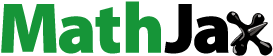
ABSTRACT
Many nitrogen-efficient (N-efficient) maize cultivars have been bred to meet increased food demand and allay urgent environmental concerns, but the effects of these cultivars on increasing N accumulation and reducing N loss in the maize system have not been reported. A two-year field pot experiment was conducted in Southwest China to evaluate the advantages of an N-efficient maize cultivar on N accumulation and N loss in 2014 and 2015. The results showed that the N-efficient maize cultivar ZH 311 had higher N uptake and accumulation ability than the N-inefficient maize cultivar XY 508, especially in the middle and late growth stages. ZH 311 maintained higher residual soil inorganic N in the appropriate growth stage, promoting soil N supply capability and enhancing N uptake while decreasing residual soil inorganic N at harvest and reducing the risk of N leaching. Higher nitrogen accumulation and residual soil inorganic N contributed to lower apparent N loss and apparent N loss efficiency for the N-efficient cultivar than for the N-inefficient cultivar. Moreover, N loss during the maize growth season mainly occurred before silking, and the N-efficient maize cultivar could effectively reduce N loss before silking because of its earlier N uptake ability. Overall, the selection of N-efficient maize cultivars is not only an important measure to enhance N use efficiency but also an important means to reduce the loss of N and environmental pollution, especially at low and medium N levels.
1 Introduction
Maize (Zea mays L.) is one of the most important cereal crops in the world, and it requires the largest portion of the grain crops planting areas in China (Jiang, Citation2013; Wang et al., Citation2014). Given the greatly decreasing farmland area and the limited potential of increasing the food planting area, the improvement of crop yield per unit area is an effective method to guarantee global food safety (Yan et al., Citation2016).
Nitrogen (N) is one of the most important limiting elements in natural ecosystems and in most agricultural systems (Chen et al., Citation2013), and N is closely correlated with agronomic characters (Zhang et al., Citation2015), leaf photosynthesis (Feng et al., Citation2015; Széles, Megyes & Nagy, Citation2012), biomass production and distribution (Ning, Zheng, Han, Jiang & Li, Citation2012; Zhang et al., Citation2014), and the panicle traits of crops (Jian et al., Citation2014). It has been proven that N fertilization is the most feasible method to improve maize yield per unit area (Chen et al., Citation2015; Zhang et al., Citation2016). However, to obtain high yields, farmers have become accustomed to applying excessive amounts of synthetic N fertilizers, which has resulted in China becoming the largest consumer of N fertilizer in the world; China accounts for 9% of the world’s arable land and consumes more than 30% of the world’s N fertilizer (Chen et al., Citation2014; Shi, Zhang & Dong, Citation2013). Unreasonable N fertilization and excessive N fertilizer application might result not only in crop lodging, ripening delay, and poor yield and quality (Li et al., Citation2015; Ahmed, Humphreys, Salim & Chauhan, Citation2016) but also in low N use efficiency (Stamatiadis, Tsadilas, Samaras, Schepers & Eskridge, Citation2016), massive N loss (Qiao, Yang, Yan, Xue & Zhao, Citation2013), and environmental pollution that includes enhanced greenhouse gas emissions, surface-water eutrophication, and soil acidification and changes in biodiversity (Hamilton, Trimmer, Bradley & Pinay, Citation2016). In recent years, many methods have been proposed to reduce N loss, including N application models, site-specific N management plans, split applications to match the N needs of crops, and the development of high-efficiency N fertilizers (Hofmeier et al., Citation2015; Montemurro, Maiorana, Ferri & Convertini, Citation2006; Qiao et al., Citation2014; Sui et al., Citation2013). However, some of these approaches are difficult for farmers to implement due to higher labor and production costs.
Genetic variations in N uptake and grain yield per unit of N application have also been studied in different crops such as rice, wheat, oilseed, maize, and barley (Abdel-Ghani et al., Citation2013; Li et al., Citation2014; Singh, Agrawal, Agrawal, Singh & Singh, Citation2015; Ulas, Behrens, Wiesler, Horst & Erley, Citation2015). Technological developments in maize breeding have allowed breeders to develop N-efficient maize cultivars because they produce higher grain yields than N-inefficient cultivars with the same rate of N application (Chun, Mi, Li, Chen & Zhang, Citation2005; Coque & Gallais, Citation2010; Moll, Kamprath & Jackson, Citation1982; Pathan et al., Citation2015). The characteristics of N-efficient maize cultivars have been widely reported in recent years. Mu et al. (Citation2015) found that the root length density, root surface area, and dry weight at the silking stage were 9.6–19.5% higher in an N-efficient cultivar than in an N-inefficient cultivar, while the root distribution pattern in the soil profile showed no significant differences between the two cultivars. Pathan et al. (Citation2015) suggested that an N-efficient maize line induced larger changes in soil chemical properties and in the enzyme activity, soil microbial biomass and community structure than the N-inefficient maize line. Ma et al. (Citation2014) demonstrated that N-efficient maize cultivars have higher root numbers and root density in deeper soil layers and more effective root distribution than N-inefficient maize cultivars, which lead to stronger nitrogen uptake ability. It is proposed that these properties enable N-efficient maize cultivars to alleviate the negative effects of using urea or ammonium-based N fertilizers on the environment. However, it is unknown what differences these two types of maize cultivar have on apparent N balance, N loss period and N loss rate.
