ABSTRACT
Recent advances in rice breeding in tropical Asia led to the release of high-yielding drought-tolerant cultivars. Together with the use of these cultivars, improved nutrient management under drought should further increase rice productivity in rainfed lowland ecosystems. The objectives of this study were to evaluate cultivar differences in the responses of plant growth and grain yield to fertilizer application regimes. We compared 13 drought-tolerant rice cultivars with two irrigated rice cultivars under three nutrient management conditions in irrigated and rainfed lowlands in the Philippines during the wet seasons of 2014 and 2015. Drought stress was mild in both years, with a yield reduction of 11 to 12%, and there was no significant cultivar × nutrient management interaction in yield. The drought-tolerant cultivar NSIC Rc282 proved fertilizer-responsive, and had similar yield to those of the popular high-yielding cultivar NSIC Rc222 under all conditions. An ancillary experiment in 2016 confirmed that NSIC Rc282 was more drought-adapted than NSIC Rc222, with 11% to 37% higher yield under stress. In order to maximize the yield, NSIC Rc282 required N application only when the drought ended, whereas NSIC Rc222 required additional N during the drought. This shows that the details of drought-adaptive nutrient management differ between irrigated and drought-tolerant rice cultivars. We suggest that the introduction of high-yielding drought-tolerant rice cultivars will both improve productivity and increase the nutrient-use efficiency in rainfed environments.
GRAPHICAL ABSTRACT
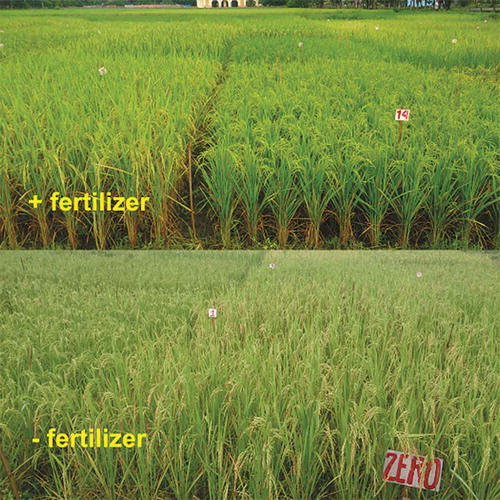
Introduction
About 45 × 106 ha of rainfed lowland rice grows in tropical Asia, where drought is a major constraint on crop production (Haefele et al., Citation2016). Rainfed lowland rice ecosystems are characterized by a high degree of heterogeneity in water and nutrient availability (Fukai & Ouk, Citation2012). Most farmers in the area are resource-poor smallholders, and thus any intervention in agricultural technologies should be designed to enhance both rice productivity and resource-use efficiency.
Rice productivity in irrigated ecosystems has increased owing to the combined use of modern semi-dwarf cultivars and high inputs of N fertilizers. However, these high-yielding cultivars are often not suitable for drought-prone environments and suffer yield losses when a soil water deficit develops (Haefele et al., Citation2016). Several modern drought-tolerant cultivars have been released in tropical Asia during the last two decades, and can significantly reduce the risk of yield loss due to drought in rainfed lowlands (Kumar et al., Citation2015). On-farm studies showed yield advantages of drought-tolerant cultivars compared with popular irrigated rice cultivars in rainfed ecosystems (Atlin et al., Citation2017; Ohno et al., Citation2018).
In addition, improved fertilizer management, known as ‘site-specific nutrient management’, was developed for use in tropical Asia, where the fertilizer requirements were estimated from the soil indigenous nutrient supply and crop demand (Dobermann et al., Citation2002). This approach has been extensively assessed in on-farm trials during the last two decades (Buresh et al., Citation2019; Pampolino et al., Citation2007). These studies show the higher N fertilizer-use efficiency (yield increase per kg of N applied) in this practice compared with the conventional practice, and thus increased farmer income by more than 100 USD ha−1 throughout tropical Asia (Banayo et al., Citation2018; Sansen et al., Citation2019).
