ABSTRACT
Enhancing crop traits that increase grain yield under elevated CO2 concentrations is an important option for increasing the future productivity of rice. Here, we compared the growth and yield of five varieties with different genetic background under Free-Air CO2 Enrichment (FACE) conditions to identify traits responsible for varietal differences in yield increase under elevated CO2. Three high-yielding and two standard rice varieties grown under FACE conditions commonly had (1) shorter growth periods, (2) higher dry matter production, (3) higher numbers of spikelets (sink capacity) and panicles; and (4) higher yield than those grown under ambient CO2. Yield enhancement by elevated CO2 (FACE/Ambient), however, differed significantly among varieties, ranging from 1.10 to 1.25. The greater response of the sink capacity, defined as the product of spikelet number and single grain mass, was the main factor involved in yield increase. Three high-yielding varieties (Momiroman, Takanari, and Hokuriku 193) had greater sink capacity than two standard varieties and the sink capacity of these varieties significantly increased under FACE condition. However, yield enhancement in elevated CO2 was lower in Hokuriku 193 than in Momiroman and Takanari. In Hokuriku 193, sink production was relatively low while dry matter production was similar to the others. Therefore, larger increase in sink production efficiency per unit of dry matter production under FACE was found to be a particularly important varietal trait, suggesting that efforts to develop varieties suited to the predicted elevated CO2 condition should focus on this and the related traits.
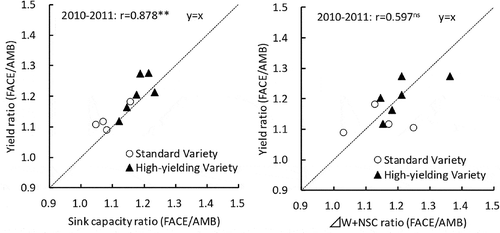
1. Introduction
Atmospheric CO2 concentration, which stood at 280 ppm 200 years ago, has risen constantly in recent years; it currently stands at approximately 400 ppm, and is expected to rise further to 550 ppm around 2050 unless strict mitigation measures are implemented (Meinshausen et al., Citation2011). The rise in CO2 concentration not only increases temperatures but is also expected to seriously affect food production. Increase in dry matter production, as a result of enhanced photosynthesis has been reported as an effect of rising CO2 levels on crop yield, but the degree of this increase differs among crop species (Ainsworth & Rogers, Citation2007; Ziska et al., Citation2012). To increase food production while addressing climate change and population growth, we need to pinpoint characteristics of crops adapted to future environments.
Rice is one of the world’s most important crops, and its consumption is growing, notably in low-income countries (GRiSP, Citation2013). However, the current rate of yield increase in rice will be insufficient to meet the growing food demand (Ray, Mueller, West & Foley, Citation2013). Our ability to maintain stable food production will depend on the development of production techniques for ensuring stable rice production and the breeding of varieties that produce high yields under elevated CO2 concentrations. This in turn urgently requires the identification of traits related to yield stabilization under elevated CO2 conditions.
Precise evaluation of crop growth response to elevated CO2 concentrations requires field experiments with crop communities. Free-air carbon dioxide enrichment (FACE) is an evaluation method developed in the late 1980s that involves elevating CO2 levels in open-air plant communities (Kobayashi, Citation2001). The method has since been used to conduct experiments on rice and many other crop species (Long, Ainsworth, Rogers & Ort, Citation2004). Examples of FACE experiments include studies conducted on rice in cool northern regions of Japan from the late 1990s up to 2008 and later resumed in the Kanto region (central Japan) in 2010. Similar studies have also been carried out in China since 2000 (Yang et al., Citation2007).
