumulation in plants at stressful conditions. Cold stress at 4 °C with putrescine treatment in zucchini fruit increased carbohydrate levels after 3 and 5 days (Palma et al., Citation2016). Sofy and Fouda (Citation2013) found that spermidine increased carbohydrate content in Helianthus tuberosus plant tuber under salt stress.
The accumulation of compatible osmolytes, such as proline, glycine betaine, and some other free amino acids is a common response in plants to tolerate water loss under various environmental osmotic stresses such as drought, salinity, and cold. In addition to osmotic balance, these solutes may play a vital protection role for plants in stabilizing cellular structures and reduce the damage caused by these stress conditions (Liu et al., Citation2013; Szabados & Savouré, Citation2010). This study demonstrated that cold treatments at 4 °C alone had not considerable induced proline or glycine betaine accumulation in stevia plants. However, the same cold conditions accompanied by polyamines stimulated stacking of the compatible solutes. It was previously pointed out that polyamines are potent to increase cry protective agents such as soluble sugars, proline, glycine betaine, other free amino acids, and metabolites in different plants (Alcázar et al., Citation2011). On the other hand, it is well known that exogenous application of polyamines induces polyamine biosynthesis process in plants from amino acid precursors and increase polyamine total level. Ornithine and arginine are the main precursors for polyamine biosynthesis and intermediate metabolites for proline biosynthesis. Other non-protein amino acids such as GABA and glycine betaine may be detected in the free amino acid pool under cold stress, as well as (Palma et al., Citation2014; Shelp et al., Citation2012). Increasing of 13 different amino acids and putrescine has been reported from maize under chilling condition (Janmohammadi et al., Citation2015; Szalai et al., Citation1997). In the present work, increasing of Bradford total protein and free amino acids contents was revealed in the s tevia plants treated with 4 °C cold combined with polyamines. The polyamine metabolism is related to hormonal biosynthesis pathways, nitrogen assimilation, and other developmental, physiological, and metabolic processes (Alcázar et al., Citation2010). It can also be said that stevia may use polyamines as a nitrogen and carbon source to synthesize proteins, amino acids, and osmolytes. Bagni et al. (Citation1978) has been shown that Helianthus tuberosus can utilize putrescine as a nitrogen source through catabolism or back-conversion of polyamines.
The results of the present study also indicated the elevation of malondialdehyde content in stevia leaves after cold treatment at 4 °C. Malondialdehyde is a characteristic sign for lipid peroxidation in the plants under stress conditions reflecting membrane system injuries and cellular metabolic deterioration caused by ROS and other free radicals (Liu et al., Citation2013). Our results showed that application of polyamine supplement before chilling can reduce malondialdehyde content of stevia leaves. It has been previously shown polyamine application on cucumber decrease malondialdehyde content and soluble proteins upon chilling, as well as (Zhang et al., Citation2009). Our results are consistent with Li et al. (Citation2014) reports that application of spermidine was effective in decreasing of malondialdehyde and H2O2 content using a increasing in glycine betaine level in plants under drought stress. It was previously shown that high level of glycine betaine in transgenic plants protects proteins and cell organelle membranes against low-temperature damages causing intense resistance to cold stress (Megha et al., Citation2014). Also Song et al. (Citation2014) reported putrescine caused decrease of malondialdehyde in tomato after 4 days of exposure to chilling. It is well known that glycine betaine like proline protects plants against chilling stress by ROS scavenging activity and water status maintenance. It can also used as a carbon and nitrogen source in plants (Giri, Citation2011).
