ABSTRACT
Intercropping is an agricultural technique where many crops are grown together on the same field, and it is becoming more widely acknowledged for its ability to improve productivity in food and forage production. This farming method allows for the strategic integration of a profitable crop with cover or non-profitable crops to achieve reciprocal benefits, while also spreading advantages across different agricultural systems. Intercropping designs differ to attain greater crop yields, reduce the use of chemicals and fertilizers, and enhance water-use efficiency, especially in the demanding circumstances of arid and semi-arid countries. This study examines new mechanisms via which intercropping enhances sustainable agriculture, including recent progress in ecological, genetic, and microbial interactions that enhance plant growth and resilience. The adaptability of the Mediterranean region, for varied agricultural methods is also assessed, with a particular focus on the possibilities for implementing intercropping systems. This study provides a thorough analysis of existing research, emphasizing the many advantages of intercropping and its contribution to the progress of sustainable agriculture.
Introduction
From more than 3 billion in 1960 to more than 8 billion in recent years, the global population has been steadily increasing (UNFPA, Citation2023). The loss of natural ecosystems like forests is a common consequence of this rise, which has happened in tandem with the expansion of agricultural regions. World Bank (Citation2023) reports that while overall agricultural land has increased, the quantity of arable land per person has declined by almost 50%, reaching around 0.18 hectares. Remarkably, undernourishment has decreased throughout this period, proving the success of the ‘Green Revolution.’ This revolution saw a substantial increase in agricultural productivity due to the use of current crop breeding methods and other innovations (Gollin et al., Citation2021).
Nevertheless, the expansion of agriculture has brought about noteworthy environmental and ecological issues. These include heightened soil erosion, excessive nutrient input resulting in eutrophication and groundwater pollution, and a substantial decline in biodiversity due to the extensive application of pesticides, herbicides, and the prevalence of monocultures (Crews et al., Citation2018).
Given these difficulties, there is an increasing fascination with sustainable agriculture methods that not only strive to guarantee food security for present and future generations, but also conserve natural resources, improve farmers’ profitability, and maintain rural communities (Koohafkan & Altieri, Citation2016). Intercropping is a sustainable strategy that includes cultivating many crop species together in the same area to take advantage of the beneficial interactions between them (Maitra et al., Citation2021). The approach has been recognized as a financially and environmentally sustainable alternative to traditional monoculture farming. It has advantages such as improved soil quality, better management of pests and diseases, higher crop production and variety, and more efficient water use (Horwith, Citation1985).
Although the advantages of intercropping have been extensively studied, there is a growing corpus of research investigating innovative methods and technological breakthroughs that provide a deeper understanding of the intricate interactions occurring in intercropped systems (Mousavi & Eskandari, Citation2011). This encompasses comprehending the influence of ecological dynamics, genetic variables, and microbial relationships on augmenting plant growth, stress resilience, and total system production (Benmrid et al., Citation2023). Hence, this study not only provides a concise summary of the well-established advantages of intercropping but also explores these innovative perspectives, presenting a full assessment of intercropping’s significance in contemporary sustainable agriculture. We have analyzed research conducted in different geographic areas and use diverse combinations of crops to emphasize the specific factors affected by intercropping, including soil fertility, pest and disease control, crop productivity and quality, and water management. Our main emphasis was on the suitability of intercropping in the Mediterranean region. By conducting this investigation, our objective was to provide important knowledge that may direct the implementation and enhancement of intercropping techniques on a global scale, therefore contributing to the progress of sustainable agricultural systems.
Presentation of different intercropping approaches and comparative analysis of intercropping patterns
Intercropping, the practice of growing two or more crop species or genotypes in the same field, is a multi-faceted farming approach that leverages the distinct qualities and interactions of various crops to offer an eco-friendly alternative to traditional farming methods (Brooker et al., Citation2023). This method is especially prevalent in agricultural systems that are resource-limited or used for subsistence (Brooker et al., Citation2015). Ecologically, intercropping presents numerous advantages such as improved use of water and nutrients and increased yield per acre (Lanker et al., Citation2020). Additionally, it naturally reduces weed growth and makes crops less susceptible to pests and diseases. For example, the slow juvenile development of certain crops, which makes them vulnerable to weed invasion, is effectively countered by the robust competitiveness of their intercropped counterparts (Mortensen et al., Citation2000). Leguminous plants in intercropping systems further enhance soil fertility through biological nitrogen fixation, highlighting the comprehensive benefits of this approach (Dai et al., Citation2019).
