ABSTRACT
The common ice plant, Mesembryanthemum crystallinum L. provides a useful model for the study of environmentally induced photosynthetic conversion and abiotic stresses tolerance. However, a procedure for the production of transgenic ice plant, which is essential for functional genomics, has not been fully established. Here we tested the factors on the transformation of cotyledonary nodes excised from the ice plant seedlings such as thidiazuron (TDZ), NaCl and phytosulfokine (PSK), a peptidyl plant growth factor using Agrobacterium tumefaciens strains EHA101 and EHA105 harboring binary vector plasmids pBI7EGFP and pCAMBIA1302, respectively. The established procedure is as follows: the explants (cotyledonary nodes) were co-cultivated with Agrobacterium for 3 days, and the explants were cultured in the medium with 0.5 mg l−1 kinetin and 100 mg l−1 carbenicillin for 72 h, and they were cultured in the medium with 0.5 mg l−1 kinetin and 100 nM PSKfor 4 weeks. Thidiazuron and NaCl enhanced the production of multiple adventitious shoot formation during regeneration but reduced the transformation efficiency due to the vitrification of adventitious shoots. PSK was effective in the production of healthy adventitious shoots. The transformation frequency at the stage of whole plants was 0.6% and 4.6% per inoculated cotyledonary nodes using the Agrobacterium strain EHA101 (pBI7EGFP) and EHA105 (pCAMBIA1302), respectively.
Graphical abstract
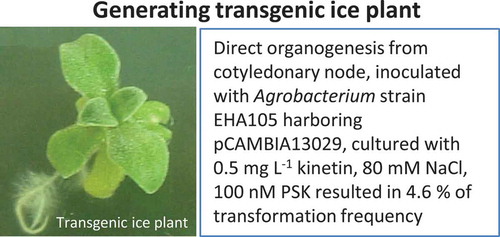
Introduction
The common ice plant, Mesembryanthemum crystallinum L. (Aizoaceae, Caryophyllales), a facultative halophyte, provides a useful model for the study of environmentally inducible switch from C3 photosynthesis to Crassulacean acid metabolism (CAM) and abiotic stresses tolerance (Bohnert & Cushman, Citation2001). Analyses of the regulation of CAM and stress responses including signaling events (Taybi & Cushman, Citation2002), circadian regulation of CAM (Boxall et al., Citation2005), biosynthesis of betalains (Vogt et al., Citation1999), scavenging system of reactive oxygen species (Slesak et al., Citation2002), regulation of salt concentration by a specialized epidermal cell (Roeurn et al., Citation2017), and identification of microRNA which associated with salinity response (Chiang et al., Citation2016) have been carried out with increasing emphasis on molecular genetic and physiological aspects. Studies for functional genomics were carried out in this species such as expressed sequence tags (ESTs) (Kore-eda et al., Citation2004) and cDNA microarray analysis (Cushman, Tillett, Wood, Branco & Schlauch, Citation2008b) and RNA-seq analysis (Tsukagoshi et al., Citation2015) of the expression profiles of genes responsible for salinity stress and mutant collection established from fast neutron-irradiated plants (Cushman et al., Citation2008a). Despite the importance of this model plant, a procedure for production of transgenic ice plant, which is essential for functional genomics, has not been established. Although hairy root cultures (Andolfatto, Bornhouser, Bohnert & Thomas, Citation1994) and callus tissues (Hwang et al., Citation2019) have been successfully transformed using Agrobacterium rhizogenes and A. tumefaciens, respectively, there have been no reports on the regeneration of transgenic plants in this species. The recalcitrance of in vitro plant regeneration has been the main reason for the lack of a rapid and efficient regeneration procedure for M. crystallinum. Regeneration protocols via organogenesis (Meiners, Thomas, Bohnert & Cushman, Citation1991) and somatic embryogenesis have been reported for the ice plant (Cushman, Wulan, Kuscuoglu & Spatz, Citation2000). However, regeneration via somatic embryogenesis has proven