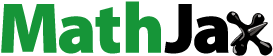
ABSTRACT
To understand the effect of heat stress on morphology and physiology of the cotton, eight cotton genotypes with their 15F1 hybrids (five lines, three testers) were grown in the field conditions under randomized complete block design (RCBD) with two treatments i.e. normal and high-temperature stresses with two replications followed by split-plot arrangement. Data were collected for biochemical and yield-related parameters. Mean values of all studied traits were reduced significantly under high-temperature stress whilst the mean value of lint%, catalase activity, total soluble proteins, peroxidase, and carotenoids were increased under high-temperature. Under both conditions, the number of bolls, boll weight, seed cotton yield, lint index, seed mass per boll, hydrogen peroxide content, catalase activity, total soluble proteins, carotenoids, and chlorophyll contents had high heritability values along with high genetic advance percent mean which revealed, these traits were controlled by additive gene action. The lint%, seed index, short fiber index, fiber strength, fiber fineness, upper half mean length and peroxidase activity had high heritability with moderate genetic advance under heat stress conditions which showed, these traits were controlled by non-additive gene action. Under both temperature conditions, FH-458, IUB-65, CRS-2, and FH-313 were good general combiners for physicochemical and yield-related traits. The cross combination of IUB-013× CRS-2 and FH-458× FH-313 were good specific combiner for plant height and seed cotton yield whilst for fiber quality and biochemical traits, the best specific combiners were VH-329× FH-313 and IUB-013× CRS-2. These identified parents and cross combinations might be used for improving already present commercial varieties under high-temperature stress.
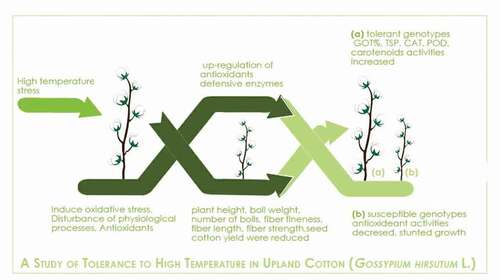
1. Introduction
Cotton is a key fiber crop of tropical and subtropical regions. It is the main source of fiber, oil and feed for livestock (M. A. Ali et al., Citation2019; R. P. Singh et al., Citation2007). It provides the basic raw material for textile industries as a major source of fiber (Imran et al., Citation2011). Ecologically, high-temperature is the major cause to diminish cotton yield worldwide (Abro et al., Citation2015). Cotton yields are affected up to 10–17% with each 1°C increase in temperature (Pettigrew, Citation2008). Dabbert and Gore (Citation2014) reported that in the near future cotton production will be affected by numerous abiotic stresses i.e. prolonged drought conditions and extremely high-temperature. Cotton is cultivated in areas of Pakistan, where temperature reached up to 50°C in summer and cotton plant faces extreme high-temperature which significantly reduces seed cotton yield, fiber quality and plant population (Rahman, Citation2006). This temperature is 20°C greater than the optimum temperature essential for normal growth and development of cotton plants and reduced yield and fiber quality. The maximum cotton growth was observed within the temperature range of 20–30°C, whilst the best thermal range for enzymatic activity, germination, flowering, and lint production is 28 ± 3°C (Burke & Wanjura, Citation2010). Heat stress adversely affects pollen viability, anthesis and fruit setting ultimately affect yield and quality (J. Farooq et al., Citation2015; N. Khan et al., Citation2014).
Heat Stress affects different physiological and biochemical processes, such as induction of reactive oxygen species (ROS), denaturation of proteins, nucleic acids, lipids, and other molecules (Qu et al., Citation2013). Heat stress triggers ROS (Mishra et al., Citation2008) which is metabolized the up-regulation of antioxidants defensive enzymes activities such as catalase (CAT), peroxidase (POX), and superoxide dismutase (SOD) (Phung & Jung, Citation2015). It is now timely, to assess genetic variability within genotypes under heat stress. High-temperature results in heat stress and drought stress. These are emerging problems that may affect cotton production in the future (Rehman et al., Citation2018; Zhang et al., Citation2014). The influence of high-temperature can be reduced by screening and breeding of pre-existing germplasm and evaluating plant types that are high yielding and can withstand harsh environmental conditions is the most important sustainable way to handle abiotic stresses i.e. heat stress. The variation among genotypes may occur due to environmental changes, natural selection or gene flow. Therefore, the selection of genotypes should be done on genetic basis rather than phenotypic or geological basis. To select genotypes for improving crop traits, the genetic divergence present among genotypes should be exploited through morphological characters as they are greatly influenced by the environment (Aarthi et al., 2018).
Heritability is the ratio of the genotypic variance to the phenotypic variance. It describes the portion of phenotypic variance due to genotypic variations. It gives a ratio of the additive variance to the phenotypic variance. Genetic advance referred to the enhancement in the performance of genotypes over the parent genotypes at selection intensity. Heritability itself provided no clue about the genetic gain as a result of selection. Heritability along with high genetic advances provided the plant breeder with chances of efficient selection. At selection pressure, the extent of genetic gain changes according to available heritability (Anjani et al., 2020). Correlation study is an important factor in the development of selection strategy for a breeding programs. The information of association among characters is crucial for the selection procedure. This association may be the result of genetic and environmental factors (Amanu, 2020).