Numerous researches have been conducted in recent years on rice growth and yield under elevated CO2 conditions, and shown that rice biomass and yield increase under FACE (Kim et al., Citation2003; Yang et al., Citation2006, Citation2007; Liu et al., Citation2008; Shimono et al., Citation2009; Hasegawa et al., Citation2013, Citation2015; Usui et al., Citation2016) and growth chambers (Baker, Citation2004; Kim, Horie, Nakagawa & Wada, Citation1996b; Moya, Ziska, Namuco & Olszyk, Citation1998; Sasaki et al., Citation2005; Yoshida, Horie, Nakazono, Ohno & Nakagawa, Citation2011; Ziska, Manalo & Ordonez, Citation1996). Most of the reports showed that the yield increase was mainly due to an increase in the number of spikelets (Kim et al., Citation2003; Yang et al., Citation2006; Liu et al., Citation2008; Hasegawa et al., Citation2013, Citation2015; Usui et al., Citation2016; Yoshida et al., Citation2011). The magnitude of yield increase reportedly differs significantly among varieties. Ziska et al. (Citation1996) showed that yield increase under elevated CO2 levels ranged from −7% to 76% in their growth chamber experiments. FACE studies have demonstrated yield increases from 3% to 18% for four varieties (Shimono et al., Citation2009), 3% to 36% for eight varieties (Hasegawa et al., Citation2013), 3% to 30% for four varieties (Sakai, Tokida, Usui, Nakamura & Hasegawa, Citation2019) and equaling 34% for an F1 hybrid Indica variety (Liu et al., Citation2008).
The yield response to elevated CO2 was found to be greater for varieties that had greater responses in biomass and nitrogen uptake (Sakai et al., Citation2019; Shimono et al., Citation2009) or in sink capacity often represented by the number of spikelets (Hasegawa et al., Citation2013, Citation2015; Yoshida et al., Citation2011). The number of spikelets is strongly influenced by both nitrogen nutrition and dry matter accumulation during the reproductive growth phase (Kobayasi & Horie, Citation1994), but the genetic variability of these traits in response to elevated CO2 remains poorly understood. Thus, in order to determine the factors related to varietal differences in the yield response to elevated CO2, the evaluations of the interactions between yield and 1) biomass production, 2) nitrogen uptake, 3) sink production is important, using diverse varieties differing in these characteristics are necessary.
In recent years, various high-yielding varieties including japonica-indica hybrids have started to be cultivated in Japan for use as animal feed or rice flour (Yoshinaga, Takai, Arai-Sanoh, Ishimaru & Kondo, Citation2013). Given that the photosynthetic ability, biomass and sink production potentials of these varieties are considerably greater than those of standard varieties (Yoshinaga et al., Citation2013), they may well possess traits that are also advantageous for boosting yields under elevated CO2 levels.
Therefore, in this study, we compared the growth under elevated CO2 conditions of both standard and recent developed high-yielding varieties in Japan to identify traits responsible for differences in rice yield, with the eventual aim of developing cultivation techniques and varieties suited to the predicted elevated CO2 conditions.
2. Materials and methods
2.1. Field experimental details
Field experiments were conducted in the Tsukuba FACE site which was established in farmers’ rice fields in Tsukubamirai City, Ibaraki prefecture in Japan (35°58ʹN, 139°60ʹE) from 2010 to 2011. The soil is a Fluvisol, which is typical of alluvial areas. Five Japanese rice (Oryza sativa L.) genotypes with different genetic background, Koshihikari, Nipponbare, Momiroman, Takanari, and Hokuriku193 were grown under irrigated conditions. Nipponbare and Koshihikari are previous and current representative conventional japonica varieties which are popular for the consumption eaten as boiled rice in Japan. The cultivated areas of Nipponbare had been largest in the 1970s and those of Koshihikari have been largest from the late 1970s to the present. The others are multipurpose varieties which were developed as high-yielding, and used for flour and/or animal feed. In this report, Takanari and Hokuriku 193 were defined as indica-dominant varieties, Momiroman were defined as japonica-dominant varieties, based on the results in Yamamoto et al. (Citation2010).
Seeds were sown in a seedling nursery box, and 30-day-old seedlings were transplanted, with three seedlings per hill, on 26 May in 2010 and 25 May in 2011. The planting density was 22.2 hills m–2, with 30-cm row spacing and 15-cm intra-row spacing. Nitrogen fertilizers were supplied with urea and controlled release fertilizers (Type LP40, LP100 and LP140 polyolefin-coated urea, JCAM AGRI Co. Ltd., Japan Tokyo). The LP40, LP100, and LP140 fertilizers release 80% of their total nitrogen content at a uniform rate until 100 and 140 days after application, respectively, at temperatures between 20°C and 30°C. For all the varieties, the basal application contained 12 g N m–2 (3 g from urea, 6 g from LP100 and 3 g from LP140). The top-dressing provided 4 g N m–2 (from LP40) with the exception of Koshihikari due to its lower lodging resistance. Basal fertilizers were applied about 1 week before transplanting, and plots were top-dressed about 30 days before heading at the neck-node differentiation stage. Phosphorus and potassium (10.0 g P2O5 m–2 and 10.0 g K2O m–2 as fused fertilizer) were applied to all plots before puddling. Weeds, insects, and diseases were controlled by using standard herbicides and pesticides as required to avoid yield loss. The experimental plots were arranged in a split-plot design (main and subplots were CO2 concentration and variety, respectively) with four replicates. The size of each plot was 3.4 m2.
