ABSTRACT
Knowledge on the existing root diversity in germplasms has been emphasized for soybean breeding to improve drought resistance. Yet, evaluation of root diversity for vast genotypes still remains challenging. Here we investigated the contribution of different genotypic roots to drought resistance by a grafting experiment. A total of 22 cultivars were used as rootstocks to graft with scions of drought-sensitive cultivar L21. Water withholding was imposed during 15 days at podding stage in pot-culture experiments. The root-related traits were measured under well-watered (WW) and water-stressed (WS) conditions. At maturity, the drought-resistance coefficient (DRC) was calculated as the yield under WS condition relative to WW yield. The DRC varied from 0.56 to 0.85 among grafts; thus, the rootstocks were categorized as drought-sensitive (DS), moderate (M) and drought-resistant (DR) types. The L21 scion grafted onto DR rootstocks resulted in higher DRC and greater drought resistance than those self-grafts. There were also significant interactions between water stress and genotype for root-related traits. With the increase of DRC, the plants showed linear increases in root length, root surface area, and root volume, but had linear decreases in root dry mass, root–shoot ratio and root tissue density under WS rather than WW conditions. We suggested that developed rooting with a low investment on dry matter identified by grafting experiments should be selected to improve drought resistance in soybean breeding.
Graphical abstract
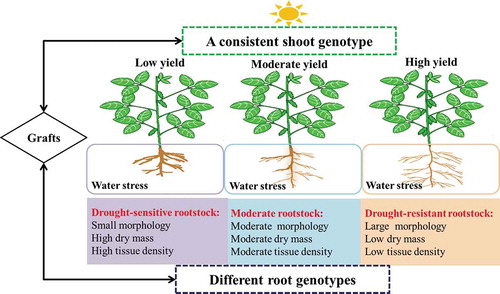
Introduction
Drought resistance in plants can be expressed by three major mechanisms: drought escape, drought tolerance, and dehydration avoidance (Levitt, Citation1980). Yield improvement through drought-resistance mechanisms decides by the drought regime (Blum, Citation2011). Enhancing the water uptake is as a focus for breeding due to its integrated compatibility with high yields (Blum, Citation2009). Water uptake maximization through a well-developed root system is a primary approach to accomplish drought avoidance in the presence of a water shortage (Tardieu, Citation2013). Roots that improved water uptake efficient can only be an advantage under WS condition if they are accompanied by appropriate shoot traits associated with high yields (Fenta et al., Citation2014; Lopes & Reynolds, Citation2011). The root morphological traits have been compared among different soybean (Glycine max L.) genotypes under WW and WS conditions (Fenta et al., Citation2014; Hudak & Patterson, Citation1996; Pantalone et al., Citation1996; Seversike et al., Citation2014). In general, the root types either penetrating deep into the soil and attaining greater ‘root mass at depth’ (Lopes & Reynolds, Citation2011) or roots with large xylem diameters and/or larger lateral root systems with more root hairs are advantageous under WS condition (Tanaka et al., Citation2014; Vadez, Citation2014).
Genetic resources having stronger water uptake via robust root system should be focused as valuable sources for drought-resistance improvement (Nakhforoosh et al., Citation2014). The vast diversity of soybean germplasms worldwide provides useful resources for identification of root traits (Manavalan et al., Citation2009). However, due to the difficulty in observing and measuring roots in situ, evaluation of root diversity for vast genotypes still remains challenging. Classic root phenotyping approaches including analysis of soil cores and applying standard excavation techniques to determine root traits were still the best methods of choice (Fenta et al., Citation2014; Manavalan et al., Citation2010; Trachsel et al., Citation2011). In addition, the diversity evaluation in soybean was predominantly based on the single root-related traits rather than root functions. As we knew, the drought resistance of plants is determined not only by water absorption capacity of roots, but also by the stomatal closure, osmotic adjustment, and defense enzyme activities of leaves (Luo, Citation2010; Nguyen et al., Citation1997). It was difficult to accurately evaluate the root contributions to drought resistance among vast soybean genotypes due to the interaction between root and shoot.