The objective of the present study was to assess the variations and genetic diversity in cotton genotypes for yield and fiber traits and analyze the association of seed cotton yield with other characters and set a benchmark to identify high-temperature tolerant and sensitive genotypes in cotton.
2. Materials and methods
2.1. Experimental Design
Eight cotton genotypes were obtained from the Department of Plant Breeding and Genetics, University of Agriculture, Faisalabad. Healthy cotton seeds were grown in randomized complete design in clay pots in glasshouse of the department (N 31° 25ʹ 46.8048”, E 73° 4ʹ 14.3112”) during November 2018. The optimal conditions were fully observed to get good germination. At flowering stage, parents were crossed in line × tester mating design. Five heat-tolerant genotypes were taken as lines and three heat-sensitive genotypes were taken as testers. During the next normal cotton growing season in June 2019, F1 seeds of fifteen crosses and their parents () were sown in the field under two conditions i.e. normal and high-temperature stress following the split-plot arrangement with randomized complete block design (RCBD) in duplicate manner. All agronomic practices were followed uniformly throughout the season.
Table 1. List of lines, tester and their cross combinations used in experiment
2.2. High-temperature stress
High-temperature stress was applied in September for 12 days at 50% flowering. The temperature was raised (5–6°C) by constructing a tunnel using polythene sheets with help of bamboo arcs and plastic ropes in the daytime and uncovered at night. The temperature in the tunnel was measured by using a mercury-based maximum and minimum thermometer. The data regarding humidity inside and outside the tunnel is given in Fig. 3. After the application of high-temperature stress, data were recorded regarding biochemical traits for analysis. The maximum and minimum temperature recorded during 12 days of stress (). The temperature outside of the tunnel was taken from agricultural meteorology cell, Department of Agronomy, UAF.
2.3. Biochemical and yield-related parameters
After 12 days of high-temperature exposure, leaf samples were collected from each genotype for quantification of hydrogen peroxide content, catalase activity, peroxidase activity, total soluble proteins, chlorophyll contents, and carotenoids contents in the leaves. At maturity data was collected regarding plant height (P.H.), height to node ratio (HNR), number of bolls, boll weight (BW), seed index (SI), seed cotton yield (SCY), GOT%, number seed per boll, seed mass per boll, from five plant of each genotype.
Plant height (cm): The final height of the plant was measured with a measuring rod from the first cotyledonary node to the apical bud, when the growth ceased.
Bolls per plant: The number of effective mature bolls from all the picks were counted and the cumulative record was maintained for each plant separately.
Seed index (g): Seed index is the 100 seed weight. 100 cotton seeds were taken at random for each sample from each genotype and weighed.
2.3.1. Boll weight (g)
The average weight per boll was obtained by dividing the total yield of seed cotton per plant by the number of bolls picked from every plant.
2.3.2. Lint (%)
Ginning out-turn (lint %) defined as the weight of lint obtained from a given weight of seed cotton and is expressed as percentage. Samples of seed cotton obtained from individual plants were ginned separately with ginning machine and weighed in the cotton laboratory. Lint-weighed values were used for the determination of GOT percentage by using the formula given below.
2.4. Fiber quality traits
A representative sample from seed cotton was weighed and ginning was done by single roller ginning machine (Testex, Model: TB510 C). The seeds were separated from the lint and ginning out turn was obtained by dividing the weight of lint in a sample by seed cotton weight of the sample, which was expressed in percentage. Lint was further processed to take out the parameters of lint mass per boll (g), fiber strength (g/tex), Reflectance, upper half mean length (mm) and uniformity ratio with high volume instrument (HVI-900, USTER, USA).
2.5. Biochemical Traits
Young leaves were collected from the top of the plant for biochemical analysis from each genotype. Enzyme extraction was performed with 0.5 g of cotton leaf sample was cut off with the help of leaf pincher and crushed in chilled condition with 10 ml of phosphate buffer (pH 7.8). For grinding of sample mortar and pestle were used under buffer solution. Prepared mixture was then centrifuged for 5 min at 1400 rpm. Residues were discarded and the supernatant was used for enzyme determination. The extracted material was stored at 4 °C (Terras et al.1993). The biochemical attributes were determined by using UV spectrometer at different wavelengths.
2.5.1. Hydrogen peroxide (µmol/g-FW)
H2O2 content was determined by following the protocol purposed by (Velikova et al., Citation2000). A half-gram Cotton leaf was homogenized with 5 ml trichloroacetic acid (TCA) of 0.1% (W/V). The homogenate was centrifuged at 14,000 × g for 10 min and 0.5 ml of the supernatant was added to 0.5 ml of 10 mM potassium phosphate buffer (pH 7.0) and 1 ml potassium iodide (KI, 1 M). Absorbance was taken through a spectrophotometer at 390 nm wavelength (NanoDrop™ 8000 Thermo Fisher Scientific) using deionized water as blank.
2.5.2. Catalase (U/mg protein)
Enzyme extract (0.1 ml) was mixed with 3 ml of the reaction mixture, containing 5.9 mM H2O2 and 50 mM potassium phosphate buffer (7.0 pH). CAT activity was measured at the wavelength of 240 nm (Liu et al., Citation2009).
2.5.3. Peroxidase (U/mg protein)
POD solution containing 0.1 ml enzyme extract, 40 mM hydrogen peroxide (H2O2), 50 mM potassium phosphate buffer (pH 5) and 20 mM guaiacol. Absorbance was taken through a spectrophotometer at 470 nm wavelength(Liu et al., Citation2009).