Intercropping systems encompass a wide array of crops and configurations to maximize agricultural efficiency and sustainability. Below is a comparative analysis of five principal intercropping patterns, each designed to exploit specific ecological interactions and benefits:
Row-Intercropping: Characterized by the simultaneous growth of crops, with at least one arranged in distinct rows, optimizing spatial arrangement and interaction (Bugilla et al., Citation2023). This pattern facilitates precise management of individual crop species, allowing for targeted interventions such as fertilization and pest control (Riemens et al., Citation2022). Its structured layout enhances accessibility and mechanization, making it suitable for farms looking to combine efficiency with ecological benefits (Maitra et al., Citation2021).
Mixed Intercropping: Crops are grown together without a specific row pattern, ideal for the combined cultivation of grasses and legumes in pastures (Gardarin et al., Citation2022). This pattern fosters rich biodiversity, enhancing below-ground interactions that promote soil health and fertility (X. Zhao et al., Citation2023). It is particularly effective in small-scale or subsistence farming systems where mechanical harvesting is less prevalent (Zakir et al., Citation2023).
Strip-Intercropping: Involves the concurrent cultivation of crops in separate strips that are wide enough for independent cultivation but narrow enough to ensure ecological interaction (Juventia et al., Citation2022). This approach optimizes edge effects, improving pollination and pest control through increased biodiversity. It is well-suited for larger fields where distinct crop sections can interact ecologically while remaining manageable (Croijmans et al., Citation2024).
Relay-Intercropping: Entails the simultaneous cultivation of crops during part of their life cycles, facilitating extended ground cover and reduced tillage (Raza et al., Citation2020). This method extends the productive use of land throughout the season, offering continuous ground cover that can reduce soil erosion and improve water retention. It’s ideal in regions with extended growing seasons, allowing for the sequential harvesting of crops (Yu et al., Citation2024).
Alternate Intercropping: Also known as transposition intercropping, this strategy involves intercropping two crops in alternating positions annually (Lv et al., Citation2023). This innovative approach merges the principles of intercropping and rotation, offering enhanced benefits beyond those achievable by either method in isolation. It leverages diversified plant interactions to improve soil health and disrupt pest and disease cycles (Chi et al., Citation2023).
Each intercropping pattern thrives under specific environmental conditions and addresses particular agricultural challenges (Y. Zhao et al., Citation2024). For instance, row intercropping may be preferred in environments where crop specificity in care and harvesting is paramount (C. Li et al., Citation2023). In contrast, mixed intercropping is favored in ecosystems where soil fertility and biodiversity are critical considerations (Glaze-Corcoran et al., Citation2020). Strip-intercropping is advantageous in landscapes that can support distinct yet interacting crop zones (Zou et al., Citation2021), while relay-intercropping and alternate intercropping are effective in regions aiming for continuous production and rotational benefits (Lamichhane et al., Citation2023), respectively.
Intercropping aligns with low-input agricultural practices, drawing attention to its potential to significantly bolster crop productivity and economic returns compared to traditional monoculture methods (Anim-Jnr et al., Citation2023). The land equivalent ratio (LER) serves as a pivotal metric for comparing the productivity of intercropped land with that of conventional agricultural systems. An LER greater than 1 signifies that intercropping yields higher productivity on the same land area compared to monoculture practices, underscoring the efficiency and sustainability of this farming approach (Morales-Rosales & Franco-Mora, Citation2009).
As we delve into the myriad effects of intercropping on soil health, pest and disease management, yield optimization, forage quality, and water management, it is crucial to understand the underlying mechanisms that drive the success of intercropping systems. The following sections will thoroughly examine these effects, providing detailed insights into the various benefits and the scientific principles that support intercropping as a fundamental aspect of sustainable agriculture ().
Table 1. The effects of intercropping in different countries.
Underlying mechanisms that contribute to the success of these patterns include improved nutrient cycling, efficient water use, enhanced pest and disease suppression through natural enemy biodiversity, and improved pollination dynamics (Ghaffar et al., Citation2022). For example, legume-based systems can fix atmospheric nitrogen, reducing the need for synthetic fertilizers (Jensen et al., Citation2020), while diverse root systems in mixed systems can explore different soil layers for nutrients and moisture, enhancing overall resource use efficiency (Homulle et al., Citation2021).
Recent studies and case studies underscore the practical applications and benefits of these intercropping patterns. For instance, research has shown that strip-intercropping of maize and legumes can significantly increase overall biomass and grain yield due to optimized light capture and reduced pest pressure (Pierre et al., Citation2022). Similarly, alternate intercropping systems have demonstrated improved soil structure and increased yield stability over time. A summary figure () highlights the advantages, appropriate conditions, and underlying mechanisms for each intercropping pattern, providing a quick reference for researchers and practitioners.