To determine the root contributions to drought resistance, it was critical to eliminate interference of shoot effects by ensuring a consistent genotypic shoot. Grafting to connect scion and rootstock of differing soybean genotypes has been used in previous studies (Li et al., Citation2017a, b, Citation2019), which could be helpful for this study. In previous study, the soybean cultivars Liaodou21 (L21) were categorized as drought-sensitive types based on their ability to maintain biomass and grain yield under drought condition (Yan et al., Citation2018). In present study, the L21 was used as common scions to graft onto the rootstocks of 22 soybean genotypes, in order to (1) evaluate the contributions of different rootstocks to drought resistance, and (2) determine the connection between root-related traits and drought resistance.
Materials and methods
Plant material and grafting procedure
A set of 22 soybean cultivars, released from Liaoning Province, China, were collected (Table S1) and used as rootstocks in the grafting experiments, respectively. The drought-sensitive cultivar L21 was used as common scions grafted onto above rootstocks of 22 genotypes, respectively. All cultivars tested were maturity group III. Selected soybean seeds were planted in plastic pots (12 cm×12 cm ×12 cm) with soil. Grafting was initiated at the cotyledon stage (VC), and the procedure was described in the reference (Pantalone et al., Citation1999). Immediately after grafting, the grafted plants were placed in a glasshouse, and the seedling management was described in the previous study (S.Y. Li et al., Citation2017b).
Pot-culture experiments
The pot-culture experiments were conducted under open field with auto-rain-shelter conditions in 2017 and 2018 at Liaoning Academy of Agricultural Sciences (41º83ʹ N, 123º56ʹ E), Shenyang, China. The survival grafts were transplanted into the pots (30 cm×30 cm×25 cm, 15.0 kg soil). The soil was loam derived from the 0 to 20 cm surface layer of field and sieved out. The field water-holding capacity was 26.5%, which was measured from above layer by gravimetric method. The physical and chemical characteristics of soil were shown in Table S2. Under WW condition, the soil moisture content was maintained at 80% of the field water-holding capacity in whole growing season. Under WS condition, the soil moisture content was maintained at 50% of the field water-holding capacity during 15 days at podding stage (about 80 days after germination). According to the plant water status commonly used to indicate the degree of water stress (Hsiao, Citation1973), the WS treatment in this study was considered as the moderate stress. After water withholding, six pots per treatment were used to measure the root-related traits, while remaining six pots were maintained at ~80% of the field water-holding capacity until maturity to measure the grain yield. The water content of soil was measured by weighing method at 16:00 every day and replenished water to the set value. There was a randomized complete block design with 12 replications (pots), and each pot contained two plants.
Root-related traits
At 15–16 days after drought treatment, root-related traits were measured in six pots (n = 6) under WW and WS conditions, respectively. The roots were washed thoroughly with water from a pot. The cleaned root samples of each pot (two plants) were scanned by root scanning image analysis system WinRHIZO Pro 2012b (Regent Instruments, Inc., Quebec, Canada) to calculate the root length, root surface area, and root volume. Then, the samples of roots were immediately oven-dried at 105°C for 30 min and then at 85°C until constant weight. Simultaneously, the remaining samples of shoots were also dried to a constant weight. The root–shoot ratio and root tissue density were calculated as follows. Root–shoot ratio = root dry mass/shoot dry mass; Root tissue density (g cm−3) = root dry mass/root volume.
Grain yield and drought-resistance coefficient
At maturity (R8), remaining six pots (n = 6) were individually harvested to measure the grain yield. These traits under WW and WS conditions were used to calculate the drought-resistance coefficient (DRC) according to the following equations. DRC = Xws/Xww. The Xws and Xww were the values of measured grain yield under WS and WW conditions, respectively.