2.5.4. Total soluble proteins (mg/g- FW)
100 µl of enzyme extracted and 5 ml of Bradford reagent mixed. Absorbance was measured at the wavelength of 595 nm by a spectrophotometer(Bradford, Citation1976).
2.5.5. Chlorophyll contents and carotenoids assay
Half gram of cotton leaf sample crushed in 8–10 ml of 80% acetone (v/v) and then homogenization was done through filter paper and absorbance of the final solution taken at 645 and 663 nm (Arnon, Citation1949). The chlorophyll a, chlorophyll b and carotenoids were quantified as under.
Carotenoids (mg/g FW) = Acar/ Em × 100
Acar = O.D 480 + 0.114 (O.D 663) – 0.638 (O.D 645)
Where
W = weight of leaf sample, V = volume of sample, Em = 2500
2.6. Statistical analysis
Collected data were analyzed through analysis of variance as specified by (Steele et al., Citation1997) to estimate genetic variability. The relationship of yield with other attributes is studied by correlation analysis by using Statistics 8.1. One trait might cause an increase or decrease in another trait due to the association present among them. The estimation of heritability was determined using the procedure proposed by (Falconer & Mackay, Citation1996). Genetic advance was determined by using the procedure suggested by Poehlman and Sleper (Citation1995) at 20% selection intensity. Line × tester analysis of (Kempthorne, Citation1957) was used for the estimation of combining ability effects of parental genotypes and crosses.
3. Results
3.1. Genetic variability
Mean squares of analysis of variance for yield and yield related attributes, fiber quality, and biochemical traits under normal and high-temperature stress condition showed that genotypes, treatments and G × T interaction exhibited maximum significant differences for all traits except treatment was non-significant for seed index, seeds per boll, seed mass per boll, short fiber and fiber strength and G × T interaction was non-significant for height to node ratio, lint percentage and maturity index. High-temperature stress adversely affects all the traits ().
Table 2. Mean square values for the influence of high-temperature stress on different agronomic, within boll yield components, fiber quality and physiological traits of cotton genotypes
Table 3d. Results for components of variability, genetic advance mean and heritability estimates of various traits under normal and high-temperature stress conditions
Table 3f. Results for components of variability, genetic advance mean and heritability estimates of various traits under normal and high-temperature stress conditions
Table 4b. Correlation among various biochemical traits of cotton genotypes under high-temperature stress condition
Table 5b. Correlation among various morphological, within boll yield components and fiber quality traits of cotton genotypes under high-temperature stress condition
3.1.1. General combining ability effects under normal and high-temperature stress conditions
Combining ability estimates for plant height showed that FH-458, IUB-013, FH-313 and Shahkar were best combiners under both conditions. For height to node ratio the line NIAB-1048 was good combiner under normal conditions whilst lines IUB-013 and FH-458 displayed good GCA values under high-temperature stress. For number of bolls and boll weight FH-458 showed best GCA effects under both conditions. The FH-458, IUB-65, FH-313, and Shahkar showed higher GCA effects for seed cotton yield under high-temperature stress conditions. For lint% and lint index best GCA was indicated by VH-329 under both conditions. For seeds per boll and lint mass per boll IUB-65 showed best GCA effects under normal conditions and among testers CRS-2, FH-313 and Shahkar were best combiner under normal and high-temperature, respectively. For short fiber and reflectance best line was IUB-013 under normal conditions. Among testers FH-313 was best combiner for most of fiber and within boll yield components under normal as well as high-temperature stress. For hydrogen peroxide content best combiners were IUB-013, IUB-65, CRS-2 and Shahkar under normal condition. Whilst under high-temperature all lines exhibited non-significant GCA effects and among testers good general combiner was FH-313. For catalase activity among parents best general combiners were FH-458, IUB-013 IUB-65 and FH-313 under both normal and high-temperature stress conditions. For peroxidase activity best GCA effects observed in FH-458, VH-329, CRS-2 and Shahkar exhibited higher GCA effects under both conditions. Under both conditions, higher GCA estimates were observed for total soluble proteins in FH-458, IUB-013, IUB-65 CRS-2 and Shahkar under both conditions. The line NIAB-1048 and tester CRS-2 displayed best GCA effects under high-temperature stress for chlorophyll a & b contents. For carotenoids contents best GCA effects exhibited by FH-458 under both conditions and in testers Shahkar showed best GCA value under high-temperature stress condition (Table S1 and S2).
2. Specific combining ability effects of crosses under normal and high-temperature stress conditions
Estimates for SCA showed that crosses IUB-65 × CRS-2 and FH-458 × FH-313 displayed significantly positive SCA effects for plant height under both conditions. For height to node ratio, the cross combination of VH-329 × FH-313 showed higher SCA effects under both conditions. For number of bolls, boll weight best combination was IUB-65 × CRS-2 under normal conditions. For seed cotton yield best SCA effects were shown by FH-458 × FH-313, FH-458 × CRS-2, VH-329 × FH-313 and IUB-65 × CRS-2 under both conditions. For most of the fiber quality traits and within boll yield components best specific combinations were FH-458 × CRS-2, VH-329 × Shahkar and IUB-013 × FH-313 under normal conditions. For hydrogen peroxide contents the hybrid of FH-458 × FH-313 and IUB-65 × Shahkar exhibited best SCA effects under both conditions. For catalase activity and carotenoids contents, the cross combination of IUB-65 × CRS-2 and IUB-013 × CRS-2 exhibited significantly positive SCA effects under both conditions. For peroxidase activity and carotenoids contents, FH-458 × FH-313 showed best positive SCA effects under both conditions. For total soluble proteins contents FH-458 × FH-313 displayed best SCA effects under both conditions. SCA effects for chlorophyll contents (a & b) revealed that IUB-65 × CRS-2 and FH-458 × FH-313 showed maximum positive significant effects under both conditions (Table S3 and S4).
