ABSTRACT
Belt-planting is a novel method for productivity of all wheat (Triticum aestivum L.) varieties, first practiced in China, in which seeds are set on a wide belt (7–12 cm in width) rather than on a conventional line swath ∼3 cm). Belt-planting allows the seeds to be uniformly distributed in the soil and increases the probability of success in establishing desirable stands, spike number per unit area and grain yield. During recent years, belt-planting has been successfully combined with other planting systems. A combination of belt-planting with precision sowing shows the integrated advantages of both technologies. Belt-planting in a strip tillage and direct drilling system has the potential to become an economically viable method for wheat productivity, facilitating the development of environmentally friendly and resource-efficient agriculture. We review the existing literature regarding the potential advantages and underlying mechanisms of belt-planting as a potential method to ensure wheat growth and productivity. We then propose future research directions related to practicing these planting methods to further improve and promote belt-planting-based technologies.
Graphical abstract
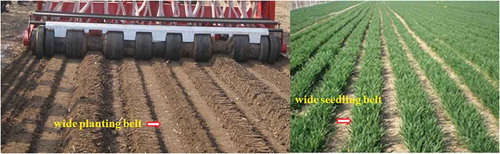
1. Introduction
In northern China, wheat (Triticum aestivum L.) is among the most important cereal crops in terms of planting area and production. However, wheat production has been facing increasing challenges with a rapidly increased food demand, resource shortages, excessive fertilizer use, soil deterioration and potential ecological disasters. In particular, water-resource deficits and insufficient precipitation are major factors during the wheat growth period limiting plant growth, development and grain yield in this region (Fan et al., Citation2019; Zhao et al., Citation2013). In pursuit of higher productivity of wheat using unreasonable means, including soil, water and nutrient management strategies, a series of environmental problems has emerged. In addition to land and freshwater degradation, rapid biodiversity loss and high greenhouse gas emissions resulting from high nutrient input and intensive tillage planting, wheat productivity has encountered a bottleneck in terms of grain yield, particularly during recent years.
In the past decades, many wheat based cropping systems are practiced in Northern China. These include multi-cropping system with two or more crops can be planted and harvested in each year, uniform planting at reduced seed rates to improve air circulation and radiation interception, high-yielding planting technology for dryland wheat by increasing both soil moisture retention capacity and higher water use efficiency (WUE), raised bed-planting system with furrow irrigation between the beds and reduced tillage systems with reduced levels of tillage for direct seeding (Wang et al., Citation2009). By applying these technologies, wheat yield has continuously increased in the earlier years; however, under these planting systems, wheat is planted using a 2–3 cm sowing swath. Under these conditions, the wheat develops a very crowded population within a limited space, therefore affecting the ability of the canopy to intercept incoming radiation, photosynthetically active radiation (PAR) capture ratio, plant growth and ultimately grain yield (Zhao et al., Citation2013). In addition, it is difficult to guarantee seeding quality in a conventional line planting system because mis-seeding frequently occurs, resulting in inferior population quality and affecting incoming radiation interception. Line planting does not appropriately coordinate the relationships between the population and individual plants as well as among the yield components, such as the spike number per unit area, grain number per spike, and 1000-grain weight (Liu et al., Citation2019).
Moreover, the present situation in wheat production is generally unsatisfactory. At present, wheat grain yield is stagnating in China, because it is facing great challenges in many aspects, including water scarcity, prevalent drought stress, increased frequencies of high temperatures, low seeding quality due to the high amount of previous crop straw retention, and low water and radiation use efficiency. Therefore, conventional planting cannot ensure a continuous increase in wheat yield. Considering that the increasing population and decreasing area of wheat croplands in China, development of a new strategy to increase the grain yield is urgently needed.
To address these problems, agronomists in China have explored many cultivation technologies. These include the development of delayed nitrogen application, precision planting, direct sowing using a reduced-tillage drill and drip irrigation and fertigation (Kong, Citation2014; Wang et al., Citation2009). These technical measures have greatly improved wheat grain yields as well as partially relieved ecological problems during the past two decades. However, in the face of these multiple challenges, building a new planting system is strongly needed to exploit potentialities of existing germplasm resources and improve the efficiency of using light, water and nutrients with the aims of both establishing a sustainable farming system and achieving desired grain yields.
