ABSTRACT
This study evaluated the effect of three N fertilization levels 60 (low), 120 (medium), and 180 (high) kg N ha−1 and soil moisture content gradients created by a line-source sprinkler on the expression of plasticity in lateral root branching and dry matter production (DMP) of upland new rice for Africa (NERICA) 1 and 4. There were no significant differences in DMP between NERICA 1 and 4 under well-watered, mild drought, and severe drought conditions regardless of N level. In contrast, under moderate drought (12–21% v/v of soil moisture content [SMC] in 2011 and 16–24% v/v of SMC in 2012), NERICA 1 had significantly higher shoot dry weight, total root length (TRL), lateral root length, and branching index than NERICA 4 at medium and high N; however, there was no significant difference between the two NERICAs in DMP at low N. TRL of NERICA 1 was significantly higher under moderate drought than well-watered conditions, but only with medium and high N. Regardless of N level, moderate drought did not enhance NERICA 4’s root system. Thus, NERICA 1’s root system exhibited plastic development, promoting lateral root branching at medium and high N. These morphological changes were associated with the greater DMP in NERICA 1 than NERICA 4 under moderate drought, whereas the lack of such plasticity at low N meant genotypic differences in DMP were obscured. Our findings implied that N application can improve upland NERICA productivity under moderate drought conditions, but differences in variety and field conditions may influence efficacy.
1. Introduction
In the 2015/2016 cropping season, Africa produced approximately 19 million tons of milled rice and consumed 30 million tons (USDA, Citation2017). To meet consumption, the continent spent approximately 8 billion USD importing rice during that season, accounting for 30% of global rice imports. African demand for rice is the fastest growing demand worldwide (IRRI, Citation2017), driven by rapid urbanization and a shift in consumer preference from the traditional staples to rice (Balasubramanian et al., Citation2007). Therefore, urgent measures are necessary to increase rice production in Africa, thereby reducing production deficits and import expenses.
Rice is generally considered as a semi-aquatic plant (Gupta & O’Toole, Citation1986). However, with the exception of Egypt and Mauritania, very little rice cultivation occurs on irrigated fields in African countries (Seck et al., Citation2012). Instead, rice is primarily cultivated in rainfed upland fields, which constitute about 45% of the rice-growing area in sub-Saharan countries (Maclean et al., Citation2002). Despite this, over half of the 420 million ha that is suitable for upland rice production remains fallow or underused in sub-Saharan Africa (Fischer et al., Citation2002). Thus, the potential for increased rice production is enormous.
New rice for Africa (NERICA) varieties are interspecific progenies of Oryza sativa and Oryza glaberrima. Currently, 18 varieties (NERICA 1 to NERICA 18) are suited for upland growth conditions (Matsunami & Kokubun, Citation2011). Dissemination of NERICA in sub-Saharan countries started soon after their initial release in 2007 (Kaneda, Citation2007); by 2009, NERICA varieties were being cultivated on approximately 700,000 ha of land in sub-Saharan Africa (Diagne et al., Citation2010). Field studies in drought-prone environments revealed that NERICA had higher yields than conventional varieties (Kijima et al., Citation2006). Thus, NERICA cultivation is expected to improve upland productivity and greatly enhance rice yield in the sub-Saharan region.
Drought is one of the most important abiotic stressors limiting rice productivity in the uplands. This ecosystem typically subjects rice to mild drought that can develop into more severe stress under conditions of high evapotranspiration (Kamoshita et al., Citation2008). Irrigation can mitigate these negative effects, but is not frequently practiced by most rain-dependent small-scale farmers, who make up approximately 80% of rice producers (Ndjiondjop et al., Citation2012). Therefore, improving drought avoidance traits in upland NERICA is a major step towards achieving self-sufficiency in Africa.
Root architecture is fundamental to plant productivity, especially in the many arid environments (Lynch, Citation1995). Traits that contribute to sustaining plant performance in droughts include thick and deep roots, as well as enhanced lateral root formation (Kano et al., Citation2011). Additionally, a plastic root system that develops differentially to environmental changes is important for stabilizing plant growth under drought conditions (Yamauchi et al., Citation2008). Previously, we showed that upland NERICA 1 had plastic lateral root development, while NERICA 4 expressed deep root plasticity under moderate drought (Menge et al., Citation2016). The plasticity in lateral root development is advantageous under upland-rice cultivation environments, where the ground is resistant to vertical root growth into the deep soil profile (Cains et al., Citation2011).
Soil nutrient status is critical to root developmental processes (Lopez-Bucio et al., Citation2003). Drought and soil nutrient availability can interact to adversely affect rice shoot/root growth (Kono et al., Citation1987; Yamauchi et al., Citation1996), photosynthesis, and transpiration (O’too et al., Citation1989). N application may result in rapid development of drought stress (Prasertsak & Fukai, Citation1997). However, rice grain yield consistently increased with N application even under water deficit conditions in lowland rice (Castillo et al., Citation1992). Specifically, N application increases root dry weight, total root length, root volume, and root surface area (Fan et al., Citation2010; Wang & Yamauchi, Citation2006). Furthermore, N application enhanced plasticity in lowland rice roots during mild drought conditions, through promoted lateral root development (Tran et al., Citation2014).
Root growth responses of upland rice may differ from lowland rice due to differences in phenological development, morphological traits such as tillering, and by the fact that soil moisture conditions are different in the two ecosystems (Kato et al., Citation2006). For instance, Bañoc et al., (Citation2000) reported that during drought the ratio (%) in root dry weight of nodal roots to the entire root system tended to be higher in lowland than upland varieties. In that study, upland varieties tended to be more efficient in lateral root formation. Therefore, the question is whether N application would affect root plasticity of upland NERICA differently or in a similar manner to lowland rice, hence influencing their adaptability and productivity under drought.