Accordingly, the choice of fertilizer-use efficient cultivars under drought appears to be the key for increasing rice productivity by improved fertilizer management in rainfed lowlands (Haefele et al., Citation2016). In drought-adaptive fertilizer management, Prasertsak and Fukai (Citation1997) showed the importance of N application for the quick recovery of rice growth upon the cessation of drought during the vegetative and early reproductive stages. Inthapanya et al. (Citation2000) showed a non-significant interaction between cultivar and fertilizer management in grain yield in a rainfed lowland experiment in Laos. However, a cultivar’s yield response to drought (i.e. its drought sensitivity) may affect its response to fertilizer under rainfed conditions. In other words, the cultivar × nutrient management interaction may depend on the severity of the drought and a cultivar’s ability to tolerate that severity (Wade et al., Citation1999). Therefore, a question to be answered is whether drought-tolerant cultivars are also fertilizer-responsive and fertilizer-use efficient in drought-prone lowlands. If this will not be the case, the generic recommendation for fertilizer management can be simply applied to any cultivar in rainfed environments.
The Philippines is one of the countries in tropical Asia where rice is widely grown in rainfed ecosystems. Rainfed lowland rice occupies 1.3 × 106 ha, ⅓ of the total rice area. Farmer practice in rainfed lowlands is similar to that in irrigated areas, and there is no specific fertilizer management recommendation for the new drought-tolerant cultivars (Banayo et al., Citation2018). Our objectives were to characterize the cultivar differences in the response of grain yield and agronomic characteristics to fertilizer management. We evaluated new drought-tolerant cultivars and popular high-yielding cultivars in both irrigated and rainfed lowlands as well as under intermittent drought stress in the Philippines.
Materials and methods
Site description
Field experiments were conducted at Mariano Marcos State University in Batac, Ilocos Norte province, the Philippines (18°3′26″ N, 120°33′1″ E, 19 m a.s.l.), during the wet seasons (July to December) of 2014, 2015, and 2016. The soil (to a depth of 20 cm) consisted of 15% sand, 42% silt and 43% clay, and had a pH (H2O) of 7.9, 15.0 g total C kg−1, 1.2 g total N kg−1, 31 mg Bray-II P kg−1, 0.9 cmolc exchangeable K kg−1, and a cation exchange capacity of 45 cmolc kg−1. The mean air temperature was 27°C (minimum 22°C; maximum 32°C). The total rainfall during the wet seasons recorded at the meteorological station at the site was 979 mm in 2014, 1097 mm in 2015, and 924 mm in 2016. More than 90% of the rainfall was recorded from 0 to 60 days after transplanting (DAT) in all years.
Experimental design and field management
In 2014 and 2015, two lowland trials were set up: irrigated and rainfed regimes. In the irrigated regime the soil was kept submerged until 2 weeks before harvest, whereas in the rainfed regime light flush irrigations were applied before fertilizer application (see below). Bunds (15 cm in height, 20 cm in width) and canals (20 cm in width, 20 cm deep) were built around the main plots to prevent movement of fertilizers between adjacent plots. The size of each plot was 25 m2 (5 m × 5 m). In 2014, three nutrient management treatments were used (270 plots in total): (1) fixed nutrient management (FNM), (2) cultivar-specific nutrient management (CSNM), and (3) zero-input (control) as the main plots. We used 15 cultivars as the subplots, arranged in a split-plot design with three replicates in each water regime (See Supplementary Table S1 for details). Compound fertilizer (14% N as a mixture of ammonium sulfate and diammonium phosphate, 14% P2O5-equivalent as diammonium phosphate, and 14% K2O-equivalent as KCl), urea, single superphosphate and KCl were applied at transplanting to adjust the inputs of N, P2O5 and K2O to the target rate in each treatment.