To date, no research on the differences in N efficiency responses to N application between different maize cultivars has been reported in Southwest China. We preliminarily screened the N-efficient maize cultivar ZH 311 and N-inefficient cultivar XY 508 from the maize cultivars currently in production and application in Southwest China using indoor and outdoor experiments in the early period (Li et al., Citation2014; Li et al., Citation2015; Li et al., Citation2016) and then conducted a two-year field pot experiment near Jianyang City in the Mid-Sichuan Hilly Region, a main maize-producing region in Southwest China. The objectives were to investigate whether N-efficient maize cultivars in the Mid-Sichuan Hilly Region can play an effective role in alleviating N losses from maize fields to the ecosystem and to identify the different effects of these two types of maize cultivars on apparent N balance, N loss period and N loss rate. The results of this study can provide useful information for understanding how N-efficient maize cultivars alleviate N loss in the fields and how to guide local farmers to implement the best N management practices according to the N loss characteristics of N-efficient and N-inefficient maize cultivars.
2 Materials and methods
2.1 Experimental materials
The N-efficient maize cultivar ‘ZH 311’ and the N-inefficient maize cultivar ‘XY 508’, screened in previous studies (Li et al. Citation2016; Li et al. Citation2014), were used as experimental materials in the present study. Both cultivars are major cultivars grown in Sichuan, and the two cultivars have similar growth periods of approximately 120 d. During the experimental period, the plant height and grain yield of the two cultivars were significantly different, and the data are presented in Figures S1 and S2.
2.2 Experimental design
A field pot experiment was conducted in 2014–2015 using soil collected from the top 20 cm of a field with long-term maize production in Jianyang, Sichuan Provence, China. The soil was air-dried and passed through a 0.5-cm mesh; visible roots and organic residue were removed manually, and the soil was mixed thoroughly before use in the pot experiment. The soil properties of the tested soil are shown in prior to the start of the pot experiment. The daily temperature and precipitation in Jianyang are shown in .
Table 1. Soil properties of the tested soil in 2014–2015.
The pot experiment included two maize hybrids, ZH 311 (which is an N-efficient maize cultivar provided by Sichuan Nongda Zhenghong Seed Co., Ltd.) and XY 508 (which is an N-inefficient maize cultivar provided by Pioneer Technology Co. Ltd.), and three rates of N application in 2014 (0, 150, and 300 kg N·ha−1) and four rates in 2015 (0, 150, 300, and 450 kg N·ha−1). A randomized block design with three replicates was used, and each replicate included 20 plastic pots (both diameter and height were 30 cm); a total of 360 pots in 2014 and 480 pots in 2015 were used. Each plot was filled with 20 kg soil and buried in a 30 cm deep ditch and arranged in a wide-narrow row (1.4 m + 0.4 m) scheme with a plant density of 52,500 plants·ha−1. Seedlings with two fully expanded leaves were transplanted into plastic pots (two plants per pot). In all treatments, 72 kg·ha−1 of P2O5 as a single superphosphate (0–12.0-0) and 90 kg·ha−1 of K2O as potassium chloride (0-0-63.1) were applied at the basal stage, whereas N fertilizer was equally split-applied (5:5) as urea (46.3-0-0) at the basal and large bell stages. Chemical products were used to control insects, diseases, and weeds to avoid yield loss.
2.3 Sampling and measurements
At the jointing stage (JS), large bell stage (LBS), silking stage (SS), filling stage (FS, 20 d after silking), and maturity stage (MS), two neighboring pots (4 plants) were sampled from each replicate, and the samples were divided into root, leaf lamina, stem plus sheath, and panicle. The fresh samples were oven-dried at 105°C for 60 min and then at 80°C until a constant weight was reached to determine the dry matter accumulation of the plants. The dried organ samples were milled and sifted through a 0.25-mm screen, and their Kjeldahl-N was determined.
