ABSTRACT
Rubisco is the key enzyme responsible for photosynthetic CO2 fixation. Previously, we succeeded to increase the catalytic rate (kcat) of Rubisco by the overexpression of sorghum C4-type Rubisco small subunit (RbcS) in rice. These Rubisco are present as a chimera of sorghum RbcS and rice RbcS. In this study, we introduced an RNAi construct for rice RbcS into a sorghum RbcS overexpression line (SS10). Obtained double-transgenic lines iSS4 and iSS5 dominantly expressed sorghum RbcS and accumulated 35% and 20% of Rubisco content of non-transgenic rice (WT), respectively. Rubisco in these double-transgenic lines showed higher kcat and Km for CO2 than WT and SS10. Moreover, iSS4 showed higher photosynthetic nitrogen use efficiency under high CO2 conditions. These results demonstrate the usefulness of C4-type RbcS to improve photosynthetic performance under elevated CO2 conditions.
Graphical abstract
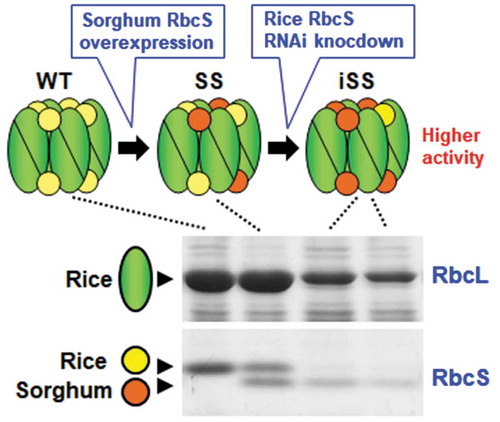
Introduction
Plant biomass and yield depend on the photosynthetic activity. Rubisco is a key photosynthetic enzyme responsible for CO2 fixation, and it also catalyzes the oxygenation reaction using O2 as a substrate, leading to wasteful photorespiration (Fernie & Bauwe, Citation2020). Many important crops, such as rice, soybean and potato, are classified as C3 plants. Unfortunately, the catalytic turnover rate (kcat) of Rubisco in most C3 plants is particularly low compared with that of C4 plants such as maize and sorghum (Sage, Citation2002; Sharwood et al., Citation2016). Thus, C3 plants accumulate a large amount of Rubisco in their leaves, which accounts for 15–30% of total leaf nitrogen content, leading to low nitrogen use efficiency (Evans, Citation1989; Makino et al., Citation1992). In contrast, Rubisco from C3 plants shows a higher affinity for CO2 (i.e. lower Kc) than C4 plants (Ishikawa et al., Citation2009; Tcherkez et al., Citation2006). Increases in the kcat usually decrease the affinity for CO2, leading to increases in photorespiration. However, the photorespiration will decrease under future elevated CO2 conditions. Thus, enhancing the kcat, though decreasing the affinity for CO2, can be a possible strategy to improve the photosynthetic capacity in the near future environment.
Rubisco consists of eight large subunits (RbcL) and eight small subunits (RbcS) in plants (Andersson & Backlund, Citation2008). The catalytic site is present in RbcL, whereas RbcS is an important determinant of the catalytic properties of Rubisco (Ishikawa et al., Citation2011). Recently, we overexpressed sorghum RbcS and knocked out rice RbcS by CRISPR/Cas9 system in rice (Matsumura et al., Citation2020). This hybrid Rubisco showed almost C4-plant-like kinetics, i.e. approximately two times higher kcat and lower affinity for CO2. Importantly, the transgenic lines accumulated reduced levels of Rubisco (44–67% of non-transgenic rice (WT)) showed higher photosynthetic capacity and similar biomass production under high CO2 conditions compared to WT. Thus, our strategy can be effective to improve the photosynthetic capacity under elevated CO2 conditions.
In this study, we applied an alternative strategy, i.e. RNAi knockdown targeted to rice RbcSs in sorghum RbcS overexpression line to replace rice RbcS with sorghum RbcS and reduce Rubisco content. We analyzed the biochemical and physiological properties of these double-transgenic lines and evaluate the effectiveness of this strategy to improve the photosynthetic capacity in rice.