to be a multi-step procedure, which requires a long time interval for the development of whole plants. Direct organogenesis, which may possess less probability of somaclonal variation, may be more appropriate as a regeneration strategy for Agrobacterium-mediated ice plant transformation. We have developed a transformation procedure using Agrobacterium-mediated transformation of cotyledonary node explants coupled with the regeneration of transformed plants via direct organogenesis from cotyledonary node (Sunagawa, Agarie, Umemoto, Makishi & Nose, Citation2007). In the present study, we have examined the effects of different Agrobacterium strains and binary vectors on the efficiency of gene transfer and the effects of cytokinin, phytosulfokine (PSK) (Matsubayashi, Goto & Sakagami, Citation2004), NaCl and antibiotics on the efficiency of regeneration of transgenic ice plants. NaCl have been suggested to stimulate the growth of shoot primordia in the ice plant. The ice plant is an euhalophyte and the growth is promoted by 100–200 mM NaCl (Tran et al., Citation2019). Cushman et al. (Citation2000) added 80 mM NaCl into the medium to promote the regeneration of the ice plant from hypocotyl explants. The PSK is a small-sulfated peptide isolated from asparagus mesophyll cell that acts as a plant growth regulator (Matsubayashi et al., Citation2004). The PSK has been shown to be effective in the differentiation and cell proliferation of various plant species (Igasaki et al., Citation2003) and to increase the transformation efficiency of carrot hypocotyl explants (Matsubayashi et al., Citation2004). The transformation efficiency obtained in the present study was higher than that of reports published previously on the production of transgenic ice plant (whole plant) (Sunagawa et al., Citation2007). It has been achieved by infection with Agrobacterium strain EHA105 harboring a binary vector pCAMBIA. The PSK was effective in shortening the period of adventitious shoot formation and enhanced the production of healthy adventitious shoots.
Material and Methods
Plant materials
Mesembryanthemum crystallinum L. seeds were sterilized in 2.0% v/v sodium hypochlorite solution and were sown onto germination medium (pH 5.7) containing 1x MS salts (Murashige & Skoog, Citation1962), 1x B-5 vitamins (Gamborg, Miller & Ojima, Citation1968), 3.0% sucrose and 0.8% agar. Seedlings were grown in a growth chamber at 12-h (26°C) light/12-h dark (18°C) cycle photoperiod under cool-white fluorescent light (70–80 μmol m−2 s−1). The cotyledonary node was excised from 5-day-old seedlings.
Agrobacterium strains and binary vectors
Agrobacterium tumefaciens strains EHA101 and EHA105 were used. The strain EHA101 harbored a binary vector pBI7EGFP which contained genes for neomycin phosphotranceferase (nptII), enhanced green fluorescent protein (EGFP) and hygromycin phosphotransferase (HPH) in the T-DNA region. The strain EHA105 harbored the binary vector plasmid pCAMBIA1302, which contains genes for hygromycin phosphotransferase gene (HPH) and EGFP. All genes were driven by the CaMV35 S promoter and were terminated by the NOS terminator. The Agrobacterium strain EHA101 was grown in YEP medium containing l0 g L−1 Bacto peptone, 10 g L−1 Bacto yeast extract, 5.0 g L−1 NaCl, 15 g L−1 agar; pH 5.2 with 50 mg L−1 kanamycin and 50 mg L−1 hygromycin. The strain EHA105 was grown in the YEP with 50 mg L−1 kanamycin for 2 days at 28°C in the dark. The cells were then inoculated in liquid medium containing the same components at 28°C for 6–7 h. Then, Agrobacterium cells were collected by centrifugation for 20 min at 3000 x G at 20°C and then resuspended in MS liquid medium containing 10 mg L−1 antibiotics described above (OD600 = 0.2, pH 5.2) for transformation.
