ABSTRACT
Changes in root development and growth in upland rice receiving subsurface irrigation were evaluated using root box experiments. Irrigated water was independently applied every day to depths of 0, 5, 10 and 20 cm from the ground surface, and root morphology and aboveground growth were determined after 2 weeks of treatment. Plant length, stem number and leaf age significantly increased along with the irrigation depth, and the aboveground dry weights of rice grown at 5-, 10- and 20-cm depths were significantly greater than those grown at 0 cm. Root surface area significantly increased along with the irrigation depth, which was attributed to the increasing lengths and branch numbers of roots. Subsurface irrigation significantly increased root lengths in deep layers (below 15 cm) and increased the deep layers’ distribution ratios by 1.2 to 5.7 times as the surface layer ratio decreased. This was accompanied with a decrease in the root diameter. This may be associated with the increase in the numbers of branches and the decrease in the numbers of thick nodal roots. These results indicate that subsurface irrigation increases root length and branching in the deep soil layers, inducing deep rooting, even with same amount of irrigation.
Graphical abstract
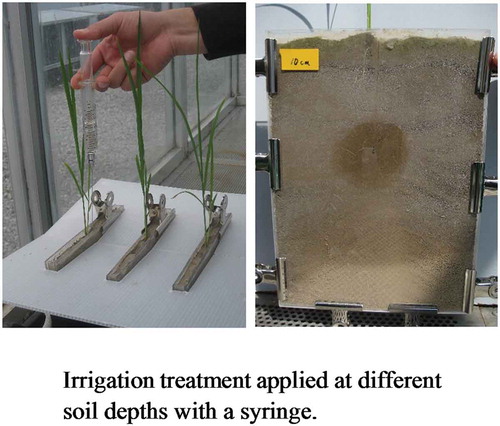
Introduction
Rainfed upland rice is grown on approximately 32% of the rice cultivation area in sub-Saharan Africa (Balasubramanian et al., Citation2007; Saito et al., Citation2018); however, the yield tends to be unstable because of the erratic and unpredictable rainfall (Serraj et al., Citation2011), remaining at less than 2 t ha−1 on average (Kijima et al., Citation2006; Saito et al., Citation2018). Increasing rice yields in rainfed cultivation areas requires the absorption of water from deep soil layers that have relatively high soil moisture contents (Banba & Ohkubo, Citation1981; Hirayama et al., Citation2007). This requires that the rice has a deep root system with high root lengths and densities in deeper soil layers (Fukai & Cooper, Citation1995; Yoshida & Hasegawa, Citation1982). In particular, the deep root system during the early seedling stage contributes to the development of aboveground parts and large root systems under drought conditions, resulting in an increased water-use efficiency (Avramova et al., Citation2016).
A deep root system can be produced by altering the root structure; rice roots elongate deeply under field conditions with low soil moisture contents compared with under paddy conditions (Oyanagi, Citation1999; Sasaki, Citation1932), and this is achieved by increasing root branching to extract soil water efficiently (Banba & Ohkubo, Citation1981). Additionally, a deep root system requires the root ability to penetrate into the soil, and this capability is strong in gramineous crops having thick roots (Materechera et al., Citation1991; Tanakamaru et al., Citation1998). Although cultivar differences and breeding affect deep root systems (Nemoto et al., Citation1998; Uga, Citation2013; Uga et al., Citation2013), it is also possible to induce their production through cultivation management. For instance, a deep application of fertilizer can promote root elongation in deep soil layers (Ao & Yoshikawa, Citation1993; Ishizuka & Tanaka, Citation1962) and increase the primary root lengths in rice (Kono et al., Citation1976). Additionally, subsurface irrigation increases maize root length and density in deep soil layers (Coelho & Or, Citation1999; Phene et al., Citation1991). These results suggest that the root systems in field crops alter their root structure to adapt to changes in the environment. This is referred to as ‘phenotypic plasticity’, which is a critical element for stabilizing plant growth under drought conditions (O’toole & Bland, Citation1987; Yamauchi et al., Citation2008). These suggest that root structure and final yield are expected to be improved by an optimum water management.
Upland rice NERICA varieties have been widely spread in sub-Saharan Africa (Diagne et al., Citation2010). To evaluate the induction of deep rooting in upland rice NERICA through water management, we quantified the effects of subsurface irrigation on root morphology and aboveground growth. Additionally, we discuss alterations in root structure to identify desirable root structures inducing deep rooting.