Components of variability, genetic advance per percent mean and heritability estimates of various traits under normal and high-temperature stress conditions
Basic statistics including mean, minimum value, maximum value and coefficient of variation were calculated for all studied traits (-f). Maximum plant height and height to node ratio was observed in IUB-65 among lines under normal condition whereas, among crosses FH-458 × FH-313 show max values under both conditions. FH-458 × FH-313 exhibited the highest values for most of the yield-related parameters. The quality of fiber is of paramount importance in cotton breeding. High-temperature stress significantly affected the quality of fiber. Among lines IUB-013 and among crosses, the cross combination of FH-548 × FH-313 and VH-329 × CRS-2 were promising for fiber quality traits under both conditions. CV% provided information about the precision of experiment. Plant height, height to node ratio, boll weight, seed cottony yield, lint%, short fiber index, fiber strength, UHML, uniformity index, reflectance, catalase activity and chlorophyll contents had minimum CV% under normal stress conditions while number of bolls, seed index, fiber fineness, lint mass per boll, hydrogen peroxide content, carotenoids contents, peroxidases activity and total soluble proteins content had moderate CV% values. Lint index, seed per boll and seed mass per boll displayed high CV% values. Under high stress conditions plant height, height to node ratio, boll weight, lint%, seed index, short fiber index, fiber strength, fiber fineness, reflectance, UHML, uniformity index, catalase and peroxidase activities had minimum CV% values. Number of bolls, seed cotton yield, lint index, lint mass per boll, seeds per boll, seed mass per boll, total soluble proteins and carotenoids contents displayed moderate CV% while hydrogen peroxide content had highest CV% under high-temperature conditions. CV% was least for maturity index in both normal and high-temperature conditions (Table 3a, 3b, 3c, 3d & 3f). The phenotypic coefficient of variation was slightly higher than the genotypic coefficient of variation for all the traits under normal and high-temperature stress conditions. These results indicated less environmental influence on the studied traits. Plant height, boll weight, number of bolls, seeds per boll, seed cotton yield, seed mass per boll, lint index, short fiber index, catalase activity, hydrogen peroxide content, total soluble proteins content, chlorophyll contents and carotenoids contents displayed high heritability along with high genetic advance per percent mean under normal conditions (-f). Lint% and maturity index had high heritability with low genetic advance per percent mean while height to node ratio, seed index, UHML, fiber fineness and reflectance exhibited low heritability and genetic advance per percent mean. Lint mass per boll and peroxidase activity had low heritability with high genetic advance. Under high-temperature stress conditions, number of bolls, boll weight, seed cotton yield, lint index, seeds per boll, seed mass per boll, lint mass per boll, hydrogen peroxide content, catalase activity, total soluble proteins content, carotenoids and chlorophyll contents had high heritability values along with high genetic advance per percent mean while plant height, lint%, seed index, short fiber index, fiber strength, fiber fineness, UHML and peroxidase activity had high heritability with moderate genetic advance. Height to node ratio, maturity index, reflectance and uniformity index exhibited high heritability with low genetic advance per percent mean (-f).
Table 3a. Results for components of variability, genetic advance mean and heritability estimates of various traits under normal and high-temperature stress conditions
3.2. Correlation estimates of various traits under normal condition
The correlation coefficient revealed that seed cotton yield had highly significant and positive association with all biochemical attributes (). Height to node ratio, boll weight and total number of bolls while positively significant relationship was found with fiber maturity. Plant height exhibited significantly positive relationship with total number of bolls, lint mass per boll and reflectance. Boll weight was linked positively and significantly with total number of bolls and lint % while highly positive and significant relation was found with height to node ratio, seed index, fiber fineness and upper half mean length. Height to node ratio was associated positively and highly significantly with fiber fineness, lint % and UHML. Seed index displayed highly significant and positive relation with lint %, lint mass per boll and micronaire value. Seed mass per boll was linked negatively and significantly with total number of bolls and fiber maturity. Lint index was found to have negative and significant relation with seed mass per boll and number of seeds per boll. Lint mass per boll had highly significant and positive association with lint %, fiber maturity and fiber fineness. Lint % had a considerable and positive relationship with UHML, fiber maturity and fiber fineness. Uniformity index displayed negative significant relation with total number of bolls and UHML while short fiber index was linked negatively and significantly with boll weight, boll number, lint mass per boll and upper half mean length. Reflectance was linked negatively and significantly with seed index ().