The ice plant transformation
The cotyledonary node explants were incubated with the Agrobacterium cells in MS liquid medium prepared as described above for 10 min at 25°C. They were then transferred to co-cultivation medium containing 10 mg L−1 acetosyringone and 8.0 g L−1 agar for 3 days at 25°C under a 16-h photoperiod at 70–80 µmol m−2 s−1 provided by cool white fluorescent lamps. To remove Agrobacterium, the infected cotyledonary node explants were washed with sterilized water contained 100 mg L−1 carbenicillin and were cultured on MS medium containing 100 mg L−1 carbenicillin, and 8.0 g L−1 agar for 3 to 7 days at 25°C under the same condition as described above.
Selection and regeneration of transformed plants
After co-cultivation with the Agrobacterium cells harboring pBI7EGFP or pCAMBIA, the cotyledonary node explants were transferred to medium containing plant growth regulators (PGRs), 10–20 mg L−1 hygromycin and 8.0 g L−1 agar, and cultured for 4 weeks at 25°C under the same conditions used for co-cultivation. In some experiments, NaCl and PSK were added to this medium. PSK was obtained as a gift from Dr. Y. Matsubayashi (Nagoya University, Chikusa, Nagoya, Japan). After selection, adventitious shoots were transfer to MS medium containing PGRs without antibiotics and cultured for 3–4 weeks. Resulting shoots were transferred to rooting medium, which consisted of MS medium without PGRs to induce root formation. Rooted plants were transferred to Magenta boxes (60 mm x 60 mm x 100 mm) filled with vermiculite containing 0.5x Hoagland’s solution No2 (Hoagland & Arnon, Citation1950), and the plants were grown in growth chambers with a 12-h light period (26°C/18°C day/night temperature) under cool-white fluorescent light (70–80 µmol m−2 s−1) until mature (approximately 12 weeks). The regenerated plants were acclimated by transferring to gradually decreasing humidity conditions in the green house for a week.
DNA extraction and polymerase chain reaction analyses
Genomic DNA was extracted from fresh leaf tissue of putative transformants (T0) using FTA PlantServer Card (Whatman, Tokyo, Japan), and the presence of the nptII-HPH gene was confirmed using PCR. The PCR analysis was carried out in GoTaq Green Master Mix (Promega, Madison, WI, USA), DNA (4.5 ng) and oligonucleotide primers to a final concentration of 0.5 μM. To amplify the nptII, we used the following primer pairs: forward primer 5ʹ-GTG GAG AGG CTA TTC GGC TAT GAC TGG GCA-3ʹ, and reverse primer 5ʹ-TCA TAG AAG GCG GCG GTG GAA TCG AAA TCT-3ʹ (Matthews et al., Citation2001) in the reaction conditions as follows: denaturing at 95°C for 30 sec, annealing at 63.7°C for 1 min, extension at 72°C for 1 min. The program was terminated by final extension step at 72°C for 7 min. The amplification products were analyzed by electrophoresis on 1.2% agarose-ethidium bromide gels (0.005% ethidium bromide) with KODAK 1D image analysis software.