Materials and methods
Root box experiments (Exps) were conducted three times (Exps 1–3) in a temperature-controlled room (daytime 6:00–18:00, 30°C; nighttime 18:00–6:00, 25°C) with natural sunlight in September–October and October–November, 2015, and February–March, 2016, respectively. Upland rice cultivar NERICA 4 was used. NERICA 4 has been most widely adopted or certified among sub-Saharan Africa countries (Diagne et al., Citation2010) and known as a variety with a deep root trait (Menge et al., Citation2016). In total, 600 g of field soil sieved through 2-mm mesh was used to fill the acrylic root box (20.9 cm in width, 28.6 cm in height and 1 cm in thickness). A compound fertilizer containing 14% N, 14% P2O5, and 14% K2O at a rate of 0.12 g per box was incorporated into the soil. The root box was covered with a black nylon bag. At 1 week after sowing, a seedling, having 2.2–2.3 leaves, was transplanted into a root box in Exp. 1 and 2. In Exp. 3, three germinated seeds were sown and thinned to a single seedling at 1 week after sowing. Irrigation was applied at 20 mL per day onto the surface of the box. As an irrigation treatment, 10 mL of tap water was applied every day for 2 weeks at 5-, 10- and 20-cm soil depths from the surface layer with a syringe (), and the same amount of water was applied at 0-cm soil surface layer as a control. The treatments were started from 11 and 7 d after transplanting with three to four replications in Exp. 1 and 2, respectively, and from 18 d after sowing with five replications in Exp. 3. The pretreatment period was adjusted in each Exp. for the size of root system to remain within the root box. The sum of sunshine hours during treatment period was 87, 69, 73 h in Exp. 1–3, respectively, which was collected from AMeDAS at the Japan Meteorological Agency, at the Kochi observation site located 13 km away from the experimental site.
All the measurements and analyses of root structures in the root boxes were conducted after the treatment following the methods of Kono et al. (Citation1987). After a side plate of the root box was removed, the root system was fixed by inserting a pin-board into the soil, and the soil attached to the roots on the pin-board was carefully removed. Roots on the pin-board were stained with 0.25% Coomassie Brilliant Blue solution for 10 min, and a recrementitious solution was rinsed in tap water. Although the staining period was shorter than that in previous reports (Kano-Nakata et al., Citation2012; Kono et al., Citation1987), most of the nodal and lateral roots were substantially stained as a comparative assessment. A plastic film stuck on the pin-board in advance was removed with the root system, and the root system was turned over carefully onto a transparent acrylic plate. An image of the root system was scanned with a scanner (GT-X970, Epson) into the computer (resolution 600 dpi). From the image, the surface area, length, average diameter and the number of branches in roots were determined every 5 cm from the ground surface using an image analysis system (WinRhizo, Basic 2007, Regent Instruments, Quebec, Canada). Average diameter was calculated from total projected area and length. In addition, the number of nodal roots was counted. The root sample was dried more than 2 d at 80°C, and the dry matter weight was measured. The aboveground material was collected from the base and used to measure the plant length, stem number, leaf age and dry matter weight.
Data collected were analyzed statistically with an analysis of variance (ANOVA) using JMP 7 (SAS institute Inc., Cray, NC, USA). Statistically significant differences between means were identified by Tukey’s honestly significant difference (HSD) test at p < 0.05.
Results
The root surface area significantly increased 30–46% on average with subsurface irrigation (). Similarly, a significant increase was observed in root length (34–63%) and the numbers of root branches (44–59%), however, root diameter and the length of one branch were not significantly affected by subsurface irrigation. The numbers of nodal roots in the 10-cm and 20-cm treatments were significantly less than in the 5-cm treatment, indicating a decrease with subsurface irrigation. This tendency was commonly observed in Exps 1–3, although there was no significant difference between treatments in Exp. 1 and 2. Root length at the deep layer (below 15 cm) significantly increased with subsurface irrigation, inducing greater percentages of root lengths at deep layers to total lengths in the 10-cm and 20-cm treatments. Root length had a positive correlation with the number of root branches at P < 0.001 ().
Table 1. Root morphology in upland rice irrigated at different soil depths.
Figure 2. Correlation between the numbers of root branches and root lengths in upland rice. *** indicates 0.1% level of significance.