For soil inorganic N (amount of inorganic N accumulation) analysis, soil samples were obtained from each plot before transplanting and at JS, LBS, SS, FS, and MS. According to the method of (Shi et al., Citation2013), fresh soil samples from the same replicate were mixed, extracted immediately using 2 mol·L−1 potassium chloride solution (soil solution ratio, 1:5), shaken at 25°C for 2 h, centrifuged at 3500 r min−1 for 15 min, and then filtered. The content of NH4-N and NO3-N were analyzed by indo-phenol blue colourimetric and ultraviolet wavelength, respectively. Taken 200 nm and 228 nm as the measurement and reference wavelengths to determine NO3-N to eliminate the interference of NO2-N (Deng, Wang, Ren & Mei, Citation2014; Yan et al., Citation2016). Soil water content was determined by oven-drying at 105°C at each sampling stage.
2.4 Calculation methods (Deng et al., Citation2014; Yan et al., Citation2016)
The amount of inorganic N accumulation (N min) of soil was calculated as follows:
The apparent N mineralization (ANM) during the maize growing season was calculated as the difference between N output (crop N accumulation + residual soil N min) and N input (initial soil N min) in the plots with no N treatment. The apparent N loss (N loss) was calculated as follows:
The apparent N loss in different growth stages and apparent N loss rate were calculated as follows:
2.5 Statistical analysis
The analyses were carried out on three replications. Statistical analysis was completed using the SPSS 18.0 software package for Windows. One-way analysis of variance (ANOVA) was used to determine whether the N treatments and cultivars had significant effects on N accumulation and residual soil N min at the 0.01 or 0.05 levels. The least significant difference (LSD) test at the 0.05 level was used to determine the differences between the means of each treatment.
3 Results
3.1 Nitrogen accumulation of maize
N fertilizer treatment, cultivar and their interaction significantly (p < 0.01) affected the N accumulation of maize in the different growth stages (). The N accumulation of the N-efficient cultivar ZH 311 was remarkably higher than that of the N-inefficient cultivar XY 508.ZH 311 was higher by 3.17, 7.10, 12.25, 14.98, and 20.57 kg·ha−1 in 2014, and by 2.38, 5.91, 8.51, 13.95, and 17.20 kg·ha−1 in 2015 at JS, LBS, SS, FS, and MS, respectively. Thus, with maize growth, the differences between the two maize cultivars on N accumulation increased. N fertilizer drastically increased maize N accumulation in the different stages, while the N effects of the two maize cultivars were significantly different. The N-efficient maize cultivar ZH 311 had higher N uptake and accumulation ability than the N-inefficient maize cultivar XY 508, especially in the middle and late growth stages. The N accumulations of both ZH 311 and XY 508 increased with increasing N level in each growth period within the range of treatments (0–300 kg N·ha−1) in 2014. However, in 2015, the N accumulation of the N-inefficient maize cultivar XY 508 increased with increasing N fertilizer level, and the highest value was observed at 450 kg N·ha−1, while the N accumulation of the N-efficient maize cultivar ZH 311 initially increased and then decreased with increasing N level, and the highest value was observed at 300 kg N·ha−1.
Table 2. Nitrogen accumulation of maize in different growth stages (kg·ha−1).
3.2 Residual soil inorganic N
The difference in residual soil inorganic N between ZH 311 and XY 508 was significant (). The residual soil inorganic N of ZH 311 was observably (p < 0.01) higher than that of the N-inefficient cultivar XY 508 (except for LBS in 2014) by 6.03%, 6.94%, 6.98%, and 8.88% in 2014 and by 7.95%, 16.82%, 6.67%, 10.97%, and 10.59% in 2015 at JS, LBS, SS, FS, and MS, respectively. N fertilizer markedly increased the residual soil inorganic N of both cultivars, and the residual soil inorganic N of both cultivars increased with increasing N rate in each growth stage. The average of 5 growth stages over two years, the regression of the difference in residual soil inorganic N between ZH 311 and XY 508 (y) and the N application rate (x) was y = −0.00003418x2 + 0.01931x+0.3668 (R2 = 0.9971**). The differences in residual soil inorganic N between ZH 311 and XY 508 first increased and then decreased with increasing N rate. The largest difference in residual soil inorganic N between ZH 311 and XY 508 was 3.09 kg·ha−1 at 282.5 kg N ha−1. There was, therefore, a significant difference in residual soil inorganic N between the N-efficient cultivar ZH 311 and the N-inefficient cultivar XY 508.
Table 3. Residual soil inorganic N in different growth stages (kg·ha−1).