The present study also demonstrates increasing of saturated fatty acids in stevia under cold stress and decreasing after polyamine or cold-polyamine treatments. A previous report indicated an alternation in fatty acid pattern of cold-sensitive and cold-tolerant genotypes of rice under cold stress. There was a considerable tendency of reduction in the unsaturated fatty acids and a rise in the saturated fatty acids in cold sensitive rice genotypes exposed to cold. In contrast, in cold tolerant genotypes, unsaturated fatty acids showed a rise, and saturated acids undergone a reduction. (Cruz et al., Citation2010). This may interpret the role of lipid unsaturation in the maintenance of cell membrane stability and then cold tolerance. It was assumed that in stevia plants treated with polyamines, desaturase enzymes might be more active to convert saturated fatty acids to unsaturated ones and intensify plasma membrane fluidity. Under chilling or freezing conditions, a declination in the plasma membrane fluidity may tend to transition of liquid-crystalline phase of membrane to a gel phase. It can cause various changes in cell metabolism and ultimately damage and death of cold sensitive plants (Uemura et al., Citation2006).
In some cold-sensitive tropical species chilling temperatures cause a serious damage in thylakoidal membranes through lipid peroxidation or phase alteration. Free radicals such as ROS are responsible for all of these damages. Under cold condition, PSII and photosynthetic linear electron transport inhibit and tend to a reduction in FV/Fm value (Paredes & Quiles, Citation2015). Our investigation indicated that polyamines moderated FV/Fm reduction in cold-treated plants. Similar results were reported for some other crops. Song et al. (Citation2014) reported putrescine increased FV/Fm value of tomato. Putrescine application increased photosynthesis and total protein in the periwinkle plant, as well as (Talaat et al., Citation2005). It was proposed that in addition to promoting antioxidant system, polyamines can induce aggregation of PSII antennae (LHC II) contributing plant stability under low-temperature condition (Tsiavos et al., Citation2012).
In stress condition, primary increasing of ROS tend to induce antioxidant enzymes in plant cells (Kolupaev et al., Citation2017). In this study, elevation in the activity of poly phenol oxidase was only seen in cold-treated plants that can be attributed to ROS rise. With continue cold condition, catalytic activity of PPO caused a reduction in the plant ROS content followed a drop in the enzyme activity. However, increment in the catalytic activities of catalase was obvious in stevia plants treated with 4 days of cold along with polyamines revealed polyamines role in stimulation of the enzyme. Elevated catalase activity in the treated plants is associated with decline of hydrogen peroxide activity. Antioxidant enzymes eliminate hydrogen peroxide to protect plants against its harmful effects on plant cell membranes and macromolecules. In the other hand, it can be proposed from the results that antioxidant enzymes induction and activity seems to be significantly different between cold-tolerant and cold-sensitive plants. In other words, each plant uses its own antioxidant system with its particular induction mechanisms. Increasing in the expression of antioxidant enzymes in response to cold is associated with extended resistance of the plants to cold stress (Caverzan et al., Citation2012). In addition to enzymatic systems, non-enzymatic antioxidant defense systems such as ascorbic acid, glutathione, phenolic compounds, non-protein amino acids, and tocopherols contribute to lowering of ROS, as well as (Gill & Tuteja, Citation2010).
The protease with proteolytic activity can be a response of stevia to low temperature for breakdown of proteins to the small and soluble peptide or amino acids. It can be responsible for osmotic pressure rise in cold condition.
Conclusion
It can be concluded that although Stevia rebaudiana indigenous to tropical and subtropical habitats, it can present a degree of tolerance to chilling with some physiological and biochemical mechanisms. In some parts of the world, such as California and Japan, stevia cultivation in cold regions has been successful (Shock, Citation1982).
However, application of polyamine supplement (Dupinix) before of cold stress tends to increase tolerance of the stevia to cold stress and inducing cold acclimation with increasing of compatible osmolytes, inducing antioxidant defense system, membrane stability, and upregulating many regulatory and structural proteins biosynthesis.
Disclosure statement
No potential conflict of interest was reported by the authors.
Supplemental data
The supplemental data for this article can be accessed at https://doi.org/10.1080/1343943X.2018.1437756.