2. Belt-planting
Wide belt-planting was recently originally proposed as a potential strategy to improve wheat yields. This planting mode is essentially characterized by a 7–12 cm planting swath, an inter-row spacing of 10–15 cm and better seed dispersal compared to those of the traditional planting system where all the seeds are sown in a line. The configuration of the row spacing is dependent on the wheat genotype. Field experiments with a large plot size were conducted in Zhengzhou of Henan province, where the soil type was classified as loam with a pH of 7.54. The top 20 cm of soil contains 11.8 g kg–1 organic matter, 0.96 g kg–1 total nitrogen, 43.5 mg kg–1 rapidly available phosphorous and 90.3 mg kg−1 rapidly available potassium. The results showed that 15-cm row spacing and an 8-cm planting belt under belt-planting is optimal for large spike winter wheat (Li et al., Citation2013c). The multi-spike cultivars with high tillering capacity developed reasonable canopy architecture with high radiation interception and favourable microclimate in a medium loam with a 12-cm row spacing and an 8-cm planting belt (Li et al, Citation2013a).
Under belt-planting, wheat seeds are uniformly distributed in the soil with a relatively larger seed (seedling) spacing instead of sowing the seeds in a narrow row spacing, when using the same seeding rate as the conventional planting. Compared to the conventional cultivation planting, improved air circulation, radiation interception and more optimal humidity are achieved (Li et al., Citation2013c). Wheat plants under belt-planting showed a higher water use efficiency, leaf area index (LAI), PAR capture ratio and canopy microclimate (Li et al., Citation2013a; Citation2013b; Zhao et al., Citation2013), higher activities of antioxidant enzymes, lower malonaldehyde content, delayed senescence processes, delayed degradation of leaf chlorophyll and increased duration of photosynthesis (Feng et al., Citation2015). Therefore, belt-planting increased the number of tillers and effective spikes per unit area and per plant (Chu et al., Citation2018; Fu, Citation2016). As a consequence, the highest grain yield was produced during winter wheat under this new planting pattern in northern China (Zhao et al., Citation2013). Grain yields under belt-planting were approximately 2.96%-15.94% higher than those under conventional line planting (Fu, Citation2016; You et al., Citation2016; Feng et al., Citation2015; Zhao et al., Citation2013). During recent years, field experiments using a large plot size were conducted in Tai’an (36°10ʹ19ʺ N, 117°9ʹ03ʺ E) and Yanzhou (35°420ʹN, 116°41ʹ E) of Shandong province in a wheat-maize rotation system. Both regions are in the warm temperate zone with a continental monsoonal climate with an annual precipitation of 620–700 mm, annual average temperature of 13.0–13.6°C, and annual accumulated sunshine of 2400–2550 h. The soil type of the experimental plots has been classified as loam. The results showed that an average percentage of yield increase of 19.0% was obtained at two trial sites (Chu et al., Citation2018). At the same time, belt-planting increased the nitrogen use efficiency (NUE) by 15.4% and 22.5% at the afore mentioned two trial sites, respectively, because of the enhanced nitrogen accumulation (Chu et al., Citation2018). More importantly, a field experiment using a split-plot design was conducted during two cropping seasons in Tai’an. The results showed that compared to the irrigations during the re-greening, jointing and anthesis stages for conventional planting, belt-planting in reduced irrigation regimes (60 mm of irrigation applied each during the jointing and heading stages) significantly increased the winter wheat leaf area index and the PAR interception 60 cm above the ground surface and thus increased the radiation use efficiency compared to that of conventional planting (Gao et al., Citation2019). In conclusion, belt-planting can be used as a feasible and promising cropping system. Currently, belt-planting has been broadly adopted in China, extending to approximately 4 million hectares annually for winter wheat planting mainly in North China Plain, including the Shandong, Henan, Hebei, Jiangsu and Anhui provinces. In these regions, the soil is mainly loam of aeolian origin, a type deposited by rivers over geological time. A monsoonal climate dominates the region with an uneven distribution of annual precipitation, only 30% of which falls during the wheat growth period (October to the following June). Wheat-maize rotation is the popular cropping system.