2.2. CO2 treatment
Atmospheric CO2 concentration in FACE experiment were controlled as described by Nakamura et al. (Citation2012). Average atmospheric CO2 concentration during growth duration in FACE plots were 584 µmol mol−1 in 2010 and 560 µmol mol−1 in 2011 and that in ambient plots was 386 µmol mol−1 in 2010 and 379 µmol mol−1 in 2011.
2.3. Measurement of dry matter production, nitrogen uptake, and NSC contents
Plants were sampled at the full-heading stage and the maturity. The full-heading date was defined as the date when about 80% of panicles in a canopy had emerged. Nine and 6 hills were sampled at full-heading and matured stage from each plot. Among them, two representative hills with average number of panicles, were selected and separated into four parts: leaf blades, leaf sheaths and culms, panicles, and dead parts. The weights of each part and of the entire plants were measured after drying for 72 h at 80°C.
Each dried sample was ground to a powder with a vibrating sample mill (TI-1001, CMT Co., Tokyo, Japan) for measuring nitrogen and non-structural carbohydrates (NSC) contents. The nitrogen content was measured by the Dumas combustion method (NCH analyzer, Sumika Chemical Analysis Service, Tokyo, Japan). The NSC contents of the stems and leaf sheaths were measured by the gravimetric method (Ohnishi & Horie, Citation1999).
2.4. Yield and yield components
At maturity, plants from 12 hills (0.72 m2) of each plot were harvested for the determination of yield and yield components. After the panicle number was counted, the panicles were threshed and the unhulled and hulled rice were weighed to measure the rough grain and hulled grain yields. Then, grains were screened by a grain sorter; the sieve size of the sorter was changed depending on the grain shape and size of each variety, namely 1.7 mm for Koshihikari, Takanari, and Hokuriku 193 with relatively longer grains, 1.8 mm for Nipponbare and Momiroman with medium grains. The 1000-grain weight was calculated using screened hulled grain. The rough grain yield, hulled grain yield, and 1000-grain weight were adjusted to 14% moisture content.
Forty to 80 g of subsample was selected from rough grain, using Sample Divider (Fujikinzoku, Tokyo, Japan). The number of spikelets per unit area was calculated as the number of spikelets of the subsample divided by the subsample weight and multiplied by the weight of the total amount of unhulled grain per unit area. The number of spikelets per panicle was calculated as the number of spikelets per area divided by the number of panicles per area. Sink capacity was defined as single grain weight multiplied by the number of spikelets per area. The sink-filling rate was calculated as the hulled grain yield divided by sink capacity.
2.5. Statistical analysis
Analysis of variance (ANOVA) was performed using ‘Statistix 9ʹ (Analytical Software, FL, USA), according to the split-plot design (main plot CO2 concentration, sub-plot variety) to assess varietal differences, the effects of CO2 concentration and of varieties X CO2 concentration interactions. The significance of mean values was analyzed using Tukey’s test (0.05).
3. Results
3.1. Climate conditions and growth duration
The climate conditions in standard variety Nipponbare control plots during the growing seasons are shown in . In both years, mean temperatures and solar radiation were higher than climatic normals during the period from transplanting to heading and during the grain-filling period (heading to maturity). This trend was particularly conspicuous during the grain-filling period in 2010, when mean temperature was 3.3°C and solar radiation was 3.6 MJ m–2 d–1 above climatic normals. Heading occurred earlier under FACE conditions than under ambient conditions (AMB), with the full-heading stage being reached 1 to 3 days earlier and growth period for all five varieties being an average of 2 days shorter in FACE plots than AMB plots ().
Table 1. Climate condition of Nipponbare with ambient in experiment years.
Table 2. Growth parameters of five rice varieties grown under ambient (AMB) and FACE.
