ABSTRACT
Natural root grafting is a well-known phenomenon in perennial tree species. This study examined whether root grafting is possible between the same and different annual crop species without any surgical action by using an instrument with a narrow and tight rooting passage to evaluate the application of natural root grafting. A part of the epidermal cell layer disappeared and cortex tissues of two individual plants became fused together in most of species examined. When two different species were grafted, sequential images proved that whole cowpea tap root was inserted into the cortex layer of mung bean. These results indicated that natural root grafting of annual crop species without any surgical action is possible when the two growing roots come in contact with each other in a narrow and rigid rooting channel.
Graphical Abstract
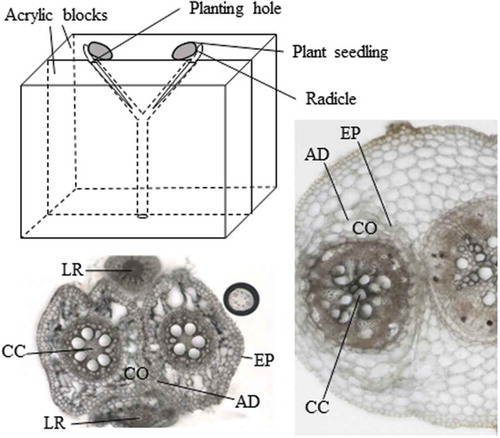
Roots of perennial woody species are known to be grafted naturally. When young roots come in contact with each other, they may fuse during continuous secondary growth over the years. Natural root grafting has been widely studied because it is a common phenomenon and often affects forest ecology (Mudge, Janick, Scofied & Goldschmidt, Citation2009). For example, Fraser, Lieffers and Landhäusser (Citation2005) reported that the occurrence of natural root grafting of lodgepole pine trees was related to planting distance between trees, i.e. it increased with decreasing the distance between trees. Natural root grafting would be influenced by spatial distance between trees and less importantly by the genetic distance between the individuals involved (Tarroux, DesRochers & Tremblay, Citation2014). Fraser, Lieffers and Landhäusser (Citation2006) concluded that naturally grafted trees growing in light-limited environments were partially supported by carbohydrates transferred across root grafts from their more vigorous partners. Water and carbohydrate can be transferred through root grafting, whereby root grafting affects tree growth. A recent study on natural root grafting of perennial trees indicated that root grafts slightly attenuated the negative effects of defoliation during and immediately after a spruce budworm (Choristoneura spp.) outbreak (Salomón, Tarroux & DesRochers, Citation2016).
As for annual species, however, natural root grafting was has not been reported up to date. Most probably, short-term primary root growth of annual species may not be enough for natural root grafting to occur as seen in perennial species. Regarding the agricultural use of ordinal grafting technique, it was widely used for perennial fruit species and annual vegetable crop species. In these crops, grafting of the stems is commonly performed and, in the case of watermelon and/or cucumber, for example, a grafting device has been developed (Bie, Nawaz, Huang, Lee & Colla, Citation2017). Numerous grafts can be carried out in a short time by using this grafting device, thus contributing to reduce labor needed for seedling preparation. This grafting instrument was developed for the shoot system of vegetable plants, and although a similar instrument for root systems has not been reported before, natural grafting of woody species may be considered while developing the grafting instrument for the root systems of annual species. In this study, we designed an instrument with narrow rooting passages to simulate the rooting environment that would lead to natural root grafting. Using this instrument, we examined whether root grafting of annual crop species is possible in order to assess the applicability of natural root grafting in field crop species without the need for any surgical action to reduce production cost.
Materials and methods
In this experiment, five leguminous crops species, i.e. pea(Pisum sativum L., cv. Akabana-Tsurunashi), soybean (Glycine max, cv. Tamahomare), mung bean (Vigna radiata, cv. Green mappe), cowpea (Vigna unguiculata, cv. Akadane-sanjaku), adzuki bean (Vigna angularis, cv. Tanba-Dainagon), and a cereal species, maize (Zea Mays, cv. Canberra) were used as experimental crop species. Seeds were surface-sterilized with 2.5% (v/v) sodium hypochlorite for 5 min and rinsed under running water for 20 min. They were sown on paper towels wetted with deionized water in a Petri dish. Pea seeds were pre-germinated in a dark incubator at 25°C for 41 h, and soybean, mung bean, cowpea, adzuki bean, and maize seeds were pre-germinated in a dark incubator at 30°C for 30, 24, 31, 45, and 42 h, respectively.