The nutrient inputs in FNM were based on the standard farmer practices in the study area. Those in CSNM were adjusted to achieve 80% of the yield potential for each cultivar (Buresh et al., Citation2019), with the amounts determined by using a Web-based calculator for nutrient requirements (Rice Crop Manager: https://phapps.irri.org/ph/rcm/). Two cultivars (PSB Rc82 and NSIC Rc222) had been released for use in irrigated ecosystems, but the other 13 cultivars were released for use in rainfed lowland ecosystems; these comprised an old cultivar released in 1992 (PSB Rc14) and 12 cultivars released between 2009 and 2013. In 2015, three nutrient management treatments were arranged in a split-plot design with three replicates of each water regime (180 plots in total): (1) CSNM, (2) CSNM+P (in which the P input was fixed at a 60 kg ha−1 of P2O5-equivalent), and (3) control as the main plots, and 10 cultivars as the subplots (Supplementary Table S2). In CSNM+P, the P input was increased relative to the CSNM level, whereas the N and K inputs were the same as in CSNM, to alleviate the problem of low P availability under non-submerged soil conditions in rainfed lowlands (Kato et al., Citation2016). Ten of the 15 cultivars used in 2014 were evaluated again in 2015. In each plot, 21-day-old seedlings were transplanted at 2 or 3 seedlings per hill (20 cm × 20 cm) on 5 August 2014 and 3 August 2015. Weeds, diseases, and insects were carefully controlled by using registered chemicals according to standard local practices.
In 2016, two factors (N regime as the main plot and cultivar as the subplot) were arranged in a split-plot design with four replicates in two drought stress trials. Bunds and canals were built around the main plots as in the 2014 trial. The size of each plot was 25 m2 (5 m × 5 m). Though the dates of the onset and end of drought imposition were identical between the two trials, the transplanting dates were staggered: 24 August in one trial (period of drought 1; hereafter, PD1) and 20 September in trial 2 (period of drought 2; PD2). The aim was to impose drought for 31 days at different phenological stages in each trial. The drought period ran from 21 DAT to 52 DAT in PD1, and from 5 DAT to 36 DAT in PD2 (Supplementary Figure S1). Irrigation was resumed on 21 September, but water in the reservoir at the site ran out and the water table depth dropped below 80 cm after early October. Two cultivars (NSIC Rc222 and NSIC Rc282), which had high yield in the 2014 and 2015 trials, were grown under three N application regimes (N1, N2, and N3). Both cultivars had a similar growth duration (110 to 115 d). In all treatments, the same compound fertilizer used in 2014 and 2015 was applied at 2 DAT at 30 kg ha−1 of N, 30 kg ha−1 of P2O5-equivalent, and 30 kg ha−1 of K2O-equivalent. N1 received 0 kg N ha−1 at 21 DAT (mid-tillering) and 40 kg N ha−1 as urea at 37 DAT (panicle initiation), N2 received 40 and 0 kg N ha−1, and N3 received 40 and 40 kg N ha−1.
Measurements and data analysis
We recorded the date when 50% heading occurred and maturity. At maturity, rice plants were collected from three representative 0.16-m2 areas in each plot, and the number of panicles was counted. The panicles were detached from the straw and threshed by hand, and filled and unfilled grains were separated by submerging them in tap water and counted. We measured the dry weights of the filled and unfilled grains, rachides, and straw after oven-drying at 80°C for 72 h. Grain yield was determined from a 5-m2 area in each plot and expressed at a water content of 0.14 g H2O g−1 grain. We then determined the number of spikelets per panicle, the filled-grain percentage (100% × filled spikelets/total spikelet number), and 1000-grain weight. Percentage yield increase was calculated as (yield with fertilizer input – yield in control)/yield in control × 100.
In 2016, crop growth was also measured during the drought imposition and recovery periods. At 21, 37, 52, and 75 DAT, we collected six plants from each plot to determine the aboveground biomass and the leaf area index (LAI). We separated green leaves from dead leaves, stems, and panicles (if any), and measured the green leaf area with a leaf area meter (LI-3100, Li-COR, Lincoln, Nebraska, USA). The dry weight of each component was determined after oven-drying at 80°C for 3 days.
We performed analysis of variance (ANOVA) in each water regime for each year using a generalized linear model procedure of STAR v. 2.0.1 software, which is open-access software implemented in the R statistical software (http://bbi.irri.org). The effects of nutrient management, cultivar, and their interaction were assessed and the statistical significance was reported at P < 0.05. When the ANOVA result was significant, we compared pairs of values using Fisher’s least-significant-difference (LSD) test.