3.2. Dry matter production and nitrogen uptake
Data for dry matter production and nitrogen uptake for each variety in both experimental years are shown in . Aboveground dry weight was found to differ among varieties at full-heading and at maturity and was found to significant increase in FACE plots relative to the AMB plots. The 2 years mean FACE/AMB ratio of aboveground dry weight for all five varieties combined was 1.11 at full-heading and 1.15 at maturity, with no interaction being evident between CO2 concentration and variety. Means for aboveground dry weight increase during grain-filling period (ΔW), accumulated NSC at full-heading, and the sum of these (ΔW+NSC) for all five varieties combined were also found to be significantly greater in FACE plots than in AMB plots, again with no evident interaction between CO2 concentration and variety. ΔW+NSC in Takanari out of five varieties were found to be significantly larger in FACE plots than in AMB plots. In the case of nitrogen uptake, although those at maturity was found to increase significantly in FACE relative to AMB plots (FACE/AMB ratio 1.04) at a P < 0.05 level, the magnitudes of increase were less than those for aboveground dry weight. Significant differences in harvest index were found among varieties but not between FACE and AMB plots.
3.3. Yield and relevant traits
Yields and yield components are shown in . The mean FACE/AMB ratio for rough grain and hulled grain yields for all five varieties combined under FACE conditions was 1.18 and 1.17. Rough grain and hulled grain yields in four out of five varieties were found to be significantly greater in FACE plots than in AMB plots. Interaction between variety and CO2 concentration was also observed, with the FACE/AMB ratio for Takanari and Momiroman under FACE conditions being larger (>1.2) than those for the three other varieties. In yield components for all five varieties combined, the number of panicles and spikelets, sink capacity and sink filling rate all increased significantly in FACE plots. The number of spikelets and sink capacity of three high-yielding varieties were greater than those of standard varieties and significantly increased under FACE condition as evidenced by a significant interaction between CO2 concentration and variety (P < 0.01). As with yield, the FACE/AMB ratio in sink capacity for Momiroman and Takanari were much larger compared with the three other varieties ().
Table 3. Yield and yield components of five rice varieties grown under ambient (AMB) and FACE.
To compare the effects of sink formation and source supply potential during grain-filling as factors responsible for boosting yield under FACE conditions, we analyzed the relationship between FACE/AMB ratios for yield and sink capacity, and the relationship between FACE/AMB ratios for yield and the sum of usable carbohydrates (ΔW + NSC) (). Our results showed a very strong significant positive correlation between FACE/AMB ratios for yield and sink capacity, but no such correlation between yield and ΔW+NSC.
Figure 1. Effects of sink capacity and source accumulation on the change of yield under FACE condition.
Notes: Sink capacity (g m−2) = No. of spikelet (m−2) × 1000 grain weight (g)/1000. ΔW: Increase of aboveground dry weight at grain-filling period. NSC: non-structural carbohydrates at full-heading stage.
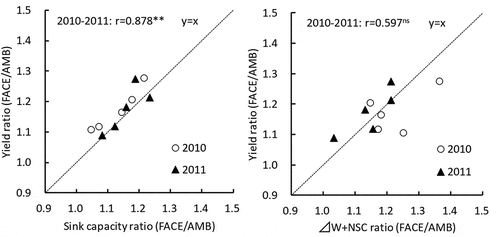
As described above, both dry matter production and sink capacity increased under FACE conditions. Whereas an interaction between CO2 concentration and variety was observed for sink capacity, no such interaction was found for dry matter production and nitrogen uptake. Varietal differences for the effects of FACE on aboveground dry weight and sink capacity are shown in . While no significant varietal differences were found in actual increase of dry matter at full-heading under FACE conditions compared with those of AMB, clear varietal differences between varieties were observed in the actual sink capacity increase under FACE conditions compared with those of AMB, with significantly higher increases in the high-yielding varieties Takanari and Momiroman than in the standard varieties Koshihikari and Nipponbare. Takanari and Momiroman also showed higher sink production efficiency per unit of dry matter production (Sink capacity/Aboveground dry weight) than did the other varieties; in both high-yielding varieties, this measure significantly increased under FACE conditions compared to AMB. On the other hand, the sink production efficiency per absorbed nitrogen (Sink capacity/Nitrogen uptake) in Takanari and Momiroman didn’t show significant difference compared with that of Koshihikari under both AMB and FACE condition.
Table 4. Increase of aboveground dry weight and sink capacity in FACE, and sink production efficiency.