3.3 Apparent N balance
Due to low total N output (low crop N uptake and residual N min after harvest), tgghe apparent N loss and apparent N loss rate during the entire growing season of the N-inefficient cultivar XY 508 was significantly (p < 0.01) higher than that of the N-efficient cultivar ZH 311 (). Increasing N fertilizer not only increased N accumulation and residual N min after harvest but also drastically increased apparent N loss and apparent N loss efficiency at the same time. The two cultivars performed consistently in both years, and the linear regression equations for the apparent N loss (y) and N fertilizer level (x) of ZH 311 and XY 508 were yZH 311 = −22.6630 + 0.73338x (R2 = 0.9671*) and yXY 508 = −14.147 + 0.7394x (R2 = 0.9881**) in 2015, respectively. With every 100 kg increase in N fertilizer, the apparent N loss increased 73.64 kg, and the ratio of apparent N loss and N supply (up to 43.15–73.67) increased with increasing N fertilizer. Regarding the cultivars, the ratio of apparent N loss and N supply of the N-inefficient maize cultivar XY 508 was clearly higher than that of the N-efficient cultivar ZH 311, and the results for the same cultivars at the same N levels in both years were largely the same.
Table 4. Apparent N balance in soil-maize system in 2014–2015.
3.4 N loss period
N fertilizer treatment, cultivar and their interaction significantly (p < 0.05) affected apparent N loss in different periods of maize growth (). The apparent N loss of the N-inefficient cultivar XY 508 was higher than that of the N-efficient cultivar ZH 311, and that of XY 508 was higher by 13.05%, 24.04%, 5.53%, 15.35%, and 61.40% in 2014 and by 8.27%, 4.72%, 4.03%, 19.45%, and 26.91% in 2015 in sowing stage-JS, JS-LBS, LBS-SS, SS-FS, and FS-MS, respectively. The differences between the two maize cultivars in apparent N loss were lowest at at JS-LBS in both years, and the highest differences were at FS-MS in both years. The differences in apparent N loss between ZH 311 and XY 508 mainly occurred at the later growth stages. Furthermore, the increased N rate drastically (p < 0.01) increased apparent N loss in different periods of maize growth. The apparent N loss of ZH 311 increased with every 100 kg increase in N fertilizer, by 0.21, 0.02, 0.22, 0.05, and 0.04 kg·ha−1 in 2014 and by 0.20, 0.09, 0.24, 0.08, and 0.01 kg·ha−1 in 2015, while that of XY 508 was 0.23, 0.03, 0.23, 0.06, and 0.06 kg·ha−1 in 2014 and was 0.22, 0.10 0.25, 0.10, and 0.02 in 2015 at sowing-JS, JS-LBS, LBS-SS, SS-FS, and FS-MS, respectively. Therefore, the increase in apparent N loss of XY 508 with every 100 kg increase in N fertilizer was higher than that of ZH 311 in all the growth periods. That is, with the increased N rate, the pollution risk of planting XY 508 was much higher than that of planting ZH 311.
Table 5. Apparent N loss in different periods (kg·ha−1).
3.5 N loss rate
The highest apparent N loss rate was observed at LBS-SS in both years because of its high temperature and soil mineral N, while the lowest values were at JS-LBS and FS-MS in 2014 and 2015, respectively (). Furthermore, the apparent N loss rate of the N-inefficient cultivar XY 508 in different periods was higher than that of the N-efficient cultivar ZH 311, and that of XY 508 was higher by 13.05% at sowing stage-JS, 24.04% at JS-LBS, 5.53% at LBS-SS, 15.34% at SS-FS, and 61.41% at FS-MS in 2014, and by 8.27%, 4.72%, 4.03%, 19.45%, and 26.91% in 2015, respectively. The differences between the two maize cultivars in apparent N loss rate at FS-MS were higher than those at the other periods. With increasing N fertilizer, the apparent N loss rate in different periods significantly (p < 0.01) increased, while the increases in ZH 311 and XY 508 were observably different. With every 100 kg increase in N fertilizer, the average over the 5 growth stages in the apparent N loss rate of ZH 311 increased by 4.97 g·ha−1·d−1 in 2014 and by 6.08 g·ha−1·d−1 in 2015. That of XY 508 increased by 5.62 g·ha−1·d−1 in 2014 and by 6.53 g·ha−1·d−1 in 2015. Moreover, every 100 kg increase in N fertilizer increased the apparent N loss of XY 508 more than that of ZH 311, by 13.08% in 2014 and 7.40% in 2015, and the largest difference between ZH 311 and XY 508 was at FS-MS.
Table 6. Apparent N loss rate in different periods (kg·ha−1·d−1).