Results
Yield response to fertilizer inputs of new drought-tolerant cultivars
Grain yield in the rainfed regime was an average of 12% lower than that in the irrigated regime in 2014 for all cultivars combined (; 4.16 vs. 3.65 t ha−1). Yield differed significantly between treatments and cultivars, while percentage yield increase was significantly different on cultivar only. There was no significant cultivar × nutrient management interaction in either water regime. The yield increases compared with control were 78% in CSNM and 66% in FNM in the irrigated regime, and 100% and 107%, respectively, in the rainfed regime. The two popular irrigated rice cultivars (NSIC Rc222 and PSB Rc82) had the highest yield in the irrigated regime, but their yield did not differ significantly from those of 7 of the drought-tolerant cultivars. Percentage yield increase was not different between CSNM and FNM in both water regimes, while NSIC Rc282 had the highest percentage yield increase among cultivars in both water regimes.
Table 1. Grain yield and percentage yield increase of 15 rice cultivars grown in three nutrient management regimes under irrigated and rainfed lowland conditions in 2014
In 2015, grain yield in the rainfed regime was 11% lower than that in the irrigated regime (; 3.93 vs. 3.48 t ha−1). Yield differences were significant between treatments and cultivars, but without a significant cultivar × nutrient management interaction. The yield increases compared with control were 62% in CSNM and 56% in CSNM+P in the irrigated regime, and 81% and 82%, respectively, in the rainfed regime. Yield in CSNM+P was not significantly higher than that in CSNM. NSIC Rc282 had the highest mean yield in the irrigated regime, followed by NSIC Rc274, and NSIC Rc222 had the highest mean yield in the rainfed regime, followed by NSIC Rc282. Percentage yield increase did not differ significantly between nutrient management treatments or cultivars in irrigated regime, while NSIC Rc282 had the highest percentage yield increase among cultivars in rainfed regime.
Table 2. Grain yield and percentage yield increase of 10 rice cultivars grown in three nutrient management regimes under irrigated and rainfed lowland conditions in 2015
In both years, NSIC Rc274, NSIC Rc282, NSIC Rc286 and PSB Rc14 had similar yield to NSIC Rc222 in both water regimes. Particularly, average yield of NSIC Rc282 was the same as that of NSIC Rc222 (4.24 t ha−1) across water regimes and years.
Effects of N on grain yield in response to drought at different growth stages
In the drought experiment in 2016, grain yield in PD2 was lower than that in PD1 (2.33 vs. 3.60 t ha−1) owing to the long period of drought after the reproductive stage (Supplementary Figure S1). Differences in yield were significant between cultivars and nutrient management treatments in PD1 and between cultivars in PD2, but their interaction was significant only in PD2 (). NSIC Rc282 consistently had higher yield than NSIC Rc222, whereas N2 had the lowest yield in PD1. Interestingly, N1 and N3 gave similar yields in NSIC Rc282 in both PD1 and PD2, but N1 gave a lower yield than N3 in NSIC Rc222.
Table 3. Effects of different timings of drought imposition on grain yield of two cultivars grown in three N management regimes in 2016
Supplementary Table S3 summarizes the yield components of NSIC Rc282 and NSIC Rc222 in 2016. Although spikelets per m2 were not different between cultivars in both PD1 and PD2, NSIC Rc282 had higher percentage of filled grains in PD1 and larger grain weight in PD2 than NSIC Rc222. N application at 37 DAT (N1 and N3) contributed to larger spikelet number per m2 in PD1, but not in PD2.
NSIC Rc222 had higher LAI than NSIC Rc282 until 37 DAT in PD1 and 21 DAT in PD2, but NSIC Rc282 had higher LAI at the end of the drought imposition (52 DAT in PD1 and 37 DAT in PD2) (). NSIC Rc282 had higher aboveground biomass at 75 DAT than NSIC Rc222 in PD2. Nutrient management did not affect LAI during the drought imposition in either trial, whereas N3 had significantly higher biomass at 75 DAT in both PD1 and PD2.