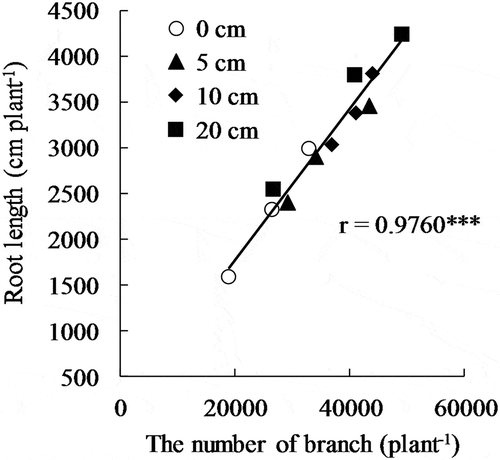
The root morphology was analyzed at every 5 cm of depth using a two-way analysis of treatments and Exps 1–3 (). The greatest root surface area was observed in the 0-cm treatment at the depth of 0–5 cm, in the 5-cm treatment at the depth of 5–10 cm, in 5-cm and 10-cm treatments at the depth of 10–15 cm, and in the 10-cm and 20-cm treatments at the depth of 15–20 cm, and in the 20-cm treatment at the depth of 20–25 cm. Similar tendencies were found for root length and the number of branches. However, root diameter significantly decreased with subsurface irrigation, especially at the depth of 10–20 cm. With subsurface irrigation, the length of one branch increased at the shallow soil layer and decreased at the deep soil layer.
Table 2. Root morphology analyzed at every 5 cm of soil depth in upland rice irrigated at different soil depths.
With subsurface irrigation, plant length, stem number and leaf age significantly increased 7–17%, 19–33% and 7–12% on average, respectively (). The aboveground dry weights in the 5-, 10-, 20-cm treatments were significantly greater by 30–50% on average compared with the 0-cm treatment, although there were no significant differences among the three treatments. Root dry weights were not significantly different among treatments, but there was a greater tendency, by 16–50% on average, in the 5-, 10- and 20-cm treatments compared with the 0-cm treatment. No significant differences among treatments were found for the shoot to root dry weight ratio.
Table 3. Growing traits in upland rice irrigated at different soil depths.
Images of root morphology are shown in . The root system was widely and deeply distributed in the 10-cm and 20-cm treatments when irrigated deeply compared with the 0-cm treatment irrigated on the ground surface. The emergence of a large number of branch roots from less nodal roots was observed in the 10-cm and 20-cm treatments.
Discussion
Subsurface irrigation increased root surface area though root length along with the numbers of root branch, inducing deep rooting in rice grown under upland conditions (, ). Rice roots elongate deeper in dry fields than in paddy fields (Sasaki, Citation1932), and the maize root lengths in deep soil layers increase along with subsurface irrigation (Coelho & Or, Citation1999; Phene et al., Citation1991). Root growth under drought conditions is promoted by irrigation applied from above in adlay, but from underneath in sorghum. However, in soybean, the increase is almost-imperceptible owing to the lower level of root plasticity (Kono & Yamauchi, Citation1996). Changes in root distributions may be attributed to root elongation owing to hydrotropism induced by decreasing soil water potential (Nakamoto, Citation1993; Oyanagi et al., Citation1992).
The alterations in root structures of deep root systems resulted from an increase in the numbers of root branches (). The increase in the number of root branch was observed mainly at the deep layer, inducing a significant increase in the lengths and surface areas of roots, resulting in a high deep root ratio. The number of root branch in upland rice increases under drought conditions, regardless of whether the variety was upland drought-tolerant or lowland-adapted (Kameoka et al., Citation2015, Citation2016; Menge et al., Citation2016). The plasticity of branch roots is dependent on the nodal rooting ability under drought conditions (Kameoka et al., Citation2016). Thus, the depth of the root system is variable depending on the cultivation conditions. However, no significant differences among treatments were observed in Exp. 2, in which the nursery period and sunshine hours were shorter than in Exps 1 and 3, resulting in shallower root systems. Therefore, the water irrigated at the deep layer might have been unavailable, although a slight increase in root morphology was observed with the 10-cm treatment in Exp. 2. This suggests that an appropriate irrigation depth, adjusted for the depth of the root system, is required. The appropriate irrigation depth would significantly increase the root amount at the irrigation depth ().