3. Belt-precision planting
3.1 Establishment of belt-precision planting based on belt-planting
Precision sowing of wheat uses well-chosen seeds, fine soil preparation, a reduced seeding rate and a uniform sowing. The seeding rate is reduced from approximately 150 to < 90 kg ha−1 but results in better air circulation and radiation interception and reduced lodging and thus a good yield benefit (Wang et al., Citation2009). Recently, a combination of wide belt-planting with precision sowing (belt-precision planting) has been established and applied in the wheat planting area of the Yellow and Huai River Valleys, aiming to improve winter wheat productivity. The planter for the belt-precision planting is fitted with a seed scatter, which is a seed metering device with precisely configured cells for single-seed precision sowing. To date, this planting system has been extensively adopted for wheat production on the North China Plain and can be appropriately used in fields with a yield potential that is at a medium (5.25–7.50 t ha−1) to high (≥ 7.50 t ha−1) level (Liu et al., Citation2019).
3.2 Effects of belt-precision planting on wheat agronomical traits
As expected, belt-precision planting ensures uniform stand establishment, better plant growth () and strong tillering and therefore significantly increases the number of tillers per plant and number of secondary roots; both were higher compared to those in conventional-cultivation planting (Fan et al., Citation2014; Li et al., Citation2015). Belt-precision planting is effective for optimizing the vertical PAR distribution in the wheat canopy and maintaining a higher leaf area index during early or late growth stages, and this effect is much more significant under no straw mulching (Liu et al., Citation2017; Zhao et al., Citation2013).
Figure 1. Wheat plants grown in a belt-precision planting system showing strong and uniformly distributed seedlings (a) and the planting configuration and wide seedling belt (b).
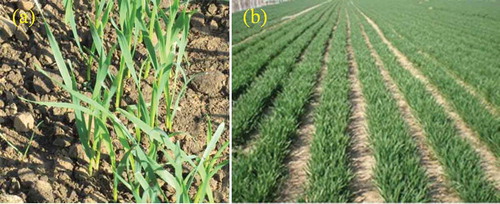
Belt-precision planting promotes root nutrient absorption because of the root vigour, resulting in a 10.6%-6.4% higher NUE compared to that of conventional technologies. In Tai’an on the North China Plain, an irrigation of 60 mm each during the jointing and heading stages improved the grain protein content, gluten index and wet gluten content as well as the wheat grain yield during belt-precision planting (Bian et al., Citation2016; Han et al., Citation2013), resulting in a significantly WUE (Lang et al., Citation2015; Li et al., Citation2015; Zhao et al., Citation2013). More recently, a two-year trial was conducted in Tai’an on the North China Plain with a mean relative humidity of 50% and total rainfall of 157 mm during the winter wheat growing seasons. The results showed that irrigation during the heading and flowering stages under belt-planting significantly increased the chlorophyll content index and maximum photochemical efficiency in the flag leaves; the higher values of these indexes were even achieved with one irrigation (60 mm) 10 days after the jointing stage, resulting in a significantly increased spike number, grain number per spike, grain yield and WUE (Fan et al., Citation2019). The higher WUE was achieved mainly because of the higher moisture and favourable topsoil temperature during the seedling establishment period, higher root vigour, increased ability to counteract drought stress (Fan et al., Citation2014), higher conversion from ineffective water consumption (i.e., soil evaporation) to effective water consumption (i.e., leaf transpiration) (Bian et al., Citation2016) and a notable increase in spike number (Li et al., Citation2015). Therefore, belt-precision planting may be a potentially suitable water-saving planting method for wheat and has distinctive advantages in semi-humid regions with decreased irrigation frequency (Bian et al., Citation2016; Fan et al., Citation2019).