Table 4. Leaf area index and aboveground biomass of two cultivars grown in three N management regimes affected by the two different timings of drought stress in 2016
Discussion
We evaluated the agronomic responses of new drought-tolerant rice cultivars and two popular irrigated rice cultivars to nutrient management in rainfed lowlands. The soil of the study fields produced a good yield without fertilizer application, at an average of 2.51 t ha−1 across water regimes and years (), comparable to previously reported yields in the Philippines and Indonesia (Dobermann et al., Citation2002). Nevertheless, fertilizer application significantly improved yield in the rainfed regime in both 2014 and 2015. The application of P has been often emphasized for infertile sandy soils in rainfed lowlands because its availability in the soil decreases sharply immediately after the disappearance of standing water (Kato & Katsura, Citation2014; Kato et al., Citation2016). However, the 2015 results showed no significant yield gain in response to the additional P input (CSNM+P versus CSNM), which suggests that the standard P fertilization rate in CSNM (21 to 35 kg ha−1 of P2O5-equivalent) is sufficient.
Water regime had little effect on grain yield in 2014 and 2015 owing to the ample rainfall. In the irrigated regime, NSIC Rc222 had the highest yield in 2014 and the third-highest yield in 2015. However, yield of three new drought-tolerant cultivars (NSIC Rc274, NSIC Rc282, and NSIC Rc286) was not significantly different from that of NSIC Rc222 in the irrigated regime in either year. In particular, we found that NSIC Rc282 was fertilizer-responsive and produced consistently high yield among the drought-tolerant cultivars. When the drought was mild, the yield response to nutrient management in the rainfed regime was not cultivar-specific; that is, there was a non-significant cultivar × nutrient interaction in both 2014 and 2015 (). This was in consistency with the results of Inthapanya et al. (Citation2000). Although the number of cultivars and the nutrient management regimes were slightly modified between the 2 years, NSIC Rc282 had consistently high percentage yield increase due to fertilizer inputs in both water regimes. On this basis, we selected NSIC Rc282 to compare the response to nutrient management under drought with a popular irrigated rice cultivar (NSIC Rc222) in the 2016 experiment.
The 2016 experiment showed that NSIC Rc282 produced higher yield than NSIC Rc222 under drought during the late vegetative and early reproductive stages (PD1) and during the early vegetative stage (PD2) (). An unexpected water scarcity occurred in October owing to exhaustion of the irrigation source, and the cultivar difference was significant for the yield components determined after heading (Supplementary Table S3): NSIC Rc282 had higher percentage of filled grains or larger grain weight than NSIC Rc222. Furthermore, our results provide direct evidence of the improved drought adaptation of NSIC Rc282. While the two cultivars had the same yield potential in our previous study (Liu et al., Citation2019), NSIC Rc282 had 10% larger grains and NSIC Rc222 had 15% more spikelets per m2 under non-stress conditions. The similarity of spikelet number per m2 between the two cultivars in 2016 may be, at least in part, due to the better growth of NSIC Rc282 under drought during the periods when the spikelet number is determined, i.e. vegetative and reproductive stages. This hypothesis is supported by the degree of leaf expansion during the drought imposition and the subsequent biomass accumulation. Compared with NSIC Rc222, NSIC Rc282 had significantly higher LAI in both PD1 and PD2 at the end of the drought imposition and significantly higher biomass at 75 DAT in PD2 (). Future research should investigate the physiological mechanisms and morphological factors that give the new high-yielding drought-tolerant cultivar better shoot growth and yield than the popular high-yielding cultivar under drought stress.
As discussed above, the yield response to nutrient management was not cultivar-specific under mild drought, with a yield reduction of less than 15% under stress. In other words, the concept for nutrient management in irrigated lowlands based on the target yield and soil fertility (Buresh et al., Citation2019) can be applied to any cultivar in favorable rainfed lowlands. On the other hand, our previous study (Banayo et al., Citation2018) showed that the improved nutrient management has had a limited impact on rice yield in drought-prone areas in the Philippines, where the yield reduction was 30% to 60%, with yields less than 4 t ha−1. Those results suggested the need to introduce both drought-tolerant cultivars and drought-adapted nutrient management. Although the cultivar × nutrient regime interaction was not significant in the 2016 drought experiment, yield was highest with N1 in NSIC Rc282 and with N3 in NSIC Rc222 in both PD1 and PD2 (). N1 differed from N2 only in the timing of the N application (i.e. during drought imposition in N2 and at the cessation of drought in N1), and N1 gave higher yield than N2 in NSIC Rc282. This result agrees with previous claims about the importance of N application at the cessation of abiotic stresses such as drought and submergence (Banayo et al., Citation2019; Prasertsak & Fukai, Citation1997). On the other hand, NSIC Rc222 required more frequent N inputs and a larger total input (N3) to enhance yield under drought, and the yield of NSIC Rc282 with less N input (N1) was still higher. It is likely that NSIC Rc282 was able to keep taking up soil N throughout the drought and use it to sustain plant growth under drought more effectively than NSIC Rc222. This hypothesis awaits further investigation in future drought studies.