Results and Discussion
Effects of plant growth regulator
In the previous report, we have shown that cotyledonary nodes of the ice plant regenerated at high efficiency with a urea type cytokinin, thidiazuron (TDZ) (Sunagawa et al., Citation2007). TDZ has higher differentiation induction ability and was effective in the regeneration of recalcitrant plants (Fasolo, Zimmerman & Fordham, Citation1989; Kerns & Meyer, Citation1986). However, in some species, TDZ caused excess proliferation, elongation and vitrification (Huetteman & Preece, Citation1993). Similar phenomena were observed in the previous report (Sunagawa et al., Citation2007) during the regeneration of cotyledonary nodes which had been infected with Agrobacterium. Murthy, Murch and Saxena (Citation1998) have shown that lowering TDZ concentration and combination of TDZ with another plant growth regulator were effective in stimulating proper or more regular plant morphogenesis. To examine combination effects of plant growth regulators on the induction of healthy shoot formation of transgenic ice plant, the explants of cotyledonary node infected with Agrobacterium strain EHA101 were cultured on MS medium supplement with 1 mg l−1 TDZ, 0.25 mg L−1 1-naphthaleneacetic acid (NAA) (these concentrations were determined based on the results reported previously) (Sunagawa et al., Citation2007), and 150 mg L−1 carbenicillin for 1 week and transferred onto the media supplement with TDZ, 6-benzylaminopurine (BAP), NAA and gibberellic acid (GA3) without antibiotics (). The survival ratio of explant and shoot formation ratio were relatively high ranging from 78% to 99% and 58% to 73%, respectively. Vitrification occurred in explants cultured in all mediums tested and tended to increase with combinations of TDZ and NAA. Many explants formed calli and multiple shoots on basal portions of cotyledons. The effect of BAP was not clear in the survival ratio, shoot formation ratio and vitrification. The survival ratio and shoot formation ratio decreased, and vitrification tended to increase with increasing of BAP concentration. The GA3 was effective in the increase of the survival ratio and the shoot formation ratio and reduction of vitrification, but those effects were limited. When GA3 was increased from 0.1 to 1 mg l-1 in a medium containing 1 mg l-1 of BAP without TDZ, the survival ratio and the shoot formation ratio increased from 87.9% to 94.2%, and 66.3% to 69.2%, respectively. While the vitrification ratio decreased from 43.5% to 30.8%, but there was no statistical significance. To increase the efficiency of shoot differentiation, we examined the effects of NaCl and phytosulfokine (PSK) on shoot growth under selection culture (). In the other plant species such as rice, shoot formation from hypocotyl-derived callus was also promoted by NaCl (Binh, Heszky, Gyulai & Csillag, Citation1992). The PSK has been suggested to be effective in the differentiation and cell proliferation (Igasaki et al., Citation2003) and it increased the transformation efficiency of carrot hypocotyl explants (Matsubayashi et al., Citation2004). In the present study plant survival at 4 weeks after the selection was promoted by NaCl. The ratio of shoot primordia formation was higher in explant cultured on medium containing either PSK or NaCl versus control medium. Adventitious shoot formation at 2 weeks after regeneration was observed in medium containing 100 nM PSK but not in the medium with 10 nM and 1000 nM PSK, indicating that the optimal concentration of PSK for ice plant organogenesis was 100 nM.
Table 1. Effects of plant growth regulators on shoot formation of transgenic ice plant.
Table 2. Effects of NaCl and PSK on shoot formation of transgenic ice plant.
Shoot vitrification was promoted by NaCl but was reduced by PSK. The vitrification rate in medium with NaCl and without PSK, with NaCl and 100 nM PSK, or without NaCl and with 100 nM PSK were 46.5%, 41% and 5.4%, respectively. Once vitrification was induced, healthy shoots were not produced. Vitrification seems to be the main factor inhibiting cell redifferentiation of explants. Humidity, the composition of inorganic ions, the concentration of carbohydrates and plant growth regulators, quality and quantity of light can influence the frequency of vitrification (Ziv, Citation1991). In cauliflower tissue culture, increased Na+ in guard cells decreased stomatal aperture and reduced transpiration, followed by an excessive increase in water content of tissues, which consequently caused vitrification (Wardle, Dixon & Simpkin, Citation1981). The accumulation of NaCl in euhalophytic ice plant tissues is likely to facilitate the absorption of water through the generation of a water potential gradient, which can increase turgor pressure of the cells. The increased turgor pressure may accelerate cell growth, but excessive water absorption likely results in vitrification. The mechanisms of the induction of vitrification are not well understood. Further study on factors inducing vitrification should be done to improve the differentiation efficiency of ice plant transformants.