Seedlings were grown in either a plant growth room with a 28/23°C day/night temperature, a 14 h photoperiod (Iijima et al., Citation2017) or a glasshouse equipped with an automatic temperature control unit and artificial light because of the limited space for repeated trials. In both cases, seedlings with 25–35 mm radicles were selected and transplanted to the natural root grafting chamber provided with a narrow passage (). Natural root grafting is known to occur when roots come in contact with other roots that are tightly fixed to the soil (Mudge et al., Citation2009). Thus, our grafting chamber was designed to enhance root grafting naturally, without surgical operation, that is, two different roots were made to grow along a narrow passage through which only one root can pass. The two root tips came in contact in the joint portion of the two channels. Then, the two single roots were pressed in close contact with each other by root growth pressure (Iijima, Higuchi, Barlow & Bengough, Citation2003). Roots must fuse with each other, otherwise they cannot continue growing. Thereafter the fused roots will grow in a single channel downward to the bottom of the chamber. The seeds were fixed by elastic tape to the chamber and the radicle was allowed to grow downward through the rooting hole. The chamber was sustained on the top of water surface of a culture solution, so that the root growth channel was always wet by capillary action. Additional distilled water was supplied from the top of the rooting channel when the channel became dry. The strength of the culture solution (Hoagland & Arnon, Citation1950) was gradually increased as plant age increased from 0.2 to 1.0 strength. In the growth chamber experiment, the seedlings of pea, cowpea, and maize were grown in the dark for the first day to avoid light stress to root growth (Iijima & Matsushita, Citation2011), and then placed under a metal halide lamp. The chamber was wrapped by a dark cloth to minimize light penetration into the chamber during the course of the study. Photosynthetically active radiation (PAR) was set to 105 ± 4, and 236 ± 8 µmol m−2 s−1 at the top of the canopy, during the first two weeks and the following two weeks, respectively.
Figure 1. Schematic diagram of the natural root-grafting chamber without any surgical operation. A Y-shape rooting passage was made on the contact surface of two transparent acrylic blocks. Radicles were inserted to the planting hole to direct the roots to grow along the rooting channel. The seeds and/or lower parts of the coleoptile were fixed on the top of an acrylic panel by a masking tape. At sampling, the two blocks were separated, and roots were easily removed from the rooting passage. Height, length, width, and planting hole diameter of the chamber are 60mm, 40mm, 30mm and 4mm, respectively.
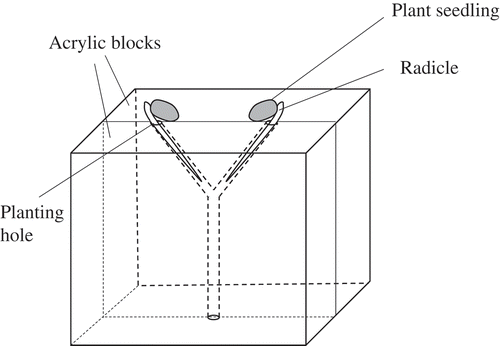
In the glasshouse experiment, all of the species were examined and the chamber was exposed to natural and artificial light (Iijima et al., Citation2016) during the course of the experiment. The temperature of the glasshouse averaged 28.8ºC, with maximum and minimum temperatures of 35.1ºC and 18.6ºC, respectively. Day length was set to 14 h using artificial light from metal halide lamps. The artificial light at the top of canopy was 318 ± 2 µmol m−2 s−1. Root sampling was done at 28 days after sowing, except for mung bean and cowpea glasshouse experiment, in which case, sampling was performed at 14 days after sowing because of combination of different species. Sampled roots were observed under a stereoscopic microscope (OLYMPUS SZ-PT, Olympus Corporation, Tokyo), and the possible portion of root fusion was fixed by FAA (Formaldehyde: Acetic acid: Ethanol (70%) = 2:1:7) after cutting.Fixed root samples were embedded in agar (6% w/w) and cross sections were obtained by a vibrating blade microtome (LEICA VT1200, Leica Microsystems, Tokyo). The thickness of the sections was 40, 80, 60, 150, and 200 µm for pea, soybean, maize, mung bean, and cowpea-mung bean, respectively. The sections were observed under a microscope(OLYMPUS BX50, Olympus Corp., Tokyo).