Thus, the objective of this study was to examine how N fertilization affects root growth and dry matter production (DMP) of upland NERICA 1 and 4 in comparison with Lemont, a lowland check, when drought occurred under conditions that inhibit deep root growth. We predicted that under optimal N fertilization upland rice would adapt to drier soil moisture conditions than lowland rice due to efficiency in lateral root development without necessarily increasing dry matter allocation to roots. Within upland rice, NERICA 1 was expected to show superior aboveground growth response than NERICA 4 under optimal N application that can be attributed to its greater plasticity in lateral root development. However, plasticity would be suppressed and aboveground growth would not differ between NERICA 1 and 4 when N fertilization was low.
2. Materials and methods
2.1. Plant materials
This study evaluated NERICA 1 and NERICA 4. The lowland variety Lemont was used as a check because, similar to upland NERICA 1 and 4, it has early maturing characteristics that is, attained 50% heading at around 90 days after sowing (Xu et al., Citation2005). Therefore, we intended to match the drought stress in similar phenological stages among the three varieties.
2.2. Field experiments
Field experiments were performed in a well-ventilated vinyl (Nobi Ace Mirai; Mitsubishi Chemical Agri Dream Co., Ltd, Tokyo, Japan) house on Togo Field, Field Science Center, Graduate School of Bioagricultural Sciences, Nagoya University, Togo-cho, Aichi, Japan (35°6′42″ N, 137°4′57″ E) in 2011 and 2012. During the experimental period, monthly mean maximum temperatures inside the shelter ranged 27–33°C in 2011 and 26–34°C in 2012; monthly mean minimum temperatures ranged 21–25°C in 2011 and 22–26°C in 2012. The soil used was sandy loam (72.3% sand, 20.9% silt, 6.8% clay) with a field capacity of 27.7% w/w (gravimetric), wilting point of 10.6% w/w, pH (H2O) of 5.5, electrical conductivity of 3.3 mS m−1, cation exchange capacity of 9.5 cmol kg−1, available K of 2.1 g kg−1, and N content of 1.3 g kg−1. The dimensions of the experimental field were 23.0 × 3.6 m (L × W) with 0.5 m foot-path all around. The field was made watertight with a PVC sheet buried at an average depth of 25 cm. Soil moisture gradients were created using a line-source sprinkler system, following the layout and procedures of previous publications (Kano et al., Citation2011; Menge et al., Citation2016). The line-source sprinkler was set 15 cm aboveground, along the mid-point of the field. The timing and intensity of irrigation was automatically regulated to create a wet-to-dry soil moisture gradient as a result of increasing distance from the sprinkler.
Seeds from the three varieties were sterilized using a fungicide (Benlate; Sumitomo Chemical Garden Products Inc. Tokyo, 0.15% w/v) for 24 h, then soaked in running water and incubated in a seed germinator at 30°C for 48 h. The pre-germinated seeds were sown in soil-filled plastic trays under well-watered conditions. Twenty-one-day-old seedlings of each variety, with an intact root system, were transplanted into unpuddled plots in rows perpendicular to the line-source sprinkler, at a rate of one seedling per hill on 4 July 2011 and 6 June 2012. Totally, 14 days after transplanting (DAT), drought stress was simulated using a sprinkler that automatically released a water mist up to 80 cm away, three times daily (at 06:00, 11:00, and 18:00) for 1 min. No standing water was observed after each irrigation episode; hence the plants were exposed to soil moisture conditions similar to those experienced in upland rice fields.
N fertilizer levels for both years were designed as 180 kg ha−1 (high), 120 kg ha−1 (medium), and 60 kg ha−1 (low), after considering the suggested N application rates in African soils in the previous studies (i.e. 80–100 kg N ha−1) (Becker et al., Citation2003; Dingkuhn et al., Citation1997; Jones et al., Citation1997; Sahrawat & Sika, Citation2001). The medium and high N fertilization levels will be referred to as optimal N in subsequent sections. P and K fertilizers were also applied to all plots at a rate of 50 kg ha−1. Fertilizers were applied as basal dressing 7 d after transplanting. Fertilizer sources were urea (46% N) for N, solophos (18% P2O5) for P, and muriate of potash (60% K2O) for K.
Shoot and root samples were collected at 67 DAT in 2011 and 123 DAT in 2012. The total soil drying duration was 53 d in 2011 and 109 d in 2012.
2.3. Experimental design
A split-split-plot design was used in which the soil moisture gradient was the main plot, the three N levels were the subplot, and the three varieties were the sub-subplot factor replicated three times. Each replication contained three 2.2-m2 plots that received a specific N amount, yielding nine plots in total. Every plot contained three rows, each with eight plants per variety that were exposed to the soil moisture gradient. N levels and varieties were randomized. To prevent lateral N contamination, two buffer rows separated plots that received different N treatments. Plants were spaced 20 cm between hills of the same variety and 40 cm between rows.
2.4. Measurements
The shoot dry weight (SDW) of each plant was determined after oven-drying for 3 d at 72°C. Roots were sampled using a stainless cylinder of diameter 15 cm and the soil cores of 20 cm in depth taken. The root sampling begun on the same day as shoot sampling and requiring 3 d to complete. Nodal root lengths were measured with a ruler. The whole root system per plant was cut into 1-cm segments and spread (with minimal overlap) in a thin film of water on transparent plastic sheets. High-resolution digital images were then taken using an EPSON scanner (ES2200, Seiko Epson Corporation, Japan) with a transparency adapter. Total root length was determined using WinRhizo version 2007 (Regent Instruments Inc., Saint-Foy, Canada) (Bouma et al., Citation2000). Lateral root lengths per plant were calculated by subtracting the nodal from the total root lengths. The branching index was the lateral root length divided by the nodal root length (Morita & Collins, Citation1990).