4. Discussion
In this study, rice plants grown under FACE conditions had (1) shorter growth periods, (2) higher dry matter production, (3) higher numbers of spikelets (sink capacity) and panicles; and (4) higher yield. Among these traits, interactions between CO2 concentration and variety were significant for sink capacity and yield.
4.1. Changes in the growth
As with the results of previous reports (Hasegawa et al., Citation2013; Kim, Horie, Nakagawa & Wada, Citation1996a), FACE induced earlier heading and reduced the growth period by an average of 2 days. In general, a shorter growth period results in lower dry matter production. However, in this study, aboveground dry weight in FACE plots exceeded that in AMB plots at the same growth stage, compensating for the effects of shorter growth period.
As shown in , no interaction was observed between CO2 concentration and variety for aboveground dry weight under FACE conditions, and as shown in , no differences were observed between varieties for increase of aboveground dry weight in FACE. Dry matter increase was also the main growth response reported in previous FACE studies (Hasegawa et al., Citation2015; Kim, Lieffering, Miura, Kobayashi & Okada, Citation2001; Usui et al., Citation2016; Yamakawa, Saigusa, Okada & Kobayashi, Citation2004; Yang et al., Citation2009; Yoshimoto, Oue & Kobayashi, Citation2005) and was attributed to accelerated single leaf photosynthetic rate under elevated CO2 conditions. In a study comparing Koshihikari, Nipponbare, and Takanari (Ohsumi et al., Citation2007), Takanari showed high stomatal conductance, suggesting high single leaf photosynthetic rate. In our study, the fact that we did not observe significant interaction between CO2 concentration and variety in aboveground dry weight () suggests that high stomatal conductance in Takanari (Chen et al., Citation2014; Ikawa et al., Citation2017) have little impact on the response in dry matter productivity to FACE conditions. Also, ΔW and NSC at the full-heading stage, both traits that are directly involved in grain-filling, increased under FACE conditions. The increase of aboveground dry weight at full-heading was largely due to the increase in the dry weight of culm and leaf sheath (data not shown). It might contribute to the increase in NSC accumulated at full-heading ().
Meanwhile, insignificant increase in nitrogen uptake was observed at full-heading despite the fact that aboveground dry weight increased under FACE conditions. Although a significant increase in nitrogen uptake under FACE conditions was observed at maturity, the magnitude of this increase was small compared with the increase in aboveground dry weight (). This imbalance between dry weight and nitrogen uptake is consistent with the results of previous studies (Ma et al., Citation2007; Yamakawa et al., Citation2004), but it effectively means that the nitrogen content of rice plants and hulled grains are reduced. Because a decrease in the nitrogen content of rice plants and hulled grains can cause reduction in grain quality especially at the high-temperature conditions (Usui et al., Citation2016; Yang et al., Citation2007), fertilizing regimens will likely need to be reviewed to ensure stable quality under FACE conditions.
4.2. Factors behind varietal differences in yield increases under FACE conditions
Many studies have reported yield increases under FACE conditions (Kim et al., Citation2003; Yang et al., Citation2006, Citation2007; Liu et al., Citation2008; Shimono et al., Citation2009; Hasegawa et al., Citation2013, Citation2015; Usui et al., Citation2016) and have attributed this increase to an increase in the number of spikelets. This study also showed that yield increase is closely related to an increase in sink capacity compared with the increase in source amounts (ΔW and NSC) (). Although sink capacity increase generally contributes to yield increase, sink capacity increase on its own cannot ensure sufficient yield increase because sink capacity increase is accompanied by a decline in sink filling (Ohsumi et al., Citation2011). In this regard, we believe that sink filling rates did not decline in conjunction with sink capacity increase, because the usable dry matter during maturation (ΔW + NSC) mostly exceeded the increase in sink capacity under FACE conditions ( and ).