Statistical analysis
Data analysis was conducted with an analysis of variance in general linear model by SPSS 19.0 (SPSS, Inc., Chicago. USA). Genotype (22 grafts), water stress (WW and WS) and genotype × water stress were as fixed effects. Years, along with the genotype × year, water stress × year, and genotype × water stress × year were regarded as a random factor. Means were subjected to the least significant difference (LSD) test at the P < 0.05 level. The between-groups linkage method was conducted in cluster analysis for DRC of different grafts. Pearson’s product moment correlations and regression coefficients between root traits and DRC were determined under WW and WS treatments.
Results
Effect of grafting on grain yield and root-relate traits
There was no difference between self-grafted and non-grafted L21 in grain yield and root-relate traits under WW and WS conditions (Fig. S1). The grafting procedure was carried out at cotyledon stage, when the hypocotyls had a stronger ability to form the callus and joint of vascular system between scions and rootstocks. The relatively short time (about 10 d) for seedling recovering resulted in non-significant influence of grafting procedure on growth and development of soybean plants. Thus, self-grafts were therefore used as controls to analyze the effect of different soybean rootstocks on grain yield and DRC of L21 under WW and WS conditions.
Grain yield of different grafts respond to water stress
Analysis of variance showed significant difference of grain yield among grafts (P < 0.001, ). As all grafts had a consistent genotypic scion L21, the difference of grain yield was only attributed to the effects of different genotypic rootstock. Grain yield for each grafted plants was plotted in WW vs. the grain yield in WS ()). Under WW condition, the L21 scions grafted onto rootstocks of three genotypes (L24, L28 and L38) had significantly lower yield than those self-grafts, while they grafted onto rootstocks of seven genotypes (T54, L15, L32, L47, L34, L26 and L14) had significant higher yield than their self-grafts. Under WS condition, the L21 scions grafted onto rootstocks of L38 had significant lower yield than those self-grafts, while they grafted onto rootstocks of five genotypes (T62, L15, T54, L34 ,and L14) had significant higher yield than their self-grafts.
Figure 1. Interaction plot (a) for grain yield for 22 grafts under well-watered (WW) and water-stressed (WS) conditions, and box plot (b) for drought-resistance coefficient (DRC) of grafts with drought-sensitive (DS), moderate (M) and drought-resistant (DR) type rootstocks. The least significant difference (α = 0.05) between water stress and genotype for grain yield was 3.38 g plant−1. The red-dashed line drawn diagonally across the plot bisects the high and low values, with the intercept constrained to zero; the solid line is the least squares regression line. Dotted lines flank the 95% confidence interval. The horizontal and vertical dashed lines indicate the yield of self-grafted L21 (check) under well-watered (WW) and water-stressed (WS) conditions. Boxes are quartiles, continuous lines are medians, cwhiskers include others, that are not outliers
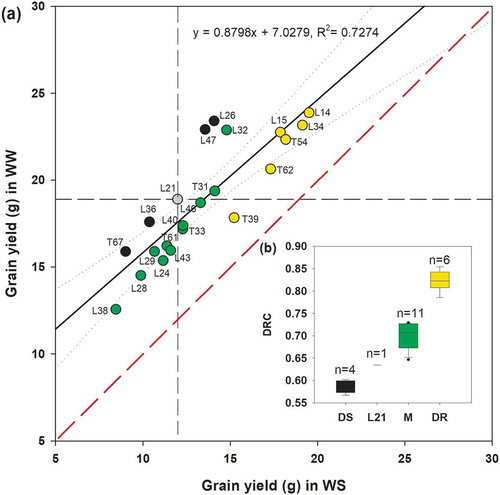
Table 1. Analysis of variance (mean square and significance) of traits evaluated in 2017 and 2018
Analysis of variance also showed significant effect of water stress on grain yield (P < 0.05, ). Grain yield for all grafts fell above the bisect line ()), illustrating that all grafts showed decreased grain yield by water stress. However, the grain yield of grafts showed differential responses to water stress due to its significant interaction between water stress and genotype (). In ), the relative low responsive genotypes to water stress would be closest to the bisecting line, while the drought-sensitive genotypes would be far away from the bisecting line. To evaluate differential response to water stress, the DRC of each graft were calculated from grain yield under WW and WS conditions, which were varied from 0.56 to 0.85. The grafts were classified into three groups by the cluster analysis for DRC (Fig. S2). Because these grafts had a consistent scion of L21, the rootstocks in three groups were accordingly identified as drought-sensitive (DS), moderate (M) and drought-resistant (DR) types, respectively ()). The grafts with DS rootstocks (T67, L26, L47, and L36) showed DRC from 0.57 to 0.60; those with M rootstocks (L32, L29, L38, L28, T61, L40, L46, T33, L24, L43, and T31) showed DRC from 0.65 to 0.73; those with DR rootstocks (L15, T54, L14, L34, T62, and T39) showed DRC from 0.79 to 0.85. Both M and DR rootstocks resulted in higher DRC of plants than L21 rootstock (0.64).