Table 4a. Correlation among various biochemical traits of cotton genotypes under normal condition
Table 5a. Correlation among various morphological, within boll yield components and fiber quality traits of cotton genotypes under normal condition
Table 3b. Results for components of variability, genetic advance mean and heritability estimates of various traits under normal and high-temperature stress conditions
Table 3c. Results for components of variability, genetic advance mean and heritability estimates of various traits under normal and high-temperature stress conditions
3.3. Correlation estimates of various traits under high-temperature stress
The correlation coefficient provided information about the association of seed cotton yield with other morphological, biochemical and fiber traits. Seed cotton yield had positive and highly-significant relationship with all biochemical traits (), plant height, height to node ratio, total number of bolls and boll weight while seed index while seed mass per boll had significant association in positive manner with seed cotton yield. Lint% displayed negative and significant relationship with seed cotton yield. Boll weight has highly positive correlation with height to node ratio and seed index. Lint index, Micronaire value and upper half mean length was positively and significantly related with boll weight. Height to node ratio had highly significant relationship with plant height, seed index and uniformity index. Total number of bolls were positively and significantly associated with seed mass per boll, height to node ratio, and number of seeds per boll. The association of uniformity index was highly considerable and positive with lint %, short fiber index and height to node ratio while positive and significant relation existed with seed index, fiber maturity, upper half mean length and fiber strength. Upper half mean length displayed positively significant relationship with height to node ratio, total bolls, boll weight, fiber maturity and short fiber index while fiber strength had highly significant and positive association with UHML and fiber maturity. Highly positive and significant relationship was exhibited by reflectance with seed mass per boll and fiber maturity. Short fiber index had positively significant association with lint % and seed index ().
4. Discussion
Cotton yield is strongly influenced by biotic and abiotic stresses however principle ecological problem is heat that antagonistically diminishes cotton yield and fiber quality (Abro et al., Citation2015). Variability among different genotypes is the prime requirement for the development of high yielding stress-tolerant genotypes by natural or a vigilant selection in Gossypium hirsutum L (Ali & Khan, Citation2007; Chorshanbiev, Citation2020; Therthappa, Citation2005). To push forward breeding programs against high-temperature tolerance, effective knowledge about variability, heritability and combining ability is a necessary (Tang et al., Citation1996). Five lines and 3 testers were crossed in Line × Tester fashion (5 × 3) and 15 F1 hybrids were developed (Ali et al. 2014). It is pertinent to mention that it provides reliable information about the genetic architecture of inheritance pattern from its combining ability estimates (Karademir et al. 2009). The range of variation for the studied traits is determined by coefficient of variation. The variation helps in boosting the yield by accumulating desirable genes from genetically diverse genotypes. Moreover, coefficient of variation displays the accuracy of experiment. The genetic variation is highly affected by the changes in the environment. The characters having high GCV and PCV with low environmental effects are favorable for selection. The genotypes with such characters should be selected for the development of desired genotypes (Chaudhari et al., Citation2017; Kaleri et al. 2016). The phenotypic variance was greater than genotypic variance for all the studied traits. Environmental, genotypic and phenotypic variances were also calculated. GCV and PCV had almost similar values while the values of environmental coefficients were low as compare to GCV. Higher PCV than GCV was previously reported by (Ali et al. Citation2020; Harvesting, Citation2019; N. Khan et al., Citation2014). High genetic advance along with high heritability was observed indicating additive type of gene action which means selection of trait will be beneficial. Interestingly the similar results were observed by (Bhatti et al., Citation2020; Nawaz et al., Citation2019; M. Singh et al., Citation2019). Combining ability is an effective tool in plant breeding which indicates which lines combine well with each other and with other genotypes (Snider et al., Citation2010). General combining ability is due to additive gene actions, whereas specific combining ability is due to non-additive gene action. Among lines the best general combiners were FH-458 and IUB-65 and among testers the best general combiners were FH-313 and CRS-2 for most of the traits. The results suggested that the lines with high GCA effects for specific traits can be utilized in the breeding program for the development of high-temperature tolerant genotypes through hybridization followed by selection breeding (Saleem et al. 2009). Among crosses best specific combiners were IUB-013× CRS-2 and FH-458× FH-313 for plant height and seed cotton yield. For fiber quality and biochemical traits, the best specific combiners were VH-329× FH-313 and IUB-013× CRS-2. FH-458× FH-313 showed significant positive SCA effects for most traits under both conditions. Results for combining ability effects corelated with (Pundir et al., Citation2017). Under heat stress, plant height was adversely affected and decreased. However, height to node ratio was not affected much. Reduction in Plant height and no affect in height to node ratio results were also observed by (A. I. Khan et al., Citation2008). In our study, high-temperature stress decreased the boll size, boll weight and seed cotton yield. The reduction in boll size results in reduced the number of seeds per boll and number of fibers per seed (Snider et al. 2009). According to Zhao et al. (Citation2005) decline in number of bolls per plant, boll weight and decrease in seed cotton yield was observed under high-temperature stress. According to the Conaty et al. (Citation2012), reduction in seed cotton yield occurred when temperature surpasses 30°C. Hence revealed that yield decreased under high-temperature stress over the normal temperature of field that is above 35°C during cotton season. Seed cotton yield and high-temperature are negatively correlated as reported by Oosterhuis (Citation2002), because high-temperature having negative impacts on agronomical characters. Fiber attributes displayed high phenotypic variance in comparison to genotypic variance as reported by (Shakeel et al.,Citation2015).PCV and GCV values had almost similar trend for all fiber traits indicating less environmental influence on studied traits.
