ABSTRACT
Previous research into the effects of ultrafine bubbles (UFB) on plant growth have been contradictory. To facilitate the resolution of these contradictions, the aim of the present study was to clarify the interspecific differences in growth responses among cereal/leguminous species under different levels of UFB concentrations. Seedlings of six species were grown hydroponically with three different UFB concentrations and two levels of plant nutrition to evaluate biomass and elongation growth. UFB growth promotion under zero-nutrition occurred in all species. Interspecific differences were noted in response to differing UFB concentration levels. Rice and soybean had higher above-ground biomass production at both low and high concentrations. Conversely, other crops exhibited promoted growth at only one of the concentrations. Negative effects occurred in full nutrient conditions except for root elongation. This study demonstrated that growth-promoting effects with UFB depended on the crop species being tested and the concentration of UFB used.
Graphical abstract
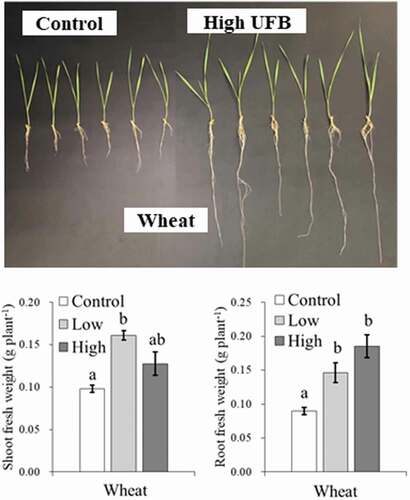
Introduction
Currently, experimental results with regard to the effects of ultrafine bubbles (hereinafter UFB) on plant growth are inconsistent. Some studies have reported growth-promoting effects (Ebina et al., Citation2013; Liu et al., Citation2016, Citation2017; Park & Kurata, Citation2009; Purwanto et al., Citation2019), while others revealed negative or statistically similar growth to the control in some agronomic traits (for example, Ahmed et al., Citation2018; Minamikawa et al., Citation2015; Mochizuki et al., 2018). In case of the latter set of studies, the main purpose was to compare different gaseous sources of UFB (Ahmed et al., Citation2018), UFB effects on soil microorganisms (Minamikawa et al., Citation2015), and to apply a growth model (Mochizuki et al, 2018). Each study has made a valuable contribution to our basic understanding of UFB in agriculture. However, previous studies that obtained negative plant growth data possibly have not been published as original research articles by other researchers (personal communication). Growth inhibition could depend on the UFB concentrations, plant species, and/or other environmental conditions involved in plant growth. Therefore, it is necessary to clarify the growth conditions that always promote the growth of major crop species by UFB application. In our previous report on UFB effects on plant growth, the environmental conditions of the water culture such as nutrient levels, fluid movement, and aeration effects were investigated (Iijima et al., Citation2020). The results clearly showed that the growth promotion of young soybean seedling (8 days after germination) was remarkable under nutrient stress when cultured using a deionized water medium with UFB. However, under full nutrient conditions, no growth promotion was observed and an inhibition of shoot biomass production occurred in non-stirring conditions. Ahmed et al. (Citation2018) discussed the toxic threshold for negative plant growth, which implies the concentration of UFB itself may contribute to the plant growth inhibition/promotion effects. Not only the environmental conditions for plant growth but also the test plant species may contribute to the UFB growth inhibition/promotion effects. To date, the experimental evidence of both interspecific variations to UFB applications and/or UFB concentration effects on plant growth remain unknown. We aim to clarify the differences in growth response among major cereal/leguminous species in different levels of UFB concentrations. We standardized the environmental growth conditions for the different crop species in the young seedling stage with a standard nutrient culture in a growth room.