The effects of strains of A. tumefaciens and vectors
In the Agrobacterium-mediated transformation, the strain of A. tumefacience and binary vector influence on the efficiency of transformation (Nadolska-Orczyk & Orczyk, Citation2000; Pniewski & Kapusta, Citation2005). Regeneration activity of transformed explants was improved by increasing Agrobacterium-mediated T-DNA delivery via reducing or overcoming factors that inhibit host–pathogen interaction. In the present study, we used A. tumefaciens strains EHA101 harboring pBI7EGFP and EHA105 harboring pCAMBIA1302. shows the survival ratios, shoot primordia formation after 4-week selection, and shoot formation and vitrification of explants infected with Agrobacterium strain EHA105 containing the binary vector plasmid pCAMBIA1302 after 2 weeks on shoot induction medium. The regeneration trends in the plant transformed with EHA105 were the same as that with EHA101 (). Survival ratios of explants and shoot primordia formation following four-weeks of selection were higher in NaCl-containing medium, and adventitious shoot formation after 2 weeks shoot induction was higher in the medium with 100 nM PSK without NaCl. The vitrification of explant treated with EHA105 occurred in the medium contained NaCl as observed in the transformants with EHA101.
Table 3. Effects of NaCl and PSK on shoot formation of ice plant transformed with Agrobacterium EHA105 harboring pCAMBIA1302.
Libik, Konieczny, Pater, Slesak and Miszalski (Citation2005) showed that kinetin (KT) reduced the vitrification of hypocotyl-derived callus of ice plant. In the present study, the cotyledonary node was transformed mediated by EHA105, and cultured on MS agar medium supplement with 0.5 mg L−1 Kinetin, 80 mM NaCl and 100 mg L−1 carbenicillin. shows the survival ratio, shoot-formation ratio, rooting ratio and transformation efficiency of acclimatized transformants after 5 weeks of culture. To avoid the inhibition of shoot growth, antibiotic selection was not carried out in this experiment. The survival ratio was higher (65–68%) for both EHA101 harboring pBI7EGFP and EHA105 harboring pCAMBIA1302, and all surviving shoots were less frequency of vitrification on the kinetin containing medium.
Table 4. Survival ratio, shoot formation ratio and rooting ratio, and transformation efficiency in the ice plant transformed with Agrobacterium EHA105 harboring pCAMBIA1302 and EHA101 harboring pBI7EGFP.
The difference between EHA101 (Hood, Helmer, Fraley & Chilton, Citation1986) and EHA105 (Hood, Gelvin, Melchers & Hoekema, Citation1993) is only the absence of a kanamycin-resistance gene that confers antibiotic selection in bacteria. pCAMBIA1302 has the replication origin of the Pseudomonas plasmid pVS1 (Itoh, Watson, Haas & Leisinger, Citation1984) whereas pBI7EGEP has RK2, the replication origin derived from the P-type plasmid RK2 (Doran, Konieczny & Helinski, Citation1998). Owing to its complete partition system, the pVS1 segment confers the genetic stability over generations without selection pressure. This characteristic, which RK2-based binary vectors lack, appears to be essential for higher transformation efficiency in plants. From these results, we suggest that the combination of EHA105 and pCAMBIA1302 was more suitable than in EHA101 and pBI7EGFP for the transformation of the ice plant.
Confirmation of the introduction of foreign gene
To confirm the existence of transgenes in the genome of putative transformants, PCR analysis was carried out. The representative PCR products, which were obtained by amplification of DNA from the leaves transformed via Agrobacterium EHA105 harboring pCAMBIA1302 were shown (). The sizes of PCR product (770 bp) was the same with that of plasmid DNA from pCAMBIA 1302. The overall transformation frequency at the stage of whole plants were 0.6% and 4.6% per inoculated cotyledonary nodes using the Agrobacterium strain EHA101 (pBI7EGFP) and EHA105 (pCAMBIA1302), respectively. These transformation efficiencies were higher than those of the report of Sunagawa et al. (Citation2007), in which the transformation efficiency was 0.3% in the transformed cotyledonary node of ice plant infected with Agrobacterium strain EHA101 harboring a binary vector pBI, and 0.02% in the other halophyte Atriplex (Uchida, Nagamiya & Takebe, Citation2003).