Relationship between root morphology and drought resistance
There were significant differences of root length (P < 0.001), root surface area (P < 0.001) and root volume (P < 0.01) among grafts (). The effects of water stress on root length, root surface area and root volume were non-significant (P > 0.05), but their interactions between water stress and genotype were significant (P < 0.05). The DS rootstocks presented here exhibited decreased trends in root length, root surface area and root volume in response to water stress, while DR rootstocks exhibited increased trends (). The root length, root surface area, and root volume had significantly positive relationships with DRC under WS rather than WW conditions. The regression coefficients of root length, root surface area and root volume on DRC under WS conditions were higher than those under WW conditions.
Figure 2. Relationships of root length (a), root surface area (b) and root volume (c) with drought-resistance coefficient (DRC) under well-watered (WW) and water-stressed (WS) conditions. DS, drought-sensitive; M, moderate; DR, drought-resistant. The error bar indicates the least significant difference (P < 0.05) between drought treatment × genotype (n = 12). **, Significant at the 0.01 probability levels
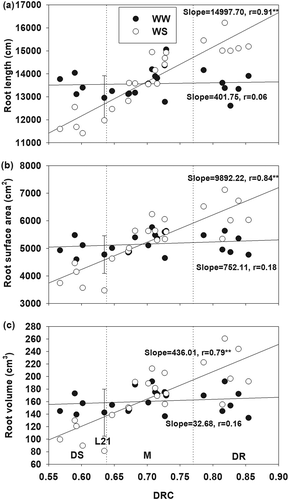
Relationship between root biomass and drought resistance
There were significant differences of root dry mass, root–shoot ratio and root tissue density among grafts (P < 0.01, ). The root–shoot ratio of grafts showed differential responses to water stress due to its significant interaction between water stress and genotype (P < 0.001, ). Under water stress imposed, the root dry mass of DS, M, and DR rootstocks were increased by 32.40%, 18.20% and 11.38%, respectively ()); the root–shoot ratio were thus increased by 104.70%, 65.74% and 42.24% after grafting onto DS, M and DR rootstocks, respectively ()). Under WW condition, the DRC showed a significantly positive relationship with root dry mass, but had no relationships with shoot dry mass and root–shoot ratio. Under WS condition, the DRC had significantly negative relationships with root dry mass and root–shoot ratio. The regression coefficients of root dry mass and root–shoot ratio on DRC under WS condition were lower than those under WW condition.
Figure 3. Relationships of root dry mass (a), root-shoot ratio (b) and root tissue density (c) with drought resistance coefficient (DRC) under well-watered (WW) and water-stressed (WS) conditions. DS, drought-sensitive; M, moderate; DR, drought-resistant. The error bar indicates the least significant difference (P < 0.05) between drought treatment × genotype (n = 12). **, Significant at the 0.05 probability levels
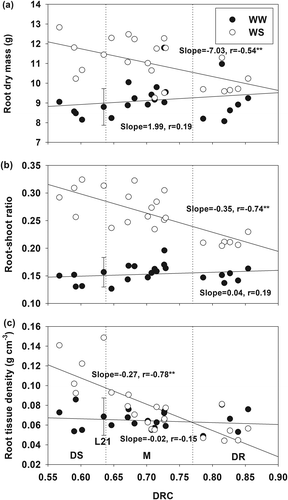
Interestingly, the DS rootstocks had lower root volume than the DR ones under WS condition ()), although they showed larger root dry mass ()). By water stress, the root tissue density of DS rootstocks showed increased trends, but that of DR rootstocks had decreased trends ()). The root tissue density showed significantly negative relationship with DRC under WS rather than WW conditions. The regression coefficient of root tissue density on DRC under WS condition was lower than that under WW condition.