Materials and methods
This short-term experiment was performed using the same conditions described in our previous report (Iijima et al., Citation2020). Briefly, plants were grown in different levels of Hoagland solution in a plant growth room at 28/23°C day/night temperature, under 14 h photoperiod and 318 ± 2 µmol m−2 s−1 of photosynthetically active radiation (PAR) at the top canopy level. The cereals wheat (Triticum aestivum ‘Norin 61ʹ), rice (Oryza sativa ‘Nipponbare’), and maize (Zea maize ‘Launchar 82ʹ) and legumes soybean (Glycine max ‘Fukuyutaka’), cowpea (Vigna unguiculate ‘Akadane sanshaku’), and adzuki bean (Vigna angularis ‘Dainagon’) were the major food crop species used to evaluate interspecific responses to UFB application. All of these are well known and widely used commercial cultivars in Japan. UFB water was created using an Ultra-fine Bubble Generator (EAT-SWHI, Eatech Co. Ltd., Japan). The bubble-size distribution was measured by nanoparticle tracking analysis using NanoSight LM10 (Malvern Panalytical, Tokyo, Japan). The bubble number concentration with particle sizes from 20 to 1,000 nm (hereinafter UFB concentration) changes depending on the number of times deionized water circulates in the generator. Three levels of UFB concentration treatments were set up: control (zero circulation), low (1 time circulation), and high (10 times circulation). As shown in , the UFB concentration of the low and high were 2.23x107 ml−1 and 7.07x107 ml−1, respectively. Therefore, the UFB concentration for the high treatment is 3.2 times higher than that for the low treatment. The average diameter of UFB was 220 and 156 nm for low and high treatments, respectively. Two nutrient levels were used in this experiment, zero and full. Zero nutrition is a nutrient stress condition where plants are grown in de-ionized water without any nutrients added during the whole experimental period. Full nutrition treatments were stress-free ideal nutrient supply conditions where plants were grown in 1/4 strength Hoagland solution for the first 4 days and then increased to 1/2 strength Hoagland solution for the remainder of the growth period. Six seedlings were grown in each container, and the effects of the two factors were analyzed for each crop species. To simplify the environmental conditions, we included continuous aeration (2.0 L air min−1 5 L container−1) and stirring (1,500 rpm) by a magnetic stirrer during the experimental period. The solution was replaced every two days. As observed in our previous study (Iijima et al., Citation2020), UFB treatment did not affect pH, electrical conductivity (EC), and dissolved oxygen (DO) during seedling growth. Pre-germination was done at 30°C in the dark for 28, 30, 48, 28, 30, and 48 hours for wheat, rice, maize, soybean, cowpea, and adzuki bean, respectively, as identified by preliminary trials. Plants were harvested at 12 and 8 days after sowing for cereals and legumes, respectively. At sampling, the length and weight of both shoot and root were measured as the agronomic parameters. For root elongation data, we measured the embryo origin roots, i.e. seminal roots (cereals) or tap roots (legumes). For wheat and maize, the longest root was measured among the six or three seminal (adventitious) roots. All statistical analyses were performed using Excel Statistics Version 2012 software (SSRI). Treatment effects were evaluated using a two-way Analysis of Variance of each plant species (Supplemental Table 1). Tukey–Kramer multiple comparison tests were conducted among the three UFB concentration treatments.
Figure 1. Bubble size distribution in de-ionized water (control), in water containing two levels of UFBs (Low, 2.23x107 ml−1; High, 7.07x107 ml−1). The tracking motion picture recorded every 30 s and distributions were calculated using Nanosight LM10. Bubble size distribution was measured three times for each condition, and the average values are provided.
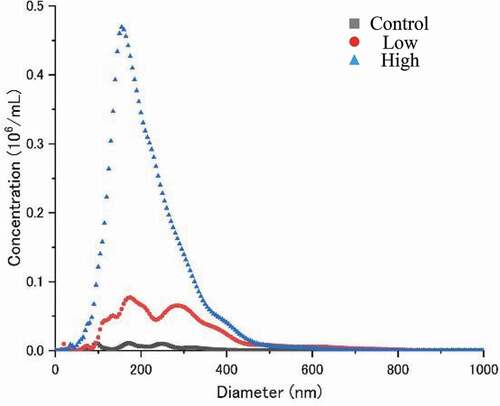
Results and discussion
illustrates typical plant responses to UFB application. The effects of UFB were evident for both root and shoot elongation. As shown in , the effect of UFB concentration on biomass production differed depending on the level of medium nutrition and crop species. First, shoot growth promotion under zero-nutrition ( (a)) occurred in all six species tested. This is consistent with previous research results (Iijima et al., Citation2020). A clearly defined interspecific difference was observed in the UFB concentration effects. Wheat, rice, cowpea, and adzuki bean showed growth promotion at the low UFB concentration. At the high UFB concentration for these species, however, the growth was either intermediate between the control and low (wheat and rice), or equivalent to the control (cowpea and adzuki bean). Conversely, maize and soybean were promoted by high concentrations. In summary, the above-ground biomass production showed that rice and soybean growth was promoted by both low and high UFB concentrations. The other crops showed growth promotion in only one treatment (low or high UFB). Our results suggest that if an experiment is conducted using only maize with a lower level of UFB concentration, the experimental results may lead to the conclusion that UFBs did not promote crop growth. If the experiment used a higher concentration of UFB, we may conclude that UFB effects do not occur in wheat, cowpea, and adzuki bean. The only exception is soybean, as its growth was promoted by both UFB concentrations. Therefore, results depend on the species and the concentration of UFB used, and the growth-promoting effects of UFB may or may not be confirmed. This may explain the inconsistency in plant growth responses to UFBs reported in previous studies. For example, small (or statistically insignificant) enhancement (Mochizuki et al., Citation2019) and significant inhibition (Ahmed et al., Citation2018) have been reported for leaf development of seedling tomatoes using air UFB.