Plant responded to reactive oxygen species (ROS) by up-regulating antioxidants defensive enzymes activities such as peroxidase (POX), catalase (CAT), and superoxide dismutase (SOD) to metabolize ROS (Phung & Jung, Citation2015). In this study, with the rise of temperature, hydrogen peroxide contents increased however due to scavenging activity of catalase activity its negative effects were averted as reported in the finding of (Chalanika De Silva & Asaeda, Citation2017). The increase in total soluble proteins content and peroxidase (POX) activity was observed in high yielding cultivars. The genotypes that showed higher value for POX activity and total soluble proteins content were classified as heat tolerant genotypes. Similar findings were also observed in cotton (Gür et al., Citation2010), wheat (Sairam et al., Citation2000) and chickpea (Kaushal et al. 2011). Peroxidase activity metabolize hydrogen peroxide into water and oxygen which helps the plant to detoxify reactive oxygen species (Zafar et al., Citation2020). Peroxidase is one of the primary enzymes that detoxify hydrogen peroxide content inside the cytosol and chloroplasts other plant cells (Farooq, Citation2019).Carotenoids also increased under stress condition as they are important antioxidants for safeguarding of singlet oxygen (McElroy & Kopsell, Citation2009). Catalase (CAT) also plays a primary role in the antioxidant system where it converts the toxic hydrogen peroxide content into the water and oxygen. Total soluble proteins content indicated a significant positive correlation with CAT activity under both conditions. Under high-temperature stress the scavenging capacity of the antioxidants was seriously impaired that led to a substantial increase in H2O2 concentration (Poschenrieder et al., Citation2013). In most plants, higher levels of the activity of the above-mentioned antioxidant enzyme are considered as a heat tolerance mechanism. Indeed, previous studies have shown that within the same species, heat tolerant cultivars generally have enhanced or higher constitutive antioxidant enzyme activity under heat stress when compared with sensitive-cultivars. This has been demonstrated in numerous plant species such as cotton, wheat and chickpea. Several studies have demonstrated that high-temperature tolerant species show increased antioxidant enzyme activities in response to heat stress, whereas heat-sensitive species fail to do so. Thus, the evidence accumulated to data indicates that intrinsic antioxidant resistance mechanisms of plants may provide a strategy to enhance high-temperature tolerance. However, to achieve efficient selection of genetically transformed high-temperature tolerant plants, the mechanisms underlying the effects of high-temperature on the morphology, physiology, growth, and antioxidative responses of plants must first be identified.
5. Conclusions
These results are limited and consequently not generalized for those cotton-growing areas that face elevated temperature stress. However, based on information generated from this research work amongst parentsFH-458, IUB-6, CRS-2 and FH-313 and among crosses FH-458 × FH-313 might be used for improving already present commercial varieties for further breeding programs to develop better cultivars with high yield and enhance adaptability to extreme temperature stress.
Ethics approval and consent to participate
Not applicable.
Consent for publication
Not applicable.
Availability of data and material
Applicable.
Authors’ contributions
AM and Zafar MM wrote the initial draft of the manuscript. AS, HF, MK and AR made all necessary corrections and carried out final editing of the manuscript. AR, YY and MR proofread the manuscript. Final approval for publication was given by the group leader AS.
Acknowledgements
We are thankful to Iqrar Ahmad Khan, Vice Chancellor, University of Agriculture Faisalabad on providing us facilities to conduct the experiment.
Disclosure statement
No potential conflict of interest was reported by the author(s).
Additional information
Funding
References
- Abro, S., Rajput, M. T., Khan, M. A., Sial, M. A., & Tahir, S. S. (2015). Screening of cotton (Gossypium hirsutum L.) genotypes for heat tolerance. Pak. J. Bot, 47(6), 2085–2091.
- Ali, M., Kundu, S., Alam, M., Biswas, B., & Hasanuzzaman, M. (2020). Selection of Genotypes and Contributing Characters to Improve Seed Cotton Yield of Upland Cotton (Gossypium hirsutum L.). Asian Research Journal of Agriculture, 31-41.
- Ali, M. A., Farooq, J., Batool, A., Zahoor, A., Azeem, F., Mahmood, A., & Jabran, K. (2019). Cotton production in Pakistan. In Cotton Production (pp. 249).
- Ali, M. A., & Khan, I. A. (2007). Assessment of genetic variation and inheritance mode of some metric traits in cotton (Gossypium hirsutum L.). J. Agric. Soc. Sci, 3(4), 112–116.
- Arnon, D. I. (1949). Copper enzymes in isolated chloroplasts. Polyphenoloxidase in Beta vulgaris. Plant Physiology, 24(1), 1. https://doi.org/https://doi.org/10.1104/pp.24.1.1
- Bhatti, M. H., Yousaf, M. I., Ghani, M. A., A., & Shehzad, A. A. (2020). Assessment of genetic variability and traits association in upland cotton (Gossypium hirsutum L.). Int. J. Bot. Studies, 5(2), 148–151.
- Bradford, M. M. (1976). A rapid and sensitive method for the quantitation of microgram quantities of protein utilizing the principle of protein-dye binding. Ann. Biochem, 72(1–2), 248–254. https://doi.org/https://doi.org/10.1016/0003-2697(76)90527-3
- Burke, J. J., & Wanjura, D. F. (2010). Plant responses to temperature extremes. In Physiology of cotton, edited by Stewart, J M, Oosterhuis, D M, Heitholt, J J, Mauney, J R. (pp. 123–128). New York: Springer.