An increasing body of evidence from a large number of experiments using reasonably large plot sizes has demonstrated that belt-precision planting improves plant development and population establishment, resulting in a reasonable canopy architecture and subsequently aboveground dry matter accumulation, higher grain weight per spike and a yield increase of 4.09%-14.23% (Bian et al., Citation2016; Fan et al., Citation2014; Han et al., Citation2013; Li et al., Citation2015, Citation2013c; Liu et al., Citation2017; Zhao et al., Citation2013). The yield increase is primarily attributed to the higher spike number per unit area, reasonable components, enhanced physiological activities of grains and delayed senescence processes during grain filling (Feng et al., Citation2015).
3.3 Contributions of yield components to grain yield in belt-precision planting
Based on the data collected from the literature for belt-precision planting in China, the correlation coefficients, direct and indirect effects and percent contributions of the yield components were evaluated using DPS Statistical Software (Tang et al., Citation2013). The results show that the yield component area positively correlated with the grain yield (). The correlation coefficients between the number of spikes per unit area and grain yield of wheat in belt-precision planting are greater than those in conventional line planting; in contrast, the correlation coefficients between the grain yield and grain number spike−1 in belt-precision planting are less than those in conventional line planting (). As a result, a high correlation coefficient between grain yield and number of spikes per unit area is observed under belt-precision planting, whereas a high correlation coefficient between grain yield per unit area and 1000-kernel weight is observed in conventional planting. Indeed, results from a field experiment (each plot was 5 m × 5 m in size with three replicates) showed that belt-precision planting could compensate for the reduction in winter wheat grain yield under straw mulching conditions on the North China Plain, primarily by significantly increasing the spike number (Liu et al., Citation2017). The higher number of spikes per unit area is substantially a result of the higher fertile percentage because the seeding rate and seedling number were the same between the two planting patterns during these experiments. No doubt, a higher fertile percentage is attributed to a much more efficient resource per seedling ratio and stronger wheat seedlings.
Figure 2. Relationships between the yield and yield components of wheat under conventional (a, b, and c) and belt-precision (d, e, and f) planting systems. Under the belt-precision planting system, the highest correlation coefficient is observed between the grain yield and the number of spikes per unit area, whereas in conventional planting, the highest correlation coefficient is observed between the grain yield per unit area and the 1000-kernel weight. Each point is relative to the mean of the data from each planting pattern.
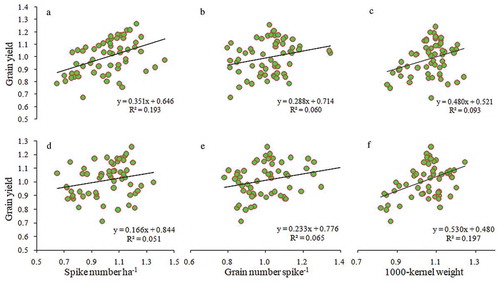
Path coefficient analysis is commonly used to partition the relative contribution of yield components via standardized partial regression coefficients and provides an effective means by which to distinguish direct and indirect sources of correlation. The direct and indirect effects of all of the components observed on grain yield are presented in . Path coefficients indicate that the total effect of the number of spikes per unit area on grain yield was greater in belt-precision planting than that in conventional line planting, largely because of the lesser negative indirect effects via the grain number per spike and 1000-grain weight (). These results indicate that the yield components of wheat might be better reciprocally coordinated in belt-precision planting and that the relationship between the population and individual plants is better coordinated.
Table 1. Direct and indirect effects of yield components on wheat grain yield in conventional line and belt-precision planting systems as indicated by path coefficients. Total effects are the sum of the direct and indirect effects.
4. Belt-planting in a strip tillage system (BPST)
The wheat-maize rotation planting system is always characterized by a large amount of maize straw retention such as in the northern China cropping zone where land preparation and sowing are expensive and time-consuming for wheat cultivation. Numerous procedures are needed for wheat planting, including maize straw smashing, tillage, soil raking and compaction. Using the newly developed wheat planter types, a strip (10–15 cm) is tilled ensuring a 7–12– cm seedling belt (). When tilling, the residue is simultaneously cut again and mixed with the soil. The ground (10–15 cm) between the tilled strips is left undisturbed. This type of planter can accomplish subsoiling (using four vibration subsoilers with a working depth of approximately 35 cm), strip rotary tillage (15–18– cm deep), base fertilizer application, precision sowing, soil covering and compaction during the same run and thereby reduce the number of field operations required (Hu et al., Citation2016; Wang et al., Citation2018). The BPST system plays an important role in reducing the drudgery of farm workers, minimizing operational time and saving production costs compared to the conventional seeding method.