Figure 2. Images of wheat seedlings grown in the control non-UFB water (nUFB) and higher concentration (7.07x107 ml−1) UFB water (UFB) with zero nutrients (12 days after seeding).
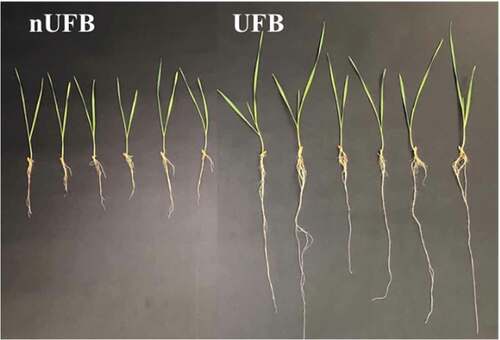
Figure 3. Biomass production of six crop species for the control (de-ionized water), low (2.23x107 ml−1), and high (7.07x107 ml−1) UFB concentrations under zero and full nutrient levels. Data are means ± standard error (SE) (n = 6). Means followed by the same lowercase letter were not significantly different at P < 0.05, according to Tukey-Kramer multiple comparison test.
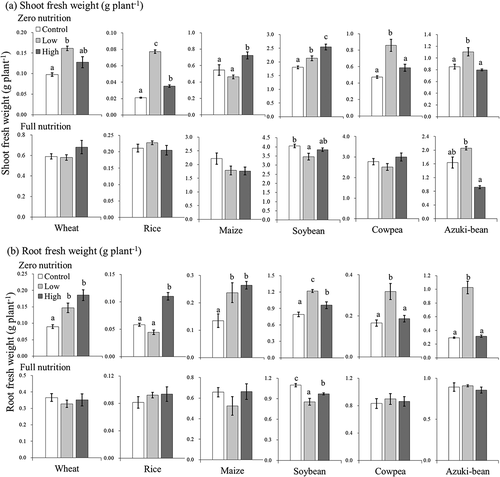
Similarly, for root biomass at zero nutrition ( (b) upper), experiments using rice, cowpea, and adzuki bean lead to the conclusion that growth promotion was not observed at either low or high concentrations. Conversely, in full nutrition ( (a) lower, (b) lower), the growth promoting effects of UFB were not observed in both shoot and root biomass for all the species tested, consistent with our previous research (Iijima et al., Citation2020). In soybean shoot growth, UFB even showed statistically suppressive effect in the low level UFB concentration, which supports the result of tomato grown in air UFB (Armed et al., 2018).
summarizes the effects of UFB concentration on the elongation growth of both shoots and representative roots using the longest seminal root (cereals) and taproot (legumes). First, in zero nutrition, the growth response of plant height and the longest root length were similar to the biomass production of shoot and roots, respectively. The only exceptions were wheat (plant height), and rice and soybean (roots). In the full nutrition treatments, however, wheat, soybean, and adzuki bean showed slight promotion of root or shoot elongations in UFB. This would agree with previous studies that showed positive effects of UFB in water culture (Ebina et al., Citation2013; Park & Kurata, Citation2009). At present, the reason for this phenomenon remains unknown. The results of this study suggest that the crop growth-promoting effect of UFB may be limited, especially under hydroponic conditions where sufficient nutrients are always available, such as in plant factories. However, due to the complex heterogeneity of soils, nutrient stresses may occur. To assess the impact of UFB on agriculture, it is therefore essential to study crop performance under field conditions.
Figure 4. Elongation growth of six crop species among control (de-ionized water), low (2.23x107 ml−1), and high (7.07x107 ml−1) UFB concentrations under zero and full nutrient levels. Data are means ± standard error (SE) (n = 6). Means followed by the same lowercase letter were not significantly different at P < 0.05, according to Tukey-Kramer multiple comparison test.