Conclusions
Our results showed that transgenic ice plant was obtained efficiently from direct organogenesis from coryledonary node explants, which were co-cultivated with Agrobacterium strain EHA105 having pCAMBIA1302 on medium containing kinetin supplemented with 80 mM NaCl and 100 nM PSK. The transformation frequency to the stage of whole plants was 4.6% per inoculated cotyledonary nodes. The relatively high transformation frequency reported here indicated that this simple transformation system can serve for rapid in planta studies of functional genomics addressing specialized traits such as CAM and halophilism.
Figure 1. PCR analysis of DNA isolated from the leaves of transformants. Transformation was made with the Agrobacterium EHA105 carrying the binary vector plasmids pCAMBIA1302. Agarose gene electrophoresis of PCR amplification was performed with primers of the kanamycin resistance gene, neomycin phosphotransferase NPT-II. C, plasmid DNA; M, DNA size marker. 1–11: transformants, 12–15, non-transformants. DNA was isolated by FTA PlantServer Card (Whatman, Tokyo, Japan).
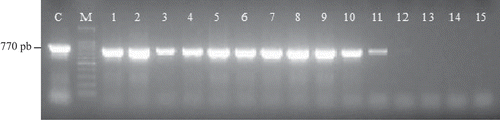
Acknowledgments
This research was supported by a grant-in-aid for Scientific Research (KAKENHI) from the Ministry of Education, Science, Sports and Culture of Japan (No. 18380015). Authors are deeply grateful to Dr. Y. Matsubayashi, Nagoya University, Chikusa, Nagoya, Japan for providing PSK.
Disclosure Statement
No potential conflict of interest was reported by the authors.
References
- Andolfatto, P., Bornhouser, A., Bohnert, H. J., & Thomas, J. C. (1994). Transformed hairy roots of Mesembryanthemum crystallinum: Gene expression patterns upon salt stress. Physiologia Plantarum, 90, 708–714.
- Binh, D. Q., Heszky, L. E., Gyulai, G., & Csillag, A. (1992). Plant regeneration of NaCl - pretreated cells from long - term suspension culture of rice (Oryza sativa L.) in high saline conditions. Plant Cell, Tissue and Organ Culture, 29, 75–82.
- Bohnert, H. J., & Cushman, J. C. (2001). The ice plant cometh: Lessons in abiotic stress tolerance. Journal of Plant Growth Regulation, 19, 334–346.
- Boxall, S. F., Foster, J. M., Bohnert, H. J., Cushman, J. C., Nimmo, H. G., & Hartwell, J. (2005). Conservation and divergence of circadian clock operation in a stress-inducible Crassulacean acid metabolism species reveals clock compensation against stress. Plant Physiology, 137, 969–982.
- Chiang, C.-P., Yim, W. C., Sun, Y.-H., Ohnishi, M., Mimura, T., Cushman, J. C., & Yen, H. E. (2016). Identification of ice plant (Mesembryanthemum crystallinum L.) microRNAs using RNA-seq and their putative roles in high salinity responses in seedlings. Frontiers in Plant Science, 7. doi:10.3389/fpls.2016.01143
- Cushman, J. C., Agarie, S., Albion, R. L., Elliot, S. M., Taybi, T., & Borland, A. M. (2008a). Isolation and characterization of mutants of common ice plant deficient in Crassulacean acid metabolism. Plant Physiology, 147, 228–238.
- Cushman, J. C., Tillett, R. L., Wood, J. A., Branco, J. M., & Schlauch, K. A. (2008b). Large-scale mRNA expression profiling in the common ice plant, Mesembryanthemum crystallinum, performing C3 photosynthesis and Crassulacean acid metabolism (CAM). Journal of Experimental Botany, 59, 1875–1894.
