Abstract
Rainfed lowland rice fields are characterized by soil moisture fluctuations (SMF) and the presence of hardpan that impedes deep rooting and thus limits water extraction from deep soil layer during the periods of drought. In this study, we used rootboxes with three layers; shallow layer, artificial hardpan, and deep and wet layer below the hardpan, to evaluate differences in the plasticity of nodal roots elongation through the hardpan and promote root branching below the hardpan in response to SMF among four rice varieties; Sasanishiki, Habataki, Nipponbare, and Kasalath. Experiments were conducted during the summer and autumn seasons. Plasticity was computed as the difference in root traits within each variety between the SMF and continuously well-watered treatments. In both experiments, Habataki consistently tended to exhibit higher root plasticity than the other three varieties by increasing number of nodal roots that penetrated the hardpan during rewatering period in SMF, when the soil moisture increased and penetration resistance decreased. This root plasticity then contributed to greater water use at the deeper soil during the subsequent drought period and overall shoot dry matter production. Habataki had significantly higher δ13C value in roots at deep layer than roots at the shallow and hardpan layers under SMF, which may indicate that these were relatively newly grown roots as a consequence of root plasticity. This study also indicates that CSSLs derived from Sasanishiki and Habataki varieties may be suitable for the analysis of QTLs associated with root plasticity expression in rainfed lowland with hardpan and experiencing SMF.
Introduction
Approximately 19% of the world’s rice production comes from 52 million ha of rainfed lowlands (IRRI, Citation2013). Rainfed rice environments experience multiple abiotic stresses and high uncertainty in the timing, duration, and intensity of rainfall. In rainfed lowland ecosystems, soil moisture fluctuation (SMF) or the alternating recurrence of drought and rewetting depending on the rainfall patterns, are two of the main limiting factors that reduce production (Suralta et al., Citation2016). Typical rainfed lowland fields are characterized by a 5-cm high bulk density hardpan layer underneath the cultivated layer at approximately 20 cm depth (Samson et al., Citation2002; Yano et al., Citation2006). The presence of hardpan is important for reducing the loss of irrigated water in lowland fields but it can also hinder both the penetration of root to deeper soil layer as well as the movement of water between soil layers (Cairns et al., Citation2011). In rainfed lowlands, the soil generally tends to start drying from the surface while there is availability of water in the deep soil horizon, which could be accessed by the roots (Kameoka et al., Citation2015, 2016; Yoshida & Hasegawa, Citation1982). Therefore, the ability of roots to penetrate the hardpan is one of the key traits for deep root system development (Fukai & Cooper, Citation1995), where water supply at the shallow layer is limited during periods of drought (Henry et al., Citation2011; Siopongco et al., Citation2009).
The penetration resistance of soil including that of hardpan is inversely related with the prevailing soil moisture content (SMC) (Cairns et al., Citation2011), because soil strength increased with decrease in soil moisture (Tuzet et al., Citation2003). Several studies have demonstrated that increased soil water extraction from the deeper soil layers is associated with the increase in root length density at this layer (Henry et al., Citation2011; Kameoka et al., Citation2015, 2016) and contributed to the maintenance in dry matter production (Gowda et al., Citation2011; Henry et al., Citation2012; Uga et al., Citation2011). Therefore, the plasticity of roots to penetrate the hardpan is essential for rice plant to adapt to rainfed lowlands with cycles of wet and dry conditions (Bañoc et al., Citation2000a, 2000b; Cairns et al., Citation2011; Samson et al., Citation2002).
Roots play significant roles in plant adaption to various stress soil conditions such as unsaturated (Kato & Katsura, Citation2014) and water-deficit soils (Kato et al., Citation2006) by responding developmentally to such conditions. Under SMF conditions in rainfed lowlands, it is generally believed that root penetration through the hardpan happened in response to drought conditions (Samson et al., Citation2002). With the progress of understanding the functional significance of root plasticity, we examined the timing of root plasticity expression in relation to the soil moisture and penetration resistance interaction by conducting a series of experiments using our newly developed rootbox-hardpan experimental system with three layers: water-fluctuating topsoil, heavily compacted artificial hardpan, and a wet soil below the hardpan (Suralta et al., Citationin press). Using this system, we clearly showed that hardpan penetration resistance interacted with the changes in soil moisture during SMF (Suralta et al., Citationin press) as opposed with the previously reported experimental systems that have hardpan with fixed penetration resistance regardless of prevailing soil moisture conditions (Clark et al., Citation2002; Kamoshita et al., Citation2002; Price et al., Citation2000; Samson et al., Citation2002). We also proved that deep root system development was a result of the plasticity in nodal root elongation through the hardpan, which was triggered by rewatering that makes the hardpan relatively softer during SMF. Such root plasticity enhanced water uptake in the deep soil layer during the drought periods and contributed to the increase in overall dry matter production (Suralta et al., Citationin press). In that same study, we used a recurrent parent IR64 and its introgression line, YTH304 that are highly genetically similar with each other showing similar shoot and root growth performance under non-stress conditions. These two genotypes did differ only under stressed conditions such as rainfed lowland field conditions (Kano-Nakata et al., Citation2013) and experimentally controlled SMF stress with YTH 304 showing greater performance. Thus, the utilization of those genetic materials was effective to explicitly show the functional contribution of the plasticity in nodal root elongation through the hardpan to dry matter production through enhanced water uptake in the deep layer under SMF. Further, we pointed out the need to further confirm such root plasticity and its functions using other genetic mapping populations with parents that have wider genotypic variations and thus can be useful for future breeding program in rainfed lowland rice (Suralta et al., Citationin press).
The plasticity in root branching in the deeper soil layer where the soil moisture is more available during drought could help plants maintain dry matter production (Kameoka et al., Citation2015) through more efficient root exploration in deeper soil and greater water acquisition, which may be closely linked with promoted carbon assimilate allocation to the deep roots during soil drying (Gowda et al., Citation2011; Zhu et al., Citation2010). Drought stress had stronger effects on δ13C levels in sink parts such as roots and panicles than the other plant parts (Grant et al., Citation2012; Monti et al., Citation2006; Peuke et al., Citation2006), indicating that roots are the prominent sink for photo-assimilated carbon (Kano-Nakata et al., Citation2014). Kano-Nakata et al. (Citation2014) also reported that the plants that are well adapted to mild drought stress showed greater variance in carbon isotope ratio (δ13C) value among different plant parts. It is well established that such variation in δ13C among plant parts is related to the isotope fractionation during transport, the synthesis of metabolites, and the chemical composition such as the amounts of lipids, lignin and cellulose contents (Brugnoli & Farquhar, Citation2000) while the mechanism for the isotope fractionations is yet the subject for further study. Several researchers also used δ13C for evaluating belowground carbon allocation (Meier & Leuschner, Citation2008; Scartazza et al., Citation2015; Yao et al., Citation2016). Meier and Leuschner (Citation2008) reported that higher δ13C values were found for roots grown under drought relative to those grown under well-watered conditions in European beech (Fagus sylvatica L.). This was attributed to higher carbohydrate demand of the droughted root system, which led to an increased rate of fine root mass turnover (Meier & Leuschner, Citation2008). These facts also indicate that the newly produced roots tend to have higher δ13C values than older roots under drought.
