ABSTRACT
Frost injury is a concern in early-sown spring wheat (Triticum aestivum) in the Tokai region of Japan. To assess the efficacy of ground rolling as a technique for avoiding frost injury, we investigated its effects on the apical development and yield of ‘Ayahikari’ spring wheat. In both 2018–19 and 2019–20, rolling delayed spikelet initiation by 4–5 days, the start of internode elongation by 5–12 days, and jointing by 3–9 days, but did not affect the apical development rate, which depended on the thermal time. Ground rolling prevented frost injury in 2018–19 but not in 2019–20, probably because the warmer-than-average winter promoted earlier jointing, and so plants were exposed to later low temperatures in February. These results suggest that ground rolling is an effective method for avoiding frost injury in spring wheat during cold winters.
GRAPHICAL ABSTRACT
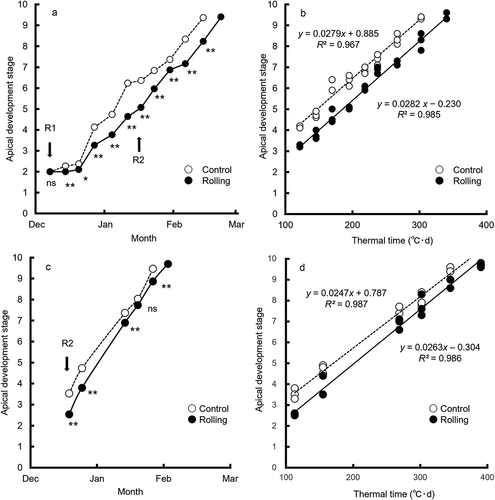
Introduction
Spring wheat cultivars are widely grown in the Tokai region (Gifu, Aichi, and Mie Prefectures) of Japan. In 2018–19, the area planted to spring wheat cultivars, such as ‘Ayahikari’ and ‘Kinuakari’, was ca. 12 000 ha, whereas that planted to winter wheat cultivars, such as ‘Satonosora’ and ‘Iwainodaichi’, was ca. 3000 ha. The optimum sowing time of spring wheat cultivars is narrower than that of winter wheat cultivars (Tanio et al., Citation2016), so as farmers have expanded their plantings, it has become more difficult to sow spring wheat cultivars within the optimum time. Late sowing decreases yield through lower biomass production and increases the risk of preharvest sprouting in the rainy season. Early sowing, on the other hand, increases the risk of frost injury in early spring because it advances internode elongation, which starts at the floret differentiation stage, owing to the low vernalization requirement of spring wheat cultivars (Inamura et al., Citation1958; Tanio et al., Citation2016). Frost injuries such as frozen, deformed, or sterilized spikes reduce grain yield and quality (Ohtani, Citation1942; Takeda, Citation1976; Uchijima, Citation1978a, Citationb). Therefore, farmers must delay spikelet initiation (the transition from vegetative to reproductive phase) in early-sown spring wheat cultivars.
Takeda (Citation1976) reported that when spikes grow to ≥3 mm, they become susceptible to frost damage, and the temperature at which the damage occurs depends on the developmental stage of spikes: spikes with a length of about 3 mm froze to death at a minimum temperature of ≤−2°C, and spikes with a length of 20–30 mm froze to death at a minimum temperature of ≤0°C; in addition, spikes 8–10 days before heading became sterile when exposed to temperatures below −1°C for several hours (Takeda, Citation1976). Inamura et al. (Citation1958) showed that early internode elongation caused the early emergence of young spikes above ground, exposing them to freezing temperatures.
The practice of pressing down on young wheat plants by ground rolling or trampling has been used in Japan for a long time, but the effect of trampling on yield has not yet been established (Ohtani, Citation1950). On the other hand, rolling at the seedling stage can delay spikelet initiation, apical development, and internode elongation (Ohtani, Citation1950), although the mechanisms responsible are not well understood. It would be expected, therefore, that ground rolling at the seedling stage could be used to avoid frost injury in early-sown cultivation of spring wheat.
Here, to test the possibility of avoiding frost injury by ground rolling, we sowed wheat early in 2018–19 and 2019–20 to determine the effects of rolling on the timing of spikelet initiation and on yield.