Figure 1. Comparative overview of intercropping patterns: Advantages, optimal conditions, and mechanisms
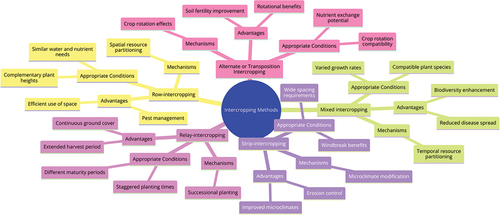
Based on the above facts, selecting the appropriate intercropping pattern based on agricultural goals, environmental conditions, and resource availability is crucial for maximizing the benefits of this sustainable farming approach (H. Yang et al., Citation2021). The potential for innovative combinations and novel applications of these patterns offers exciting prospects for addressing contemporary agricultural challenges, including those related to climate change, food security, and ecosystem sustainability ().
Novel mechanisms and recent advancements in intercropping systems
Numerous new processes and developments have been uncovered via research into intercropping systems, which greatly aid in sustainable agriculture (Blessing et al., Citation2022). There are many less-known advantages to intercropping than the more obvious ones, like climate resilience and pollinator attraction (Huss et al., Citation2022), in addition to the more well-known ones, like increased yield and pest control (Stomph et al., Citation2020). Intercropping also encourages a web of ecological interactions, genetic contributions to crop resilience, and dynamic microbial activities impacting soil health (T. Yang et al., Citation2020). Realizing the full potential of intercropping systems requires a knowledge of these complex relationships and advantages.
Efficient utilization of resources and a harmonious agricultural environment are achieved via ecological interactions within intercropping systems (Jhariya et al., Citation2021). The byproducts of one crop may be used as a resource by another crop as a consequence of these interactions. Leguminous plants, for instance, improve soil quality for plants that do not fix atmospheric nitrogen (Yuvaraj et al., Citation2020). How to maximize production while minimizing environmental effects may be better understood thanks to recent research that has illuminated these ecological synergies. Improving light interception, decreasing evaporation, and encouraging more efficient use of space and resources may be achieved by optimizing planting densities and arrangements (Haque & Sakimin, Citation2022).
The genetic diversity within intercropping systems is becoming more and more associated with the resistance of crops in these systems to stresses including pests, diseases, and changeable climatic conditions (Stomph et al., Citation2020). Improved resistance mechanisms may result from interactions between diverse crop genotypes, and certain crops can even defend their neighbors (Bass et al., Citation2024). The possibility for focused breeding efforts to further improve intercropping efficiency has been raised by recent genomic studies that have started to discover certain genetic markers linked with these advantageous features (Bourke et al., Citation2021).
Soil fertility and plant nutrition are both affected by the microbial dynamics of intercropping systems (Maitra & Ray, Citation2019). Soil microbiomes may be dramatically changed by the variety of root exudates in mixed cropping systems, leading to the promotion of beneficial microbial communities that aid in nutrient cycling, soil structure, and disease suppression (Q. Li et al., Citation2018). The intricate web of relationships between intercropping plants and soil microorganisms is now being mapped out using cutting-edge molecular tools like metabolomics and metagenomics (David, Citation2023). This is illuminating the processes that underlie the reported improvements in soil fertility and plant development.
Additionally, intercropping is beginning to get attention for its less-studied advantages, such as its ability to withstand climate change and attract pollinators (Garrett et al., Citation2009). Because they increase biodiversity and make better use of resources, intercropping systems are better able to endure the challenges brought on by climate change, such as sudden shifts in temperature and precipitation patterns (Ghaffar et al., Citation2022). Improved pollination services and biodiversity protection may result from intercropping systems’ use of a larger range of crops, which in turn attracts more pollinators. In addition to helping the crops, this also helps the larger ecosystem services that are important for the long-term viability of agriculture (Nicholls & Altieri, Citation2013).
Research on intercropping has recently advanced, illuminating new processes and complex interconnections that greatly improve agricultural systems’ sustainability and production. Research into plant-to-plant communication in intercropping systems has shown that plants are capable of intricate biochemical conversations, which is a major new development in this field (Pickett & Khan, Citation2016). Because plants nearby may communicate with one another to alert one another to impending insect invasions or disease outbreaks, this kind of contact might increase resistance to these threats (Pélissier et al., Citation2021). As an example, research has shown that when pests attack certain crop combinations, the plants emit volatile organic compounds (VOCs). These chemicals may activate defensive systems in nearby plants, creating a cooperative defense approach that ultimately minimizes insect damage (Huang et al., Citation2020).