For plants to well adapt to soil with hardpans and experiencing SMF, it is advantageous for them to develop roots in deeper soil layer well below the hardpan. In our present study therefore, we hypothesized that there should be genotypic variations in δ13C values among roots developed in different soil layers under SMF conditions, and the genotypes that are better adapted to SMF would show higher δ13C value in roots in deep layer because these roots are expected to consist of more nodal roots that newly elongated and penetrated through the hardpan during rewatering, which then branched into lateral roots during the subsequent progressive drought period as compared with those roots from other soil layers. We also assume that δ13C value can be used as an indicator for root plasticity that is expressed under SMF conditions.
In this study, we aimed to compare four rice varieties in terms of the plasticity in nodal root elongation through the hardpan during rewatering periods in SMF, subsequent deep root system development, and their functional contributions to dry matter production.
Materials and methods
Plant materials
We used four varieties; Sasanishiki, Habataki, Nipponbare, and Kasalath. The seeds were provided by the Rice Genome Research Center of the National Institute of Agrobiological Sciences, Japan (http://www.rgrc.dna.affrc.go.jp/jp/). Nipponbare and Kasalath are the parents of a set of Chromosome Segment Substition Lines (CSSLs). Using this set of CSSLs derived from a cross between Nipponbare and Kasalath, several studies have precisely quantified the root plasticity with minimal effects of genetic confounding (Kano-Nakata et al., Citation2011, 2013; Kano et al., Citation2011; Kano-Nakata et al., Citation2017; Tran et al., Citation2014, 2015; Suralta & Yamauchi Citation2008: Suralta et al., Citation2008, Citation2010), as well as the detection of QTLs associated with root plasticity (Niones et al., Citation2013, 2015). On the other hand, Sasanishiki and Habataki are the parents of another set of CSSLs (http://www.rgrc.dna.affrc.go.jp/jp/). Katsura and Nakaide (Citation2011) showed that Habataki had an increase in grain yield in aerobic culture relative to flooded culture while Sasanishiki had no differences between the two water treatments, indicating that Habataki may be more adaptable to water saving aerobic conditions.
Experimental design and treatments
Two experiments were conducted in a glasshouse of Nagoya University, Japan (136o56’6’’E, 35o9’5’’N) during the summer (28th May to 30th July, Experiment 1) and autumn (27th August to 3rd November, Experiment 2) seasons in 2015. The monthly mean maximum and minimum temperature inside the glasshouse during the duration of the experiment was 31.8 and 23.3 °C in Experiment 1, and 24.7 and 16.4 °C in Experiment 2, respectively. These data were collected using a thermometer (T AND D Thermo Recorder TR-72Ui, T&D Corporation, Japan).
The seeds of each variety were soaked in water mixed with benomyl fungicide (Benlate; Sumitomo Chemical Garden Products Inc. Tokyo, .05% w/v) and washed thoroughly prior to incubation in a seed germinator at 30 °C for 72 h prior to sowing. Pre-germinated seeds of each variety were sown in soil-filled PVC rootbox (34.0 cm × 9.5 cm × 64.0 cm, L × W × H) previously described by Suralta et al. (Citationin press). The experiments were arranged in a split-plot design in Randomized Complete Block Design with three replications. The soil moisture treatments were assigned as mainplot while the varieties as subplot. The seedlings were thinned to two plants per rootbox at five days after sowing (DAS).
Soil hardpan preparation and soil moisture measurement
Air-dried sandy loam soil was sieved through a 3-mm mesh and mixed thoroughly with compound fertilizer (14-14-14) at the rate of 60 mg per kg soil. Three soil layers were set; shallow at 0–16 cm depth (above the hardpan layer) with 1.25 g cm−3 bulk density, heavily compacted hardpan at 16–21 cm depth with 1.70 g cm−3 bulk density, and deep layer at 21–63 cm depth (below the hardpan) with 1.25 g cm−3 bulk density. The deep layer below the hardpan was first filled up with 15.55 kg soil to achieve a bulk density of 1.25 g cm−3. Afterward, the hardpan layer (5 cm thick) was filled up with 2.75 kg of dried soil containing 45% sandy loam, 45% sandy soil, premixed with 10% kaolinite. We firstly mixed well these soil components and then gradually put into a rootbox with adding a little water, and continued compacting up to 5 cm thick to achieve a bulk density of 1.70 g cm−3. Finally, the shallow soil layer was filled up with 6.46 kg soil to achieve the same bulk density (1.25 g cm−3) as that of the deep soil layer.
Soil moisture contents (% v/v, SMC) of the shallow and hardpan soil layers were measured with time domain reflectometry probe (TDR; Tektronix Inc., Wilsonville, OR, U.S.A). Two stainless steel rods (15 cm in length) were inserted into the soil at a depth of 0–12 cm leaving 3-cm length protruding above the soil surface, where TDR probes were attached to obtain SMC readings (Fujita et al., Citation2011). Soil penetration resistance (SPR) (MPa) was calculated based on the SMC using an equation generated by Suralta et al. (Citationin press) as below.
Water treatments and water use measurement
Plants were grown under two soil moisture conditions, Well-watered (WW, control) and SMF. In Experiment 1, WW was imposed by maintaining the SMC above 37% v/v through daily watering of the soil surface. On the other hand, in SMF conditions, the soil was first maintained under WW condition from 0 to 25 DAS. Thereafter, it was subjected to the first progressive drought from 26 to 45 DAS by withholding water until the target SMC reached down to 10% v/v at both shallow and hardpan soil layers. Then, plants were rewatered up to the WW conditions from 45 to 52 DAS and maintained to that condition by daily rewatering of the soil surface. Finally, the second progressive drought was imposed from 53 to 60 DAS by withholding water until SMC at shallow soil layer reached down to 10% v/v.