Discussion
In our grafting experiment, the rootstocks of different genotypes were grafted with a consistent shoot genotype, and then all root systems were assumed to be the ‘black box’. The differences of grafts in response to drought were only attributed to the effects of different root genotypes. Based on their contributions to maintain grain yield under WS condition, the rootstocks were categorized as DS, M, and DR types, respectively. Grafting onto DR rootstocks could improve the grain yield under WS condition, although the shoot genotype L21 was sensitive to drought. Improvement of drought resistance by using of grafting to alter the root genotypes has been reported in modern vegetable production (Kumar et al., Citation2017). Although the grafting technology was difficult to realize the application in soybean production under field conditions, it could provide a feasible way to screen the DR roots among germplasms, which had valuable resources for drought-resistance improvement.
Among the cultivars used as DR rootstocks in this study, the Liaodou15 (L15) and Liaodou14 (L14) had wide adaptability and high yield in soybean production. For instance, the L15 was one of currently adapted cultivars in soybean production regions in China, which had larger extending area of more than 0.98 million ha from 2004 to 2019. The L14 had produced the highest yield records of 4, 908 kg/ha in Northeast China soybean production regions (song et al., Citation2001), is therefore known as the record-yield cultivars in China. The L14 was used as rootstock in previous study could lead to an increase of grain yield, especially for the modern cultivars (Li et al., Citation2017a). The present study suggested that the important cause of wide adaptability and high yield for L15 and L14 was the superiority of their roots.
The effects of drought on root morphology of soybean have been reported in many studies. Soybean plants have the robust ability to increase root growth at the early stage of drought stress to absorb the water in deep soil (Hirasawa et al., Citation1994). Non-irrigated soybeans showed an increase in root length when compared with irrigated plants (Huck et al., Citation1983). Decreased root lengths have been also reported in many soybean accessions under drought conditions (Thu et al., Citation2014). Under drought imposed, the root length, root surface, and root volume were decreased in the DS soybean cultivar, but were greatly increased in the drought-escaping or intermediate drought-tolerant cultivar (Fenta et al., Citation2014). The DS rootstocks presented here exhibited decreased trends in root length, root diameter, root surface area and root volume in response to WS treatment, while DR rootstocks exhibited increased trends. In addition, significant correlations existed in soybean between drought resistance and various root traits such as total length, volume, and number of lateral roots (Liu et al., Citation2005). In the present study, the root morphological traits had significantly positive correlations with the DRC under WS rather than WW conditions. These indicated that the roots could maintain rapid growth and larger morphology under water stress imposed, which would be important for drought-resistant improvement.
Drought not only changes root morphology but also partitioning of root to shoot biomass with an increase in root mass (Fenta et al., Citation2014). The grafted plants in the study showed increases in root dry mass and root–shoot ratio in response to drought by stimulating or maintaining root growth while reducing shoot growth. The contrasting growth behaviors between root and shoot determined the difference in sensitivity to water deficit (Hsiao & Xu, Citation2000). Increased root versus shoot growth could improve hydraulic status under WS condition as the improvement of plant capacity for acquiring water to support existing shoots (Comas et al., Citation2013). However, the DR rootstocks in the study had lower root dry mass and root–shoot ratio than the DS ones under WS condition. The negative correlation between root-shoot ratio and yield has been reported under high soil resource levels (Zhu et al., Citation2019a). The yield and water-use efficiency of winter wheat could be increased by root pruning in water-limited environments (Fang et al., Citation2010a). It is possible that the lowering root-shoot ratio could increase the water utilization efficiency and grain yield in arid and semiarid areas by decreasing its competitive ability and improving root efficiency (Ma et al., Citation2010). Reducing or eliminating the response of root proliferation to root interactions would be further potential to increase yields (Fang et al., Citation2010b; Zhu et al., Citation2019b). Interestingly, the DS rootstocks had lower root volume than the DR ones under WS condition, although they showed larger root dry mass. Root tissue density, defined as the amount of structural material invested by unit of root volume, has been increasingly used as an indicator of resource use strategy of plant (Birouste et al., Citation2014). The present study showed a negative correlation between DRC and root tissue density under WS condition. These indicated that the low density of DR rootstocks could enable a fast relative growth rate and a rapid resource acquisition with a low investment on dry matter under water deficit. However, as only one cultivar of scions and one soil type were evaluated for grafting experiments, further studies are needed to determine whether other cultivars of scions and other soil types would have similar results to this study.