TPPS_1437756_Supplementary_material.docx
Download MS Word (1.5 MB)References
- Aarti, P. D., Ryouichi, T., & Ayumi, T. (2006). Effects of oxidative stress on chlorophyll biosynthesis in cucumber (Cucumis sativus) cotyledons. Physiologia Plantarum, 128, 186–197.10.1111/ppl.2006.128.issue-1
- Aebi, H. (1984). Catalase in vitro. Methods in enzymology, 105, 121–126.10.1016/S0076-6879(84)05016-3
- Alcázar, R., Altabella, T., Marco, F., Bortolotti, C., Reymond, M., Koncz, C., … Tiburcio, A. F. (2010). Polyamines: Molecules with regulatory functions in plant abiotic stress tolerance. Planta, 231, 1237–1249.10.1007/s00425-010-1130-0
- Alcázar, R., Cuevas, J. C., Planas, J., Zarza, X., Bortolotti, C., Carrasco, P., … Altabella, T. (2011). Integration of polyamines in the cold acclimation response. Plant Science, 180, 31–38.10.1016/j.plantsci.2010.07.022
- Arnon, D. I. (1949). Copper enzymes in isolated chloroplasts. Polyphenoloxidase in Beta vulgaris. Plant physiology, 24, 1–5.
- Bagni, N., Calzoni, G. L., & Speranza, A. (1978). Polyamines as sole nitrogen sources for Helianthus tuberosus explants in vitro. New Phytologist, 80, 317–323.10.1111/NPH.1978.80.ISSUE-2
- Bates, L. S., Waldren, R. P., & Teare, I. D. (1973). Rapid determination of free proline for water-stress studies. Plant and Soil, 39, 205–207.10.1007/BF00018060
- Bradford, M. M. (1976). A rapid and sensitive method for the quantitation of microgram quantities of protein utilizing the principle of protein-dye binding. Analytical Biochemistry, 72, 248–254.10.1016/0003-2697(76)90527-3
- Caverzan, A., Passaia, G., Rosa, S. B., Ribeiro, C. W., Lazzarotto, F., & Margis-Pinheiro, M. (2012). Plant responses to stresses: Role of ascorbate peroxidase in the antioxidant protection. Genetics and Molecular Biology, 35, 1011–1019.10.1590/S1415-47572012000600016
- Cruz, R. P. D., Golombieski, J. I., Bazana, M. T., Cabreira, C., Silveira, T. F., & Silva, L. P. D. (2010). Alterations in fatty acid composition due to cold exposure at the vegetative stage in rice. Brazilian Journal of Plant Physiology, 22, 199–207.10.1590/S1677-04202010000300007
- Gill, S. S., & Tuteja, N. (2010). Reactive oxygen species and antioxidant machinery in abiotic stress tolerance in crop plants. Plant Physiology and Biochemistry, 48, 909–930.10.1016/j.plaphy.2010.08.016
- Giri, J. (2011). Glycinebetaine and abiotic stress tolerance in plants. Plant Signaling & Behavior, 6, 1746–1751.10.4161/psb.6.11.17801
- Grieve, C. M., & Grattan, S. R. (1983). Rapid assay for determination of water soluble quaternary ammonium compounds. Plant and Soil, 70, 303–307.10.1007/BF02374789
- Groppa, M. D., & Benavides, M. P. (2008). Polyamines and abiotic stress: Recent advances. Amino Acids, 34, 35–45.10.1007/s00726-007-0501-8
- Heath, R. L., & Packer, L. (1968). Photoperoxidation in isolated chloroplasts: I. Kinetics and stoichiometry of fatty acid peroxidation. Archives of Biochemistry and Biophysics, 125, 189–198.10.1016/0003-9861(68)90654-1
- Hwang, M. N., & Grace, Mary E. (1975). Rapid hippurate hydrolysis method for presumptive identification of group B streptococci. Journal of Clinical Microbiology, 1, 114–115.