Materials and methods
Plant materials and growth conditions
Rice (Oryza sativa L. cv. Nipponbare) and its transgenic lines were grown in soil under natural sunlight conditions in a temperature-controlled greenhouse (30°C day/25°C night). Rice seedlings at 4.5 leaf stage (number of leaves in the main stem) were transplanted into 1.0 L pots filled with paddy soil containing a chemical fertilizer (N:P:K = 8:8:8) at 2.0 g, a slow-release fertilizer (LP70, JCAM AGRI, Tokyo, Japan) at 0.5 g, a micronutrient (High Green, MC FERTICOM, Tokyo, Japan) at 0.4 g and a silicate fertilizer (Softsilica Milion, Softsilica, Tokyo, Japan) at 1.5 g per pot.
To analyze the dry weight, plants were grown in a growth chamber on a 30°C/25°C night cycle with a day period of 14 h under illumination at a photosynthetically active photon flux density (PPFD) of 400 μmol m−2 s−1. The CO2 treatment (38 and 100 Pa as ambient and elevated CO2, respectively) was started immediately after germination. Rice seedlings at the leaf stage of 4.0 were transplanted into 0.5 L pots filled with a commercial granular soil containing the same concentration of the fertilizers described above. Thirty days after germination, shoot and root were sampled and dried for 3 days at 80°C to determine the dry weight.
Plasmid construction and transformation of rice
The C-terminal 335 bp coding region of OsRbcS3 (Supplementary Figure 1) was amplified by RT-PCR using gene-specific primers (Supplementary Table 1). The PCR fragment was cloned into the RNAi vector pANDA-β containing the bialaphos resistance gene (generous gifts of Prof. Ko Shimamoto, Nara Institute of Science and Technology). The construct was introduced into calli derived from the transgenic line SS10, which overexpresses sorghum RbcS (Ishikawa et al., Citation2011) via Agrobacterium-mediated gene transfer. Bialaphos-resistant transgenic plants were regenerated from calli and planted in soil. Expression of RbcS was analyzed by SDS-PAGE as described previously (Ishikawa et al., Citation2011). Homozygous transgenic plants at T3 or T4 generations were used for subsequent analyses.
Measurement of Rubisco activity
Rubisco activity was determined at 28°C using [14C] NaHCO3 (specific activity, 37 MBq mmol−1) by assaying the incorporation of 14C into acid-stable products, as described previously (Ishikawa et al., Citation2009). Rubisco catalytic site concentrations were determined by measuring the stoichiometric binding of [14C] carboxyarabinitol-1,5-bisphosphate (CABP; specific activity, 1.85 GBq mmol−1) as described previously (Ishikawa et al., Citation2009). The kcat of Rubisco (mol mol−1 s−1) was determined by calculating the ratio of the in vitro maximum Rubisco activity to the Rubisco catalytic sites. The Kc was calculated from the Hanes-Woolf plot of the Rubisco activities at six different NaH14CO3 concentrations (0.5–15 mM).
Measurement of photosynthetic rate
The gas-exchange of the uppermost fully expanded leaf was measured using an open gas-exchange system (LI-6400, Li-Cor, Lincoln). The measurement was performed at a leaf temperature of 28°C, 21% O2, a PPFD of 1500 μmol quanta m−2 s−1, a leaf-to-air vapor pressure difference of 1.0–1.2 kPa and three different CO2 partial pressures (14, 38 and 88 Pa). The Sc/o was estimated by the slope of the regression lines of the dependence of CO2 compensation point on the O2 concentration as described previously (Ishikawa et al., Citation2011).
Determination of Rubisco, soluble protein, chlorophyll and nitrogen
The contents of Rubisco, soluble protein and chlorophyll in the leaves were determined as described previously (Matsumura et al., Citation2020). Rubisco content was determined using [14C] CABP as described above. The total soluble protein was determined by the colorimetric assay using Coomassie blue. The chlorophyll was extracted with 80% (v/v) acetone and determined spectrophotometrically. The nitrogen content was determined by the indophenol colorimetric assay after the Kjeldahl digestion.