A decrease in the root diameter was induced by subsurface irrigation (). This is partly attributed to increased fine branch numbers; however, the root diameter decreased with subsurface irrigation even at the shallow layers, where the branch numbers decreased. This is dependent on the significant decrease in the numbers of thick nodal roots emerging from the bases of hulls. The emergence of nodal roots is remarkably inhibited in rice grown under dry conditions, and lateral roots emerge from less nodal roots (Banoc et al., Citation2000; Kameoka et al., Citation2015, Citation2016; Suralta et al., Citation2010). Root structures having less nodal roots and a deeper rooting ratio are genetically observed in drought-tolerant cultivars (Kameoka et al., Citation2015, Citation2016; Suralta et al., Citation2010). Therefore, inhibiting the emergence of nodal roots, which elongate into the deep soil layers where lateral roots develop, appears to be an efficient strategy to alter the structure into the deep root system.
Drought-tolerant crops have large root surface areas for absorbing water from a wide soil rhizosphere under drought conditions (Kono & Yamauchi, Citation1996). As a branching pattern (Yamauchi et al., Citation1987), they have a number of nodal roots that elongate obliquely into the soil with a number of L-type lateral roots (thick, long and highly branching) and a smaller number of S-type lateral roots (relatively thin, short and unbranching). The numbers of L-type lateral primary roots increase under drought conditions with enlarging root systems, and the number is higher in the drought-tolerant upland rice variety (Kono & Yamauchi, Citation1996). Different types of lateral roots may have the specific potential of water absorption (Niones et al. Citation2015; Suganuma et al., Citation2012).
The diameter of the primary root is closely associated with the ability of roots to penetrate through the compacted soil layers or hardpans in all plant species (Materechera et al., Citation1991). Zheng et al. (Citation2000) implied that thick roots have strong root-penetration abilities, and they lead to more deep roots in a double-haploid population. Tanakamaru et al. (Citation1998) reported that gramineous crops with large-diameter seminal roots have a greater ability to penetrate the soil. Therefore, an increase in thick roots appears to play a crucial role in forming deep root systems.
Thus, a deep root system appeared to be induced by subsurface irrigation, which resulted from a decrease in the numbers of nodal roots and an increase in the numbers of root branches in the deep soil layer ( and ). The root structure alteration offers important insights into a desirable root structure that is adapted to drought conditions, and the efficacy of the deep root system was verified by the promotion of the aboveground growth (). As the aboveground growth was improved by the water management, the drought condition was assumed to be imposed during the treatment period, although soil moisture was not determined in this study. Aboveground growth and grain yield in maize significantly increase with subsurface irrigation (Douh & Boujelben, Citation2011). The increased aboveground growth through subsurface irrigation might be synergistically attributed to an increased water-use efficiency owing to less soil evaporation (Hamada et al., Citation2015). These results suggest strongly that even with same amount of water, differences in irrigation methods strengthen the capacity of deep rooting in upland NERICA. Our results will be useful for further research on efficient water-use management strategies and cultivar breeding to identify root structures that adapt to drought conditions.
Correction Statement
This article has been republished with minor changes. These changes do not impact the academic content of the article.
Acknowledgments
We thank Lesley Benyon, PhD, from Edanz Group (www.edanzediting.com/ac) for editing a draft of this manuscript.
Disclosure statement
No potential conflict of interest was reported by the authors.
Additional information
Funding
References
- Ao, H., & Yoshikawa, S. (1993). Effect of the deep placement of fertilizer application using a subsoiler for soybean plants on the rotational upland fields. Bulletin of the Mie Agricultural Technical Center, 21, 1–11. (in Japanese with English summary).
- Avramova, V., Nagel, K. A., AbdElgawad, H., Bustos, D., DuPlessis, M., Fiorani, F., & Beemster, G. T. S. (2016). Screening for drought tolerance of maize hybrids by multi-scale analysis of root and shoot traits at the seedling stage. Journal of Experimental Botany, 67, 2453–2466.
- Balasubramanian, V., Sie, M., Hijmans, R. J., & Otuka, K. (2007). Increasing rice production in sub-saharan Africa: Challenges and opportunities. Advances in Agronomy, 94, 55–133.