- Janmohammadi, M., Zolla, L., & Rinalducci, S. (2015). Low temperature tolerance in plants: Changes at the protein level. Phytochemistry, 117, 76–89.10.1016/j.phytochem.2015.06.003
- Khalil, S. A., Kamal, N., Sajid, M., Ahmad, N., Zamir, R., Ahmad, N., & Ali, S. (2016). Synergism of polyamines and plant growth regulators enhanced morphogenesis, stevioside content, and production of commercially important natural antioxidants in Stevia rebaudiana Bert. In Vitro Cellular & Developmental Biology, 52, 174–184.10.1007/s11627-016-9749-6
- Kolupaev, Yu E, Firsova, E. N., Yastreb, T. O., & Lugovaya, A. A. (2017). The Participation of calcium ions and reactive oxygen species in the induction of antioxidant enzymes and heat resistance in plant cells by hydrogen sulfide donor. Applied Biochemistry and Microbiology, 53, 573–579.10.1134/S0003683817050088
- Lemus-Mondaca, R., Vega-Gálvez, A., Zura-Bravo, L., & Ah-Hen, K. (2012). Stevia rebaudiana Bertoni, source of a high-potency natural sweetener: A comprehensive review on the biochemical, nutritional and functional aspects. Food Chemistry, 132, 1121–1132.10.1016/j.foodchem.2011.11.140
- Li, Z., Peng, Y., Zhang, X. Q., Pan, M. H., Ma, X., Huang, L. K., & Yan, Y. H. (2014). Exogenous spermidine improves water stress tolerance of white clover (Trifolium repens L.) involved in antioxidant defence, gene expression and proline metabolism. Plant Omics, 7, 517.
- Liu, W., Yu, K., He, T., Li, F., Zhang, D., & Liu, J. (2013). The low temperature induced physiological responses of Avena nuda L., a cold-tolerant plant species. The Scientific World Journal, 2013, 1–7.
- Megha, S., Basu, U., & Kav, N. N. (2014). Metabolic engineering of cold tolerance in plants. Biocatalysis and Agricultural Biotechnology, 3, 88–95.10.1016/j.bcab.2013.11.007
- Moraes, R. M., Donega, M. A., Cantrell, C. L., Mello, S. C., & McChesney, J. D. (2013). Effect of harvest timing on leaf production and yield of diterpene glycosides in Stevia rebaudiana Bert: A specialty perennial crop for Mississippi. Industrial Crops and Products, 51, 385–389.10.1016/j.indcrop.2013.09.025
- Nakano, Y., & Asada, K. (1981). Hydrogen peroxide is scavenged by ascorbate-specific peroxidase in spinach chloroplasts. Plant and Cell Physiology, 22, 867–880.
- Pál, M., Szalai, G., & Janda, T. (2015). Speculation: Polyamines are important in abiotic stress signaling. Plant Science, 237, 16–23.10.1016/j.plantsci.2015.05.003
- Palma, F., Carvajal, F., Jamilena, M., & Garrido, D. (2016). Putrescine treatment increases the antioxidant response and carbohydrate content in zucchini fruit stored at low temperature. Postharvest Biology and Technology, 118, 68–70.10.1016/j.postharvbio.2016.03.009
- Palma, F., Carvajal, F., Jamilena, M., & Garrido, D. (2014). Contribution of polyamines and other related metabolites to the maintenance of zucchini fruit quality during cold storage. Plant Physiology and Biochemistry, 82, 161–171.10.1016/j.plaphy.2014.06.001
- Paredes, M., & Quiles, M. J. (2015). The effects of cold stress on photosynthesis in Hibiscus plants. Plos One, 10, e0137472.10.1371/journal.pone.0137472
- Penner, D., & Aston, F. M. (1969). Hormonal control of proteinase activity in squash cotyledons. Plant physiology, 42, 791–796.
- Prasann, K., Padmanabh, D., & Pallavi, S. (2012). Role of polyamine in combating heavy metal stress in Stevia rebaudiana Bertoni. under in vitro conditions. International Journal of Agriculture Environment & Biotechnology, 5, 193–198.