Results
RNAi knockdown of RbcS in transgenic rice overexpressing sorghum RbcS
In our previous study, the overexpression of sorghum RbcS increased the kcat and content of Rubisco in transgenic rice (SS line, Ishikawa et al., Citation2011). Rice contains four photosynthetic RbcS genes (OsRbcS2-5). To replace rice RbcS with sorghum counterpart and reduce Rubisco content in the SS line, we introduced an RNAi construct for OsRbcS3, which showed the highest expression levels among rice RbcS genes (Suzuki et al., Citation2009). The nucleotide sequences among OsRbcS2-5 are highly conserved (Morita et al., Citation2014). RNAi should not affect the expression of sorghum RbcS. The homology of the C-terminal region of sorghum RbcS with OsRbcS2-5 is lower than that of the N-terminal region (see supplementary Figure 1). Thus, the trigger for RNAi was designed at the C-terminal region of OsRbcS3. The RNAi construct was introduced into transgenic line SS10, which express sorghum RbcS by 44% of total RbcS (Ishikawa et al., Citation2011). Twenty independent double-transgenic lines were obtained from bialaphos-resistant calli. In the parent SS10 line, both rice RbcS and sorghum RbcS were detected by SDS-PAGE analysis with Coomassie blue staining (). The expression of rice RbcS was almost undetectable in most double-transgenic lines, indicating that RNAi knockdown was successful in our experiment (). However, the expression of sorghum RbcS was concomitantly decreased in all double-transgenic lines. As a result, the expression of RbcL in these transgenic lines also decreased, suggesting that the Rubisco content was decreased. Most transgenic lines exhibited stunted growth possibly due to reduced Rubisco content. Thus, among these progeny, two homozygous transgenic lines showing a moderate reduction in RbcL, designated as iSS4 and iSS5, were selected and used for subsequent analyses.
Figure 1. Expression of RbcS and kinetic properties of Rubisco. (a) Expression of RbcS. Soluble proteins (6.4 μg) extracted from leaves were separated by 14% SDS-PAGE and stained by Coomassie blue. Fractions around RbcL (upper panel) and RbcS (lower panel) are shown. (b) Kinetic properties of Rubisco. kcat, Kc and Sc/o values are the means ± SE of 4–5 biological replicates. Different letters above the bars indicate significant difference (P < 0.05) between lines determined by Tukey’s test. WT, non-transgenic rice; SS10, sorghum RbcS overexpression line; iSS4 and iSS5; double-transgenic lines expressing sorghum RbcS with RbcS knockdown by RNAi
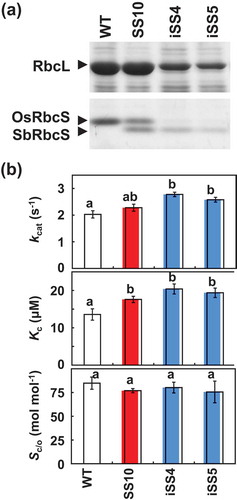
Kinetic properties of Rubisco
SS10 showed higher values of kcat and Kc of Rubisco compared with WT (). These trends were more pronounced in iSS4 and iSS5. While there were no significant differences, the specificity factors (Sc/o) in SS10, iSS4 and iSS5 were slightly lower than that in WT. These findings confirm that the incorporation of sorghum RbcS shifts the kinetic properties to increase the activity of Rubisco, and this effect is further enhanced by nearly complete replacement of rice RbcS with sorghum RbcS. However, complete hybrid-Rubisco in CSS lines showed a 1.8–1.9 fold increase in kcat compared with WT (Matsumura et al., Citation2020). These increases were larger than iSS lines (1.3–1.4 fold), suggesting that partial incorporation of rice RbcS reduced the effect of sorghum RbcS on Rubisco kinetics in iSS lines.