4 Discussion
The use of N fertilizer has been one of the most effective ways to close the ‘yield gap’ between the amount of maize production and the demand (Ning et al., Citation2012; Chen et al., 2015). In this study, both cultivars and N application rates have been discussed; our findings might allow increased N uptake in maize and reduce N loss from maize production to the environment in Sichuan, China.
4.1 N-efficient maize cultivar increase N accumulation
Because N accumulation is highly related to experimental conditions (especially soil fertility and meteorological factors) (Stamatiadis et al., Citation2016), N accumulation after jointing and total N accumulation were markedly higher in 2014 than in 2015 because of the high soil fertility and appropriate precipitation in the former year. Previous studies have demonstrated that the N accumulation of a crop mainly depends on the N rate and cultivar (Montemurro et al., Citation2006; Chen et al., Citation2015). N application was found to have a significant influence on N accumulation in this study, where the total N accumulation increased with an increase in N application (). Furthermore, the N-efficient maize cultivar ZH 311 took up significantly more N than the N-inefficient maize cultivar XY 508 during the maize growing season in both years (). Li et al. (Citation2010) also found similar outcomes from other N-efficient maize cultivars (Xianyu 335 and Nongda 108, maize hybrids) and showed that N-efficient maize cultivars could improve total N accumulation by increasing N accumulation and delaying leaf senescence after silking to produce more photosynthetic products. We also found that the difference between the N-efficient cultivar ZH 311 and the N-inefficient cultivar XY 508 on total N accumulation was significantly influenced by N fertilizer level and decreased with increasing N fertilizer level. That is, the ratios of total N accumulation in ZH 311 and XY 508 were negatively correlated with the level of N fertilizer, and the regression equation of the ratio of total N accumulation in ZH 311 and XY 508 (y) and N fertilizer level (x) was y = 1.4623–0.000863x (R2 = 0.9728*), indicating that the advantages of the N-efficient cultivar are more prominent under low and medium N levels.
Except for the yield-increasing effect and ammonia volatilization loss, the level of residual mineral N in the soil is the most direct reflection of whether N fertilization is reasonable (Hamilton et al., Citation2016; Qiao et al., Citation2013). Previous studies have shown that N application drastically increases mineral N accumulation in the topsoil after harvest and that higher residual mineral N enhances soil N supply capacity, potentially migrates to the subsoil and leads to nitrate pollution of groundwater (Qiao et al., Citation2014; Yan et al., Citation2016). N fertilization was found to drastically increase residual soil inorganic N in each stage in our study, and mineral N accumulation increased with increased N application (). Moreover, the residual soil inorganic N of the N-efficient maize cultivar ZH 311 was significantly higher than that of the N-inefficient maize cultivar XY 508 in the appropriate growth stage, while that of the N-inefficient maize cultivar was higher than that of the N-efficient maize cultivar ZH 311 at MS under the low N treatment (150 N). These results indicate that the N-efficient maize cultivar ZH 311 could increase residual soil inorganic N at the appropriate growth stage, promoting soil N supply capability and enhancing N uptake while decreasing residual soil inorganic N at harvest and reducing the risk of N leaching.
4.2 N-efficient maize cultivar reduces N loss
According to Yan et al. (Citation2016) and Deng et al. (Citation2014), N balance requires the quantification of both N inputs (including initial soil N min, N mineralization, N fertilizer, and artificially provided N) and N outputs (including crop N uptake, N loss, and N immobilization) during the crop growing season and is widely used to evaluate the sustainability or efficiency of N applications, as well as the ability to maintain soil fertility in crop systems. Wang et al. (Citation2014) reported that apparent N loss increased significantly with increasing N application rate and was consistent with our study. Notably, apparent N loss is greatly related to environmental conditions and field management practices, and the apparent N min, crop N uptake and apparent N loss were noticeably higher in 2014 than in 2015 because of the high soil fertility and excessive precipitation. Compared to the N-inefficient maize cultivar XY 508, the N-efficient maize cultivar ZH 311 drastically increased the total N output by enhancing crop N uptake, which decreased the apparent N loss by 10.62% in 2014 and 6.94% in 2015 (). Nevertheless, the differences between the two maize cultivars in apparent N loss first increased and then decreased with increasing N application rate, suggesting that the N-efficient maize cultivar could effectively reduce apparent N loss compared with the N-inefficient maize cultivar and that the advantage of the N-efficient maize cultivar ZH 311 with respect to N uptake and apparent N loss was more obvious under low and medium N treatments. The loss of N can easily cause environmental pollution, so the selection of an N-efficient maize cultivar is important not only to enhance N use efficiency but also to reduce the loss of N and environmental pollution.