Stomatal conductance was measured only in 2012. The measurements were obtained using a portable photosynthesis analyzer (LI-6400, LI-COR, Lincoln, NE, USA) on the abaxial side of the topmost fully developed leaf (67‒69 DAT) on the main stem. Measurements were taken between 09:00 and 12:00. Only two replications were performed, precluding statistical analyses.
Volumetric soil moisture content (SMC) per plant was monitored at 0–12 cm soil depth using a Time Domain Reflectometry probe (TDR; Tektronix Inc., Wilsonville, OR, USA). Soil moisture measurements 10 cm from opposite sides of a plant were averaged to yield the SMC. The SMC was divided into four ranges using published criteria (Menge et al., Citation2016): well-watered (> 30% v/v of SMC in 2011 and 2012), mild drought (21–30% v/v of SMC in 2011 and 24–30% v/v of SMC in 2012), moderate drought (12–21% v/v of SMC in 2011 and 16–24% v/v of SMC in 2012) and severe drought (<12% v/v of SMC in 2011 and <16% v/v of SMC in 2012) (henceforth, mentioning of SMC will be in v/v). These divisions allowed for statistical evaluations of how various drought-stress intensities affected shoot and root growth. All plants in each SMC category and N treatment were sampled from three replicate groups to identify varietal differences in SDW and total root length. Values from three replicates were averaged for statistical analyses.
2.5. Statistical analysis
To examine the effects of SMC, N, variety, and their interactions on SDW and total root length, an ANOVA was performed using the general linear model (GLM) procedure in SAS version 9.0 (SAS Institute Inc., Cary, NC, USA). Soil moisture was the main plot factor, N treatment was the subplot factor, and variety was the sub-subplot factor. Multiple comparisons of the three varieties by least significant difference (LSD) were conducted at each soil moisture range for each N fertilization level. The student t-test was also conducted for shoot dry weight and total root length to detect their differences from well-watered conditions for each variety at each N fertilization level. The relationship between total root length and SDW was determined in Microsoft Excel 2007 (Redmond, WA, USA).
3. Results
3.1. Shoot growth response
In both study years, SMC, N, and variety significantly affected SDW (), as did the interactions between SMC and N, SMC and variety, as well as N and variety. Furthermore, all three factors interacted to influence SDW in both years.
Table 1. Analysis of variance results showing significant differences in shoot dry weight (SDW, g plant−1) and total root length (TRL, m plant−1) at 67 d and 123 d after transplanting in 2011 and 2012, respectively, based on soil moisture content (SMC), nitrogen (N) level, variety (V), and their interactions.
Average heading dates ranged 67‒71 DAT for both NERICA varieties, thus sampling was done at different growth stages in the two years. However, genotypic differences in shoot growth were consistent in both years. Shoot dry weights of NERICA 1, NERICA 4, and Lemont were lower in 2011 than in 2012 (). Minimum and maximum SMC were 5% and 44%, respectively (). When optimal N fertilization was applied, NERICA 1 and 4 SDW peaked at a lower SMC than Lemont SDW. The lowest SMC to yield an SDW peak was 26% at medium N fertilization in NERICA 1 and 29% at high N in NERICA 4, both during 2011 (). Under low N, DMP of all varieties peaked at SMC >30%.
Figure 1. Relationships between soil moisture content and shoot dry weight in NERICA 1 (●—), NERICA 4 (○--), and Lemont (×–·) at 60 (low N), 120 (medium N), and 180 (high N) kg N ha−1 in 2011 (a‒c) and 2012 (d‒f). Data from the two years were measured at 67 and 123 d after transplanting, respectively. The regression equation for the curve y=ax2+bx+c was used to determine the soil moisture content to yield a shoot dry weight peak using the formula – b/2a.
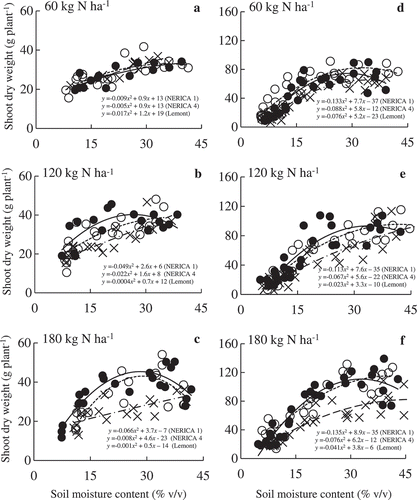
Under optimal N fertilization, Lemont SDW was lower than the SDW of both NERICAs during well-watered, mild drought, and moderate drought conditions (). Under severe drought, however, stunted growth and signs of wilting were observed. Consequently, dry matter accumulation in both years was the lowest under severe drought. The relative reduction in SDW under severe drought did not differ across varieties at any N treatment; in 2011 and 2012, respectively, the decrease ranged from 41 to 49% and 64 to 74% of the SDW in well-watered plants. N fertilization had a significant effect on NERICA shoot growth, but only under moderate drought. Under medium and high N, NERICA 1 had consistently and significantly higher SDW than NERICA 4 during moderate drought; however, these differences were unclear under low N (). Under optimal N fertilization, Lemont decreased DMP by approximately 40%, the largest drop among the three varieties in response to moderate drought ().