Photosynthesis and leaf constituents
Rubisco contents were significantly decreased in both double-transgenic lines compared with SS10 and WT (). The levels of Rubisco in iSS4 and iSS5 were 35% and 25% of WT, respectively. Thus, iSS4 accumulated more Rubisco than iSS5. The nitrogen and soluble protein contents were also decreased in iSS4 and iSS5, possibly due to large decreases in Rubisco content. The decreases in the chlorophyll content were observed in SS10, iSS4 and iSS5.
Figure 2. Rubisco, total soluble protein, chlorophyll and nitrogen contents. Uppermost fully expanded leaves (10th leaf blade at 10.5 leaf stage) were used for experiments. Data represent the mean ± SD of five biological replicates. Different letters above the bars indicate significant difference (P < 0.05) between lines determined by Tukey’s test
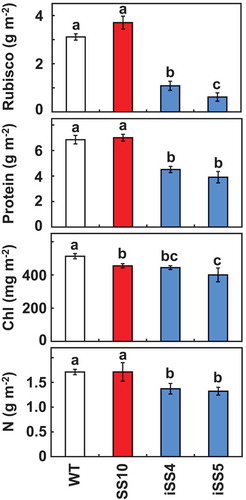
The photosynthetic CO2 assimilation rates were measured at three different CO2 partial pressures (). The photosynthetic rates under less than ambient CO2 partial pressures (14 and 38 Pa) were significantly decreased in iSS4 and iSS5 compared with SS10 and WT. These trends were evident based on both the leaf area and the nitrogen content. In contrast, the photosynthetic rates in double-transgenic lines approached WT and CSS10 under high CO2 conditions (88 Pa). Moreover, iSS4 showed a significantly higher photosynthetic rate at high CO2 conditions based on the nitrogen content. These results demonstrate the effectiveness of our strategy, introducing high activity Rubisco and reducing its content by RNAi to improve the photosynthetic nitrogen use efficiency.
Figure 3. Photosynthetic rate under different CO2 partial pressures. The CO2 assimilation rates are shown based on the leaf area (a) and nitrogen content (b). The CO2 assimilation rates of uppermost fully expanded leaves (10th leaf blade at 10.5 leaf stage) were measured at a photon flux density of 1,500 μmol m−2 s−1, leaf temperature of 28°C and CO2 partial pressures of 14, 38 and 88 Pa. Data represent the mean ± SD of four biological replicates. Different letters above the bars indicate significant difference (P < 0.05) between lines determined by Tukey’s test
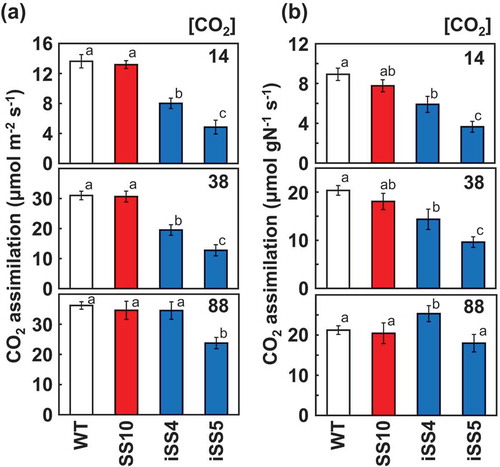
Plant growth
We grew plants in growth chamber under ambient and elevated CO2 conditions for a relatively short period and determined the dry weights of the shoot and root (). Under ambient CO2 condition, the dry weights of the shoots and roots of iSS4 and iSS5 were significantly lower than those of WT and SS10. The decreases in these dry weights were more marked in iSS5. At elevated CO2 level, the dry weights of the shoot and root of iSS4 recovered to the level of control lines. Thus, iSS4 contains substantially reduced levels of Rubisco while also maintaining normal biomass production under elevated CO2 conditions.