Results and discussion
In this study we examined the possibility of natural root grafting in six annual crop species including, five leguminous and one cereal species (). The fusion of the root axes of two different plants was evaluated by the morphological changes in the transverse section of first emerged seminal roots for maize, tap roots of leguminous species, and 1st order lateral roots (Iijima, Kono, Yamauchi & Pardales, Citation1991) for both cereal and leguminous species. In most species examined, a part of the epidermal cell layer disappeared (), and cortex tissue of the two different plants was fused, which is designated as ‘cortex fusion’ (). Five combination of cortex fusion, that is, seminal vs seminal, tap vs tap, seminal vs lateral, tap vs lateral, and lateral vs lateral were observed, except for seminal vs tap roots. In cowpea and adzuki bean, however, epidermal cell layer was visible and cortex fusion was not observed, which is designated as ‘epidermal adhesion’ (). In epidermal adhesion, the epidermal layer was tightly compressed and the two root axes did not depart during sample preparation. The combination of any of the five patterns above and both epidermis and cortex fusion were regarded as success, and success rates were calculated (). The success rates were around 10 to 30% for all the combination. The occurrence of natural root grafting may be related to the duration of root contact and/or plant age as reported by Tarroux and Des Rochers (Citation2010). shows the example of cortex fusion for pea, soybean, mung bean, and maize. The shape of transverse sections of individual roots were not circular but more or less looked collapsed, oval and/or fan. Root cross sections showed that a part of the epidermis and/or cortex layer was remarkably deformed. In soybean, most of the cortex layer disappeared and the central cylinder of the two plants was positioned at a close distance. Typical callus differentiation and vascular connection observed in grafted plants (Flaishman, Loginovsky, Golobowich & Lev-Yadun, Citation2008) or connection of the cambium in naturally grafted roots (Fraser et al., Citation2005) were not observed in this study.
Table 1. Success rates of natural root grafting without surgical operation using the experimental chamber designed (). The criteria for epidermis adhesion and cortex fusion are explained in the text.
The possibility of natural root-grafting in two different plant species was examined between mung bean and cowpea (). These species were selected because they are leguminous plants with secondary growth and similar growth rate immediately after germination. Sequential images proved that the whole cowpea tap-root was inserted into the cortex layer of the mung bean plant. The two species shared their cortex tissues, suggesting that they can utilize nutrients and/or water contained in their plant roots. Only one or two cortex cell layers were visible between central cylinders from two different species, although they never became fused together during the experimental period. These results indicated that, in the case of annual crop species, natural root grafting is possible for different plants of the same species. As for leguminous species, a combination of two different species was also possible.
In conclusion, root grafting of annual crop species without any surgical action is possible by the application of natural root grafting. When the two growing roots come in contact in a rigid rooting channel, the two roots of annual crop species fuse into one single root and share their cortex layers. Further research is necessary to study the vascular connection between the young roots of two individual plants.
Figure 2. Transverse sections of grafted roots without surgical operation of two different plants of a same annual crop species using the experimental chamber (). The disappearance of the epidermal layer and the adhesion of the root cortex cells are visible. a, Pea; b, Soybean; c, Mung bean; d, Maize. Bars = 200 μm. EP:Epidermis, CO:Cortex, CC:Central cylinder, LR:Lateral root, AD:Adhesion.
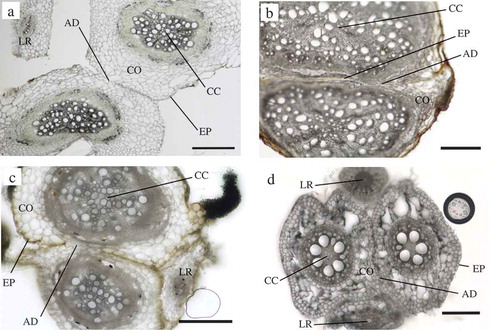
Figure 3. The invagination phenomenon of cowpea roots into mung bean roots by root grafting without surgical operation. Left, Cowpea; Right, Mung bean. a-x; Serial section from the joint portion of the Y-shape rooting passage in the experimental chamber (3160–15,700 μm). The positions of serial sections are shown in the vertical scale bar. s’ shows a partially expanded image of s. In s’, the horizontal bar represents 200 μm. EP:Epidermis, CO:Cortex, CC:Central cylinder, LR:Lateral root, AD:Adhesion.