- Cushman, J. C., Wulan, T., Kuscuoglu, N., & Spatz, M. D. (2000). Efficient plant regeneration of Mesembryanthemum crystallinum via somatic embryogenesis. Plant Cell Reports, 19, 459–463.
- Doran, K. S., Konieczny, I., & Helinski, D. R. (1998). Replication origin of the broad host range plasmid RK2. Positioning of various motifs is critical for initiation of replication. Journal of Biological Chemistry, 273, 8447–8453.
- Fasolo, F., Zimmerman, R. H., & Fordham, I. (1989). Adventitious shoot formation on excised leaves of in vitro grown shoots of apple cultivars. Plant Cell, Tissue and Organ Culture, 16, 75–87.
- Gamborg, O. L., Miller, R. A., & Ojima, K. (1968). Nutrients requirements of suspension culture of soybean roots cells. Experimental Cell Research, 50, 150–158.
- Hoagland, D. R., & Arnon, D. I. (1950). The water-culture method for growing plants without soil. California Agricultural Experiment Station Circular, 347, 1–39.
- Hood, E. E., Gelvin, S. B., Melchers, L. S., & Hoekema, A. (1993). New Agrobacterium helper plasmids for gene transfer to plants. Transgenic Research, 2, 208–218.
- Hood, E. E., Helmer, G. L., Fraley, R. T., & Chilton, M. (1986). The hypervirulence of Agrobacterium tumefaciens A281 is encoded in a region of pTiBo542 outside of T-DNA. Journal of Bacteriology, 168, 1291–1301.
- Huetteman, C. A., & Preece, J. E. (1993). Thidiazuron: A potent cytokinin for woody plant tissue culture. Plant Cell, Tissue and Organ Culture, 33, 105–119.
- Hwang, H. H., Wang, C. H., Chen, H. H., Ho, J. F., Chi, S. F., Huang, F. C., & Yen, H. E. (2019). Effective Agrobacterium-mediated transformation protocols for callus and roots of halophyte ice plant (Mesembryanthemum crystallinum). Botanical Studies, 60. doi:10.1186/s40529-018-0249-3
- Igasaki, T., Akashi, N., Ujino-Ihara, T., Matsubayashi, Y., Sakagami, Y., & Shinohara, K. (2003). Phytosulfokine stimulates somatic embryogenesis in Cryptomeria japonica. Plant Cell Physiology, 44, 1412–1416.
- Itoh, Y., Watson, J. M., Haas, D., & Leisinger, T. (1984). Genetic and molecular characterization of the Pseudomonas plasmid pVS1. Plasmid, 11, 206–220.
- Kerns, H. R., & Meyer, M. M., Jr. (1986). Tissue culture propagation of Acerfreemanii using thidiazuron to stimulate shoot tip proliferation. HortScience, 21, 1209–1210.
- Kore-eda, S., Cushman, M. A., Akselrod, I., Bufford, D., Fredrickson, M., Clark, E., & Cushman, J. C. (2004). Transcript profiling of salinity stress responses by large-scale expressed sequence tag analysis in Mesembryanthemum crystallinum. Gene, 341, 83–92.
- Libik, M., Konieczny, R., Pater, B., Slesak, I., & Miszalski, Z. (2005). Differences in the activities of some antioxidant enzymes and in H2O2 content during rhizogenesis and somatic embryogenesis in callus culture of the ice plant. Plant Cell Reports, 23, 834–841.
- Matsubayashi, Y., Goto, T., & Sakagami, Y. (2004). Chemical nursing: Phytosulfokine improves genetic transformation efficiency by promoting the proliferation of surviving cells on selective media. Plant Cell Reports, 23, 155–158.
- Matthews, P. R., Wang, M.-B., Waterhouse, P. M., Thornton, S., Fieg, S., Gubler, J. F., & Jacobsen, J. V. (2001). Marker gene elimination from transgenic barley, using co-transformation with adjacent ‘twin T-DNAs’ on a standard Agrobacterium transformation vector. Molecular Breeding, 7, 195–202.