Figure 4. Shoot and root dry weights of plants grown under different CO2 levels. Rice plants were grown under CO2 partial pressures of 38 Pa (a) and 100 Pa (b). Shoot and root dry mass were determined at 30 days after germination. Different letters above the bars indicate significant difference (P < 0.05) between lines determined by Tukey’s test
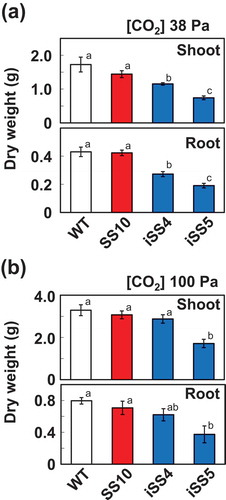
Discussion
In our previous work, the chimeric incorporation of sorghum RbcS was shown to enhance the kcat of Rubisco in transgenic rice, whereas the photosynthetic rate of these plants was not increased (Ishikawa et al., Citation2011). In these plants, the level of Rubisco is considered to be in excess of photosynthesis. Subsequently, we knocked out Rice RbcS in sorghum RbcS overexpression lines by CRISPR-Cas9 system (CSS line) and succeeded to increase the photosynthetic nitrogen efficiency under elevated CO2 conditions (Matsumura et al., Citation2020). In this study, we applied an alternative strategy, i.e. knockdown rice RbcS by RNAi. One of the transgenic lines iSS4 showed higher photosynthetic nitrogen use efficiency than WT under elevated CO2 conditions (). As described above, CSS line also showed higher nitrogen use efficiency, whereas Rubisco content in iSS4 was only 35% of WT, and lower than those in CSS lines. In antisense RbcS transgenic rice, much higher Rubisco content (65% of WT) was needed to increase the photosynthetic nitrogen use efficiency (Makino et al., Citation1997). As CSS lines, iSS lines showed significantly higher kcat than WT (). Thus, it is likely that the changes in kinetic properties should contribute to higher photosynthetic nitrogen use efficiency in our transgenic lines. Maximum Rubisco limited CO2 assimilation rates estimated by kcat and Rubisco content in WT, SS10, iSS4 and iSS5 () were 93.5, 118.2, 44.9 and 23.7 μmol m−2 s−1, respectively. This parameter of iSS4 was markedly lower than that of WT. Actually, ribulose-1,5-bisphosphate regeneration capacity should limit the CO2 assimilation rates under high CO2 conditions (Sharwood et al., Citation2016). Thus, WT, SS10 and iSS4 showed similar CO2 assimilation rate at CO2 partial pressure of 88 Pa (). iSS4 achieves this CO2 assimilation rate with lower nitrogen content, this led to the improvement of photosynthetic nitrogen use efficiency (). However, this higher nitrogen use efficiency in iSS4 did not enhance the dry weight of shoot and root (). Basically, the photosynthetic rate based on the leaf area was not enhanced in iSS lines compared to WT (). In this study, we grew rice plants with sufficient nitrogen supply until the time of sampling. It will be interesting to compare the growth of iSS4 and WT under nitrogen-limited conditions.
The reduction was somewhat specific to rice RbcS. Therefore, the double-transgenic lines dominantly expressed sorghum RbcS (). However, given the substantial decrease in the Rubisco content (), RNAi should also reduce the expression of sorghum RbcS. In this study, SS10 expressing sorghum RbcS at 44% of the total RbcS level (inversely, rice RbcS expressed at 56% of the total level) was used as a parent line for the introduction of the RNAi construct. In this line, the overexpression of sorghum RbcS did not affect the expression of native rice RbcSs (Ishikawa et al., Citation2011). Thus, after translation, these RbcSs should compete with each other for incorporation into Rubisco. Therefore, by simply assuming that the 56% expression level of rice RbcS in this transgenic line is equivalent to 100% expression in WT, the sorghum RbcS expression level should be 78.6% of the WT if the RNAi specifically and completely suppresses the rice RbcS. In our double-transgenic lines, the expression of Rubisco was less than 78.6% of the WT (), suggesting that sorghum RbcS also knocked down. The effectiveness of RNAi knockdown strongly correlates with the sequence homology between the trigger region and the target sequence (Miki et al., Citation2005). The region of rice RbcS used for the RNAi trigger shares 77.8% homology with the sorghum RbcS sequence. This level of homology is within the range of off-target effects for RNAi mediated suppression in rice (Miki et al., Citation2005). In the plant RNAi system, relatively longer trigger sequences, i.e. 300 to 500 bp, are necessary to effectively suppress a target gene (Miki & Shimamoto, Citation2004). Thus, designing an exclusive and specific trigger sequence for RNAi to the target gene in the coding region is difficult. Although it takes significant time and effort, the specific knockdown of each OsRbcSs by RNAi was successfully demonstrated using 3ʹUTR as a trigger (Ogawa et al., Citation2012), thus pyramiding can be possible. Alternatively, the tandem fusion of multiple 3ʹUTRs can be used to specific multi-genes knockdown by RNAi (Miki et al., Citation2005). However, if specificity is important, it is currently better to knock out using CRISPR-Cas9 system (Matsumura et al., Citation2020). Possibly, RNAi may have potential when Rubisco kinetics, i.e. ratio of rice RbcS and sorghum RbcS needs to change depending on leaf development or environmental conditions.