Conclusion
Integration of root traits into breeding programs requires an understanding of not only root morphological diversity but also root functional diversity in soybean germplasms. Our grafting experiment provided an effective and applicable approach to assess the root functions and effects of different genotypes. Based on the grafting technique, six roots of DR genotypes were screened that could improve the photosynthesis and yield under WS condition. The present study further clarified the root characteristics that could contribute to the great drought resistance of whole plants. The DR roots had large morphology, but low root dry mass and root tissue density under WS condition. Our results suggested that developed rooting with a low investment on dry matter should be selected to improve drought resistance in soybean breeding.
PPS2020_064RP-File008.docx
Download MS Word (20.6 KB)Disclosure statement
No potential conflict of interest was reported by the authors.
Supplementary material
Supplemental data for this article can be accessed here.
Additional information
Funding
References
- Birouste, M., Zamora-Ledezma, E., Bossard, C., Pérez-Ramos, I. M., & Roumet, C. (2014). Measurement of fine root tissue density, a comparison of three methods reveals the potential of root dry matter content. Plant and Soil, 374(1–2), 299–313. https://doi.org/10.1007/s11104-013-1874-y
- Blum, A. (2009). Effective use of water (EUW) and not water-use efficiency (WUE) is the target of crop yield improvement under drought stress. Field Crops Research, 112(2–3), 119–123. https://doi.org/10.1016/j.fcr.2009.03.009
- Blum, A. (2011). Drought resistance and its improvement. Plant breeding for water-limited environments. Springer.
- Comas, L., Becker, S., Cruz, V. M. V., Byrne, P. F., & Dierig, D. A. (2013). Root traits contributing to plant productivity under drought. Frontiers in Plant Science, 4, 442. https://doi.org/10.3389/fpls.2013.00442
- Fang, Y., Xu, B., Turner, N. C., & Li, F. (2010a). Does root pruning increase yield and water-use efficiency of winter wheat? Crop and Pasture Science, 61(11), 899–910. https://doi.org/10.1071/CP10125
- Fang, Y., Xu, B. C., Turner, N. C., & Li, F. M. (2010b). Grain yield, dry matter accumulation and remobilization, and root respiration in winter wheat as affected by seeding rate and root pruning. European Journal of Agronomy, 33(4), 257–266. https://doi.org/10.1016/j.eja.2010.07.001
- Fenta, B., Beebe, S., Kunert, K., Burridge, J., Barlow, K., Lynch, J., & Foyer, C. (2014). Field phenotyping of soybean roots for drought stress tolerance. Agronomy, 4(3), 418–435. https://doi.org/10.3390/agronomy4030418
- Hirasawa, T., Tanaka, K., Miyamoto, D., Takei, M., & Ishihara, K. (1994). Effects of pre-flowering moisture deficits on dry matter production and ecophysiological characteristics in soybean plants under drought conditions during grain filling. Japanese Journal of Crop Science, 63(4), 721–730. https://doi.org/10.1626/jcs.63.721
- Hsiao, T. C. (1973). Plant responses to water stress. Annual Review of Plant Physiology, 24(1), 519–570. https://doi.org/10.1146/annurev.pp.24.060173.002511
- Hsiao, T. C., & Xu, L. K. (2000). Sensitivity of growth of roots versus leaves to water stress, biophysical analysis and relation to water transport. Journal of Experimental Botany, 51(350), 1595–1616. https://doi.org/10.1093/jexbot/51.350.1595
- Huck, M. G., Ishihara, K., Peterson, C. M., & Ushijima, T. (1983). Soybean adaptation to water stress at selected stages of growth. Plant Physiology, 73(2), 422–427. https://doi.org/10.1104/pp.73.2.422
- Hudak, C. M., & Patterson, R. P. (1996). Root distribution and soil moisture depletion pattern of a drought-resistant soybean plant introduction. Agronomy Journal, 88(3), 478–485. https://doi.org/10.2134/agronj1996.00021962008800030020x
- Kumar, P., Rouphael, Y., Cardarelli, M., & Colla, G. (2017). Vegetable grafting as a tool to improve drought resistance and water use efficiency. Frontiers in Plant Science, 8, 1130. https://doi.org/10.3389/fpls.2017.01130
- Levitt, J. (1980). Responses of plants to environmental stresses. Volume II. Water, radiation, salt, and other stresses. Academic Press.