During the second progressive drought, water was supplied only to the deep soil layer through a small plastic tube that was installed at 55 cm below the soil surface on a side of each box. This tube served as the connector between the box and the graduated cylinder. The top end of the graduated cylinder was sealed with a rubber stopper with plastic tube inserted on it. In this setup, the water table in the deep soil layer in the rootbox could be maintained to the level of the bottom end of plastic tube in the graduated cylinder, following the principle of Mariotte bottle technique (Yamauchi et al., Citation1995). The amount of water reduced from the graduated cylinder was equivalent to the amount of water absorbed by the roots in the deep soil layer, which was recorded as water use. Then, the amount of water reduced inside the graduated cylinder was replenished accordingly.
In Experiment 2, the SMC was maintained above 30.9% v/v under WW by daily watering of the soil surface, whereas under SMF conditions, the soil was first maintained at WW condition from 0 to 21 DAS, and then progressively drought stressed from 22 to 43 DAS by withholding water until the SMC at shallow and hardpan soil layers reached down to 10% v/v and then rewatered back to WW condition from 43 to 50 DAS. Finally, the second progressive drought was imposed from 51 to 66 DAS by withholding again the water until the target 10% SMC v/v was reached in the shallow soil layer.
Computation of root plasticity
At 31 cm below the open end of the box (below the hardpan), a transparent plastic tube (7 cm in diameter and 40 cm in length) was horizontally installed for monitoring root growth using a Minirhizotron camera (Regents Instruments Inc., U.S.A). The images were scanned and recorded in a computer. The number of penetrated nodal roots visually observed from each image was counted. Following the methods used by Sandhu et al. (Citation2016) and Suralta et al. (Citationin press), the plasticity in nodal root penetration through the hardpan, which was expressed under SMF conditions was calculated using single replicates from the SMF treatment and mean values from the control (WW) treatment as follows:
where X is the number of nodal roots that penetrated the hardpan.
Photosynthesis and stomatal conductance measurements
Photosynthetic rate (Pn) and stomatal conductance (gs) were measured at 25, 45, 54, 60 DAS in Experiment 1 and at 32, 43, 49, 56, 63, 66 DAS in Experiment 2 using a portable photosynthesis analyzer (Li-6400, Li-COR Inc., U.S.A) on the abaxial side of the topmost fully developed leaf on the main stem between 10 and 12 h under the following settings: leaf temperature, 30 °C; CO2 concentration, 380 μ L L−1; relative humidity, 65–75% and quantum flux density, 1200 μmol m−2 s−1. We measured 3 plants (1 plant = 1 replication) for each treatment.
Shoot and root growth measurements
Shoots were cut at the base and oven-dried at 70 °C for 3 days to record the shoot dry weight at 60 DAS in Experiment 1 and 66 DAS in Experiment 2. Roots were sampled separately from the shallow, hardpan, and deep layers, and carefully washed inside the strainer with running water. The samples were stored in a freezer at -20 °C for length measurement and δ13C analysis as described later. For total root length measurement, each root sample was cut into approximately 1-cm segments and spread on a transparent plastic sheet with minimal overlapping. Digital tiff files were taken using an image scanner (EPSON Expression 10000XL) with a resolution of 400 dpi. Scanned images were analyzed for root length using WinRhizo v. 2009d (Regent Instrument Inc.) (Bouma et al., Citation2000). A pixel threshold value was set at 175 for the root length analysis (Suralta et al., Citationin press). The total lateral root length was estimated as the total length of roots with less than .3 mm in diameter. The total lateral root length was computed as the difference between the total root length and total nodal root length (Yamauchi et al., Citation1996).
The deep root length ratio was calculated as the ratio of the root length in the deep soil layer to the total root length of the whole root system .
Carbon isotope ratio (δ13C) measurement
Harvested plants were separated into shoots and roots. The roots were also separated by soil layer from where (i.e. shallow layer, hardpan layer, and deep layer) they were sampled. Dried shoot or root samples were milled into fine powder. The δ13C values of each sample were analyzed with an isotope mass spectrometer (Sercon Ltd., Cheshire, UK) and expressed in per mil using the following equation:
where R refers to 13C/12C and standard refers to the international Pee Dee Belemnite standard (a fossil Belemnite from the Pee Dee formation in South Carolina) (Farquhar et al., Citation1989). Measurements were performed with three repetitions for each sample.
Statistic analysis
Data were analyzed using the generalized linear model (GLM) procedure (SAS Institute, Citation2003). Analysis of variance was performed separately for each experiment to test the effects of varieties, water treatments and their interaction. The varietal difference in each water treatment was analyzed using the Tukey’s test at p < .05. The effect of water treatment was assessed by analysis of variance in each variety. The relationships among measured traits were analyzed using Pearson’s R correlation.
Results
Environmental conditions, and soil moisture and penetration resistance
The climate data inside glasshouse were collected from 21 DAS until the end of experiment. During these periods, the average temperature in Experiment 1 (27.8 °C) was higher than in Experiment 2 (21.6 °C). The relative humidity was lower in Experiment 1 (43.0%) than in Experiment 2 (68.5%) due to the lower temperature, more rainfall and cloudy days in the latter experiment. The duration of the second progressive drought in Experiment 1 was 7 days (53–60 DAS), which was shorter than the first progressive drought in Experiment 2 (18 days from 26 to 44 DAS) due to higher temperature and expected higher evapotranspiration in Experiment 1. On the other hand, the second progressive drought in Experiment 2 lasted for 15 days (51–66 DAS), which was 8 days longer than the second progressive drought in Experiment 1 partially due to the lower temperature and higher air humidity in Experiment 2. Accordingly, plants under SMF in Experiment 2 were less affected by periods of drought than those in Experiment 1.
The SMC and the estimated soil penetration resistance at shallow and hardpan layers in Experiment 1 are shown in Figure . Under SMF, SMC at the shallow layer ranged from 8.4 to 42.8% and those at the hardpan ranged from 9.6 to 28.1%. The SMC values in the hardpan were lower than those in the shallow layer especially when the soil was wetter (early stage of progressive drought and rewatering period) while there was no difference between layers when the soil was already dry toward the end of progressive drought period at 45 DAS. The SMC at the shallow soil layer under WW ranged from 37.1 to 44.4% which were higher than those at the hardpan layer (27.3 to 31.2%) (Figure (a)).
Figure 1. Soil moisture content (a) and estimated soil penetration resistance (b) at shallow and hardpan soil layers under well-watered (WW) and soil moisture fluctuation (SMF) conditions in Experiment 1.