Rice varieties contain considerable natural variation in the extent of the response to elevated CO2 concentrations and photosynthetic capacity (Hasegawa et al., Citation2013; Shimono et al., Citation2009). For example, a high-yielding variety, Takanari, maintains higher levels of Rubisco and photosynthetic capacity under elevated CO2 conditions (Chen et al., Citation2014). Thus, it is interesting to introduce the C4-like catalytic properties of Rubisco into rice species showing different CO2 responses and photosynthetic capacities.
PPS2020_141SR-File003.pptx
Download MS Power Point (86.9 KB)Disclosure statement
No potential conflict of interest was reported by the authors.
Supplemental material
Supplemental data for this article can be accessed here.
Additional information
Funding
References
- Andersson, I., & Backlund, A. (2008). Structure and function of Rubisco. Plant Physiology & Biochemistry, 46(3), 275–291. https://doi.org/10.1016/j.plaphy.2008.01.001
- Chen, C. P., Sakai, H., Tokida, T., Usui, Y., Nakamura, H., & Hasegawa, T. (2014). Do the rich always become richer? Characterizing the leaf physiological response of the high-yielding rice cultivar Takanari to free-air CO2 enrichment. Plant & Cell Physiology, 55(2), 381–391. https://doi.org/10.1093/pcp/pcu009
- Evans, J. R. (1989). Photosynthesis and nitrogen relationships in leaves of C3 plants. Oecologia, 78, 9–19. https://link.springer.com/article/10.1007/BF00377192
- Fernie, A. R., & Bauwe, H. (2020). Wasteful, essential, evolutionary stepping stone? The multiple personalities of the photorespiratory pathway. The Plant Journal, 102(4), 666–677. https://doi.org/10.1111/tpj.14669
- Hasegawa, T., Sakai, H., Tokida, T., Nakamura, H., Zhu, C., Usui, Y., Yoshimoto, M., Fukuoka, M., Wakatsuki, H., Katayanagi, N., Matsunami, T., Kaneta, Y., Sato, T., Takakai, F., Sameshima, R., Okada, M., Mae, T., & Makino, A. (2013). Rice cultivar responses to elevated CO2 at two free-air CO2 enrichment (FACE) sites in Japan. Functional Plant Biology, 40(2), 148–159. https://doi.org/10.1071/FP12357
- Ishikawa, C., Hatanaka, T., Misoo, S., & Fukayama, H. (2009). Screening of high kcat Rubisco among Poaceae for improvement of photosynthetic CO2 assimilation in rice. Plant Production Science, 12(3), 345–350. https://doi.org/10.1626/pps.12.345
- Ishikawa, C., Hatanaka, T., Misoo, S., Miyake, C., & Fukayama, H. (2011). Functional incorporation of sorghum small subunit increases the catalytic turnover rate of Rubisco in transgenic rice. Plant Physiology, 156(3), 1603–1611. https://doi.org/10.1104/pp.111.177030
- Makino, A., Sakashita, H., Hidema, J., Mae, T., Ojima, K., & Osmond, B. (1992). Distinctive responses of ribulose-1,5-bisphosphate carboxylase and carbonic anhydrase in wheat leaves to nitrogen nutrition and their possible relationships to CO2 transfer resistance. Plant Physiology, 100(4), 1737–1743. https://doi.org/10.1104/pp.100.4.1737