Furthermore, advances in comprehending the function of the rhizosphere in intercropping systems have brought attention to the significance of root exudates in molding the communities of soil microbes (Lai et al., Citation2022; Wasaki & Dissanayaka, Citation2021). Beneficial bacteria that aid in nutrient cycling, promote soil structure, and inhibit soil-borne diseases may be selectively enhanced by these exudates (Qu et al., Citation2020). For instance, in an intercropping system, all crops benefit from the increased nitrogen availability caused by the particular flavonoids released by legume roots, which promote nitrogen-fixing bacteria (Dong et al., Citation2022). Because synthetic fertilizers are not utilized in organic farming, this nutrient augmentation via microbes is very important (Ye et al., Citation2020).
New research suggests that cultivating various crop species in close proximity may change gene expression linked to stress tolerance and nutrient absorption (Mickelbart et al., Citation2015). This finding adds to the innovative understanding of the effect of intercropping on plant genetic expression. Plants in intercropping systems have the ability to upregulate certain genes that improve resilience and resource-use efficiency, which allows them to respond better to environmental challenges like drought or nutrient deficits (Mazzafera et al., Citation2021).
Significant progress has been made via the use of intercropping in reducing the impact of climate change and increasing water-use efficiency (Hatfield & Dold, Citation2019). Soil moisture is retained for longer periods of time and evaporation rates are reduced by the creation of microclimates inside the crop canopy through intercropping (Nyawade et al., Citation2019). To further improve drought resistance and minimize water loss, crops with varying root depths may be strategically planted to maximize water utilization across the soil profile (Wu et al., Citation2016). When water is scarce, agricultural output suffers, and this is especially true in semi-arid and dry areas.
Lastly, a new dimension has been added to intercropping research via the incorporation of technology tools and models (Bourke et al., Citation2021). Researchers are now able to examine intercrop dynamics with a level of precision never before seen by using cutting-edge imaging methods including 3D root architecture modeling and drone-based multispectral photography (Canicattì & Vallone, Citation2024). To maximize output while minimizing environmental impact, these technologies enable the exact assessment of plant growth, health, and resource usage in intercropping systems (Jamil et al., Citation2022).
To sum up, the inventive processes and new developments in intercropping systems highlight the practice’s versatility (). Intercropping is a powerful tool for sustainable agriculture because it promotes ecological interactions, uses genetic variety to its advantage, improves microbial dynamics, and helps with both climate resilience and pollinator populations (H. Yang et al., Citation2021). The more we learn about these interconnected processes, the more possibilities there are for creative uses of intercropping in various agroecological zones, which bodes well for a more sustainable and fruitful agricultural future.
Enriching earth: the transformative impact of intercropping on soil fertility and health
When it comes to sustainable agriculture, intercropping is a game-changer when it comes to improving soil fertility and health (Kumar et al., Citation2023). By growing many crops close together, this technique allows the soil ecosystem to reap the advantages of a wide variety of plant species working in harmony (Shrestha et al., Citation2021). The incredible ability of intercropping systems to increase soil nutrient levels, especially nitrogen (N) and phosphorus (P), and to cultivate varied and strong microbial communities has been the subject of several research (Janati et al., Citation2023).
For cereal-legume combinations in particular, intercropping’s capacity to promote symbiotic nitrogen fixation is crucial to the crop’s growth. This interaction improves the efficiency of nitrogen fixation by increasing the soil’s bioavailable nitrogen content and decreasing the detrimental effects of nitrate inhibition on nodulation genes. These kinds of biological activities have a crucial role in ensuring that soil remains fertile and in encouraging long-term agricultural output (Latati et al., Citation2017; Liu et al., Citation2011).
Furthermore, research has shown that intercropping has a substantial impact on soil microbial dynamics (Wang et al., Citation2021). The intricate patterns of root exudation and the transfer of carbon and nitrogen from plants to the rhizosphere lead to a rise in the number and variety of microbes. Rahman et al. (Citation2021) and Ma et al. (Citation2022) found that intercropped systems improve nutrient cycling and soil health due to this complex network of interactions
The significance of intercropping in increasing the bioavailability of phosphorus in soil is remarkable. Intercropping certain crop combinations may significantly enhance accessible P, according to different researches (Cong et al., Citation2015; X. Zhao et al., Citation2022), which helps with one of the biggest problems with agricultural nutrient management.
It is possible to increase soil organic carbon (C) storage by intercropping, which is another major benefit. To maintain a healthy soil structure, fertility, and carbon cycle equilibrium, it is essential to have a variety of root systems (Cong et al., Citation2015). This diversity helps to increase organic matter accumulation and C sequestration.
A new way to deal with micronutrient deficits, such as iron (Fe), is by intercropping. Improved bioavailability of iron and other micronutrients might result from changes in rhizosphere conditions brought about by interactions between various plant species. Especially in areas with scarce Fe-rich calcareous or alkaline soils, this has major consequences for crop nutrition and yield (Cañasveras et al., Citation2014; Dai et al., Citation2019).