Materials and methods
Plant materials and cultivation methods
After rice harvest, we sowed ‘Ayahikari’ spring wheat (winter growth habit Class I–II) on 2 November 2018 and 1 November 2019 (2 weeks earlier than standard) in a drained paddy field at Tsu (34°46′N, 136°26′E, different plots about 200 m apart between years). With a shallow-tillage drill-seeder, seeds were sown in nine broad beds (2.1 m × 85 m) at ~180 seeds m−2 in rows 0.24 m apart at a depth of about 2 cm (Watanabe et al., Citation2009). Plants were grown according to the intensive production system of Tanio et al. (Citation2019). One bed each was used for the rolling and control treatments (). The rolling beds were rolled twice – at the three-leaf stage (GS13 of Zadoks et al., Citation1974) and five-leaf stage (GS15) in 2018–19 and at the two-leaf stage (GS12) and three-leaf stage (GS13) in 2019–20 () – with a rolling machine (a small tractor with two rollers measuring 45 cm diameter × 48 cm wide, total 114 kg, and a stand-on roller measuring 32 cm diameter × 40 cm wide, total 79 kg). Another five beds were used for a large-plot experiment (900 m2), rolled as above. The remaining two beds (either side of the rolling experiment) were used as borders (). Fertilizer was supplied at 2.0 g N m−2, 0.87 g P m−2, and 1.66 g K m−2 at sowing as a basal dressing, and at 6.0 g N m−2 at the three-leaf stage and 12.0 g N m−2 at the start of internode elongation as topdressing. Weeds and diseases were controlled with the standard agrochemicals used in the region. Ethephon was sprayed at 30 mg m−2 on 2 April 2019 and 13 March 2020 to prevent lodging.
Table 1. Calendar dates of sowing, rolling, and ethephon spraying.
Evaluation of developmental and agronomic characteristics, and statistical analysis
Three plots of 2.1 m × 5.0 m were allocated to each treatment. Ten plants per plot were dug out at about weekly intervals during winter (December to February). We examined their main shoots under a stereomicroscope and determined apical development stage, spike length, and stem length. After categorization by the method of Inamura et al. (Citation1955), the apical development stages were numbered from 2 to 10 for statistical analysis (see footnotes to for details). The mean of the 10 plants in each sample was calculated, unless otherwise noted. Agronomic characteristics () were measured in two or three plots. The date when 50% of spikes headed was recorded as the heading date. Frost injury (22 April 2019; 10 April 2020) was scored by eye according to NARC (Citation1986). The undisturbed central part of each plot (2.1 m × 1.0 m, 2.1 m2) was harvested by hand. The temperature in the field was recorded at three points, 5 cm above the ground, at 30-min intervals, and averaged. The accumulated daily mean temperature was calculated as thermal time (°C·d) above a base temperature of 0°C from the date of the 3-leaf-stage rolling in each year. Regression analyses (apical development stage x vs. log-transformed stem or spike length y; thermal time x vs. apical development stage y) were carried out according to the method of Kawabata (Citation1989), and the Student's t-test was used to compare agronomic characteristics between the rolling and control treatments.
Figure 2. Time course of apical development in (a) 2018–19 and (c) 2019–20, and regression analysis between apical development stage and thermal time in (b) 2018–19 and (d) 2019–20.
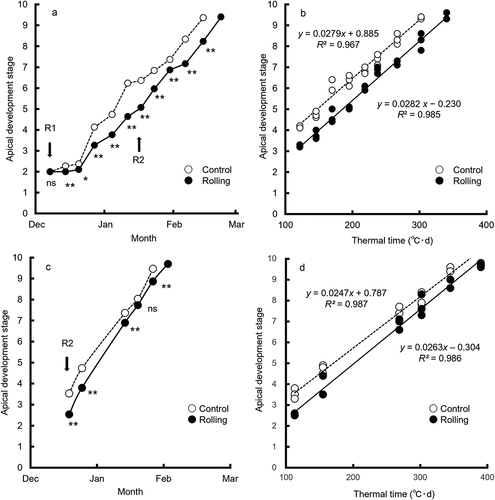
Table 2. Calendar dates of spikelet initiation, start of internode elongation, and jointing stages in rolling treatment and control estimated from regression analysis.
Table 3. Means heading and maturity dates; score of frost injury; lengths of spike and culm; and yield.