Table 2. Shoot dry weights at 67 d and 123 d after transplanting in 2011 and 2012, respectively for NERICA 1, NERICA 4, and Lemont under well-watered conditions (>30% v/v), mild drought (21‒30% v/v of soil moisture content [SMC] in 2011 and 24‒30% v/v of SMC in 2012), moderate drought (12‒21% v/v of SMC in 2011 and 16‒24% v/v of SMC in 2012), and severe drought (<12% v/v of SMC in 2011 <16% v/v of SMC in 2012) at varying levels of N fertilization.
3.2. Root growth response
In both years, SMC, N, variety, as well as the SMC by N and SMC by variety interactions, significantly affected total root length. In 2012 only, the interactive effect of N and variety on total root length was significant. In addition, during 2011, the three factors interacted to significantly affect total root length.
Under optimal N, the development of NERICA 1 and 4 root systems increased sharply in response to intensifying drought, compared with being under low N (). Regardless of N treatment, total root lengths of NERICAs peaked under drier soil moisture conditions. One exception was the low N condition in 2012, where the total root lengths of all varieties peaked under well-watered conditions. Overall, total root length of NERICA 1 tended to have peak root length at a lower soil moisture than NERICA 4 and Lemont across N treatments.
Figure 2. Relationships between soil moisture content and total root length in NERICA 1 (●—), NERICA 4 (○--), and Lemont (×–·) at 60 (low N), 120 (medium N), and 180 (high N) kg N ha−1 in 2011 (a‒c) and in 2012 (d‒f). Data from the two years were measured at 67 and 123 d after transplanting, respectively. The regression equation for the curve y=ax2+bx+c was used to determine the soil moisture content to yield a total root length peak using the formula – b/2a.
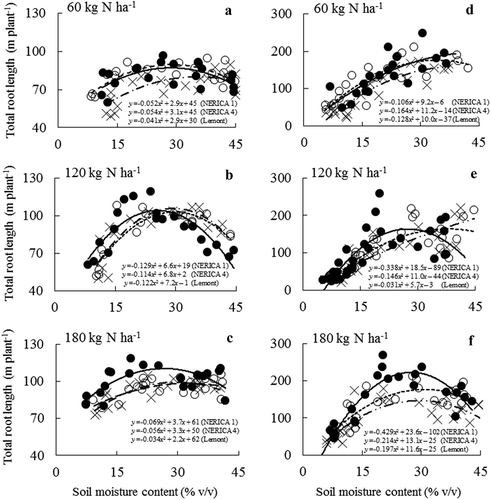
Using as reference the SMC that led to peak NERICA 1 root length, we calculated and compared total root lengths across the three varieties. At medium N in 2012, NERICA 1’s total root length peaked at 164 m (27.4% of SMC), whereas NERICA 4 and Lemont root lengths were 148 and 130 m, respectively. At high N, NERICA 1’s peak root length was 222 m at 27.5% of SMC compared with 174 m for NERICA 4 and 146 m for Lemont (). Under optimal N fertilization in both years, NERICA 1 tended to have a larger root system during drought than NERICA 4 and Lemont. However, under low N in 2011, NERICA 1’s total root length peaked at 87 m (28.6% of SMC) compared with 89 m for NERICA 4 and 78 m for Lemont.
Regardless of N treatment, NERICA 1 and NERICA 4 root lengths did not differ under well-watered, mild drought, or severe drought conditions in either year (). In contrast, at medium and high N fertilization, NERICA 1 had significantly higher root length than NERICA 4 under moderate drought conditions (12–21% of SMC in 2011 and 16–24% of SMC in 2012). NERICA 1 and NERICA 4 had comparable total root lengths under moderate drought with low N fertilization. Differences in root system development between Lemont and NERICAs were greatest under moderate drought with medium or high N fertilization ().
Table 3. Total root length at 67 d and 123 d after transplanting in 2011 and 2012, respectively, for NERICA 1, NERICA 4, and Lemont under well-watered conditions (>30% v/v of soil moisture content [SMC] in 2011 and 2012), mild drought (21‒30% v/v of SMC in 2011 and 24‒30% v/v of SMC in 2012), moderate drought (12‒21% v/v of SMC in 2011 and 16‒24% v/v of SMC in 2012), and severe drought (<12% v/v of SMC in 2011 <16% v/v of SMC in 2012) at varying levels of N fertilization.
Compared with well-watered conditions, severe drought stress significantly reduced total root length for all varieties (2011 range in relative reduction: 11–37%; 2012 range: 30–67%), regardless of N treatment (). However, under mild drought, the 2011 total root length for NERICA 1 significantly increased by 23% at medium N, while the 2012 total root length increased by 33% and 26% for NERICA 1 and 4 at high N, respectively. In contrast, under moderate drought for both years, only NERICA 1’s total root length significantly increased (relative to well-watered conditions) by 26–33% and 10–51% at medium and high N, respectively. The development of Lemont’s root system under mild and moderate drought was comparable to development under well-watered conditions when N supply was optimal. Moreover, low N fertilization did not significantly enhance root system development under moderate or mild drought for any variety.
Because we observed genotypic differences between NERICA 1 and 4 in root growth enhancement under moderate drought, we evaluated developmental traits contributing to this response (). Nodal root length did not significantly differ across varieties except at high N in 2012, being significantly higher in NERICA 1 than in the other two varieties (). Lateral root length was also significantly higher in NERICA 1 than in NERICA 4 and Lemont at medium and high N, but not at low N.
Table 4. Nodal root length (NRL), lateral root length (LRL), branching index (BI) and root-to-shoot ratio at 67 d and 123 d after transplanting in 2011 and 2012, respectively, for NERICA 1, NERICA 4, and Lemont under moderate drought (12‒21% v/v of SMC in 2011, and 16‒24% v/v of SMC in 2012) at varying N fertilization levels.