- Banba, H., & Ohkubo, T. (1981). Relationship between root distribution of upland crops and their yield. III. Influence of soil moisture levels on root distribution and root dry matter of upland-cultured paddy rice, crossbred rice of paddy rice and upland rice, and upland rice. Japanese Journal of Crop Science, 50, 1–7. (in Japanese with English summary).
- Banoc, D. M., Yamauchi, A., Kamoshita, A., Wade, L. J., & Pardales, J. R. (2000). Dry matter production and root system development of rice cultivars under fluctuating soil moisture. Plant Production Science, 3, 197–207.
- Coelho, E. F., & Or, D. (1999). Root distribution and water uptake patterns of corn under surface and subsurface drip irrigation. Plant and Soil, 206, 123–136.
- Diagne, A., Midingoyi, S. G., Wopereis, M., & Akintayo, I. (2010). The NERICA success story: Development, achievements and lessons learned. (online). Retrieved from file:///C:/Users/Owner/Desktop/NERICA-Success-Story-11-2010.pdf.
- Douh, B., & Boujelben, A. (2011). Effects of surface and subsurface drip irrigation on agronomic parameters of maize (Zea mays L.) under Tunisian climatic condition. Journal of Natural Product and Plant Resources, 1, 8–14.
- Fukai, S., & Cooper, M. (1995). Development of drought-resistant cultivars using physio-morphological traits in rice. Field Crops Research, 40, 67–86.
- Hamada, K., Yuge, K., Anan, M., Hirakawa, A., & Shinogi, Y. (2015). Evaluation of soil moisture condition and water saving effect under subsurface irrigation in Shimajiri Mahji soil fields. Journal of Food, Agriculture and Environment, 13, 54–59.
- Hirayama, M., Nemoto, H., & Hirasawa, H. (2007). Relation between root system and drought resistance in Japanese upland rice (Oryza sativa L.) varieties with medium to late maturing under field conditions. Japanese Journal of Crop Science, 76, 245–252. (in Japanese with English abstract).
- Ishizuka, Y., & Tanaka, A. (1962). Studies on the position of fertilizer application in rice. Japanese Journal of Soil Science and Plant Nutrition, 33, 88–92. Tokyo: Crop Science Society of Japan. (in Japanese).
- Kameoka, E., Suralta, R. R., Mitsuya, S., & Yamauchi, A. (2015). Matching the expression of root plasticity with soil moisture availability maximizes production of rice plants grown in an experimental sloping bed having soil moisture gradients. Plant Production Science, 18, 267–276.
- Kameoka, E., Suralta, R. R., Mitsuya, S., & Yamauchi, A. (2016). Developmental plasticity of rice root system grown under mild drought stress condition with shallow soil depth; Comparison between nodal and lateral roots. Plant Production Science, 19, 411–419.
- Kano-Nakata, M., Suralta, R. R., Niones, J. M., & Yamauchi, A. (2012). Root sampling by using a root box-pinboard method. In H. E. Shashidhar, A. Henry, & B. Hardy (Eds.), Methodologies for root drought studies in rice (pp. 3–8). Metro Manila: International Rice Research Institute.
- Kijima, Y., Sserunkuuma, D., & Otsuka, K. (2006). How revolutionary is the “NERICA revolution”? Evidence from Uganda. The Developing Economies, 44, 252–267.
- Kono, Y., & Yamauchi, A. (1996). VI. Ideotype of roots and soil. In A. Yamauchi (Ed.), Ideotype of plant root system (pp. 149–172). Tokyo: Hakuyusha Co. (in Japanese).
- Kono, Y., Tatsumi, J., & Kawamura, N. (1976). Studies on root structure and function in rice. V. Position of top-dressing and root development. Report of the Tokai Branch of Crop Science Society of Japan, 77, 5–11. (in Japanese).
- Kono, Y., Yamauchi, A., Nonoyama, T., Tatsumi, J., & Kawamura, N. (1987). A revised experimental system of root-soil interaction for laboratory work. Environmental Control in Biology, 25, 141–151.
- Materechera, S. A., Dexter, A. R., & Alston, A. M. (1991). Penetration of very strong soils by seedling roots of different plant species. Plant and Soil, 135, 31–41.
- Menge, D. M., Kameoka, E., Kano-Nakata, M., Yamauchi, A., Asanuma, S., Asai, H., … Makihara, D. (2016). Drought-induced root plasticity of two upland NERICA varieties under conditions with contrasting soil depth characteristics. Plant Production Science, 19, 389–400.