Since the N recovery (ratio of the increase in absorbed N to the applied N) under drought is generally low (Tuong et al., Citation2002), high N inputs would be needed to grow popular irrigated rice cultivars in rainfed lowlands. In contrast, omitting the N application during the drought period (i.e. 70 kg N ha−1 in N1 versus 110 kg N ha−1 in N3) did not greatly reduce the yield of drought-adapted NSIC Rc282. Accordingly, we suggest that the use of high-yielding drought-tolerant cultivars should contribute not only to yield improvement, but also to resource-use efficient rice cultivation in rainfed lowlands.
Conclusions
In our yield trials of 15 rice cultivars grown in three nutrient management regimes under irrigated and rainfed conditions, we identified a few drought-tolerant cultivars with high fertilizer-responsiveness that are suitable for use in rainfed lowlands. These cultivars produced yield as high as two popular irrigated rice cultivars under well-watered conditions. We found that the recommended nutrient management for irrigated lowlands can be applied to any rice cultivar in rainfed lowlands when drought is mild, with a yield reduction of less than 20%. However, our results also suggest the importance of cultivar-specific management under moderate drought. A drought-tolerant cultivar that is well adapted to moderate drought can effectively utilize the applied N during the drought and thus would not require additional N inputs, whereas an irrigated rice cultivar would need greater N application under drought. We suggest that the introduction of high-yielding drought-tolerant rice cultivars contributes not only to improved crop productivity, but also to fertilizer-use efficient rice cultivation in drought-prone environments.
PPS2020_110RP-File003.docx
Download MS Word (82.5 KB)Disclosure statement
The authors report that they have no potential conflict of interest.
Supplementary material
Supplemental data for this article can be accessed here.
Additional information
Funding
References
- Atlin, G. N., Cairns, J. E., & Das, B. (2017). Rapid breeding and varietal replacement are critical to adaptation of cropping systems in the developing world to climate change. Global Food Security, 12, 31–37. https://doi.org/10.1016/j.gfs.2017.01.008
- Banayo, N. P. M. C., Haefele, S. M., Desamero, N. V., & Kato, Y. (2018). On-farm assessment of site specific nutrient management for rainfed lowland rice in the Philippines. Field Crops Research, 220, 88–96. https://doi.org/10.1016/j.fcr.2017.09.011
- Banayo, N. P. M. C., Mabesa-Telosa, R. C., Singh, S., & Kato, Y. (2019). Improved early season management of sub1 rice varieties enhances post-submergence recovery and yield. Experimental Agriculture, 55(1), 105–116. https://doi.org/10.1017/S0014479717000588
- Buresh, R. J., Castillo, R. L., Dela Torre, J. C., Laureles, E. V., Samson, M. I., Sinohin, P. J., & Guerra, M. (2019). Site-specific nutrient management for rice in the Philippines: Calculation of field-specific fertilizer requirements by Rice Crop Manager. Field Crops Research, 239, 56–70. https://doi.org/10.1016/j.fcr.2019.05.013
- Dobermann, A., Witt, C., Dawe, D., Gines, G. C., Nagarajan, R., Satawathananont, S., Satawathananont, S., Son, T. T., Tan, P. S., Wang, G. H., Chien, N. V., Thoa, V. T. K., Phung, C. V., Stalin, P., Muthukrishnan, P., Ravi, V., Babu, M., Chatuporn, S., Sookthongsa, J., Fu, R., … Adviento, M. A. A. (2002). Site-specific nutrient management for intensive rice cropping systems in Asia. Field Crops Research, 74(1), 37–66. https://doi.org/10.1016/S0378-4290(01)00197-6