- Chalanika De Silva, H. C., & Asaeda, T. (2017). Effects of heat stress on growth, photosynthetic pigments, oxidative damage and competitive capacity of three submerged macrophytes. Journal of Plant Interactions, 12(1), 228–236. https://doi.org/https://doi.org/10.1080/17429145.2017.1322153
- Chaudhari, M., Faldu, G., & Ramani, H. J. A. I. B. (2017). Genetic variability, Correlation and Path coefficient analysis in cotton (Gossypium hirsutum L.). Adv. Biores., Vol 8(6), 226–233. DOI: https://doi.org/10.15515/abr.0976-4585.8.6.226233.
- Chorshanbiev, N. E. (2020). INHERITANCE AND VARIABILITY OF BOLL WEIGHT IN F1-F2 PLANTS OF FINE-FIBER COTTON VARIETIES. Theoretical & Applied Science, (7)(7), 180–185. https://doi.org/https://doi.org/10.15863/TAS.2020.07.87.41
- Conaty, W., Burke, J., Mahan, J., Neilsen, J., & Sutton, B. (2012). Determining the optimum plant temperature of cotton physiology and yield to improve plant-based irrigation scheduling. Crop Science, 52(4), 1828–1836. https://doi.org/https://doi.org/10.2135/cropsci2011.11.0581
- Dabbert, T., & Gore, M. A. (2014). Challenges and perspectives on improving heat and drought stress resilience in cotton. Journal of Cotton Science, 18(3), 393–409.
- Falconer, D., & Mackay, T. (1996). Introduction to quantitative genetics, Longman. England.
- Farooq, J., Mahmood, K., Rehman, A. U., Javaid, M. I., Petrescu-Mag, V., & Nawaz, B. (2015). High-temperature stress in cotton Gossypium hirsutum L. ELBA Bioflux, 7(1), 34–44.
- Farooq, M. A. (2019). The potential of breeding Gossypium hirsutum L. for salinity tolerance. University of Agriculture, Faisalabad.
- Gür, A., Demirel, U., Özden, M., Kahraman, A., & Çopur, O. (2010). Diurnal gradual heat stress affects antioxidant enzymes, proline accumulation and some physiological components in cotton (Gossypium hirsutum L.). African Journal of Biotechnology, 9(7), 1008–1015. https://doi.org/https://doi.org/10.5897/AJB09.1590
- Harvesting, I. W. (2019). Estimation of Phenotypic Coefficients of Variation (PCV), Genotypic Coefficients of Variation (GCV), Heritability and Genetic Gain for Yield and its Components in Rice Landraces of Odisha 181. International Journal of Agriculture, Environment and Biotechnology, 12(3), 181–185. DOI :https://doi.org/10.30954/0974-1712.08.2019.1.
- Imran, M., Shakeel, A., Farooq, J., Saeed, A., Farooq, A., & Riaz, M. (2011). Genetic studies of fiber quality parameter and earliness related traits in upland cotton (Gossypium hirsutum L.). Advances in Agriculture Botanics, 3(3), 151–159.
- Kempthorne, O. (1957). “An introduction to genetic statistics.”
- Khan, A. I., Khan, I. A., & Sadaqat, H. A. (2008). Heat tolerance is variable in cotton (Gossypium hirsutum L.) and can be exploited for breeding of better yielding cultivars under high-temperature regimes. Pakistan Journal of Botany, 40(5), 2053–2058.
- Khan, N., Azhar, F. M., Khan, A., & Ahmad, R. (2014). Measurement of canopy temperature for heat tolerance in upland cotton: Variability and its genetic basis. Pak. J. Agri. Sci, 51(2), 359–365.
- Liu, D., Zou, J., Meng, Q., Zou, J., & Jiang, W. (2009). Uptake and accumulation and oxidative stress in garlic (Allium sativum L.) under lead phytotoxicity. Ecotoxicology, 18(1), 134–143. https://doi.org/https://doi.org/10.1007/s10646-008-0266-1
- McElroy, J. S., & Kopsell, D. A. (2009). Physiological role of carotenoids and other antioxidants in plants and application to turfgrass stress management. New Zealand Journal of Crop and Horticultural Science, 37(4), 327–333. https://doi.org/https://doi.org/10.1080/01140671.2009.9687587
- Mishra, S., Heckathorn, S. A., Barua, D., Wang, D., Joshi, P., Hamilton, I. I. I., . E. W., & Frantz, J. (2008). Interactive effects of elevated CO2 and ozone on leaf thermotolerance in field‐grown Glycine max. J. Integrative. Plant Biol, 50(11), 1396–1405. https://doi.org/https://doi.org/10.1111/j.1744-7909.2008.00745.x
- Nawaz, B., Naeem, M., Malik, T. A., Muhae-Ud-Din, G., Ahmad, Q., & Sattar, S. (2019). Estimation of Gene Action, Heritability and Pattern of Association among Different Yield Related Traits in Upland Cotton. Int. J. Innov. Approach. Agric. Res, 3(1), 25–52. DOI: https://doi.org/https://doi.org/10.29329/ijiaar.2019.188.4.
- Oosterhuis, D. (2002). Day or night high-temperatures: A major cause of yield variability. Cotton Grower, 46(9), 8–9.