Previous studies have shown that N loss occurred more readily after fertilization events and at the early stages of crop growth due to the large proportion of N fertilizer input and the relatively slow N uptake by plants at the early stages (Chen et al., 2015). In this study, it was found that 80.09%~83.14% and 82.72%~84.50% of N loss occurred before the silking stage in 2014 and in 2015 (), respectively. Furthermore, the apparent N loss rate before silking was clearly higher than that after silking, indicating that apparent N loss mainly occurred before silking because all N fertilizer was applied before silking in Sichuan, and the period before silking is a relatively slow growth stage in the maize growth season. In this study, the apparent N loss and apparent N loss rate in each period of the N-efficient maize cultivar ZH 311 were noticeably lower than that of the N-inefficient maize cultivar XY 508, while the difference between the two maize cultivars in N loss at sowing stage-JS was significantly higher than that at the other stages. These results were in agreement with Chen et al. (2015), who reported that N-efficient rice cultivars showed significant reductions in N loss via NH3 volatilization, N2O emission, leaching and runoff compared with N-inefficient rice cultivars, and that the difference between the two cultivars in N loss mainly comes from the higher ability of the N-efficient cultivar to uptake more N at the early growth stages. Therefore, the N loss during the maize growth season mainly occurred before silking, and the N-efficient maize cultivar could effectively reduce N loss before silking because of its earlier N uptake ability. we researched the differences between N-efficient and N-inefficient maize cultivars on apparent N loss under different N fertilizer rates; N loss pathways, such as NH3 volatilization, N2O emission, leaching and runoff, require further research.
5 Conclusion
The N-efficient maize cultivar ZH 311 had higher N uptake and accumulation ability than the N-inefficient maize cultivar XY 508, especially in the middle and late growth stages. ZH 311 maintained higher residual soil inorganic N in the appropriate growth stage, promoting soil N supply capability and enhancing N uptake while decreasing residual soil inorganic N at harvest and reducing the risk of N leaching. Higher nitrogen accumulation and residual soil inorganic N contributed to a lower apparent N loss and apparent N loss efficiency for the N-efficient cultivar than for the N-inefficient cultivar. Moreover, the N loss during the maize growth season mainly occurred before silking, and the N-efficient maize cultivar could effectively reduce N loss before silking because of its earlier growth N uptake ability. It was concluded that the selection of N-efficient maize cultivars is not only an important measure to enhance N use efficiency but also an important means to reduce the loss of N and environmental pollution, especially at low and medium N levels.
Supplemental Material
Download MS Word (248.2 KB)Disclosure statement
No potential conflict of interest was reported by the authors.
Supplementary material
Supplemental data for this article can be accessed here.
Additional information
Funding
References
- Abdel-Ghani, A. H., Kumar, B., Reyes-Matamoros, J., Reyes-Matamoros, J., Gonzalea-Portilla, P. J., Jansen, C., … Lubberstedt, T. (2013). Genotypic variation and relationships between seedling and adult plant traits in maize (Zea mays L.) inbred lines grown under contrasting nitrogen levels. Euphytica, 189, 123–133.
- Ahmed, S., Humphreys, E., Salim, M., & Chauhan, B. S. (2016). Growth, yield and nitrogen use efficiency of dry-seeded rice as influenced by nitrogen and seed rates in Bangladesh. Field Crop Research, 186, 18–31.
- Chen, G., Chen, Y., Zhao, G. H., Cheng, W. D., Guo, S. W., Zhang, H. L., & Shi, W. M. (2015). Do high nitrogen use efficiency rice cultivars reduce nitrogen losses from paddy fields?. Agriculture, Ecosystems and Environment, 209, 26–33.
- Chen, J. W., Yang, Z. Q., Zhou, P., Hai, M. R., Tang, T. X., Liang, Y. L., & An, T. X. (2013). “Biomass accumulation and partitioning, photosynthesis, and photosynthetic induction in field-grown maize (Zea mays L.) under low- and high-nitrogen conditions. Acta Physiologiae Plantarum, 35, 95–105.
- Chen, X. P., Cui, Z. L., Fan, M. S., Vitousek, P., Zhao, M., Ma, W. Q., & Wang, Z. L. (2014). Producing more grain with lower environmental costs. Nature, 514, 486–489.
- Chen, Y. L., Xiao, C. X., Wu, D. L., Xia, T. T., Chen, Q. W., Chen, F. J., … Mi, G. H. (2015). Effects of nitrogen application rate on grain yield and grain nitrogen concentration in two maize hybrids with contrasting nitrogen remobilization efficiency. European Journal Agronomy, 62, 79–89.
- Chun, L., Mi, G. H., Li, J. S., Chen, F. J., & Zhang, F. S. (2005). Genetic analysis of maize root characteristics in response to low nitrogen stress. Plant Soil, 276, 369–382.