- Nakamoto, T. (1993). Effect of soil water content on the gravitropic behavior of nodal roots in maize. Plant and Soil, 152, 261–267.
- Nemoto, H., Suga, R., Ishihara, M., & Okutsu, Y. (1998). Deep rooted rice varieties detected through the observation of root characteristics using the trench method. Breeding Science, 48, 321–324.
- Niones, J. M., Inukai, Y., Suralta, R. R., & Yamauchi, A. (2015). QTL associated with lateral root plasticity in response to soil moisture fluctuation stress in rice. Plant and Soil, 391, 63–75.
- O’toole, J. C., & Bland, W. L. (1987). Genetic variation in crop plant root systems. Advances in Agronomy, 41, 91–145.
- Oyanagi, A. (1999). Soil conditions and root development. Journal of the Japanese Society of Soil Physics, 82, 3–9. (in Japanese with English abstract).
- Oyanagi, A., Sato, A., & Wada, M. (1992). Effect of water potential of culture medium on geotropic response of primary seminal root in Japanese wheat cultivars. Japanese Journal of Crop Science, 61, 119–123.
- Phene, C. J., Davis, K. R., Hutmacher, R. B., Bar-Yosef, B., Meek, D. W., & Misaki, J. (1991). Effect of high frequency surface and subsurface drip irrigation on root distribution of sweet corn. Irrigation Science, 12, 135–140.
- Saito, K., Asai, H., Zhao, D., Laborte, A. G., & Grenier, C. (2018). Progress in varietal improvement for increasing upland rice productivity in the tropics. Plant Production Science, 21, 145–158.
- Sasaki, T. (1932). A preliminary report on the form of the root system in rice plants. Proceedings of the Crop Science Society of Japan, 4, 200–225. (in Japanese with English summary).
- Serraj, R., McNally, K. L., Slamet-Loedin, I., Kohli, A., Haefele, S. M., Atlin, G., & Kumar, A. (2011). Drought resistance improvement in rice: An integrated genetic and resource management strategy. Plant Production Science, 14, 1–14.
- Suganuma, A., Mitsuya, S., & Yamauchi, A. (2012). Hydraulic conductance of rice root system in relation to heterorhizy in lateral roots. Abstracts of the 233rd Meeting of the Crop Science Society of Japan (pp. 216–217). (in Japanese).
- Suralta, R. R., Inukai, Y., & Yamauchi, A. (2010). Dry matter production in relation to root plastic development, oxygen transport, and water uptake of rice under transient soil moisture stresses. Plant and Soil, 332, 87–104.
- Tanakamaru, S., Hayashida, S., Mochizuki, T., & Furuya, T. (1998). Specific difference in root penetration into the compacted soil cakes in crop plants. Japanese Journal of Crop Science, 67, 63–69. (in Japanese with English abstract).
- Uga, Y. (2013). Genetic improvement of drought resistance using gene associated with deep rooting in rice. Root Research, 22, 131–139. (in Japanese with English abstract).
- Uga, Y., Sugimoto, K., Ogawa, S., Rane, J., Ishitani, M., Hara, N., … Yano, M. (2013). Control of root system architecture by DEEPER ROOTING 1 increases rice yield under drought conditions. Nature Genetics, 45, 1097–1102.
- Yamauchi, A., Inukai, Y., Kano, M., Suralta, R., Ogawa, A., Kobayashi, N., & Serraj, R. (2008). Role of roots for stabilizing rice production under water stress conditions. In International Crop Science Society (Ed.). Proceedings of the 5th international crop science congress (pp. 178–179). Jeju: International Crop Science Society.
- Yamauchi, A., Kono, Y., & Tatsumi, J. (1987). Quantitative analysis on roots system structures of upland rice and maize. Japanese Journal of Crop Science, 56, 608–617.
- Yoshida, S., & Hasegawa, S. (1982). The rice root system: Its development and function. In International Rice Research Institute (Ed.), Drought resistance in crops with emphasis on rice (pp. 97–114). Los Banos: International Rice Research Institute.
- Zheng, H. G., Babu, R. C., Pathan, M. S., Ali, L., Huang, N., Courtois, B., & Nguyen, H. T. (2000). Quantitative trait loci for root-penetration ability and root thickness in rice: Comparison of genetic backgrounds. Genome, 43, 53–61.