All things considered, these results highlight the many ways in which intercropping improves soil health. Intercropping is an important technique for developing sustainable agricultural systems because it increases soil organic matter, boosts microbial diversity, and increases nutrient availability. It highlights the significance of incorporating different cropping patterns into current agricultural operations and adds to ecological balance while also paving the path for more productive and resilient cropping systems.
Enhancing crop resilience: the role of intercropping in managing pests, diseases, and weeds
One way to improve soil health and control pests, diseases, and weeds is by intercropping, which involves growing two or more crops close together. To maximize resource use and enhance crop resilience to biotic stresses, this approach takes advantage of the ecological and biological interactions across plant species (Homulle et al., Citation2021). A wide range of intercropping systems, including row intercropping, mixed intercropping, strip intercropping, relay intercropping, and the new alternate intercropping, provide different ecological advantages depending on the goals and circumstances of the farm.
Improved soil fertility and microbial variety are two outcomes of the ecological harmony made possible by intercropping. Researchers have shown that cereal-legume intercropping systems may improve soil nutrient content by symbiotic nitrogen fixation (Latati et al., Citation2017; Liu et al., Citation2011). Research by Rahman et al. (Citation2021) and Ma et al. (Citation2022) also shows that intercropping increases soil carbon stock and improves phosphate bioavailability via root exudates. These enhancements to soil health not only promote plant development but also help build a stronger ecosystem that can control pests and diseases that are carried by the soil.
The benefits of intercropping extend far beyond improved soil quality to include the mitigation of insect and disease problems. As an illustration of the ability of some plant combinations to naturally discourage pests, research conducted by X. W. Li et al. (Citation2021) found that intercropping sweet pepper with rosemary greatly decreased populations of important insect species. This impact is probably caused by the emission of volatile chemicals that deter predators or by improving habitat for predators in the wild. Zhou et al. (Citation2023) also discovered that various combinations of chickpea and flax intercropping decreased the occurrence of Ascochyta blight in chickpeas, demonstrating that intercropping may suppress diseases by creating better-growing conditions.
Another important benefit of intercropping is that it may inhibit weeds, providing a more environmentally friendly option to conventional pesticides. The development and multiplication of weeds may be reduced by strategic crop combinations, as shown in research by Kaur et al. (Citation2016) and Bibi et al. (Citation2019). Because intercropping increases ground cover and changes microclimate conditions, which are unfavorable for weed development, it suppresses weeds. Furthermore, intercropping has a multiplicative effect on soil health, disease, and insect resistance, and weed reduction. A stronger plant defense mechanism against pests and diseases is achieved by the development of soil microbial diversity and nutrient cycling (Kumawat et al., Citation2022). Additionally, overall crop health and production are supported by the competitive exclusion of weeds.
Based on the above facts, farmer resilience, increased crop yields, and less dependence on chemical inputs may all be yours when you master the ecological relationships between intercropped species. To fully harness the benefits of this environmentally friendly farming method, further studies and practical implementation of intercropping techniques are required. This is especially true because we are facing global issues like climate change and food insecurity.
Maximizing harvests: the impact of intercropping on crop yields
The advantage of intercropping is increasingly recognized for its potential to enhance yields beyond what is achievable through traditional monoculture practices (Glaze-Corcoran et al., Citation2020). Among the benefits, nitrogen-fixing legumes play a pivotal role by enriching the soil with bioavailable nitrogen, thus benefiting their non-leguminous companions. Research, such as the work by Davis and Woolley (Citation1993), demonstrated the effectiveness of intercropping bean genotypes with maize, highlighting improved yields due to enhanced nitrogen availability. Similarly, Roder et al. (Citation1992) observed a 12–15% yield increase in potatoes when intercropped with maize, emphasizing the mutual benefits derived from such combinations.
The Land Equivalent Ratio (LER) concept is instrumental in illustrating intercropping’s efficiency. An LER greater than 1 indicates a yield advantage over monoculture, showcasing the spatial and temporal complementarity effects that intercropping systems can offer. Willey (Citation1990) reported a notable LER of 1.67 in sorghum/pigeon pea and millet/groundnut intercropping, signifying a 67% increase in yield over monoculture systems on the same land area.
Intercropping’s applicability extends to diverse crop combinations, including non-traditional pairings such as walnut and wheat, where Qiao et al. (Citation2021) noted an enhancement in grain quality despite a yield reduction, suggesting a strategic compromise between crop quality and quantity in agroforestry systems. Further, Kahraryan et al. (Citation2021) emphasized the optimal intercropping ratios, with a specific barley and vetch mix significantly improving grain yield and forage quality.