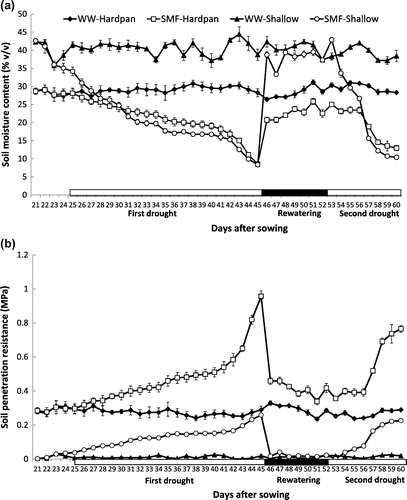
The estimated soil penetration resistance showed an inverse relationship with SMC (Figure (b)). Under SMF, soil penetration resistance of the shallow layer ranged from 0 to .26 MPa while those at the hardpan ranged from .30 to .95 MPa. This showed that the preparation of the heavily compacted hardpan was successful. Under WW, the estimated soil penetration resistance ranged from 0 to .02 MPa at the shallow layer which was lower than those at the hardpan which ranged from .24 to .33 MPa.
In Experiment 2, similar trends were observed although SMC tended to be higher and thus soil penetration resistance was lower than those in Experiment 1 (data not shown). The magnitude of the increase in soil penetration resistance with the decrease in SMC was greater in the hardpan than in the shallow soil layer owing to the greater bulk density of the former than the latter soil layer in both experiments.
Shoot and root growth
Table shows the shoot and root traits of the four varieties grown under WW and SMF treatments in Experiments 1 and 2. In both experiments, no significant genotypic differences were found in shoot dry weight (SDW) under WW conditions while under SMF, Habataki and Kasalath showed significantly higher shoot dry weight than the other two varieties in Experiment 1, and no significant genotypic differences were found in Experiment 2. For root traits, Kasalath developed significantly longer root system than Sasanishiki only under WW and Sasanishiki and Habataki under SMF in Experiment 1. In Experiment 2, only Nipponbare developed a significantly shorter root system than Habataki only under WW while no significant genotypic differences was observed under SMF. For the root length at different soil layers, these four varieties showed no significant differences under WW conditions in both experiments except for Kasalath which produced longer total root length and total nodal root (NR) length than Sasanishiki and Nipponnbare but not Habataki at hardpan layer. Under SMF conditions, one distinct difference was consistently recognized, i.e. Kasalath showed the higher values than Sasanishiki in these roots traits except length of lateral roots (LR) at shallow layer in Experiment 1 but such consistent difference was not observed in Experiment 2. In the other varieties, no consistent trends were observed under SMF.
Table 1. Shoot dry weight (SDW), root length of whole root system, nodal roots (NR) and lateral roots (NR), and the total root length in each soil layer (shallow layer, hardpan, deep layer), and deep root length ratio (DRLR) of four varieties under well-watered (WW) and soil moisture fluctuation (SMF) conditions in Experiments 1 and 2.
We then examined the interactions between water treatment and variety, and found out that the significant interactions were not detected for most of the traits examined in both Experiment 1 and 2 (Supplement 1) because of the limited number of replicates, but the varieties tended to show different responses to the water regimes (Table ).
In this aspect, Habataki showed significantly greater shoot dry weight under SMF than WW in both experiments. Likewise, it produced longer roots at the deep soil layer under SMF relative to WW in Experiment 1, while root development of the four varieties tended be decreased under SMF conditions in Experiment 2, but the reductions at the deep layer tended to be smaller in Habataki as compared with the rest of the varieties. Furthermore, the deep root length ratio of Habataki was increased by SMF relative to WW in both experiments.
Plasticity in nodal root elongation through the hardpan during SMF
In Experiment 1, Habataki expressed its plasticity in nodal root penetration through hardpan specifically during rewatering period (Figure (a)) when the soil penetration resistance in the hardpan layer was low (Figure (b)) while the rest of the varieties showed no clear root plasticity.
Figure 2. The plasticity in number of nodal roots that penetrated hardpan layer of Sasanishiki, Habataki, Nipponbare and Kasalath in Experiments 1 (a) and 2 (b).
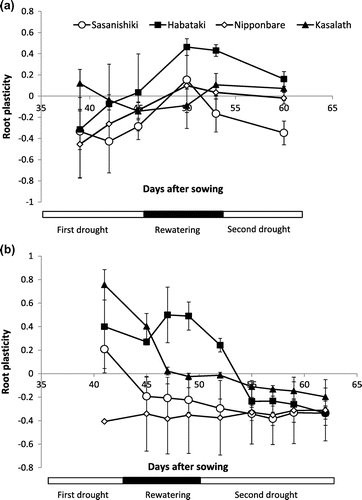
In Experiment 2, Habataki also expressed its plasticity in nodal root penetration through the hardpan during rewatering period in SMF (Figure (b)). Kasalath also showed plasticity but in response to the first drought period but not to rewatering. Both Sasanishiki and Nipponbare did not show any root plasticity under SMF.
Stomatal conductance (gs)
Genotypic variations in stomatal conductance among the four varieties under each water condition are shown in Figure . In both experiments, Habataki tended to show higher gs than the other three varieties under WW conditions. Stomatal conductance under SMF mostly increased during rewatering period in all four varieties with Habataki showing the sharpest increase by 649 and 335% in Experiment 1 and 2, respectively.
Figure 3. Stomatal conductance of Sasanishiki, Habataki, Nipponbare and Kasalath under well-watered (WW) and soil moisture fluctuation (SMF) conditions in Experiments 1 and 2.
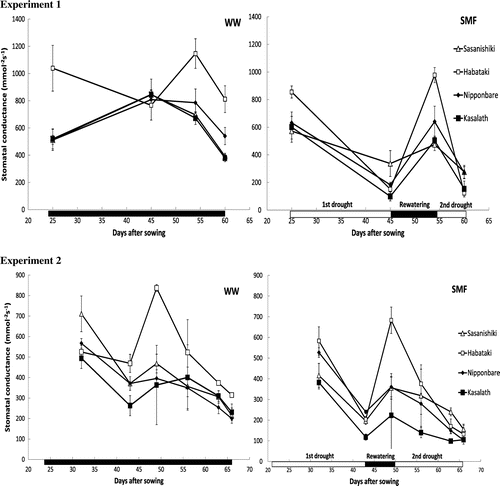
Photosynthetic rate showed no consistent trend among the four varieties under WW while similar response to rewatering was observed in Habataki with that in gs in both experiments (Supplement 2).
Relationship between shoot dry weight, nodal roots that penetrated the hardpan layer, deep root development below the hardpan layer, and water use during the second progressive drought
Table shows the relationships among traits in Habataki variety whose root system tended to be more plastic than those of the other varieties. In Experiment 1, positive and significant correlations were observed between shoot dry weight with water use during the second progressive drought, total root length at deep layer, deep root length ratio, number of nodal roots that penetrated hardpan layer during rewatering period, and number of nodal roots that penetrated hardpan layer at the end of experiment (second progressive drought). In addition, positive and significant correlations were observed between water use with total root length at deep layer, deep root length ratio and number of nodal roots that penetrated hardpan layer during rewatering period, total root length at deep layer with deep root length ratio (DRLR) and number of nodal roots that penetrated hardpan layer during rewatering period (NRHR), and deep root length ratio with number of nodal roots that penetrated hardpan layer during rewatering period and number of nodal roots that penetrated hardpan layer at the end of experiment (second progressive drought).