In this study, FACE/AMB ratio of hulled grain yield varied between 1.10 and 1.25 depending on variety, and Momiroman and Takanari showed a consistent increase and exceeded 1.20 (). Although Shimono et al. (Citation2009) have suggested that differences in yield may reflect differences in biomass and nitrogen uptake up to the heading stage, biomass and nitrogen uptake of Momiroman and Takanari, the two varieties that showed relatively large yield increases under FACE conditions in this study, did not differ markedly from those of the other varieties. Regarding this discrepancy in results, it should be noted that the Shimono et al. (Citation2009) study was conducted under cool conditions (under 20°C) that would facilitate the translation of dry matter production into yield increase. Studies carried out by Yoshida et al. (Citation2011) and Hasegawa et al. (Citation2013) also demonstrated a strong positive correlation between sink capacity and the magnitude of yield increase under FACE conditions, suggesting that varieties with large sink capacities are better equipped to produce increased yields under FACE conditions. Nakano et al. (Citation2017) also indicated quantitative trait loci for large sink capacity stimulate yield increase under FACE. Similarly, in a study by Liu et al. (Citation2008) in which the yield of F1 hybrids increased markedly under FACE conditions, the authors speculated that large sink capacity was involved in yield increase. The Momiroman, Takanari and Hokuriku 193 varieties used in this study have all been designated as high-yielding varieties (Yoshinaga et al., Citation2013) with high sink capacities. Although those three varieties showed a significant increase of sink production efficiency per unit of absorbed nitrogen under FACE condition (), the increase of sink capacity and yield under FACE in Hokuriku 193 was lower than those in Momiroman and Takanari (). As shown in , sink production efficiency per unit of dry matter production for Hokuriku 193 was low under both AMB and FACE conditions. And the value did not increase under FACE condition compared with AMB, although those of other two high-yielding varieties significantly increased. Thus, the lower sink production efficiency per unit of dry matter production may be the reason for this variety’s relative lower yield increase, and this can be the critical trait for the selection of favourable varieties for the increase in yield under high CO2 conditions.
4.3. Significance for future research
This study has revealed that sink production efficiency per unit of dry matter production is an important trait contributing to increased yield under FACE conditions. Since several quantitative trait loci (QTL), including GN1 and APO1 (Nakano et al., Citation2017) have been identified as contributing to sink capacity increase and have already been introgressed into multiple varieties, these QTLs should be actively utilized in the breeding of varieties suited to elevated CO2 levels. Ensuring stable high-yielding cultivation under high CO2 conditions will also require an examination of cultivation management methods, using sink production efficiency per unit of fertilizer nitrogen as an indicator to determine the most effective timing and quantity of top dressing to increase spikelet number.
Acknowledgments
Thanks are due to the support staff at NARO Institute of Crop Science.
Disclosure statement
No potential conflict of interest was reported by the authors.
Additional information
Funding
References
- Ainsworth, E. A., & Rogers, A. (2007). The response of photosynthesis and stomatal conductance to rising [CO2]: Mechanisms and environmental interactions. Plant, Cell & Environment, 30, 258–270.
- Baker, J. T. (2004). Yield responses of southern US rice cultivars to CO2 and temperature. Agricultural and Forest Meteorology, 122, 129–137.
- Chen, C. P., Sakai, H., Tokida, T., Usui, Y., Nakamura, H., & Hasegawa, T. (2014). Do the rich always become richer? Characterizing the leaf physiological response of the high-yielding rice cultivar Takanari to free-air CO2 enrichment. Plant and Cell Physiology, 55, 381–391.
- GRiSP. (2013). Rice almanac (4th, pp. 1–283.) Los Banos: International Rice Research Institute.
- Hasegawa, T., Sakai, H., Tokida, T., Shimono, H., Nakamura, H., Shimono, H., … Okada, M. (2015). Rice free-air carbon dioxide enrichment studies to improve assessment of climate change effects on rice agriculture. Functional Plant Biology, 40, 148–159.
- Hasegawa, T., Sakai, T., Tokida, T., Nakamura, H., Zhu, C., Usui, Y., … Makino, A. (2013). Rice cultivar responses to elevated CO2 at two Free-Air CO2 Enrichment (FACE) sites in Japan. Functional Plant Biology, 40, 148–159.
- Ikawa, I., Chen, C. P., Silma, M., Yoshimoto, M., Sakai, H., Tokida, T., … Hasegawa, T. (2017). Increasing canopy photosynthesis in rice can be achieved without a large increase in water use -A model based on free-air CO2 enrichment. Global Change Biology, 24, 1321–1341.
- Kim, H. Y., Horie, T., Nakagawa, H., & Wada, K. (1996a). Kohon koh CO2 noudo joken ga suitou no seiiku syuryo ni oyobosu eikyo: Dai 1 hou Hatsuiku kanbutsuseisan oyobi seityosyokeisitsu ni tuite [Effects of elevated CO2 concentration and high temperature on growth and yield of rice. I. The effect on development, dry matter production and some growth characteristics]. Nihon Sakumotsu Gakkai Kiji, 65, 634–643.