Emerging research avenues include the integration of soil amendments to augment intercropping yields. Studies by Hu et al. (Citation2018) and L. Wang et al. (Citation2023) investigated the impacts of nitrogen fertilization and biochar application on pea-maize and maize-soybean systems, respectively, highlighting the potential of such interventions to enhance yield, water use efficiency, and nutrient recovery efficiency.
Spatial configuration within intercropping systems, such as the narrow-wide-row relay-intercropping studied by Feng et al. (Citation2019), demonstrated improvements in light interception and photosynthetic rates, thus contributing to yield enhancements. This underscores the critical role of spatial arrangement in maximizing intercropping benefits.
The extensive body of research supports intercropping as a viable strategy to significantly improve agricultural yields through mechanisms such as enhanced nutrient use efficiency, optimized spatial arrangements, and the judicious application of soil amendments. These findings advocate for the broader adoption of intercropping practices as a sustainable solution to the challenges of increasing agricultural productivity while preserving ecosystem health.
Enhancing nutritional value: the influence of intercropping on forage quality
Both food crops and forage production have reaped enormous advantages from intercropping, which has greatly increased fodder productivity and improved its quality. The goal of this farming method is to increase forage yields relative to traditional single-crop farming by planting many crops close to one another. Research by Zhang et al. (Citation2015) has shown that intercropping systems of maize and rye with alfalfa significantly boost dry matter production and crude protein yield. Synergistic interactions between plant species increase resource consumption and boost total output, which is why this effect is seen.
Legumes are an essential part of these systems when planted alongside grains or grasses; they increase soil nitrogen availability, which improves the quality of fodder. When the clover content was increased in intercropping systems, Pourali et al. (Citation2023) discovered that the crude protein content, dry matter digestibility, and overall fodder quality were all improved. Furthermore, Eskandari et al. (Citation2009) emphasized the advantages of cereal-legume intercropping in creating high-quality fodder, highlighting the fact that legumes have a better nutritional profile.
Soil salinity and nutrient depletion are two examples of the unique agricultural problems that may be solved by intercropping techniques. To improve the overall sustainability of agricultural cycles, Monti et al. (Citation2019) demonstrated that grain legume intercropping with cereals produced sustainable fodder solutions in addition to cash crops such as durum wheat. Ullah et al. (Citation2018) also showed that fodder quality and biomass output may be greatly improved by strategically combining forage crops with the right fertilizer.
It has been shown that intercropping sorghum and forage grasses with maize greatly increases biomass in tropical and integrated crop-livestock systems (Sarto et al., Citation2021). In addition to bolstering ecological sustainability via increased biodiversity and soil health, this method helps make agricultural systems more economically viable by making better use of available land.
Due to the region’s distinct climate, intercropping has become popular throughout the Mediterranean. Saia et al. (Citation2016) emphasized the effective use of natural resources by legume-ryegrass intercrops and argued that intercropping may be a sustainable agricultural approach in Mediterranean climates. Moreover, alfalfa and wheat intercropping systems may help reduce soil salinity, which is good for soil health and structure (Su et al., Citation2024).
To sum up, intercropping is an eco-friendly substitute for conventional farming that shows promise in increasing both fodder quality and output. Farmers may improve soil health, increase the resilience of their agricultural systems, and increase the nutritional qualities of forage by using the complimentary interactions between diverse crop species. Improvements in fodder production, which benefit cattle nutrition and agricultural sustainability, may be achieved by the use of intercropping in conjunction with suitable management measures, such as nitrogen fertilizer.