Regardless of N treatment, NERICA 1 tended to have a higher branching index than NERICA 4, with the differences being more pronounced at medium and high N than at low N. Furthermore, NERICA 1’s branching index was greater with increased N application. Across N treatments, NERICA 1 and NERICA 4 had comparable root-to-shoot ratios, but both tended to be lower than Lemont at optimal N fertilization. In NERICAs, root-to-shoot ratio decreased with increasing N fertilization under moderate drought, while in Lemont, the trait remained relatively constant.
3.3. Response of stomatal conductance
Across N treatments, Lemont stomatal conductance decreased linearly with increasing drought intensity (). A similar response was apparent for NERICA 1 and NERICA 4 at low N fertilization. In contrast, stomatal conductance under medium and high N peaked at 24–30% of SMC for NERICA 1, while the peak for NERICA 4 occurred under high N. Although not statistically tested, differences between the two NERICAs in stomatal conductance were negligible under well-watered conditions and severe drought. Under moderate drought with high N, stomatal conductance between the two NERICAs exhibited the greatest difference, being 0.35 mol m−2s−1 higher in NERICA 1 than in NERICA 4 (). Stomatal conductance of NERICA 4 tended to be lower than that of the lowland check under moderate drought at medium N application.
Figure 3. Stomatal conductance of the newest, fully developed leaf on the main tiller for NERICA 1, NERICA 4, and Lemont at 60 (low N), 120 (medium N), and 180 (high N) kg N ha−1 under different soil moisture conditions in 2012. Each bar shows an average of two replicates, precluding statistical analysis. Stomatal conductance was determined between 10:00 and 12:00 h, 54 d after transplanting.
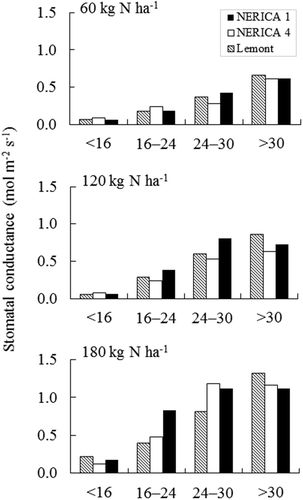
3.4. Relationship between total root length and shoot dry weight
Total root length and SDW were not significantly correlated under well-watered, mild drought, or severe drought conditions for any variety (data not shown). However, under moderate drought, the two traits were significantly and positively correlated in NERICA 1 subjected to medium and high N fertilization (). In contrast, the two traits were not significantly related in any variety at low N under moderate drought.
Figure 4. Relationships between total root length and shoot dry weight for NERICA 1 (● –), NERICA 4 (○), and Lemont (×) grown under 12‒21% (v/v) soil moisture content in 2011 (a‒c) and 16‒24% (v/v) soil moisture content in 2012 (e‒f). Rice were subjected to 60 (low N), 120 (medium N), and 180 (high N) kg N ha−1 fertilization. Shoot dry weights and total root lengths were determined at 67 d and 123 d after transplanting in 2011 and 2012, respectively.
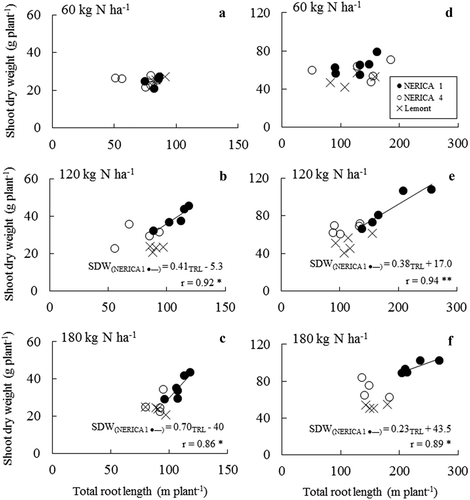
4. Discussion
Previously, we showed that enhanced root growth in a shallow soil layer (due to physical impediments preventing deep root development) was a key trait in upland NERICA that are adapted to drought stress (Menge et al., Citation2016). In this study, we evaluated the combined effects of soil moisture and N availability on shoot DMP and root system development in two upland NERICA varieties, using the lowland variety Lemont as a check. A line-source sprinkler system was used to simulate a soil moisture gradient in field conditions that was set up to impede deep root growth. We observed stunted growth and leaf rolling under severe drought, while between-variety differences in stomatal conductance were present under mild and moderate drought (). These results indicate that the soil moisture manipulation was appropriate for evaluating differences in how rice varieties respond to drought at varying N fertilization levels.
At optimal N fertilization, Lemont’s SDW tended to peak under wetter soil moisture conditions (> 30%) than those of the two NERICAs indicating that upland rice adapted to drier soil moisture conditions than lowland rice. On the other hand, NERICA 1 experienced peak SDW under drier conditions than NERICA 4, with deep rooting characteristics, at medium and high N (). This variation in aboveground responses between the two NERICAs indicates that soil depth and nutrient status influenced the differing drought-avoidance traits in tested varieties.