Figure 3. Belt-planting in a strip tillage system showing direct wheat sowing immediately after maize harvest using a strip rotary drill.
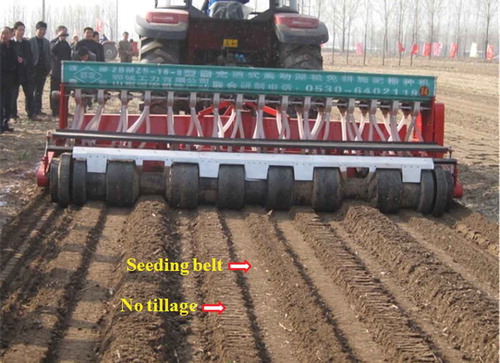
As an essential component of the BPST system, subsoiling is beneficial in enhancing soil water availability and promoting root growth and thus increases the absorption capacity of soil water, dry matter accumulation and maintenance of a high photosynthetic ability (Chu et al., Citation2016; Wang et al., Citation2015; Zheng et al., Citation2011, Citation2014). Ultimately, subsoiling increased the spike number, 1000-grain weight and grain yield of winter wheat (Sang et al., Citation2016).
Compaction can produce good seed-soil contact and promote a better plant stand. This procedure is particularly important in annual double-cropping regions (winter wheat and summer maize) with large volumes of residue remaining on the soil surface after maize harvesting. Furthermore, compaction can effectively aid in improving the carbon sequestration, water retention and biological activity of the soil and thereby aid in solubilizing soil nutrients and reducing the need for chemical and irrigation inputs (Sang et al., Citation2016; Zhang et al., Citation2009). The photosynthetic rate of the flag leaves, 1000-grain weight, grain yield and WUE also increased following moderate topsoil compaction in Yanzhou of Shandong province (Sang et al., Citation2016).
This planting system can also be used in semiarid regions such as in Shanxi province where the area of drylands accounts for 76.92% of the total arable land. The field experiments that were performed in drylands of this province have demonstrated the advantages of BPST over those of conventional planting in terms of plant agronomical traits, grain yield and flour processing quality including dough stability time and flour extension area (), largely due to the better seeding quality and high WUE under this technology.
Table 2. Comparison of grain yield, yield components and flour processing quality of wheat grown in conventional line and BPST planting systems. Data were collected from experiments using a large plot size (667 m2 for each treatment) in dry regions of Shanxi province (Zhang et al., Citation2018).
5. Concluding remarks and perspectives
A large body of evidence shows that belt-planting is implemented as a strategy to improve winter wheat yield compared to that of conventional-cultivation winter wheat planting. During the early growth stages, the uniform distribution and wide spacing between plants can minimize competition for nutrients and water resources compared to conventional line planting, which in turn results in more strong tillers and a higher percentage of effective tillers and thus a higher spike number per unit area and more rational canopy architecture. During the middle and late growth stages, the wide plant spatial distribution increases the amount of solar radiation intercepted by leaves, improves light conditions within the canopy, delays leaf senescence, increases leaf photosynthesis and maintains a longer duration of grain filling. In conclusion, wheat plants under belt-planting show significant potential for increasing yields. This planting method should be extended throughout the North China Plain. Currently, belt-planting, particularly belt-precision planting, is mainly based on extensive soil tillage in China. However, frequent soil tillage leads to degradation of soil properties, and soil organic matter and soil biodiversity are reduced (Wang et al., Citation2009). Therefore, continued exploration of agricultural machinery and agronomical technologies to perfectly integrate belt-planting with only seedling belt tillage is encouraged. This combination will achieve direct seeding and retention of adequate crop residue on the soil surface and thus accomplish the principle of conservation agriculture, particularly with alternate tillage and no-tillage during different years.