Table 2. The relationships between shoot dry weight and root traits in Habataki variety under well-watered (WW) and soil moisture fluctuation (SMF) conditions in Experiments 1 and 2.
In Experiment 2, positive and significant correlations were observed between shoot dry weight and water use, and deep-rooting-related traits such as deep root length ratio and number of nodal roots that penetrated hardpan layer during rewatering period. On the other hand, shoot dry weight did not show any significant correlation with total root length at deep layer because although Habataki was able to increase shoot dry weight in response to SMF, its total root length at the deep layer did not change under SMF relative to WW. In addition, water use had positive and significant correlation with deep root length ratio.
δ13C value of roots at different soil layers
Among the four varieties examined, we chose Sasanishiki and Habataki that showed wide genotypic differences in the plasticity in nodal root elongation through the hardpan during rewatering period as well as deep root development in Experiment 1, and measured the δ13C value of the shoots and roots grown under SMF conditions (Figure ). δ13C in roots in the deep soil layer was significantly higher than those in the shallow and hardpan layers in Habataki only. Furthermore, the δ13C in shoots was not significantly different from those in the roots at shallow and hardpan layers in both varieties and had significantly lower δ13C value than the roots at deep layer in Habataki.
Figure 4. δ13C value of roots at different soil layers and shoots of Sasanishiki and Habataki under soil moisture fluctuation condition in Experiment 1.
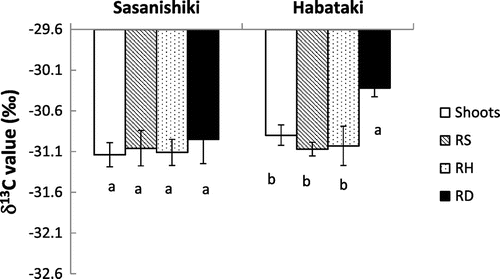
Discussion
Root plasticity expression and soil penetration resistance dynamics
Soil moisture and bulk density were reported to greatly influence soil penetration resistance (Cairns et al., Citation2011). Cairns et al. (Citation2004) showed that there was a large increase in soil penetration resistance as drought progressed. In our experiments, under SMF conditions, when the SMC decreased, the soil penetration resistance increased in all soil layers (Figure ), which were consistent with those reported by Cairns et al. (Citation2011), Sanusan et al. (Citation2010), Gao et al. (Citation2016) and Suralta et al. (Citationin press). In contrast, we found that soil penetration resistance at the hardpan layer was reduced during the rewatering period thus, nodal roots could have a chance to penetrate the hardpan layer in preparation for the subsequent drought period if the genotype confers root plasticity. Then, the promoted nodal root elongation due to plasticity triggered by SMC change from dry to wet under SMF could result in subsequent penetration through the hardpan only if the penetration resistance is reduced especially when the soil becomes wet.
Suralta et al. (Citationin press) determined that matching of root plasticity expression and reduced soil penetration resistance due to rewetting of hardpan promoted water uptake and dry matter production using a recurrent parent IR64 and its introgression line, YTH304. Furthermore, they pointed out the need to examine genotypic variations in the functional significance of root plasticity especially such ability in growth response of the nodal roots due to plasticity could be the key trait for the adaptability in rainfed lowland fields which are characterized by fluctuating hardpan penetration resistance. In this study, we compared four varieties, and found that Habataki tended to express sharper root plasticity in nodal root penetration through the hardpan than the other varieties, which consistently contributed to the overall growth under SMF in both experiments.
Significance of root plasticity in deep root development, water uptake and dry matter production under SMF
SMF is one of the constraints in rice production, which could cause reduction in dry matter production (Niones et al., Citation2015; Siopongco et al., Citation2008). Katsura (Citation2013) demonstrated that the average grain yield of the Sasanishiki/Habataki CSSLs under aerobic conditions was consistently higher than that of Sasanishiki in a two-year experiment. This implies that the Sasanishiki/Habataki CSSLs may have QTLs related to high grain yield that are inherited from Habataki. Our present study also showed that Habataki had significantly greater shoot dry weight under SMF than under WW in both experiments whereas for Sasanishiki, there was no significant difference in shoot dry weight between the two water treatments (Table ). Thus, considering the fact that aerobic conditions inherently contains wetting and drying cycles of soil, Habataki may have a potential to produce higher shoot dry matter under SMF as compared with WW.
Root plasticity analysis can be done by comparing the roots of plants of the same genotype that are grown under different environments such as those between different water-stressed and non-stressed (control) conditions (Kameoka et al., Citation2015; Kano-Nakata et al., Citation2011; Menge et al., Citation2016). Under SMF conditions in this study, we found that Habataki showed consistently higher number of nodal roots that penetrated the hardpan during the rewatering period (Figure ) as well as significant increase of total root length (Experiment 1) and maintenance of total root length (Experiment 2) at the deep layer (Table ). This means that Habataki had the ability to penetrate the hardpan layer through root plasticity via elongation, thereby exhibiting deep root development below the hardpan layer.
In Experiment 1, under SMF, Habataki showed root plasticity at deep layer where water is available as a result of significantly higher total lateral root length as well as total nodal root length than those under WW (Table ). Kameoka et al. (Citation2015) suggested that the root plasticity expressed in soil portions where the soil moisture is more available increases potential adaptation of rice plants to water deficit conditions. In addition, enhanced lateral root development is known to increase root surface area and soil water extraction (Henry et al., Citation2011; Kameoka et al., Citation2015; Kato et al., Citation2007, 2011; Menge et al., Citation2016) and water use (Kobata et al., Citation1996; Suralta et al., Citation2010). Likewise, nodal root development is also important to enhance the contribution of lateral root branching to the entire root system development (Kameoka et al., Citation2016).
Deep root development in rice can contribute to shoot dry matter production through enhanced water uptake (Gowda et al., Citation2011; Uga et al., Citation2013). In our studies, the deep root length ratio of Habataki, which was significantly higher in both experiments, and the higher total root length at deep layer in Experiment 1 could enhance water use below the hardpan layer especially during the second progressive drought in SMF (Table ). Such enhanced water uptake clearly resulted in sharp increases in gs during the rewatering periods in SMF (Figure ) and consequently contributed to higher photosynthetic rates (Supplement 2) and shoot dry matter production in Habataki under SMF conditions (Table ). Kato and Okami (Citation2011) indicated that more assimilates in rice plants were allocated to the deep roots under aerobic culture than flooded conditions. Under water limited conditions, changes in assimilate partitioning tend to favor the development of deep root in rice (Gowda et al., Citation2011), suggesting that genetic improvement in deeper rooting would be one of the effective goals.