- Coque, M., & Gallais, A. (2010). Genetic variation among european maize varieties for nitrogen use efficiency under low and high nitrogen fertilization. Maydica, 52, 383–397.
- Deng, F., Wang, L., Ren, W. J., & Mei, X. F. (2014). Enhancing nitrogen utilization and soil nitrogen balance in paddy fields by optimizing nitrogen management and using polyaspartic acid urea. Field Crop Research, 169, 30–38.
- Feng, W., He, L., Zhang, H. Y., Guo, B. B., Zhu, Y. J., Wang, C. Y., & Guo, T. C. (2015). Assessment of plant nitrogen status using chlorophyll fluorescence parameters of the upper leaves in winter wheat. European Journal Agronomy, 64, 78–87.
- Hamilton, R. L., Trimmer, M., Bradley, C., & Pinay, G. (2016). Deforestation for oil palm alters the fundamental balance of the soil N cycle. Soil Biology and Biochemistry, 95, 223–232.
- Hofmeier, M., Roelcke, M., Han, Y., Lan, T., Bergmann, H., Böhm, D., … Nieder, R. (2015). Nitrogen management in a rice–Wheat system in the Taihu Region: Recommendations based on field experiments and surveys. Agriculture Ecosystem and Environment, 209, 60–73.
- Jian, Z., Wang, F., Li, Z., Chen, Y., Ma, X., Nie, L., & Cui, S. (2014). Grain yield and nitrogen use efficiency responses to N application in Bt (Cry1Ab/Ac) transgenic two-line hybrid rice. Field Crop Research, 155, 184–191.
- Jiang, T. (2013). Effects of nitrogen application regime on yield, quality and plantnutrient contents of summer maize. Journal of Plant Nutrition and Fertilizer, 19, 559–565.
- Li, H., Cong, R., Ren, T., Li, X., Ma, C., Zhang, L., … Lu, J. (2015). Yield response to N fertilizer and optimum N rate of winter oilseed rape under different soil indigenous N supplies. Field Crop Research, 181, 52–59.
- Li, M., Zhang, H., Yang, X., Ge, M., Ma, Q., Wei, H., … Luo, D. (2014). Accumulation and utilization of nitrogen, phosphorus and potassium of irrigated rice cultivars with high productivities and high N use efficiencies. Field Crop Research, 161, 55–63.
- Li, Q., Luo, Y. H., Yu, D. H., Kong, F. L., Yang, S. M., & Yuan, J. C. (2015). Effects of low nitrogen stress on photosynthetic characteristics and chlorophyll fluorescence parameters of maize cultivars tolerant to low nitrogen stress at the seedling stage. Journal of Plant Nutrition and Fertilizer, 25, 1132–1141.
- Li, Q., Ma, X. J., Cheng, Q. B., Dou, P., Yu, D. H., Luo, Y. H., & Yuan, J. C. (2016). Effects of nitrogen fertilizer on post-silking dry matter production and leaves function characteristics of low-nitrogen tolerance maize. Chinese Journal of Eco-Agriculture, 24, 17–26.
- Li, Q., Tan, J., Kong, F. L., Yang, S. M., & Yuan, J. C. (2014). Indexes screening and comprehensive evaluation of low nitrogen tolerance of hybrid maize cultivars at seedling stage. Chinese Journal of Eco-Agriculture, 22, 1190–1199.
- Li, W. J., He, P., Gao, Q., Yin, J. Y., Hou, Y. P., Yin, C. X., & Zhang, G. H. (2010). Dry matter formation and nitrogen uptake in two maize cultivars differing in nitrogen use efficiency. Journal of Plant Nutrition and Fertilizer, 16, 51–57.
- Ma, C. J., Liu, P., Zhao, B. Q., Zhang, S. P., Feng, H. J., Zhao, J., … Zhao, B. (2014). Regulation of nitrogen application rate on temporal and spatial distribution of roots and nitrogen uptake in different N use efficiency maize cultivars. Journal of Plant Nutrition and Fertilizer, 20, 845–859.
- Moll, R. H., Kamprath, E. J., & Jackson, W. A. (1982). Analysis and interpretation of factors which contribute to efficiency of nitrogen utilization. Agronomy Journal, 74, 562–564.
- Montemurro, F., Maiorana, M., Ferri, D., & Convertini, G. (2006). Nitrogen indicators, uptake and utilization efficiency in a maize and barley rotation cropped at different levels and sources of N fertilization. Field Crop Research, 99, 114–124.
- Mu, X. H., Chen, F. J., Wu, Q. P., Chen, Q. W., Wang, J. F., Yuan, L. X., & Mi, G. H. (2015). Genetic improvement of root growth increases maize yield via enhanced post-silking nitrogen uptake. European Journal Agronomy, 63, 55–61.