- Kim, H. Y., Horie, T., Nakagawa, H., & Wada, K. (1996b). Kohon koh CO2 noudo joken ga suitou no seiiku syuryo ni oyobosu eikyo: Dai 2 hou Syuryo oyobi syuryokouseiyouso nit suite [Effects of elevated CO2 concentration and high temperature on growth and yield of rice. II. The effect on yield and its components of Akihikari rice]. Nihon Sakumotsu Gakkai Kiji, 65, 644–651.
- Kim, H.-Y., Lieffering, M., Kobayashi, K., Okada, M., Mitchell, M. W., & Gumpertz, M. (2003). Effects of free-air CO2 enrichment and nitrogen supply on the yield of temperate paddy rice crops. Field Crops Research, 83, 261–270.
- Kim, H.-Y., Lieffering, M., Miura, S., Kobayashi, K., & Okada, M. (2001). Growth and nitrogen uptake of CO2 enriched rice under field conditions. New Phytologist, 150, 223–229.
- Kobayashi, K. (2001). FACE (kaihokei CO2 zouka) jikken [FACE (Free-Air CO2 Enrichment) experiment]. Nihon Sakumotsu Gakkai Kiji, 70, 1–16.
- Kobayasi, H., & Horie, T. (1994). Suitou no eika narabini sikoubunnka ni oyobosu seisyokuseityouki no tainaitisso no eikyo [The effect of plant nitrogen condition during reproductive stage on the differentiation of spikelets and rachis branches in rice]. Nihon Sakumotsu Gakkai Kiji, 63, 193–199.
- Liu, H., Yang, L., Wang, Y., Huang, J., Zhu, J., Yunxia, W., … Liu, G. (2008). Yield formation of CO2 enriched hybrid rice cultivar Shanyou 63 under fully open-air field conditions. Field Crops Research, 108, 93–100.
- Long, P. S., Ainsworth, E. A., Rogers, A., & Ort, R. O. (2004). Rising atmospheric carbon dioxide: Plants FACE the future. Annual Review of Plant Biology, 55, 591–628.
- Ma, H., Zhu, J., Xie, Z., Liu, G., Zeng, Q., & Han, Y. (2007). Responses of rice and winter wheat to Free-Air CO2 Enrichment (China FACE) at rice/wheat rotation system. Plant and Soil, 294, 137–146.
- Meinshausen, M., Smith, S. J., Calvin, K., Daniel, J. S., Kainuma, M. L. T., Lamarque, J., … van Vuuren, D. P. P. (2011). The RCP greenhouse gas concentrations and their extensions from 1765 to 2300. Climatic Change, 109, 213–241.
- Moya, T. B., Ziska, L. H., Namuco, O. S., & Olszyk, D. (1998). Growth dynamics and genotypic variation in tropical, field-grown paddy rice (Oryza sativa L.) in response to increasing carbon cioxide and temperature. Global Change Biology, 4, 645–656.
- Nakamura, H., Tokida, T., Yoshimoto, M., Sakai, H., Fukuoka, M., & Hasegawa, T. (2012). Performance of the enlarged Rice-FACE system using pure CO2 installed in Tsukuba, Japan. Journal of Agricultural Meteorology, 68, 15–23.
- Nakano, H., Yoshinaga, S., Takai, T., Arai-Sanoh, Y., Kondo, K., Yamamoto, T., … Kondo, M. (2017). Quantitative trait loci for large sink capacity enhance rice grain yield under free-air CO2 enrichment conditions. Scientific Reports, 7, 1827.
- Ohnishi, M., & Horie, T. (1999). Jyuryohou niyoru suitou kaku kikantyu no hikouzousei tansuikabutsu no kan-i teiryohou [A proxy analysis of nonstructural carbohydrate in rice plant by using the gravimetric method]. Nihon Sakumotsu Gakkai Kiji, 68, 126–136.
- Ohsumi, A., Hamasaki, A., Nakagawa, H., Yoshida, H., Shiraiwa, T., & Horie, T. (2007). A model explaining genotypic and ontogenetic variation of leaf photosynthetic rate in rice (Oryza sativa) based on leaf nitrogen content and stomatal conductance. Annals of Botany, 99, 263–273.