Results and discussion
Effect of rolling on apical development
Rolling significantly delayed apical development in both years (; P < 0.05). Apical development stage was highly correlated with thermal time in a single regression analysis in each treatment (; P < 0.001). In both years, the slopes were not significantly different between treatments but the intercepts were different, indicating that rolling delayed spikelet initiation but did not affect apical development rate. In addition, rolling after spikelet initiation (GS15) in 2018–19 had no effect on apical development rate over thermal time ().
The developmental delay due to rolling can be estimated as a developmental stage of nearly 1.0 in 2018–19, equivalent to 37°C·d of thermal time, and of nearly 0.7 in 2019–20, equivalent to 27°C·d of thermal time (). Rolling before spikelet initiation delayed spikelet initiation in early-sown spring wheat in both years, but this effect was smaller in 2019–20. The difference in the delay effect of rolling between 2018–19 and 2019–20 may have arisen before spikelet initiation, because ground rolling after spikelet initiation did not affect apical development rate (). The small delay effect in 2019–20 can be explained by the higher temperatures during several weeks following rolling, which helped to recover the delay in apical development caused by rolling
Relationships of apical development stage with stem length and spike length
The relationships of apical development stage with stem length and spike length did not differ between years or treatments, so we analyzed the 2 years of data combined. The rate of stem length elongation increased at stage 7, irrespective of rolling or year (). The jointing stage (stem length y = 2.0 cm) was estimated as stage 8.5 (95% confidence interval [CI] 7.9–9.1) in single regression analysis with pooled data. Spike length was also closely related to apical development stage (), and the regression lines differed little between treatments (data not shown). The spike length at the start of internode elongation (apical development stage x = 7.0) was estimated to be 2.3 mm (95% CI 1.9–2.7 mm) in a single regression analysis with pooled data. That at the jointing stage (apical development stage x = 8.5) was estimated to be 3.5 mm (95% CI 2.9–4.2 mm). Takeda (Citation1976) reported that when spikes grow to ≥3 mm, they become susceptible to frost injury; therefore, the spikes after this stage were considered to be at high risk of frost injury.
Effect of rolling on agronomic characteristics
The two regression equations shown in indicate that in 2018–19, rolling delayed spikelet initiation by 5 days, the start of internode elongation by 12 days, and jointing by 9 days compared with the control (). It significantly reduced frost injury and significantly increased yield (). In addition, it slightly but significantly delayed heading and maturity but did not affect culm or spike length (). In 2019–20, rolling delayed spikelet initiation by 4 days, the start of internode elongation by 5 days, and jointing by 3 days. There was no significant difference in either frost injury or yield in 2019–20 ().
In 2018–19, the daily minimum temperatures during the 2 months immediately following the first rolling fluctuated around the 30-year average (Figure S1). Control plants jointed at the time of the lowest minimum temperature (−1.85°C on 15 Feb; Figure S1), but rolled plants jointed 9 days later, avoiding the coldest day. Thus, control plants suffered significant frost injury owing to early internode elongation, whereas young spikes of rolled plants avoided freezing temperatures owing to delayed internode elongation, explaining rolling’s significant effect on reducing frost injury and increasing yields (). However, there were then several days with minimum temperatures around 0°C before ear emergence. These low temperatures may have caused some frost injury to both control and rolling plants, as shown in .
On the other hand, in 2019–20, the daily minimum temperatures during the 2 months immediately following the first rolling were considerably higher than the average for the last 30 years (Figure S1). As a result, jointing of control plants grown in this winter season occurred about 2 weeks earlier than that of control plants grown in the last winter season, and the delay of jointing of rolled plants compared to that of control plants was only 3 days (). After the jointing, the rolled plants, as well as the control plants, were exposed to the lowest minimum temperatures. Therefore, the ground rolling in 2019–20 may not induce the avoidance of frost injury and yield increase.
Future prospects for studies of ground rolling
These results indicate that rolling before spikelet initiation is useful for avoiding frost injury in early-sown spring wheat. However, its effect is related to the temperature between rolling and jointing, and if the temperature is high enough, jointing is accelerated, exposing plants to potential frost injury. To induce a stable effect of rolling, it will be important to investigate the most effective timing, numbers, and intensity of rolling treatments. In addition, as ethylene treatment delays the transition from vegetative to reproductive phase in spring wheat (Mizumoto, unpublished data), ethylene may be involved in the effect of rolling, so it will be necessary to clarify the relationship between rolling and ethylene production.