We found that upland rice growth and yield were significantly affected by the interaction between drought stress and nutrient availability (), consistent with previous reports on rainfed ecosystems (Prasertsak & Fukai, Citation1997). However, the two NERICA varieties only differed significantly in shoot-growth responses to N fertilization under moderate drought (). This outcome suggests that upland rice production has considerable potential for improvement under cultivation environments that are moderately dry. Existing evidence suggests that appropriate fertilizer management is an important strategy for increasing rice yield in rainfed lowland conditions (Haefele et al., Citation2008). Here, we demonstrated that soil nutrient availability strongly influenced drought traits of upland rice, as NERICA 1 maintained DMP during moderate drought under optimal N fertilization, while that of NERICA 4 was reduced (). Furthermore, there is probably a varietal difference in how fertilizer management improved NERICA drought tolerance, given the observed interactive effect among soil moisture, N level, and variety on shoot growth (). In contrast, N application seemed to negatively affect tolerance to drought for lowland rice as Lemont experienced the greatest dry matter reduction under moderate drought with high N fertilization (). This finding is consistent with that reported by Yambao and O’Toole (Citation1984) in lowland rice indicating that the variety IR24 used in that study suffered a greater decrease in DMP under high N fertilization than low N.
Phenotypic plasticity is known to stabilize plant yield in stressful environments (Yamauchi et al., Citation1996). A shift from well-watered to drought conditions increased total root length in both NERICAs ( & ), demonstrating root plasticity. However, drought severity has differential effects on rice root growth, given that total root length under moderate drought and optimal N fertilization was higher for NERICA 1 but not for NERICA 4 and Lemont (). These observed varietal differences can be exploited to improve drought tolerance in upland rice.
In lowland rice root plasticity was clearly expressed when applied N was optimal, whereas low N suppresses that plasticity (Tran et al., Citation2014). Such a scenario was confirmed in this study in upland rice where the root lengths of NERICA 1 and 4 were comparable under moderate drought and low N ( and ). However, at higher N fertilization, NERICA 1 had significantly greater root lengths than NERICA 4. In most sub-Saharan Africa, countries that have widely adopted NERICA, fertilizer application rates are below recommended levels (Oikeh et al., Citation2008). Our findings suggest that the low productivity of upland rice in this region can be partly attributed to poor root development, considering the importance of roots in adapting to environments that experience frequent episodes of soil moisture deficit.
Under moderate drought, genotypic differences in root developmental traits were influenced by N availability. For instance, both NERICAs had higher root-to-shoot ratios at low N than when N fertilization was optimal while in the lowland check, Lemont, this trait was relatively unchanged across N treatments (). Root-to-shoot ratio is a trait that increases under drought (Matsui & Singh, Citation2003). Therefore, our findings suggest that if low nutrient stress compounds drought stress, more photosynthetic assimilates are likely to be portioned to the roots. In the report by Tran et al. (Citation2014) on lowland rice, the mechanism of the expression or suppression of root plasticity under drought at different N soil conditions was not clarified. The findings of this study indicate that under the conditions of low N, the suppression of root plasticity under moderate drought may not be a result of dry matter distribution to roots, but rather a direct effect of insufficient N supply. Lemont tended to have a higher root-to-shoot ratio than both NERICAs under moderate drought and optimal N fertilization, but these differences were only significant when N fertilization was high (). This implies that the share of assimilates portioned to roots under drought may be higher in lowland than upland rice. However, the increased portioning of dry matter to roots may not be directly correlated with drought-induced root plasticity in Lemont because its root growth was not enhanced under moderate drought (). In contrast, NERICA 1 enhanced its branching index under moderate drought (), leading to greater lateral root length and root plasticity than NERICA 4 and Lemont (at medium and high N fertilization; and ). This pattern indicates that drought-induced root plasticity in NERICA is attributable to efficiency in lateral root development. Moreover, NERICA 1 had significantly greater nodal root length than NERICA 4 and Lemont in 2012, suggesting that nodal root formation and elongation are important to drought-related root plasticity. Although nodal roots constitute a small proportion of the rice root system, they provide the framework for lateral root distribution in the soil profile (Araki et al., Citation2002; Yamauchi et al., Citation1996).
NERICA 1 exhibited a significant correlation between total root length and SDW under moderate drought and optimal N fertilization (), again indicating that root plasticity is critical to drought resistance in rice. Furthermore, the interaction between soil moisture and N appears to affect the functional roles of root plasticity, consistent with previous findings by Tran et al. (Citation2014) for lowland rice. In that study however, the relationship between root length and SDW in lowland rice was recorded under conditions of greater soil moisture than our current study. These findings indicate that rice varieties differ in soil moisture range at which their degree of root plasticity is most effective.
Due to its increased root development under moderate drought, NERICA 1 experienced elevated DMP, a morphological change reflected in its higher stomatal conductance compared with NERICA 4 and Lemont (). Stomatal conductance is an indirect measure of root water uptake under soil water deficit (Kano et al., Citation2011; Matsuo et al., Citation2010; Tran et al., Citation2014). Thus, NERICA 1’s larger root system allowed it to obtain more water than NERICA 4 and Lemont under moderate drought. Stomatal closures resulting from low water uptake is a likely effect of poor root system development (Suralta et al., Citation2010). In this study, NERICA 4 was expected to show a similar growth response to Lemont during drought under the conditions of an impediment to deep root growth. However, the former variety tended to be superior in maintaining DMP under moderate drought than the latter variety (). In contrast, stomatal conductance was lower in NERICA 4 than Lemont during moderate drought specifically under medium N fertilization (), which may suggest that the stomatal closure in NERICA 4 was an adaptive strategy to avoid water loss. Thus, differences in aboveground growth between NERICA 4 and Lemont probably indicate that upland rice may respond more efficiently to drought stress than lowland rice by switching to other drought avoidance mechanisms if the root traits are not expressed. However, further studies are needed to clarify the physiological functions of root plasticity such as water or nutrient uptake in upland rice, and clarify differences between upland and lowland rice in other drought avoidance traits, besides rooting characteristics.