- Makino, A., Shimada, T., Takumi, S., Kaneko, K., Matsuoka, M., Shimamoto, K., Nakano, H., Miyao-Tokutomi, M., Mae, T., & Yamamoto, N. (1997). Does decrease in ribulose-1,5-bisphosphate carboxylase by antisense RbcS lead to a higher N-use efficiency of photosynthesis under conditions of saturating CO2 and light in rice plants? Plant Physiology, 114, 483–491. https://doi.org/10.1104/pp.114.2.483
- Matsumura, H., Shiomi, K., Yamamoto, A., Taketani, Y., Kobayashi, N., Takuya Yoshizawa, T., Tanaka, S., Yoshikawa, H., Endo, M., & Fukayama, H. (2020). Hybrid Rubisco with complete replacement of rice Rubisco small subunits by sorghum counterparts confers C4 plant-like high catalytic activity. Molecular Plant, 13(11), 1570–1581. https://doi.org/10.1016/j.molp.2020.08.012
- Miki, D., Itoh, R., & Shimamoto, K. (2005). RNA silencing of single and multiple members in a gene family of rice. Plant Physiology, 138(4), 1903–1913. https://doi.org/10.1104/pp.105.063933
- Miki, D., & Shimamoto, K. (2004). Simple RNAi vectors for stable and transient suppression of gene function in rice. Plant & Cell Physiology, 45(4), 490–495. https://doi.org/10.1093/pcp/pch048
- Morita, K., Hatanaka, T., Misoo, S., & Fukayama, H. (2014). Unusual small subunit that is not expressed in photosynthetic cells alters the catalytic properties of Rubisco in rice. Plant Physiology, 164(1), 69–79. https://doi.org/10.1104/pp.113.228015
- Ogawa, S., Suzuki, Y., Yoshizawa, R., Kanno, K., & Makino, A. (2012). Effect of individual suppression of RBCS multigene family on Rubisco contents in rice leaves. Plant Cell & Environment, 35(3), 546–553. https://doi.org/10.1111/j.1365-3040.2011.02434
- Sage, R. F. (2002). Variation in the kcat of Rubisco in C3 and C4 plants and some implications for photosynthetic performance at high and low temperature. Journal of Experimental Botany, 53(369), 609–620. https://doi.org/10.1093/jexbot/53.369.609
- Sharwood, R. E., Ghannoum, O., & Whitney, S. M. (2016). Prospects for improving CO2 fixation in C3-crops through understanding C4-Rubisco biogenesis and catalytic diversity. Current Opinion in Plant Biology, 31, 135–142. https://doi.org/10.1016/j.pbi.2016.04.002
- Shimono, H., Okada, M., Yamakawa, Y., Nakamura, H., Kobayashi, K., & Hasegawa, T. (2009). Genotypic variation in rice yield enhancement by elevated CO2 relates to growth before heading, and not to maturity group. Journal of Experimental Botany, 60(2), 523–532. https://doi.org/10.1093/jxb/ern288
- Suzuki, Y., Nakabayashi, K., Yoshizawa, R., Mae, T., & Makino, A. (2009). Differences in expression of the RBCS multigene family and rubisco protein content in various rice plant tissues at different growth stages. Plant & Cell Physiology, 50(10), 1851–1855. https://doi.org/10.1093/pcp/pcp120
- Tcherkez, G. G., Farquhar, G. D., & Andrews, T. J. (2006). Despite slow catalysis and confused substrate specificity, all ribulose bisphosphate carboxylases may be nearly perfectly optimized. Proceeding of the National Academy of Sciences of the United States of America, 103(19), 7246–7251. https://doi.org/10.1073/pnas.0600605103