- Li, S., Teng, F., Rao, D., Yao, X., Zhang, H., Wang, H., Song, S., St. Martin, S., & Xie, F. (2017a). Agronomic traits of soybean cultivars released in different decades after grafting record-yield cultivar as rootstock. Plant Breeding, 136(2), 133–138. https://doi.org/10.1111/pbr.12436
- Li, S., Wang, W., Cao, Y., Wang, C., Yan, C., Dong, L., Wu, L., Xie, F., & Song, S. (2019). How root traits would be affected by soybean yield improvement? An examination of historical cultivars grafted with record-yield cultivar scion. Plant and Soil, 439(1–2), 19–30. https://doi.org/10.1007/s11104-018-3792-5
- Li, S. Y., Teng, F., Rao, D. M., Zhang, H. J., Wang, H. Y., Yao, X. D., Yu, C. M., Li, C. H., Zhao, M. Z., St. Martin, S., & Xie, F. T. (2017b). Photosynthesis of soybean cultivars released in different decades after grafting onto record-yield cultivars as rootstocks. Photosynthetica, 55(4), 579–587. https://doi.org/10.1007/s11099-016-0666-z
- Liu, Y., Gai, J. Y., Lu, H. N., Wang, Y. J., & Chen, S. Y. (2005). Identification of drought tolerant germplasm and inheritance and QTL mapping of related root traits in soybean (Glycine max (L.) Merr.). Acta Genetica Sinica, 32(8), 855–863. (in Chinese). https://doi.org/10.1016/0008-6215(90)80036-3
- Lopes, M. S., & Reynolds, M. P. (2011). Drought adaptive traits and wide adaptation in elite lines derived from resynthesized hexaploid wheat. Crop Science, 51(4), 1617–1626. https://doi.org/10.2135/cropsci2010.07.0445
- Luo, L. J. (2010). Breeding for water-saving and drought-resistance rice (WDR) in China. Journal of Experimental Botany, 61(13), 3509–3517. https://doi.org/10.1093/jxb/erq185
- Ma, S. C., Li, F. M., Xu, B. C., & Huang, Z. B. (2010). Effect of lowering the root/shoot ratio by pruning roots on water use efficiency and grain yield of winter wheat. Field Crops Research, 115(2), 158–164. https://doi.org/10.1016/j.fcr.2009.10.017
- Manavalan, L. P., Guttikonda, S. K., Nguyen, V. T., Shannon, J. G., & Nguyen, H. T. (2010). Evaluation of diverse soybean germplasm for root growth and architecture. Plant and Soil, 330(1–2), 503–514. https://doi.org/10.1007/s11104-009-0222-8
- Manavalan, L. P., Guttikonda, S. K., Tran, L. S. P., & Nguyen, H. T. (2009). Physiological and molecular approaches to improve drought resistance in soybean. Plant and Cell Physiology, 50(7), 1260–1276. https://doi.org/10.1093/pcp/pcp082
- Nakhforoosh, A., Grausgruber, H., Kaul, H. P., & Bodner, G. (2014). Wheat root diversity and root functional characterization. Plant and Soil, 380(1-2), 211–229. https://doi.org/10.1007/s11104-014-2082-0
- Nguyen, H. T., Babu, R. C., & Blum, A. (1997). Breeding for drought resistance in rice, physiology and molecular genetics considerations. Crop Science, 37(5), 1426–1434. https://doi.org/10.2135/cropsci1997.0011183X003700050002x