δ13C value and deep root development
We quantified δ13C value of Sasanishiki and Habataki varieties, which showed wider genotypic differences in the root plasticity in nodal root penetration through the hardpan (Figure ). The significantly higher δ13C value of the roots observed in the deep soil layer than those at shallow and hardpan layers indicate that these may be relatively newly roots produced under drought conditions as discussed earlier. Specifically, we may speculate that these roots mostly would be the ones that expressed plasticity in response to rewatering, elongated through the hardpan and subsequently branched at the deep soil layer during the second drought period. This subsequently contributed to the increase in water uptake below the hardpan layer and thus increased dry matter production. The genotypes that show higher δ13C value in roots at deep layer may be more adapted to SMF than the ones with lower value because Habataki showed such responses in this study. In this aspect, the future study is needed to examine whether the δ13C can be an indicator for root plasticity that is expressed under SMF stress conditions.
Conclusion
Among four varieties (Sasanishiki, Habataki, Nipponbare, and Kasalath) examined in two experiments, Habataki tended to exhibit higher plasticity in nodal root elongation, which resulted in hardpan penetration, and consequently deep root development than the other varieties under SMF conditions. We showed that such plasticity in nodal root elongation through the hardpan occurred during the rewatering period in SMF, when the soil moisture increased and penetration resistance decreased. This root plasticity then contributed to greater shoot dry matter production through enhanced water uptake in deep soil layer below the hardpan where moisture was relatively higher than those in the shallow and hardpan layers. The roots that penetrated through the hardpan as a consequence of the plasticity in nodal root elongation were assumed to be comprised with relatively new roots as prominent sink for newly photo-assimilated carbon acquired during the subsequent progressive drought period because these roots showed higher δ13C values than the roots in and above the hardpan layers as well as those in shoots. We need further study if the δ13C value could be used as an indicator for root plasticity under SMF. Further studies using the CSSLs (Sasanishiki/Habataki) are ongoing to identify QTLs associated with root plasticity traits in hardpan penetration during SMF.
Funding
This research was funded by the Grant-in-Aid for Scientific Research [grant number 15H02644] from the Japan Society for the Promotion of Science; partially supported by the Japan Science and Technology Agency (JST)/Japan International Cooperation Agency (JICA) and Science and Technology Research Partnership for Sustainable Development (SATREPS).
Supplemental data
The supplemental data for this article can be accessed at https://doi.org/10.1080/1343943X.2018.1439757
Disclosure statement
No potential conflict of interest was reported by the authors.
TPPS_1439757_Supplementary_material.docx
Download MS Word (115.2 KB)Acknowledgments
We are thankful to Dr. Mayumi Kikuta and Mr. Takuya Kabuki for their valuable assistance in data analyses.
References
- Bañoc, D. M., Yamauchi, A., Kamoshita, A., Wade, L. J., & Pardales, J. R., Jr (2000a). Dry matter production and root system development of rice cultivars under fluctuating soil moisture. Plant Production Science, 3, 197–207.
- Bañoc, D. M., Yamauchi, A., Kamoshita, A., Wade, L. J., & Pardales, J. R., Jr (2000b). Genetypic variations in response of lateral root development to fluctuating soil moisture in rice. Plant Production Science, 3, 335–343.
- Bouma, T. J., Nielsen, K. L., & Koutstaal, B. (2000). Sample preparation and scanning protocol for computerised analysis of root length and diameter. Plant and Soil, 218, 185–196.10.1023/A:1014905104017
- Brugnoli, E., & Farquhar, G. D. (2000). Photosynthetic fractionation of carbon isotopes. In R. C. Leegood, T. D. Sharkey, & S. Von Caemmerer (Eds.), Photosynthesis: Physiology and Metabolism (pp. 352–434). Dordrecht: Kluwer Academic.
- Cairns, J. E., Audebert, A., Townend, J., Price, A. H., & Mullins, C. E. (2004). Effect of soil mechanical impedance on root growth of two rice varieties under field drought stress. Plant and Soil, 267, 309–318.10.1007/s11104-005-0134-1
- Cairns, J. E., Impa, S. M., O’Toole, J. C., Jagadish, S. V. K., & Price, A. H. (2011). Influence of the soil physical environment on rice (Oryza sativa L.) response to drought stress and its implications for drought research. Field Crops Research, 121, 303–310.10.1016/j.fcr.2011.01.012
- Clark, L. J., Cope, R. E., Whalley, W. R., Barraclough, P. B., & Wade, L. J. (2002). Root penetration of strong soil in rainfed lowland rice: Comparison of laboratory screens with field performance. Field Crops Research, 76, 189–198.10.1016/S0378-4290(02)00039-4
- Farquhar, G. D., Ehleringer, J. R., & Hubick, K. T. (1989). Carbon isotope discrimination and photosynthesis. Annual Review of Plant Physiology and Plant Molecular Biology, 40, 503–537.10.1146/annurev.pp.40.060189.002443
- Fujita, A., Nakamura, M., & Kameoka, T. (2011). Soil moisture measurement to support production of high-quality oranges for Information and Communication Technology (ICT) application in production orchards. Agricultural Information Research, 20, 86–94.10.3173/air.20.86
- Fukai, S., & Cooper, M. (1995). Development of drought-resistant cultivars using physio-morphological traits in rice. Field Crops Research, 40, 67–86.10.1016/0378-4290(94)00096-U
- Gao, W., Hodgkinson, L., Jin, K., Watts, C. W., Ashton, R. W., Shen, J., … Whalley, W. R. (2016). Deep roots and soil structure. Plant, Cell and Environment, 39, 1662–1668.10.1111/pce.12684
- Gowda, V. R. P., Henry, A., Yamauchi, A., Shashidhar, H. E., & Serraj, R. (2011). Root biology and genetic improvement for drought avoidance in rice. Field Crops Research, 122, 1–13.10.1016/j.fcr.2011.03.001
- Grant, O. M., Davies, M. J., James, C. M., Johnson, A. W., Leinonen, I., & Simpson, D. W. (2012). Thermal imaging and carbon isotope composition indicate variation amongst strawberry (Fragaria × ananassa) cultivars in stomatal conductance and water use efficiency. Environmental and Experimental Botany, 76, 7–15.10.1016/j.envexpbot.2011.09.013
- Henry, A., Gowda, V. R. P., Torres, R. O., McNally, K. L., & Serraj, R. (2011). Variation in root system architecture and drought response in rice (Oryza sativa): Phenotyping of the OryzaSNP panel in rainfed lowland fields. Field Crops Research, 120, 205–214.10.1016/j.fcr.2010.10.003
- Henry, A., Cal, A. J., Batoto, T. C., Torres, R. O., & Serraj, R. (2012). Root attributes affecting water uptake of rice (Oryza sativa) under drought. Journal of Experimental Botany, 63, 4751–4763.10.1093/jxb/ers150
- IRRI. (2013). Rice Almanac (4th ed.). Los Baños. Philippines: International Rice Research Institute.