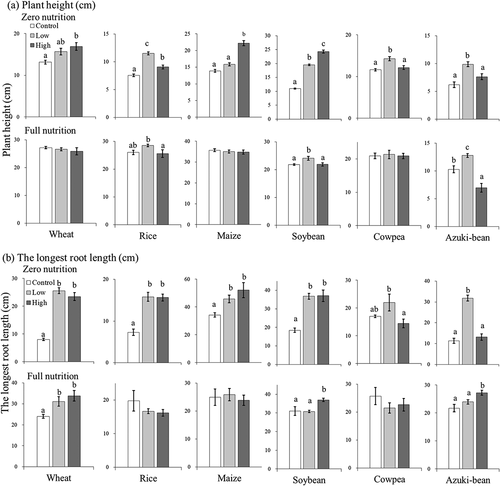
In conclusion, this study clearly demonstrated that there are UFB concentrations that promote or suppress crop growth, depending on the crop species. The mechanism of both interspecific differences and contrasting responses to the nutrient conditions are unknown at the moments. Perhaps the thresholds of endogenous reactive oxygen species (Liu et al., Citation2016) and/or plant growth regulators produced by the presence of UFB differs between species. We expected that a comprehensive diagnosis of endogenous production in both plant growth regulators and reactive oxygen species may improve our understanding of the effects of UFB on plant growth.
Acknowledgments
We thank Mr. Eisei Sakata, Mr. Yasuhiro Kodoi and Teruki Kuroki of Eatech Co. Ltd for their supports for using an Ultra-fine Bubble Generator. We also thank Ms. Y. Horiguchi, Mr. A. Sunami, Mr. T. Ma, Mr. K. Yamamoto, Mr. Y. Miyake and the other members of crop science laboratory, Faculty of Agriculture, Kindai University for their support.
Disclosure statement
No potential conflict of interest was reported by the author(s).
References
- Ahmed, A. K. A., Shi, X., Hua, L., Manzueta, L., Qing, W., Marhaba, T., & Zhang, W. (2018). Influences of air, oxygen, nitrogen, and carbon dioxide nanobubbles on seed germination and plant growth. Journal of Agricultural and Food Chemistry, 66(20), 5117–5124. https://doi.org/https://doi.org/10.1021/acs.jafc.8b00333
- Ebina, K., Shi, K., Hirao, M., Hashimoto, J., Kawato, Y., Kaneshiro, S., Morimoto, T., Koizumi, K., Yoshikawa, H., & Balcazar, J. L. (2013). Oxygen and air nanobubble water solution promote the growth of plants, fishes, and mice. PLoS ONE, 8(6), e65339. https://doi.org/https://doi.org/10.1371/journal.pone.0065339
- Iijima, M., Yamashita, K., Hirooka, Y., Ueda, Y., Yamane, K., & Kamimura, C. (2020). Ultrafine bubbles effectively enhance soybean seedling growth under nutrient deficit stress. Plant Production Science, 23(3), 366–373. https://doi.org/https://doi.org/10.1080/1343943X.2020.1725391
- Liu, S., Oshita, S., Kawabata, S., Makino, Y., & Yoshimoto, T. (2016). Identification of ROS produced by nanobubbles and their positive and negative effects on vegetable seed germination. Langmuir, 32(43), 11295–11302. https://doi.org/https://doi.org/10.1021/acs.langmuir.6b01621
- Liu, S., Oshita, S., Kawabata, S., & Thuyet, D. Q. (2017). Nanobubble Water’s Promotion Effect of Barley (Hordeum vulgare L.). Sprouts Supported by RNA-Seq Analysis. Langmuir, 33(43), 12478–12486. https://doi.org/https://doi.org/10.1021/acs.langmuir.7b02290
- gMinamikawa, K., Takahashi, M., Makino, T., Tago, K., & Hayatsu, M. (2015). Irrigation with oxygen-nanobubble water can reduce methane emission and arsenic dissolution in a flooded rice paddy. Environmental Research Letters, 10(8), 084012. https://doi.org/https://doi.org/10.1088/1748-9326/10/8/084012
- Mochizuki, Y., Zhao, T., Kanematsu, W., Kawasaki, T., Saito, T., Ohyama, A., Nakano, A., & Higashide, T. (2019). Application of a growth model to validate the effects of an ultrafine-bubble nutrient solution on dry matter production and elongation of tomato seedlings. The Horticulture Journal, 88(3), 380–386. https://doi.org/https://doi.org/10.2503/hortj.UTD-055
- Park, J. S., & Kurata, K. (2009). Application of microbubbles to hydroponics solution promotes lettuce growth. Horticulture Technology, 19(1), 212–215. https://doi.org/https://doi.org/10.21273/HORTSCI.19.1.212
- Purwanto, Y. A., Maulana, N. N., Sobir, S., Naibaho, N., & Naibaho, N. (2019). Effect of ultrafine bubbles water on seed germination. IOP Conference Series: Earth and Environmental Science, 355(1), 012073. https://doi.org/https://doi.org/10.1088/1755-1315/355/1/012073