PPS2022_047SR-File007.pdf
Download PDF (294.4 KB)Acknowledgments
We thank Hiroshi Saito, Katsuyuki Yamauchi, Yukio Araki, Naoto Minamikurosawa (National Institute of Vegetable and Floriculture Science, NARO), and Miki Arakaki (Central Region Agricultural Research Center: CARC, NARO) for their technical assistance. We express our sincere thanks to Satoshi Yoshinaga (CARC) for his critical reading of this manuscript. We are grateful to the Iwaki Farmer Group for the use of the field, to the Tokai Regional Agricultural Administration Office for planted area data, and to the Japan Meteorological Agency for temperature data.
Disclosure statement
No potential conflict of interest was reported by the author(s).
Supplementary material
Supplemental data for this article can be accessed online at https://doi.org/10.1080/1343943X.2022.2136097
References
- Inamura, H., Suzuki, K., & Nonaka, S. (1955). On the standard of successive stages of barley and wheat spike development. Bulletin Kanto–Tosan Agricultural Experiment Station, 8( in Japanese with English summary), 75–91. https://iss.ndl.go.jp/books/R000000004-I9850157-00
- Inamura, H., Yamaga, I., Suzuki, K., & Gokan, M. (1958). Study on the breeding of early varieties of wheat and barley. (I.) Varietal differences of the early wheat and barley in the internode-elongation in relation to the death of young ear by frost damage in early spring. Bulletin Kanto–Tosan Agricultural Experiment Station, 11(in Japanese with English summary), 20–28. https://iss.ndl.go.jp/books/R000000004-I9850106-00
- Kawabata, K. (1989). Simple regression analysis. In editorial committee (chairman Tadakazu Okuno). In Applied Statistics Handbook(6th) ed., pp. 91–120 (in Japanese).
- NARC. (1986). Evaluation standards for wheat characteristics(1st).174. National Agricultural Research Center (in Japanese). http://www.naro.affrc.go.jp/publicity_report/publication/archive/files/komugi_chousakizyun.pdf
- Ohtani, Y. (1942). Frozen damage in spring in wheat and barley [1]. Agriculture and Horticulture, 17(3), 285–292. in Japanese
- Ohtani, Y. (1950). Studies on the stamping of wheat and barley. Bulletin National Agricultural Experiment Station, 67(in Japanese), 1–76. https://iss.ndl.go.jp/books/R000000004-I10044032-00
- Takeda, M. (1976). Initiation and development of the wheat spike (Chairman Shigeyoshi Kajiwara). In japanese, Compendium of agricultural technology, crops 4 (1st) ed., pp. 73–74). Ruaral Culture Association Japan.
- Tanio, M., Tateishi, K., Nakazono, K., & Watanabe, K. (2016). Pseudostem length as an indicator of the start of internode elongation in spring and winter wheat cultivars. Japanese Journal of Farm Work Research, 51(1), 1–9. https://doi.org/10.4035/jsfwr.51.1
- Tanio, M., Watanabe, K., Nakazono, K., Uchino, A., & Mizumoto, A. (2019). High-yielding intensive production system in early-sown winter wheat. Japanese Journal of Farm Work Research, 54(4), 209–223. https://doi.org/10.4035/jsfwr.54.209
- Uchijima, T. (1978a). Frost injury in wheat and barley [1] – Its damage and causal factors. Agriculture and Horticulture, 53(4), 545–548. in Japanese. https://iss.ndl.go.jp/books/R000000004-I1944744-00
- Uchijima, T. (1978b). Frost injury in wheat and barley [2] – Its damage and causal factors. Agriculture and Horticulture, 53(5), 653–658. in Japanese. https://cir.nii.ac.jp/crid/1521136280829730048
- Watanabe, T., Fukami, K., Tanio, M., Masuda, K., Matsuo, K., & Nakanishi, Y. (2009). Broad-bed planting system with shallow tillage to prevent wet injury in crop rotation system on paddy field in Japan. Proceedings 18th ISTRO Conference T1–016, 1–5. https://www.istro.org/index.php/publications/proceedings/24-istro-2009-proceedings/file
- Zadoks, J. C., Chang, T. T., & Konzak, C. F. (1974). A decimal code for the growth stages of cereals. Weed Research, 14(6), 415–421. https://doi.org/10.1111/j.1365-3180.1974.tb01084.x