5. Conclusion
This study examined how N fertilization affected upland NERICA 1 and 4 root plasticity when deep root growth is impeded in comparison with Lemont, a lowland variety. NERICA 1 was better able to maintain DMP than NERICA 4 and Lemont under moderate drought at medium and high N treatments, because of enhanced root development. The root plastic response in NERICA 1 was as a result of lateral root development rather than increased dry matter allocation to roots. At low N, however, the two NERICA varieties had comparable growth responses because root plasticity was suppressed probably due to inability to utilize the dry matter allocated to roots as a direct effect of insufficient N soil status. Our results revealed that regulation of N application rates can manipulate root morphology in upland NERICA, implying that productivity is improvable through N fertilization. However, the efficacy of N may differ across variety and field conditions. Thus, an appropriate strategy for enhancing productivity in a drought-prone environment (e.g. uplands of sub-Saharan Africa) should involve both the selection of suitable, well-adapted varieties and appropriate management. However, we note that our results must be validated under actual upland conditions in sub-Saharan Africa. Moreover, the association of root plasticity with NERICA yield and yield components during drought should be clarified.
Acknowledgments
This work was supported by the Strategic Funds for the Promotion of Science and Technology, Ministry of Education, Culture, Sports, Science and Technology (MEXT) of Japan, and partially supported by the Japan Science and Technology Agency (JST)/Japan International Cooperation Agency (JICA), as well as the Science and Technology Research Partnership for Sustainable Development (SATREPS).
Disclosure statement
No potential conflict of interest was reported by the authors.
References
- Araki, H., Morita, S., Tatsumi, J., & Iijima, M. (2002). Physiol-morphological analysis on axile root growth in upland rice. Plant Production Science, 5, 286–293.
- Balasubramanian, V., Sie, M., Hijmans, R. J., & Otsuka, K. (2007). Increasing rice production in sub-Saharan Africa: Challenges and opportunities. Advances in Agronomy, 94, 55–133.
- Bañoc, D. M., Yamauchi, A., Kamoshita, A., Wade, L. J., & Pardales, J. R. (2000). Dry matter production and root system development of rice cultivars under fluctuating soil moisture. Plant Production Science, 3(2), 197–207.
- Becker, M., Johnson, D. E., Wopereis, M. C. S., & Sow, A. (2003). Rice yield gaps in irrigated systems along agro-ecological gradient in West Africa. Journal of Plant Nutrition and Soil Science, 166, 61–67.
- Bouma, T. J., Nielsen, K. L., & Koutstaal, B. (2000). Sample preparation and scanning protocol for computerised analysis of root length and diameter. Plant and Soil, 218, 185–196.
- Cairns, J. E., Impa, S. M., O’Toole, J. C., Jagadish, S. V. K., & Price, A. H. (2011). Influence of the soil physical environment on rice (Oryza sativa L.) response to drought stress and its implications for drought research. Field Crops Research, 121, 303–310.
- Castillo, E. G., Buresh, R. J., & Ingram, K. T. (1992). Lowland rice yield as affected by timing of water deficit and nitrogen fertilization. Agronomy Journal, 84, 152–159.
- Diagne, A., Midingoyi, S. G., Wopereis, M., & Akintayo, I. (2010). The NERICA success story: Development, achievements and lessons learned. Cotonou: Africa Rice Cente. Retrieved from http://siteresources.worldbank.org/AFRICAEXT/Resources/258643-1271798012256/NERICA-Success-Story-11-2010.pdf
- Dingkuhn, M., Jones, M. P., Johnson, D. E., & Sow, A. (1997). Growth and yield potential of Oryza sativa and Oryza glaberrima upland rice cultivars and their interspecific progenies. Field Crops Research, 57, 57–69.
- Fan, J. B., Zhang, Y. L., Turner, D., Duan, Y. H., Wang, D. S., & Shen, Q. R. (2010). Root physiological and morphological characteristics of two rice cultivars with different nitrogen-use efficiency. Pedosphere, 20, 446–455.
- Fischer, G., van Velthuizen, H. T., Shah, M. M., & Nachtergaele, F. O. (2002). Global agroecological assessment for agriculture in the 21st century: Methodology and results. Laxenburg: IIASA.
- Gupta, P. C., & O’Toole, J. C. (1986). Upland rice: A global perspective. Los Baños: International Rice Research Institute.
- Haefele, S. M., Jabbar, S. M. A., Siopongco, J., Tirol-Padre, A., Amarante, S. T., Cruz, P. C. S., & Cosico, W. C. (2008). Nitrogen use efficiency in selected rice (Oryza sativa L.) genotypes under different water regimes and nitrogen levels. Field Crops Research, 107, 137–146.
- IRRI. (2017). Ricepedia: The online authority on rice. Retrieved from http://ricepedia.org/rice-as-food/the-global-staple-rice-consumers
- Jones, M. P., Dingkuhn, M., Aluko, K. G., & Semon, M. (1997). Interspecific Oryza sativa L. × O. glaberrima Steud. progenies in upland rice improvement. Euphytica, 92, 237–246.
- Kamoshita, A., Babu, R. C., Boopathi, N. M., & Fukai, S. (2008). Phenotypic and genotypic analysis of drought-resistance traits for development of rice cultivars adapted to rainfed environments. Field Crops Research, 109, 1–23.
- Kaneda, C. (2007). Breeding and dissemination efforts of NERICA (1) Breeding of upland rice. Japanese Journal of Tropical Agriculture, 51, 1–4.
- Kano, M., Inukai, Y., Kitano, H., & Yamauchi, A. (2011). Root plasticity as the key root trait for adaptation to various intensities of drought stress in rice. Plant and Soil, 342, 117–128.