- Pettigrew, W. (2008). The effect of higher temperatures on cotton lint yield production and fiber quality. Crop Science, 48(1), 278–285. https://doi.org/https://doi.org/10.2135/cropsci2007.05.0261
- Phung, T.-H., & Jung, S. (2015). Alterations in the porphyrin biosynthesis and antioxidant responses to chilling and heat stresses in Oryza sativa. Biol. Plantarum, 59(2), 341–349. https://doi.org/https://doi.org/10.1007/s10535-015-0505-2
- Poehlman, J., & Sleper, D. (1995). “Breeding field crops 4th ed”. City: Panima Publishing Corporation, New Dehli.
- Poschenrieder, C., Cabot, C., Martos, S., Gallego, B., & Barceló, J. J. P. (2013). Do Toxic Ions Induce Hormesis in Plants?, Plant Sci. 212, 15–25. https://doi.org/https://doi.org/10.1016/j.plantsci.2013.07.012
- Pundir, S., Sangwan, O., Nimbal, S., Sangwan, R., Siwach, S., & Mandhania, S. (2017). Heterosis and combining ability for seed cotton yield and its component traits of desi cotton (Gossypium arboreum L.). Journal of Cotton Research Development, 31(1), 24–28.
- Qu, A.-L., Ding, Y.-F., Jiang, Q., & Zhu, C. (2013). Molecular mechanisms of the plant heat stress response. Biochem. Biophys. Res. Commun, 432(2), 203–207. https://doi.org/https://doi.org/10.1016/j.bbrc.2013.01.104
- Rahman, H.-U. (2006). Number and weight of cotton lint fibres: Variation due to high-temperatures in the field. Australian J. Agric. Res, 57(5), 583–590. https://doi.org/https://doi.org/10.1071/AR05135
- Rehman, A., Azhar, M., Shakeel, A., & Basra, S. (2018). Genetic analysis of some polygenic traits for drought tolerance in Gossypium Hirsutum L. JAPS: Journal of Animal & Plant Sciences, 28, 5.
- Sairam, R., Srivastava, G., & Saxena, D. (2000). Increased antioxidant activity under elevated temperatures: A mechanism of heat stress tolerance in wheat genotypes. Biologia Plantarum, 43(2), 245–251. https://doi.org/https://doi.org/10.1023/A:1002756311146
- Shakeel, A., Talib, I., Rashid, M., Saeed, A., Ziaf, K., & Saleem, M. F. (2015). Genetic diversity among upland cotton genotypes for quality and yield related traits. Pak. J. Agric. Sci, 52, 73–77.
- Singh, M., Singh, V., Yadav, G., & Kumar, P. (2019). Studies on variability, heritability (narrow sense) and genetic advance analysis for growth, yield and quality traits in pumpkin (Cucurbita moschata Duch. ex. Poir). J. Pharmacogn. Phytochem, 8(3), 3621–3624.
- Singh, R. P., Prasad, P. V., Sunita, K., Giri, S., & Reddy, K. R. (2007). Influence of high-temperature and breeding for heat tolerance in cotton: A review. Advances in Agronomy, 93(1), 313–385. https://doi.org/https://doi.org/10.1016/S0065-2113(06)93006-5
- Snider, J. L., Oosterhuis, D. M., & Kawakami, E. M. (2010). Genotypic differences in thermotolerance are dependent upon prestress capacity for antioxidant protection of the photosynthetic apparatus in Gossypium hirsutum. Physiologia Plantarum, 138(3), 268–277. https://doi.org/https://doi.org/10.1111/j.1399-3054.2009.01325.x
- Steele, R., Torrie, J., & Dickey, D. (1997). Transformations in Principles and Procedures of Statistics—A Biometrical Approach. McGraw-Hill.
- Tang, B., Jenkins, J., Watson, C., McCarty, J., & Creech, R. (1996). Evaluation of genetic variances, heritabilities, and correlations for yield and fiber traits among cotton F 2 hybrid populations. Euphytica, 91(3), 315–322. https://doi.org/https://doi.org/10.1007/BF00033093
- Therthappa, B. (2005). Application of multivariate techniques in evaluation of sunflower genotypes (Helianthus Annus L.). UAS, Dharwad India.
- Velikova, V., Yordanov, I., & Edreva, A. (2000). Oxidative stress and some antioxidant systems in acid rain-treated bean plants: Protective role of exogenous polyamines. Plant Science, 151(1), 59–66. https://doi.org/https://doi.org/10.1016/S0168-9452(99)00197-1
- Zafar, M. M., Razzaq, A., Farooq, M. A., Rehman, A., Firdous, H., Shakeel, A., Mo, H., Ren, M., Ashraf, M., & Youlu, Y. (2020). Genetic Variation Studies of Ionic and within Boll Yield Components in Cotton (Gossypium Hirsutum L.) Under Salt Stress. Journal of Natural Fibers, 1–20.
- Zhang, J., Zou, D., Li, Y., Sun, X., Wang, -N.-N., Gong, S.-Y., Zheng, Y., & Li, X.-B. (2014). GhMPK17, a cotton mitogen-activated protein kinase, is involved in plant response to high salinity and osmotic stresses and ABA signaling. J PloS One, 9(4), 95642. https://doi.org/https://doi.org/10.1371/journal.pone.0095642
- Zhao, D., Reddy, K. R., Kakani, V. G., Koti, S., & Gao, W. (2005). Physiological causes of cotton fruit abscission under conditions of high-temperature and enhanced ultraviolet‐B radiation. Physiologia Plantarum, 124(2), 189–199. https://doi.org/https://doi.org/10.1111/j.1399-3054.2005.00491.x