- Rajab, R., Mohankumar, C., Murugan, K., Harish, M., & Mohanan, P. (2009). Purification and toxicity studies of stevioside from Stevia rebaudiana Bertoni. Toxicology International., 16, 49.
- Raymond, J., Rakariyatham, N., & Azanza, J. L. (1993). Purification and some properties of polyphenoloxidase from sunflower seeds. Phytochemistry, 34, 927–931.10.1016/S0031-9422(00)90689-7
- Shelp, B. J., Bozzo, G. G., Trobacher, C. P., Chiu, G., & Bajwa, V. S. (2012). Strategies and tools for studying the metabolism and function of γ-aminobutyrate in plants. I. Pathway structure. Botany, 90, 651–668.
- Shock, C. C. (1982). Experimental cultivation of Rebaudi’s stevia in California ( Davis Agronomy Progress Report, 122). University of California.
- Sofy, M. R., & Fouda, H. M. (2013). Spermidine as modulator of growth, some metabolic activities and reproductive development of Helianthus tuberosus plants grown in two types of soil. Nature and Science, 11, 161–171.
- Song, Y., Diao, Q., & Qi, H. (2014). Putrescine enhances chilling tolerance of tomato (Lycopersicon esculentum Mill.) through modulating antioxidant systems. Acta Physiologiae Plantarum, 36, 3013–3027.10.1007/s11738-014-1672-z
- Soufi, S., D -Urso, G., Pizza, C., Rezgui, S., Bettaieb, T., & Montoro, P. (2016). Steviol glycosides targeted analysis in leaves of Stevia rebaudiana (Bertoni) from plants cultivated under chilling stress conditions. Food Chemistry, 190, 572–580.10.1016/j.foodchem.2015.05.116
- Szabados, L., & Savouré, A. (2010). Proline: A multifunctional amino acid. Trends in Plant Science, 15, 89–97.10.1016/j.tplants.2009.11.009
- Szalai, G., Janda, T., Bartok, T., & Paldi, E. (1997). Role of light in changes in free amino acid and polyamine contents at chilling temperature in maize (Zea mays). Physiologia Plantarum, 101, 434–438.10.1111/ppl.1997.101.issue-2
- Talaat, I. M., Bekheta, M. A., & Mahgoub, M. H. (2005). Physiological response of periwinkle plants (Catharanthus roseus L.) to tryptophan and putrescine. International Journal of Agriculture and Biology, 7, 210–213.
- Tsiavos, T., Ioannidis, N. E., & Kotzabasis, K. (2012). Polyamines induce aggregation of LHC II and quenching of fluorescence in vitro. Biochimica et Biophysica Acta (BBA) – Bioenergetics, 1817, 735–743.10.1016/j.bbabio.2012.01.007
- Uemura, M., Tominaga, Y., Nakagawara, C., Shigematsu, S., Minami, A., & Kawamura, Y. (2006). Responses of the plasma membrane to low temperatures. Physiologia Plantarum, 126, 81–89.10.1111/ppl.2006.126.issue-1
- Velikova, V., Yordanov, I., & Edreva, A. (2000). Oxidative stress and some antioxidant systems in acid rain-treated bean plants: protective role of exogenous polyamines. Plant Science, 151, 59–66.10.1016/S0168-9452(99)00197-1
- Von Deutsch, A. W., Mitchell, C. D., Williams, C. E., Dutt, K., Silvestrov, N. A., Klement, B. J., … Von- Deutsch, D. A. (2005). Polyamines protect against radiation-induced oxidative stress. Gravitation and Space Biology Bulletin, 18, 109–110.
- Zhang, W., Jiang, B., Li, W., Song, H., Yu, Y., & Chen, J. (2009). Polyamines enhance chilling tolerance of cucumber (Cucumis sativus L.) through modulating antioxidative system. Scientia Horticulturae, 122, 200–208.10.1016/j.scienta.2009.05.013