A planting system that combines multiple procedures, including belt-planting, precision sowing and reduced tillage, should be developed to produce greater yields and better quality with less input. Even more desirable is this combination further integrated with a micro-irrigation and fertigation system to create a potentially perfect planting system of wheat that could precisely control the irrigation and fertilizer amount, maximize the synergy between these two farm inputs and improve fertilizer and WUE. Most importantly, this planting system not only would be economically feasible but also environmentally friendly and sustainable.
Disclosure statement
No potential conflict of interest was reported by the authors.
Additional information
Funding
References
- Bian, C., Ma, C., Liu, X., Gao, C., Liu, Q., Yan, Z., … Li, Q. (2016). Responses of winter wheat yield and water use efficiency to irrigation frequency and planting pattern. PLoS One, 11(5), e0154673.
- Chu, J., Zhu, W., Yin, L., Shi, Y., Deng, S., Zhang, L., … Dai, X. (2018). Effects of wide-range planting on the yield and nitrogen use efficiency of winter wheat cultivar Tainong 18. Chinese Journal of Applied Ecology, 29, 2517–2524.
- Chu, P., Zhang, Y., Yu, Z., Guo, Z., & Shi, Y. (2016). Winter wheat grain yield, water use, biomass accumulation and remobilisation under tillage in the North China Plain. Field Crops Research, 193, 43–53.
- Fan, Q., Meng, F., Li, L., Li, B., Wang, J., Zhou, Z., & Kong, L. (2014). Broad precise sowing and high yield cultivation techniques test of wheat in Tancheng county. Journal of Anhui Agricultural Science, 42(32), 11268–11269. (in Chinese, with English abstract). .
- Fan, Y., Liu, J., Zhao, J., Ma, Y., & Li, Q. (2019). Effects of delayed irrigation during the jointing stage on the photosynthetic characteristics and yield of winter wheat under different planting patterns. Agricultural Water Management, 221, 371–376.
- Feng, W., Li, S., Wang, Y., Kang, G., Duang, J., & Guo, T. (2015). Effects of spacing intervals on the ageing process and grain yield in winter wheat under wide bed planting methods. Acta Ecologica Sinica, 35, 2686–2694. (in Chinese, with English abstract). .
- Fu, H. (2016). Introduction, demonstration and trials of wheat belt plating in Ning county. Gansu Agriculture, 12, 23–24. (in Chinese, with English abstract).
- Gao, C., Ren, Y., & Li, Q. (2019). Radiation use efficiency of winter wheat in different planting patterns and irrigation frequencies in the North China Plain. Archives of Agronomy and Soil Science, 65, 1010–1021.
- Han, H., Zhao, D., Shen, J., Lang, K., Liu, Q., & Li, Q. (2013). Effect of irrigation amount and stage on yield and quality of winter wheat under wide-precision planting pattern. Transactions of the CSAE, 29(14), 109–114. (in Chinese with English abstract).
- Hu, H., Li, H., Li, C., Wang, Q., He, J., Li, W., & Zhang, X. (2016). Design and experiment of broad width and precision minimal tillage wheat planter in rice stubble field. Transactions of the CSAE, 32(4), 24–32. (in Chinese with English abstract). .
- Kong, L. A. (2014). Maize residues, soil quality, and wheat growth in China. A review. Agronomy for Sustainable Development, 34, 405–416.
- Lang, K., Liu, Q. R., Bian, C. Y., Liu, X. H., & Li, Q. Q. (2015). Effect of delayed irrigation at jointing stage on canopy temperature and leaf water use efficiency of winter wheat in wide-precision planting pattern. Acta Ecologica Sinica, 35, 5262–5268. (in Chinese with English abstract). .
- Li, Q. Q., Bian, C. Y., Liu, X. H., Ma, C. J., & Liu, Q. R. (2015). Winter wheat grain yield and water use efficiency in wide-precision planting pattern under deficit irrigation in North China Plain. Agricultural Water Management, 153, 71–76.