- Ning, T. Y., Zheng, Y. H., Han, H. F., Jiang, G. M., & Li, Z. J. (2012). Nitrogen uptake, biomass yield and quality of intercropped spring- and summer-sown maize at different nitrogen levels in the North China Plain. Biomass and Bioenergy, 47, 91–98.
- Pathan, S. I., Ceccherini, M. T., Pietramellara, G., Puschenreiter, M., Giagnoni, L., Arenella, M., … Renella, G. (2015). Enzyme activity and microbial community structure in the rhizosphere of two maize lines differing in N use efficiency. Plant Soil, 387, 413–424.
- Qiao, J., Yang, L. Z., Yan, T. M., Xue, F., & Zhao, D. (2013). Rice dry matter and nitrogen accumulation, soil mineral N around root and N leaching, with increasing application rates of fertilizer. European Journal Agronomy, 49, 93–103.
- Qiao, Y. F., Miao, S. J., Han, X. Z., You, M. Y., Zhu, X., & Horwath, W. R. (2014). The effect of fertilizer practices on N balance and global warming potential of maize–Soybean–Wheat rotations in Northeastern China. Field Crop Research, 161, 98–106.
- Shi, D. Y., Zhang, H. Y., & Dong, S. T. (2013). Effects of nitrogen application on nitrogen balance and use efficiency and yield of summer maize in soil with high residual nitrogen. Journal of Plant Nutrition and Fertilizer, 19, 37–44.
- Singh, P., Agrawal, M., Agrawal, S. B., Singh, S., & Singh, A. (2015). Genotypic differences in utilization of nutrients in wheat under ambient ozone concentrations: Growth, biomass and yield. Agriculture Ecosystem and Environment, 199, 26–33.
- Stamatiadis, S., Tsadilas, C., Samaras, V., Schepers, J. S., & Eskridge, K. (2016). Nitrogen uptake and N-use efficiency of Mediterranean cotton under varied deficit irrigation and N fertilization. European Journal Agronomy, 73, 144–151.
- Sui, B., Feng, X., Tian, G., Hu, X., Shen, Q., & Guo, S. (2013). Optimizing nitrogen supply increases rice yield and nitrogen use efficiency by regulating yield formation factors. Field Crop Research, 150, 99–107.
- Széles, A. V., Megyes, A., & Nagy, J. (2012). Irrigation and nitrogen effects on the leaf chlorophyll content and grain yield of maize in different crop years. Agricultural Water Management, 107, 133–144.
- Ulas, A., Behrens, T., Wiesler, F., Horst, W. J., & Erley, G. S. (2015). Defoliation affects seed yield but not N uptake and growth rate in two oilseed rape cultivars differing in post-flowering N uptake. Field Crop Research, 179, 1–5.
- Wang, X. H., Peng, L. Q., Zhang, L. P., Yin, G. D., Zhao, C., & Piao, S. L. (2014). Divergence of climate impacts on maize yield in Northeast China. Agriculture Ecosystem and Environment, 196, 51–58.
- Wang, Z., Li, J. S., & Li, Y. F. (2014). Effects of drip system uniformity and nitrogen application rate on yield and nitrogen balance of spring maize in the North China Plain. Field Crop Research, 159, 10–20.
- Yan, L., Zhang, Z. D., Zhang, J. J., Gao, Q., Feng, G. Z., Abelrahman, A. M., & Chen, Y. C. (2016). Effects of improving nitrogen management on nitrogen utilization, nitrogen balance, and reactive nitrogen losses in a Mollisol with maize monoculture in Northeast China. Environmental Science Pollution Research, 23, 4576–4584.
- Zhang, A. Y., Guo, E. H., Wang, J., Fan, H. P., Li, Y. H., Wang, L. X., … Cheng, L. P. (2015). Effect of nitrogen application rate on agronomic, photosynthetic characteristics and yield of spring foxtail millet. Scientia Agricultura Sinica, 48, 2939–2951.
- Zhang, Q., Wu, S., Chen, C., Shu, L. Z., Zhou, X. J., & Zhu, S. N. (2014). Regulation of nitrogen forms on growth of eggplant under partial root-zone irrigation. Agricultural Water Management, 142, 56–65.
- Zhang, Y., Dai, X. L., Jia, D. Y., Li, H. Y., Wang, Y. C., Li, C. X., … He, M. R. (2016). Effects of plant density on grain yield, protein size distribution, and breadmaking quality of winter wheat grown under two nitrogen fertilisation rates. European Journal Agronomy, 73, 1–10.