- Kameoka, E., Suralta, R. R., Mitsuya, S., & Yamauchi, A. (2015). Matching the expression of root plasticity with soil moisture availability maximizes production of rice plants grown in an experimental sloping bed having soil moisture gradients. Plant Production Science, 18, 267–276.10.1626/pps.18.267
- Kameoka, E., Suralta, R. R., Mitsuya, S., & Yamauchi, A. (2016). Developmental plasticity of rice root system grown under mild drought stress condition with shallow soil depth: Comparison between Nodal and lateral roots. Plant Production Science, 19, 411–419.10.1080/1343943X.2015.1128094
- Kamoshita, A., Wade, L. J., Ali, M. L., Pathan, M. S., Zhang, J., Sarkarung, S., & Nguyen, H. T. (2002). Mapping QTLs for root morphology of a rice population adapted to rainfed lowland conditions. Theoretical and Applied Genetics, 104, 880–893.
- Kano, M., Inukai, Y., Kitano, H., & Yamauchi, A. (2011). Root plasticity as the key root trait for adaptation to various intensities of drought stress in rice. Plant and Soil, 342, 117–128.10.1007/s11104-010-0675-9
- Kano-Nakata, M., Inukai, Y., Wade, L. J., Siopongco, J. D., & Yamauchi, A. (2011). Root development, water uptake, and shoot dry matter production under water deficit conditions in two CSSLs of rice: Functional roles of root plasticity. Plant Production Science, 14, 307–317.10.1626/pps.14.307
- Kano-Nakata, M., Gowda, V. R. P., Henry, A., Serraj, R., Inukai, Y., Fujita, D., … Yamauchi, A. (2013). Functional roles of the plasticity of root system development in biomass production and water uptake under rainfed lowland conditions. Field Crops Research, 144, 288–296.10.1016/j.fcr.2013.01.024
- Kano-Nakata, M., Tatsumi, J., Inukai, Y., Asanuma, S., & Yamauchi, A. (2014). Effect of various intensities of drought stress on δ13C variation among plant organs in rice: Comparison of two cultivars. American Journal of Plant Sciences, 5, 1686–1693.10.4236/ajps.2014.511183
- Kano-Nakata, M., Inukai, Y., Siopongco, J. D. L. C., Mitsuya, S., & Yamauchi, A. (2017). Quantitative evaluation of plastic root responses to continuous water gradient in rice. Plant Root, 11, 70–78.10.3117/plantroot.11.70
- Kato, Y., Abe, J., Kamoshita, A., & Yamagishi, J. (2006). Genotypic variation in root growth angle in rice (Oryza sativa L.) and its association with deep root development in upland fields with different water regimes. Plant and Soil, 287, 117–129.10.1007/s11104-006-9008-4
- Kato, Y., Kamoshita, A., & Yamagishi, J. (2007). Evaluating the resistance of six rice cultivars to drought: Restriction of deep rooting and the use of raised beds. Plant and Soil, 300, 149–161.10.1007/s11104-007-9397-z
- Kato, Y., Henry, A., Fujita, D., Katsura, K., Kobayashi, N., & Serraj, R. (2011). Physiological characterization of introgression lines derived from an indica rice cultivar, IR64, adapted to drought and water-saving irrigation. Field Crops Research, 123, 130–138.10.1016/j.fcr.2011.05.009
- Kato, Y., & Okami, M. (2011). Root morphology, hydraulic conductivity and plant water relations of high-yielding rice grown under aerobic conditions. Annals of Botany, 108, 575–583.10.1093/aob/mcr184
- Kato, Y., & Katsura, K. (2014). Rice adaptation to aerobic soils: Physiological consideration and implications for agronomy. Plant Production Science, 17, 1–12.10.1626/pps.17.1
- Katsura, K. (2013). Agronomic traits for high productivity of rice grown in aerobic culture in progeny of a Japonica cultivar and a high-yielding Indica cultivar. Agronomy and Crop Ecology, 16, 317–324.
- Katsura, K., & Nakaide, Y. (2011). Factors that determine grain weight in rice under high-yielding aerobic culture: The importance of husk size. Field Crops Research, 123, 266–272.10.1016/j.fcr.2011.05.023
- Kobata, T., Okuno, T., & Yamamoto, T. (1996). Contributions of capacity for soil water extraction and water use efficiency to maintenance of dry matter production in rice subjected to drought. Japanese journal of crop science, 64, 652–662.10.1626/jcs.65.652
- Meier, I. C., & Leuschner, C. (2008). Belowground drought response of European beech: Fine root biomass and carbon partitioning in 14 mature stands across a precipitation gradient. Global Change Biology, 14, 2081–2095.10.1111/gcb.2008.14.issue-9
- Menge, M. D., Kameoka, E., Kano-Nakata, M., Yamauchi, A., Asanuma, S., Asai, H., … Makihara, D. (2016). Drought-induced root plasticity of two upland NERICA varieties under conditions with contrasting soil depth characteristics. Plant Production Science, 19, 389–400.10.1080/1343943X.2016.1146908
- Monti, A., Amaducci, M. T., Pritoni, G., & Venturi, G. (2006). Variation in carbon isotope discrimination during growth and at different organs in sugar beet (Beta vulgaris L.). Field Crops Research, 98, 157–163.10.1016/j.fcr.2006.01.002
- Niones, J. M., Suralta, R. R., Inukai, Y., & Yamauchi, A. (2013). Roles of root aerenchyma development and its associated QTL in dry matter production under transient moisture stress in rice. Plant Production Science, 16, 205–216.10.1626/pps.16.205
- Niones, J. M., Inukai, Y., Suralta, R. R., & Yamauchi, A. (2015). QTL associated with lateral root plasticity in response to soil moisture fluctuation stress in rice. Plant and Soil, 391, 63–75.10.1007/s11104-015-2404-x
- Peuke, A. D., Gessler, A., & Rennenberg, H. (2006). The effect of drought on C and N stable isotopes in different fractions of leaves, stems and roots of sensitive and tolerant beech ecotypes. Plant, Cell and Environment, 29, 823–835.10.1111/pce.2006.29.issue-5
- Price, A. H., Steele, K. A., Moore, B. J., Barraclough, P. B., & Clark, L. J. (2000). A combined RFLP and AFLP linkage map of upland rice (Oryza sativa L.) used to identify QTLs for root penetration ability. Theoretical and Applied Genetics, 100, 49–56.10.1007/s001220050007
- Samson, B. K., Hasan, M., & Wade, L. J. (2002). Penetration of hardpans by rice lines in the rainfed lowlands. Field Crops Research, 76, 175–188.10.1016/S0378-4290(02)00038-2
- Sandhu, N., Anitha Raman, K., Torres, R. O., Audebert, A., Dardou, A., Kumar, A., & Henry, A. (2016). Rice root architectural plasticity traits and genetic regions for adaptability to variable cultivation and stress conditions. Plant Physiology, 17, 2562–2576.