Unlocking agroecological potential: intercropping as a sustainable pathway in the Mediterranean region
According to Lionello et al. (Citation2006), the Mediterranean climate is defined by mild winters with moderate precipitation and hot, dry summers. Native Mediterranean plants have adapted to the region’s scorching, dry summers and long, rainy winters. Mediterranean climate is characterized by evergreen trees such as laurel, pine, olive, and oak, as well as cool season plants such as chickpea, parsley, and pea, and warm season plants such as sesame, sunflower, and fruit trees like walnut and grape. Some issues have been seen across the Mediterranean region, including an increase in the surface area of the Mediterranean Sea, more frequent natural catastrophes, a rise in population, and higher average temperatures (Cramer et al., Citation2018). Temperatures in the Mediterranean area are increasing at a rate 25% faster than the global average and at a rate 40% quicker during the summer. Reduced availability, altered supply, and diminished quality of food are all possible outcomes of climate change. Several causes, such as increasing temperatures, changing precipitation patterns, changing the frequency of severe weather, and reducing water availability, might lead to lower agricultural productivity (Alrteimei et al., Citation2022). To meet future food demand while sustainably managing current land and water resources, irrigation farming in semi-arid areas like the Mediterranean must adapt to changing climate and socioeconomic conditions (Harmanny & Malek, Citation2019). For example, in the Mediterranean region, rainfed viticulture is subject to unpredictable rainfall patterns. However, by implementing an effective irrigation system and practicing intercropping, it was shown that the most adaptable approach achieves the best long-term balance between agricultural output and environmental benefits, even during severe droughts (Ripoche et al., Citation2011). Many agricultural advancements concerning intercropping apply to the Mediterranean area because of the mild environment. For example, instead of fescue, you may intercrop walnut trees (Juglans nigra L. and Juglans nigra x regia NG23) with fodder legumes such as sainfoin (Onobrychis sativa L.) or alfalfa (Medicago sativa L.). Water competition, fixed nitrogen transfer from leguminous crops to trees, tree growth, soil water depletion, and nitrogen content of tree leaves were all factors that Dupraz et al. (Citation1999) examined in these systems. According to Dupraz et al. (Citation1999), the nitrogen content of walnut leaves was found to be higher when nitrogen-fixing plants were intercropped. Plants employ chlorophyll, which absorbs light and converts it into sugars, which contain nitrogen. Plant growth, grain production, P availability, and P absorption were compared in a research by Latati et al. (Citation2014) in the Mediterranean climate area of Algeria. The plants studied were sole-cropped cowpea (Vigna unguiculata L. cv. Moh Ouali) and maize (Zea mays L. cv. ILT), intercropped cowpea-maize, and fallow. In an alkaline soil, cowpeas intercropped with maize to increase P availability in the rhizosphere, which in turn boosted P absorption. Plant biomass and grain output were both increased when cowpea was intercropped with maize, according to this research. Yin et al. (Citation2020) recommend that researchers look at the interplay between water migration across zones, water competition and complementarity among different intercrops, and future studies. . To sum up, growing legumes as part of an intercropping system has the potential to enhance nitrogen balance, which in turn reduces the need for inorganic nitrogen fertilizer. Efficient use of water is another benefit of this gardening approach. This means that the Mediterranean climate, and maybe other climate zones as well, is suitable for growing the many crops that are prepared for intercropping.
Conclusions
Alternative agricultural crop production techniques that outperform traditional methods in terms of sustainability and productivity are now attracting a lot of attention. Intercropping is one approach that makes strategic use of the interaction effects between several plants. This research revealed these consequences, which include affecting soil nutrients and microbes, especially by taking use of legumes’ nitrogen-fixing abilities. Simultaneously, evidence suggests that intercropping might lessen the prevalence of some pests and plant diseases, which in turn decreases the need for potentially dangerous pesticides. Additionally, it was said that intercropping may, under some conditions, help minimize crop water usage, enabling crops to be cultivated with little or no irrigation. This might be particularly useful in the Mediterranean, where the often-hot summers need irrigation. Taken together, the results demonstrate that various intercropping strategies have great potential for efficient and fruitful farming. This review study included the essential information on the impacts of intercropping that is needed for future applications.
Acknowledgments
We appreciate the Scientific Research Projects Coordination Unit of Akdeniz University for continuous support.
Disclosure statement