- Meiners, M. S., Thomas, J. C., Bohnert, H. J., & Cushman, J. C. (1991). Regeneration of multiple shoots and plants from Mesembryanthemum crystallinum. Plant Cell Reports, 9, 563–566.
- Murashige, T., & Skoog, F. (1962). A revised medium for rapid growth and bioassays with tobacco tissue cultures. Physiologia Plantarum, 15, 473–479.
- Murthy, B. N. S., Murch, S. J., & Saxena, P. K. (1998). Thidiazuron: a potent regulator of in vitro plant morphogenesis. In Vitro Cellular Developmental Biology-Plant, 34, 267–275.
- Nadolska-Orczyk, A., & Orczyk, W. (2000). Study of the factors influencing Agrobacterium-mediated transformation of pea (Pisum sativum L.). Molecular Breeding, 6, 185–194.
- Pniewski, T., & Kapusta, J. (2005). Efficiency of transformation of Polish cultivars of pea (Pisum sativum L.) with various regeneration capacity by using hypervirulent Agrobacterium tumefaciens strains. Journal of Applied Genetics, 46, 139–147.
- Roeurn, S., Hoshino, N., Soejima, K., Inoue, Y., Cushman, J. C., & Agarie. (2017). MYB and HD-ZIP IV homologs related to trichome formation are involved in epidermal bladder cell development in the halophyte Mesembryanthemum crystallinum L. Plant Production Science, 20, 72–82.
- Slesak, I., Miszalski, Z., Karpinska, B., Niewiadomska, E., Ratajczak, R., & Karpinski, S. (2002). Redox control of oxidative stress responses in the C3-CAM intermediate plant Mesembryanthemum crystallinum. Plant Physiology and Biochemistry, 40, 669–677.
- Sunagawa, H., Agarie, S., Umemoto, M., Makishi, Y., & Nose, A. (2007). Effect of urea-type cytokinins on the adventitious shoots regeneration from cotyledonary node explant in the common ice plant, Mesembryanthemum crystallinum. Plant Production Science, 10, 47–56.
- Taybi, T., & Cushman, J. C. (2002). Abscisic acid signaling and protein synthesis requirements for CAM induction in the common ice plant. Journal of Plant Physiology, 159, 1235–1243.
- Tran, D. Q., Konishi, A., Cushman, J. C., Morokuma, M., Toyota, M., & Agarie, S. (2019). Ion accumulation and expression of ion homeostasis-related genes associated with halophilism, NaCl-promoted growth in a halophyte Mesembryanthemum crystallinum L. Plant Production Science. doi:10.1080/1343943X.2019.1647788
- Tsukagoshi, H., Suzuki, T., Nishikawa, K., Agarie, S., Ishiguro, S., & Higashiyama, T. (2015). RNA-Seq analysis of the response of the halophyte, Mesembryanthemum crystallinum (ice Plant) to high salinity. PloS One, e0118339. eCollection 2015. doi:10.1371/journal.pone.0118339
- Uchida, A., Nagamiya, K., & Takebe, T. (2003). Transformation of Atriplex gmelini plants from callus lines using Agrobacterium tumefaciens. Plant Cell, Tissue and Organ Culture, 75, 151–157.
- Vogt, T., Ibdah, M., Schmidt, J., Wray, V., Nimtz, M., & Strack, D. (1999). Light-induced betacyanin and flavonol accumulation in bladder cells of Mesembryanthemum crystallinum. Phytochemistry, 52, 583–592.
- Wardle, K., Dixon, P. A., & Simpkin, I. (1981). Sodium accumulation by leaves of cauliflower plantlets and the effect on the mode of plant formation. Annals of Botany, 44, 653–659.
- Ziv, M. (1991). Vitrification: Morphological and physiological disorders of in vitro plants. In P. C. Debergh & R. H. Zimmerman (Eds.), Micropropagation: Technology and applications (pp. 45–69). Dordrecht: Springer.