- Kato, Y., Kamoshita, A., Yamagishi, J., & Abe, J. (2006). Growth of three rice (Oryza sativa L.) cultivars under upland conditions with different levels of water supply 1. Nitrogen content and dry matter production. Plant Production Science, 9(4), 422–434.
- Kijima, Y., Sserunkuuma, D., & Otsuka, K. (2006). How revolutionary is the “NERICA revolution”? Evidence from Uganda. Developing Economies, 44, 252–267.
- Kono, Y., Tomida, K., Tatsumi, J., Nonoyama, T., Yamauchi, A., & Kitano, J. (1987). Effects of soil-moisture conditions on the development of root systems of soybean plants (Glycine max Merr). Japanese Journal of Crop Science, 56, 597–607.
- Lopez-Bucio, J., Cruz-Ramirez, A., & Herrera-Estrella, L. (2003). The role of nutrient availability in regulating root architecture. Current Opinion in Plant Biology, 6, 280–287.
- Lynch, J. (1995). Root architecture and plant productivity. Plant Physiology, 109, 7–13.
- Maclean, J., Dawe, D. C., Hardy, B., & Hettel, G. (2002). Rice Almanac. Oxford: CABI.
- Matsui, T., & Singh, B. B. (2003). Root characteristics in cowpea related to drought tolerance at the seedling stage. Experimental Agriculture, 39, 29–38.
- Matsunami, M., & Kokubin, M. (2011). Yield responses of upland NERICAs under rainfed upland conditions with different levels of nitrogen application. Japan Agricultural Research Quarterly (JARQ), 45, 243–249.
- Matsuo, N., Ozawa, K., & Mochizuki, T. (2010). Physiological and morphological traits related to water use by three rice (Oryza sativa L.) genotypes grown under aerobic rice systems. Plant and Soil, 335, 349–361.
- Menge, D. M., Kameoka, E., Kano-Nakata, M., Yamauchi, A., Asanuma, S., Asai, H., … Makihara, D. (2016). Drought-induced root plasticity of two upland NERICA varieties under conditions with contrasting soil depth characteristics. Plant Production Science, 19(3), 389–400.
- Morita, S., & Collins, H. (1990). A method to describe root branching. Japanese Journal of Crop Science, 59, 580–581.
- Ndjiondjop, M. N., Futakuchi, K., Cisse, F., Baimey, H., & Bocco, R. (2012). Field evaluation of rice genotypes from the two cultivated species (Oryza sativa L. and Oryza glaberrima Steud.) and their interspecifics for tolerance to trought. Crop Science, 52, 524–538.
- O’too, E., Ishii, R., & Kumura, A. (1989). Interaction of nitrogen supply and soil water stress on photosythesis and transpiration in rice. Japanese Journal of Crop Science, 58, 424–429.
- Oiken, S. O., Nwilene, F., Diatta, S., Osiname, O., Touŕe, A., & Okeleye, K. A. (2008). Responses of upland NERICA rice to nitrogen and phosphorus in forest agroecosystems. Agronomy Journal, 100(3), 735–741.
- Prasertsak, A., & Fukai, S. (1997). Nitrogen availability and water stress interaction on rice growth and yield. Field Crops Research, 52, 249–260.
- Sahrawat, K., & Sika, M. (2001). Comparative tolerance of Oryza sativa and Oryza glaberrima rice cultivars for iron toxicity in West Africa. International Rice Research Notes, 27(2), 31–32.
- Seck, P. A., Diagne, A., Mohanty, S., & Wopereis, M. C. S. (2012). Crops that feed the world 7: Rice. Food Security, 4, 7–24.
- Suralta, R. R., Inukai, Y., & Yamauchi, A. (2010). Dry matter production in relation to root plastic development, oxygen transport, and water uptake of rice under transient soil moisture stresses. Plant and Soil, 322, 87–104.
- Tran, T. T., Nakata-Kano, M., Takeda, M., Menge, D., Mitsuya, S., Inukai, Y., & Yamauchi, A. (2014). Nitrogen application enhanced the expression of developmental plasticity of root systems triggered by mild drought stress in rice. Plant and Soil, 378, 139–152.
- USDA. (2017). Production, supply and distribution online. Retrieved from http://www.fas.usda.gov/psdonline/
- Wang, H., & Yamauchi, A. (2006). Growth and function of roots under abiotic stress soils. In B. Huang (Ed.), Plant-environment interactions (3rd ed., pp. 271–319). New York, NY: CRC Press. doi:10.1201/9781420019346.ch10
- Xu, J. L., Lafitte, H. R., Gao, Y. M., Fu, B. Y., Torres, R., & Li, Z. K. (2005). QTLs for drought escape and tolerance identified in a set of random introgression lines of rice. Theoretical and Applied Genetics, 111, 1642–1650.
- Yamauchi, A., Inukai, Y., Kano, M., Suralta, R., Ogawa, A., Kobayashi, N., & Serraj, R. (2008). Role of roots for stabilizing rice production under water stress conditions. In The 5th international crop science congress (pp. 178–179). Jeju: International Crop Science Society.
- Yamauchi, A., Pardales, J. R., Jr., & Kono, Y. (1996). Root system structure and its relation to stress tolerance. In O. Ito, C. Johansen, J. J. Adu-Gyamfi, K. Katayama, J. V. D. K. Kumar, & T. J. Rego (Eds.), Dynamics of roots and nitrogen in cropping systems of the semi-arid tropics (pp. 211–223). Tsukuba: Japan International Research Center for Agricultural Sciences.
- Yambao, E. B., & O’Toole, J. C. (1984). Effects of nitrogen nutrition and root medium water potential on growth, nitrogen uptake and osmotic adjustment of rice. Physiologia Plantarum, 60, 507–515.