- Pantalone, V. R., Rebetzke, G. J., Burton, J. W., & Carter, T. E. (1996). Phenotypic evaluation of root traits in soybean and applicability to plant breeding. Crop Science, 36(2), 456–459. https://doi.org/10.2135/cropsci1996.0011183X003600020039x
- Pantalone, V. R., Rebetzke, G. J., Burton, J. W., Carter, T. E., & Israel, D. W. (1999). Soybean PI416937 root system contributes to biomass accumulation in reciprocal grafts. Agronomy Journal, 91(5), 840–844. https://doi.org/10.2134/agronj1999.915840x
- Seversike, T. M., Sermons, S. M., Sinclair, T. R., Carter, T. E., & Rufty, T. W. (2014). Physiological properties of a drought-resistant wild soybean genotype, Transpiration control with soil drying and expression of root morphology. Plant and Soil, 374(1–2), 359–370. https://doi.org/10.1007/s11104-013-1757-2
- Song, S. H., Wang, W. B., & Lu, G. L. (2001). Research on technology for super high yielding in spring soybean. Chinese J. Oil Crops Sciences, 23, 48–50. ( in Chinese). https://doi.org/10.3321/j.issn:1007-9084.2001.04.012
- Tanaka, N., Kato, M., Tomioka, R., Kurata, R., Fukao, Y., Aoyama, T., & Maeshima, M. (2014). Characteristics of a root hair-less line of Arabidopsis thaliana under physiological stresses. Journal of Experimental Botany, 65(6), 1497–1512. https://doi.org/10.1093/jxb/eru014
- Tardieu, F. (2013). Plant response to environmental conditions, assessing potential production, water demand, and negative effects of water deficit. Frontiers in Physiology, 4, 17. https://doi.org/10.3389/fphys.2013.00017
- Thu, N. B. A., Nguyen, Q. T., Hoang, X. L. T., Thao, N. P., & Tran, L. S. P. (2014). Evaluation of drought tolerance of the Vietnamese soybean cultivars provides potential resources for soybean production and genetic engineering. BioMed Research International. 2014,809736. http://dx.doi.org/10.1155/2014/809736
- Trachsel, S., Kaeppler, S. M., Brown, K. M., & Lynch, J. P. (2011). Shovelomics: High throughput phenotyping of maize (Zea mays L.) root architecture in the field. Plant and Soil, 341(1–2), 75–87. https://doi.org/10.1007/s11104-010-0623-8
- Vadez, V. (2014). Root hydraulics, the forgotten side of roots in drought adaptation. Field Crops Research, 165(3), 15–24. https://doi.org/10.1016/j.fcr.2014.03.017
- Yan, C., Wang, W., Cao, Y., Sun, X., Song, S., Wang, C., & Zhang, L. (2018). Response of physiological characteristics of different drought-tolerant soybean varieties to different rainfall climatic conditions. Soybean Science, 37(3), 359–368. (in Chinese). https://doi.org/10.11861/j.issn.1000-9841.2018.03.0359
- Zhu, Y. H., Weiner, J., & Li, F. M. (2019b). Root proliferation in response to neighbouring roots in wheat (Triticum aestivum). Basic and Applied Ecology, 39, 10–14. https://doi.org/10.1016/j.baae.2019.07.001
- Zhu, Y. H., Weiner, J., Yu, M. X., & Li, F. M. (2019a). Evolutionary agroecology, Trends in root architecture during wheat breeding. Evolutionary Applications, 12(4), 733–743. https://doi.org/10.1111/eva.12749