- Li, S. Y., Feng, W., Wang, Y. H., Wang, C. Y., & Guo, T. C. (2013a). Effects of spacing interval of wide bed planting on canopy characteristics and yield in winter wheat. Chinese Journal of Plant Ecology, 37(8), 758–767. (in Chinese with English abstract). .
- Li, S. Y., Hou, C., Feng, W., Wang, Y. H., Wang, C. Y., & Guo, T. C. (2013b). Effects of wide bed planting on canopy microclimate and water use efficiency in winter wheat. Acta Agriculturae Boreali-occidentalis Sinica, 22, 80–84. (in Chinese with English abstract).
- Li, S. Y., Wang, Y., Feng, W., Hou, C., Zhu, Y., & Guo, T. (2013c). Effects of wide belt planting on canopy characteristics and yield winter wheat with large-spike. Journal of Triticeae Crops, 33, 320–324. (in Chinese with English abstract).
- Liu, X., Ren, Y., Gao, C., Yan, Z., & Li, Q. (2017). Compensation effect of winter wheat grain yield reduction under straw mulching in wide-precision planting in the North China Plain. Scientific Reports, 7, 213.
- Liu, X., Wang, W. X., Lin, X., Gu, S. B., & Wang, D. (2019). The effects of intraspecific competition and light transmission within the canopy on wheat yield in a wide-precision planting pattern. Journal of Integrative Agriculture, 18, 2–10.
- Sang, X., Wang, D., & Lin, X. (2016). Effects of tillage practices on water consumption characteristics and grain yield of winter wheat under different soil moisture conditions. Soil and Tillage Research, 163, 185–194.
- Tang, Q. Y, & Zhang, C. X. (2013). Data Processing System (DPS) software with experimental design, statistical analysis and data mining developed for use in entomological research. Insect Science, 20(2), 254–260.
- Wang, F., He, Z., Sayre, K., Li, S., Si, J., Feng, B., & Kong, L. A. (2009). Wheat cropping systems and technologies in China. Field Crops Research, 111, 181–188.
- Wang, H., Guo, Z., Shi, Y., Zhang, Y., & Yu, Z. (2015). Impact of tillage practices on nitrogen accumulation and translocation in wheat and soil nitrate-nitrogen leaching in drylands. Soil and Tillage Research, 153, 20–27.
- Wang, Z., Zhang, Y., Diao, P., & Diao, H. (2018). Design and experiment on wheat precision no-tillage planter with subsoiling layered fertilization and wide seedling. Journal of Agricultural Mechanization Research, 6, 102–106.
- You, Y., Liu, G., Zhou, D., Zhang, H., Wen, J., & Hu, J. (2016). Effects of wide precision seeding on main characters and yield of spring wheat in the yellow river irrigation area of central Gansu province. Gansu Agricultural Science and Technology, 1, 41–43. (in Chinese with English abstract).
- Zhang, R., Li, S., Wang, R., Zhang, Y., Zhang, Z., & Huang, X. (2018). Effect of wide strip rotary on yield and quality of wheat. Journal of Northwest A&F University, 46, 79–85.
- Zhang, S., Lövdahl, L., Grip, H., Tong, Y., Yang, X., & Wang, Q. (2009). Effects of mulching and catch cropping on soil temperature, soil moisture and wheat yield on the Loess Plateau of China. Soil and Tillage Research, 102, 78–86.
- Zhao, D., Shen, J., Long, K., Liu, Q., & Li, Q. (2013). Effects of irrigation and wide-precision planting on water use, radiation interception, and grain yield of winter wheat in the North China Plain. Agricultural Water Management, 118, 87–92.
- Zheng, C., Yu, Z., Shi, Y., Cui, S., Wang, D., Zhang, Y., & Zhao, J. (2014). Effects of tillage practices on water consumption, water use efficiency and grain yield in wheat field. Journal of Integrative Agriculture, 13(11), 2378–2388.
- Zheng, C. Y., Cui, S. M., Wang, D., Yu, Z. W., Zhang, Y., & Shi, Y. (2011). Effects of soil tillage practice on dry matter production and water use efficiency in wheat. Acta Agronomica Sinica, 37, 1432–1440.