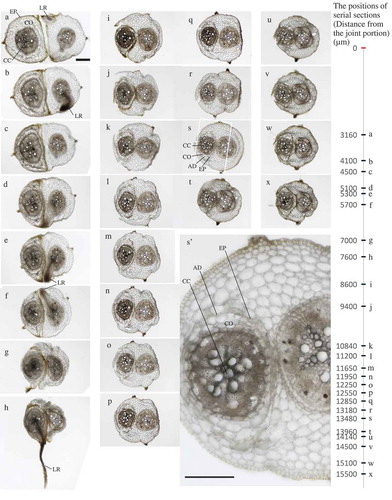
Acknowledgments
We thank the members of the project entitled ‘Flood- and Drought-adaptive Cropping Systems to Conserve Water Environments in Semi-arid Regions’ by the framework of the ‘Science and Technology Research Partnership for Sustainable Development (SATREPS)’ funded by the Japan Science and Technology Agency (JST) and the Japan International Cooperation Agency (JICA). We also thank the students and technical assistants of, crop science Laboratory, Faculty of Agriculture, Kindai University for their support.
Disclosure statement
No potential conflict of interest was reported by the authors.
References
- Bie, Z., Nawaz, M. A., Huang, Y., Lee, J. M., & Colla, G. (2017). Introduction of vegetable grafting. In G. Colla, F. P. Alfocea, & D. Schwarz (Eds.), Vegetable grafting, principles and practices (1st ed., pp. 1–21). Wallingford, UK: CABI Publishing.
- Flaishman, M. A., Loginovsky, K., Golobowich, S., & Lev-Yadun, S. (2008). Arabidopsis thaliana as a model system for graft union development in homografts and heterografts. Journal of Plant Growth Regulation, 27, 231–239.
- Fraser, E. C., Lieffers, V. J., & Landhäusser, S. M. (2005). Age, stand density, and tree size as factors in root and basal grafting of lodgepole pine. Canadian Journal of Botany, 83, 983–988.
- Fraser, E. C., Lieffers, V. J., & Landhäusser, S. M. (2006). Carbohydrate transfer through root grafts to support shaded trees. Tree Physiology, l26, 1019–1023.
- Hoagland, D. R., & Arnon, D. I. (1950). The water-culture method for growing plants without soil. Circular. California Agricultural Experiment Station, 347, 1–32.
- Iijima, M., Awala, S. K., Watanabe, Y., Kawato, Y., Fujioka, Y., Yamane, K., & Wada, K. C. (2016). Mixed cropping has the potential to enhance flood tolerance of drought-adapted grain crops. Journal of Plant Physiology, 192, 21–25.
- Iijima, M., Higuchi, T., Barlow, P. W., & Bengough, A. G. (2003). Root cap removal increases root penetration resistance in maize (Zea mays L.). Journal of Experimental Botany, 54, 2105–2109.
- Iijima, M., Hirooka, Y., Kawato, Y., Watanabe, Y., Wada, K. C., Shinohara, N., … Yamane, K. (2017). Short term evaluation of oxygen transfer from rice (Oryza sativa) to mixed planted drought-adapted upland crops under hydroponic culture. Plant Production Science, 20, 434–440.
- Iijima, M., Kono, Y., Yamauchi, A., & Pardales, J. R., Jr. (1991). Effects of soil compaction on the development of rice and maize root systems. Environmental and Experimental Botany, 31, 333–342.
- Iijima, M., & Matsushita, N. (2011). A circadian and an ultradian rhythm are both evident in root growth of rice. Journal of Plant Physiology, 168, 2072–2080.
- Mudge, K., Janick, J., Scofied, S., & Goldschmidt, E. E. (2009). A history of grafting. Horticultural Reviews, 35, 437–493.
- Salomón, R. L., Tarroux, E., & DesRochers, A. (2016). Natural root grafting in Picea mariana to cope with spruce budworm outbreaks. Canadian Journal of Forest Research, 46, 1059–1066.
- Tarroux, E., & Des Rochers, A. (2010). Frequency of root grafting in naturally and artificially regenerated stands of Pinus banksiana: Influence of site characteristics. Canadian Journal of Forest Research, 40, 861–871.
- Tarroux, E., DesRochers, A., & Tremblay, F. (2014). Molecular analysis of natural root grafting in jack pine (Pinus banksiana) trees: How does genetic proximity influence anastomosis occurrence? Tree Genetics & Genomes, 10, 667–677.