- Fukai, S., & Ouk, M. (2012). Increased productivity of rainfed lowland rice cropping systems of the Mekong region. Crop Pasture Science, 63, 944–973. https://doi.org/10.1071/CP12294
- Haefele, S. M., Kato, Y., & Singh, S. (2016). Climate ready rice: Augmenting drought tolerance with best management practices. Field Crops Research, 190, 60–69. https://doi.org/10.1016/j.fcr.2016.02.001
- Inthapanya, P., Sipaseuth, S., Sihathep, P., Chanphengsay, V., Fukai, M., Basnayake, J., & Basnayake, J. (2000). Genotypic performance under fertilised and nonfertilized conditions in rainfed lowland rice. Field Crops Research, 65(1), 1–14. https://doi.org/10.1016/S0378-4290(99)00065-9
- Kato, Y., & Katsura, K. (2014). Rice adaptation to aerobic soils: Physiological consideration and implications for agronomy. Plant Production Science, 17(1), 1–12. https://doi.org/10.1626/pps.17.1
- Kato, Y., Tajima, R., Toriumi, A., Homma, K., Moritsuka, N., Shiraiwa, T., Yamagishi, J., Mekwatanakern, P., Chamarerk, V., & Jongdee, V. (2016). Grain yield and phosphorus uptake of rainfed lowland rice under unsubmerged soil stress. Field Crops Research, 190, 54–59. https://doi.org/10.1016/j.fcr.2016.01.004
- Kumar, A., Dixit, S., Ram, T., Yadaw, R. B., Mishra, K. K., & Mandal, N. P. (2015). Breeding high-yielding drought-tolerant rice: Genetic variations and conventional and molecular approaches. Journal of Experimental Botany, 65(21), 6265–6278. https://doi.org/10.1093/jxb/eru363
- Liu, H., Won, P. L. P., Banayo, N. P. M., Nie, L., Peng, S., & Kato, Y. (2019). Late-season nitrogen applications improve grain yield and fertilizer-use efficiency of dry direct-seeded rice in the tropics. Field Crops Research, 233, 114–120. https://doi.org/10.1016/j.fcr.2019.01.010
- Ohno, H., Banayo, N. P. M. C., Bueno, C., Kashiwagi, J., Nakashima, T., Iwama, K., Corales, A. M., Garcia, R., & Kato, Y. (2018). On-farm assessment of a new early-maturing drought-tolerant rice cultivar for dry direct seeding in rainfed lowlands. Field Crops Research, 219, 222–228. https://doi.org/10.1016/j.fcr.2018.02.005
- Pampolino, M. F., Manguiat, I. J., Ramanathan, S., Gines, H. C., Tan, P. S., Chi, T. T. N., Rajendran, R., & Buresh, R. J. (2007). Environmental impact and economic benefits of site-specific nutrient management (SSNM) in irrigated rice systems. Agricultural Systems, 93(1–3), 1–24. https://doi.org/10.1016/j.agsy.2006.04.002
- Prasertsak, A., & Fukai, S. (1997). Nitrogen availability and water stress interaction on rice growth and yield. Field Crops Research, 52(3), 249–260. https://doi.org/10.1016/S0378-4290(97)00016-6
- Sansen, K., Wongboon, W., Jairin, J., & Kato, Y. (2019). Farmer-participatory evaluation of mechanized dry direct-seeding technology for rice in northeastern Thailand. Plant Production Science, 22(1), 46–53. https://doi.org/10.1080/1343943X.2018.1557530
- Tuong, T. P., Castillo, E. G., Cabangan, R. C., Boling, A., & Singh, U. (2002). The drought response of lowland rice to crop establishment practices and N-fertilizer sources. Field Crops Research, 74(2–3), 243–257. https://doi.org/10.1016/S0378-4290(01)00213-1
- Wade, L. J., Amarante, S. T., Olea, A., Harnpichitvitaya, D., Naklang, K., Wihardjaka, A., Sengar, S. S., Mazid, M. A., Singh, G., & McLaren, C. G. (1999). Nutrient requirements in rainfed lowland rice. Field Crops Research, 64(1–2), 91–107. https://doi.org/10.1016/S0378-4290(99)00053-2