- Ohsumi, A., Takai, T., Ida, M., Yamamoto, T., Arai-Sanoh, Y., Yano, M., … Kondo, M. (2011). Evaluation of yield performance in rice near-isogenic lines with increased spikelet number. Field Crops Research, 120, 68–75.
- Ray, D. K., Mueller, N. D., West, P. C., & Foley, J. A. (2013). Yield trends are insufficient to double global crop production by 2050. PloS One, 8, e66428.
- Sakai, H., Tokida, T., Usui, Y., Nakamura, H., & Hasegawa, T. (2019). Yield responses to elevated CO2 concentration among Japanese rice cultivars released since 1882. Plant Production Science. null-null. doi: 10.1080/1343943X.2019.1626255.
- Sasaki, H., Aoki, N., Sakai, H., Hara, T., Uehara, N., Ishimaru, K., & Kobayashi, K. (2005). Effects of CO2 enrichment on the translocation and partitioning of carbon at the early grain-filling stage in rice (Oryza sativa L.). Plant Production Science, 8, 8–15.
- Shimono, H., Okada, M., Yamakawa, Y., Nakamura, H., Kobayashi, K., & Hasegawa, T. (2009). Genotypic variation in rice yield enhancement by elevated CO2 relates to growth before heading, and not to maturity group. Journal of Experimental Botany, 60, 523–532.
- Usui, Y., Sakai, H., Tokida, T., Nakamura, H., Nakagawa, H., & Hasegawa, T. (2016). Rice grain yield and quality responses to free-air CO2 enrichment combined with soil and water warming. Global Change Biology, 22, 1256–1270.
- Yamakawa, Y., Saigusa, M., Okada, M., & Kobayashi, K. (2004). Nutrient uptake by rice and soil solution composition under atmospheric CO2 enrichment. Plant and Soil, 259, 367–372.
- Yamamoto, T., Nagasaki, H., Yonemaru, J., Ebana, K., Nakajima, M., Shibaya, T., & Yano, M. (2010). Fine definition of the pedigree haplotypes of closely related rice cultivars by means of genome-wide discovery of single nucleotide polymorphisms. BMC Genomics, 11, 267.
- Yang, L., Huang, J., Yang, H., Zhu, J., Liu, H., Dong, G., … Wang, Y. (2006). The impact of Free-Air CO2 Enrichment (FACE) and N supply on yield formation of rice crops with large panicle. Field Crops Research, 98, 141–150.
- Yang, L., Liu, H., Wang, Y., Zhu, J., Huang, J., Liu, G., … Wang, Y. (2009). Impact of elevated CO2 concentration on inter-subspecific hybrid rice cultivar Liangyoupeijiu under fully open-air field conditions. Field Crops Research, 112, 7–15.
- Yang, L., Wang, Y., Dong, Y., Gu, H., Huang, H., Zhu, H., … Han, Y. (2007). The impact of Free-Air CO2 Enrichment (FACE) and nitrogen supply on grain quality of rice. Field Crops Research, 102, 128–140.
- Yoshida, H., Horie, T., Nakazono, K., Ohno, H., & Nakagawa, H. (2011). Simulation of the effects of genotype and N availability on rice growth and yield response to an elevated atmospheric CO2 concentration. Field Crops Research, 124, 433–440.
- Yoshimoto, M., Oue, H., & Kobayashi, K. (2005). Energy balance and water use efficiency of rice canopies under free-air CO2 enrichment. Agricultural and Forest Meteorology, 133, 226–246.
- Yoshinaga, S., Takai, T., Arai-Sanoh, Y., Ishimaru, T., & Kondo, M. (2013). Varietal differences in sink production and grain-filling ability in recently developed high-yielding rice (Oryza sativa L.) varieties in Japan. Field Crops Research, 150, 74–82.
- Ziska, L. H., Bunce, J. A., Shimono, H., Gealy, D. R., Baker, J. T., Newton, P. C. D., … Wilson, L. T. (2012). Food security and climate change on the potential to adapt global crop production by active selection to rising atmospheric carbon dioxide. Proceedings of the Royal Society B: Biological Sciences, 279, 4097–4105.
- Ziska, L. H., Manalo, P. A., & Ordonez, R. A. (1996). Intraspecific variation in the response of rice (Oryza sativa L.) to increased CO2 and temperature: Growth and yield response of 17 cultivars. Journal of Experimental Botany, 47, 1353–1359.