- Sanusan, S., Polthanee, A., Audebert, A., Seripong, S., & Mouret, J. C. (2010). Suppressing weeds in direct-seeded lowland rainfed rice: Effect of cutting dates and timing of fertilizer application. Crop Protection, 29, 927–935.10.1016/j.cropro.2010.04.004
- SAS Institute. (2003). SAS user’s guide: Statistics. (Version 9.1, 2002–2003). Cary, NC: SAS Inst.
- Scartazza, A., Moscatello, S., Matteucci, G., Battistelli, A., & Brugnoli, E. (2015). Combining stable isotope and carbohydrate analyses in phloem sap and fine roots to study seasonal changes of source-sink relationships in a Mediterranean beech forest. Tree Physiology, 35, 829–839.10.1093/treephys/tpv048
- Siopongco, J. D. L. C., Sekiya, K., Yamauchi, A., Egdane, J., Ismail, A. M., & Wade, L. J. (2008). Stomatal responses in rainfed lowland rice to partial soil drying. Plant Production Science, 11, 28–41.10.1626/pps.11.28
- Siopongco, J. D. L. C., Sekiya, K., Yamauchi, A., Egdane, J., Ismail, A. M., & Wade, L. J. (2009). Stomatal responses in rainfed lowland rice to partial soil drying; Comparison of two lines. Plant Production Science, 12, 17–28.10.1626/pps.12.17
- Suralta, R. R., Inukai, Y., & Yamauchi, A. (2008). Utilizing chromosome segment substitution lines (CSSLs) for evaluation of root responses to transient moisture stresses in rice. Plant Production Science, 11, 457–465.10.1626/pps.11.457
- Suralta, R. R., & Yamauchi, A. (2008). Root growth, aerenchyma development, and oxygen transport in rice genotypes subjected to drought and waterlogging. Environmental and Experimental Botany, 64, 75–82.10.1016/j.envexpbot.2008.01.004
- Suralta, R. R., Inukai, Y., & Yamauchi, A. (2010). Dry matter production in relation to root plastic development, oxygen transport, and water uptake of rice under transient soil moisture stresses. Plant and Soil, 332, 87–104.
- Suralta, R. R., Kano-Nakata, M., Niones, J. M., Inukai, Y., Kameoka, E., Tran, T. T., … Yamauchi, A. (2016). Root plasticity for maintenance of productivity under abiotic stressed soil environments in rice: Progress and prospects. Field Crops Research,. doi:10.1016/j.fcr.2016.06.023
- Suralta, R. R., Niones, J. M., Kano-Nakata, M., Tran, T. T., Mitsuya, S., & Yamauchi, A. (in press). Plasticity in nodal root elongation through the hardpan was triggered by rewatering during soil moisture fluctuation stress in rice. Scientific Reports.
- Tran, T. T., Kano-Nakata, M., Suralta, R. R., Menge, D., Mitsuya, S., Inukai, Y., & Yamauchi, A. (2014). Root plasticity and its functional roles were triggered by water deficit but not by the resulting changes in the forms of soil N in rice. Plant and Soil, 386, 65–76.
- Tran, T. T., Kano-Nakata, M., Takeda, M., Menge, D., Mitsuya, S., Inukai, Y., & Yamauchi, A. (2015). Nitrogen application enhanced the expression of developmental plasticity of root systems triggered by mild drought stress in rice. Plant and Soil, 378, 139–152.
- Tuzet, A., Perrier, A., & Leuning, R. (2003). A coupled model of stomatal conductance, photosynthesis and transpiration. Plant, Cell and Environment, 26, 1097–1116.10.1046/j.1365-3040.2003.01035.x
- Uga, Y., Okuno, K., & Yano, M. (2011). Dro1, a major QTL involved in deep rooting of rice under upland field conditions. Journal of Experimental Botany, 62, 2485–2494.10.1093/jxb/erq429
- Uga, Y., Sugimoto, K., Ogawa, S., Rane, J., Ishitani, M., Hara, N., & Yano, M. (2013). Control of root system architecture by DEEPER ROOTING 1 increases rice yield under drought conditions. Nature Genetics, 45, 1097–1102.10.1038/ng.2725
- Yamauchi, A., Taylor, H. M., Upchurch, D. R., & McMichael, B. L. (1995). Axial resistance to water flow of intact cotton taproots. Agronomy Journal, 87, 439–445.10.2134/agronj1995.00021962008700030009x
- Yamauchi, A., Pardales, J. R., Jr, & Kono, Y. (1996). Root system structure and its relation to stress tolerance. In O. Ito, K. Katayama, C. Johansen, J. V. D. K. Kumar Rao, J. J. Adu-Gyamfi, & T. J. Rego (Eds.), Roots and nitrogen in cropping systems of the semi-arid tropics (pp. 211–234). Tsukuba: Japan International Research Center for Agriculture Sciences.
- Yano, K., Sekiya, N., Samson, B. K., Mazid, M. A., Yamauchi, A., Kono, Y., & Wade, L. J. (2006). Hydrogen isotope composition of soil water above and below the hardpan in a rainfed lowland rice field. Field Crops Research, 96, 477–480.10.1016/j.fcr.2005.09.011
- Yao, H., Wilkes, A., Zhu, G., Zhang, H., Liu, X., Ding, D., … Wang, C. (2016). Stable carbon isotope as a signal index for monitoring grassland degradation. Scientific Reports, 6, 1–5.
- Yoshida, S., & Hasegawa, S. (1982). The rice root system: Its development and function. In Drought resistance in crops with emphasis on rice (pp. 97–114). Los Baños, Philippines: International Rice Research Institute.
- Zhu, J., Brown, K. M., & Lynch, J. P. (2010). Root cortical aerenchyma improves the drought tolerance of maize (Zea mays L.). Plant, Cell and Environment, 33, 740–749.