No potential conflict of interest was reported by the author(s).
Additional information
Funding
References
- Alrteimei, H. A., Ash’aari, Z. H., & Muharram, F. M. (2022). Last decade assessment of the impacts of regional climate change on crop yield variations in the Mediterranean region. Agriculture, 12(11), 1787. https://doi.org/10.3390/agriculture12111787
- Anim-Jnr, A. S., Sasu, P., Bosch, C., Mabiki, F. P., Frimpong, Y. O., Emmambux, M. N., & Greathead, H. M. R. (2023). Sustainable small ruminant production in low-and middle-income African countries: Harnessing the potential of agroecology. Sustainability, 15(21), 15326. https://doi.org/10.3390/su152115326
- Bass, E., Mutyambai, D. M., Midega, C. A., Khan, Z. R., & Kessler, A. (2024). Associational effects of desmodium intercropping on maize resistance and secondary metabolism. Journal of Chemical Ecology, 1–20. https://doi.org/10.1007/s10886-024-01470-5
- Benmrid, B., Ghoulam, C., Zeroual, Y., Kouisni, L., & Bargaz, A. (2023). Bioinoculants as a means of increasing crop tolerance to drought and phosphorus deficiency in legume-cereal intercropping systems. Communications Biology, 6(1), 1016. https://doi.org/10.1038/s42003-023-05399-5
- Bibi, S., Khan, I. A., Hussain, Z., Zaheer, S., & Shah, S. M. A. (2019). Effect of herbicides and intercropping on weeds and yields of maize and the associated intercrops. Pakistan Journal of Botany, 51(3), 1113–1120. https://doi.org/10.30848/PJB2019-3(45)
- Bildirici, N., Aldemir, R., Karsli, M. A., & Dogan, Y. (2009). Potential benefits of intercropping corn with runner bean for small-sized farming system. Asian-Australasian Journal of Animal Sciences, 22(6), 836–842. https://doi.org/10.5713/ajas.2009.80676
- Blessing, D. J., Gu, Y., Cao, M., Cui, Y., Wang, X., & Asante-Badu, B. (2022). Overview of the advantages and limitations of maize-soybean intercropping in sustainable agriculture and future prospects: A review. Chilean Journal of Agricultural Research, 82(1), 177–188. https://doi.org/10.4067/S0718-58392022000100177
- Bourke, P. M., Evers, J. B., Bijma, P., van Apeldoorn, D. F., Smulders, M. J., Kuyper, T. W., Mommer, L.Bonnema, G. (2021). Breeding beyond monoculture: Putting the “intercrop” into crops. Frontiers in Plant Science, 12, 734167. https://doi.org/10.3389/fpls.2021.734167
- Brooker, R. W., Bennett, A. E., Cong, W. F., Daniell, T. J., George, T. S., Hallett, P. D., & White, P. J. (2015). Improving intercropping: A synthesis of research in agronomy, plant physiology and ecology. New Phytologist, 206(1), 107–117. https://doi.org/10.1111/nph.13132
- Brooker, R. W., Hawes, C., Iannetta, P. P., Karley, A. J., & Renard, D., Schmid, B. (2023). Plant diversity and ecological intensification in crop production systems. Journal of Plant Ecology, 16(6). https://doi.org/10.1093/jpe/rtad015
- Bugilla, F. B., Santo, K. G., Khalid, A. A., Afreh, D. N., Atakora, K., & Abdulai, M. (2023). Effects of spatial row arrangement and time of planting intercrops on performance of groundnut (Arachis hypogaea L.) under maize (Zea mays L.)—groundnut intercropping system in ejura. American Journal of Plant Sciences, 14(3), 264–289. https://doi.org/10.4236/ajps.2023.143019
- Cañasveras, J. C., Del Campillo, M. C., Barrón, V., & Torrent, J. (2014). Intercropping with grasses helps to reduce iron chlorosis in olive. Journal of Soil Science and Plant Nutrition, 14(ahead). https://doi.org/10.4067/S0718-95162014005000044
- Canicattì, M., & Vallone, M. (2024). Drones in vegetable crops: A systematic literature review. Smart Agricultural Technology, 7, 100396. https://doi.org/10.1016/j.atech.2024.100396
- Chadfield, V. G., Hartley, S. E., & Redeker, K. R. (2022). Associational resistance through intercropping reduces yield losses to soil‐borne pests and diseases. The New Phytologist, 235(6), 2393–2405. https://doi.org/10.1111/nph.18302
- Chi, B., Liu, J., Dai, J., Li, Z., Zhang, D., Xu, S., Nie, J., Wan, S., Li, C.Dong, H. (2023). Alternate intercropping of cotton and peanut increases productivity by increasing canopy photosynthesis and nutrient uptake under the influence of rhizobacteria. Field Crops Research, 302, 109059. https://doi.org/10.1016/j.fcr.2023.109059
- Chimonyo, V. G. P., Modi, A. T., & Mabhaudhi, T. (2016). Simulating yield and water use of a sorghum–cowpea intercrop using APSIM. Agricultural water management, 177, 317–328. https://doi.org/10.1016/j.agwat.2016.08.021
- Cong, W. F., Hoffland, E., Li, L., Six, J., Sun, J. H., Bao, X. G., Zhang, F-S., Van Der Werf, W. (2015). Intercropping enhances soil carbon and nitrogen. Global Change Biology, 21(4), 1715–1726. https://doi.org/10.1111/gcb.12738
- Cramer, W., Guiot, J., Fader, M., Garrabou, J., Gattuso, J. P., Iglesias, A., Lange, M A., Lionello, P., Llasat, M C., Paz, S., Peñuelas, J., Snoussi, M., Toreti, A., Tsimplis, M N., Xoplaki, E. (2018). Climate change and interconnected risks to sustainable development in the Mediterranean. Nature Climate Change, 8(11), 972–980. https://doi.org/10.1038/s41558-018-0299-2
- Crews, T. E., Carton, W., & Olsson, L. (2018). Is the future of agriculture perennial? Imperatives and opportunities to reinvent agriculture by shifting from annual monocultures to perennial polycultures. Global Sustainability, 1, e11. https://doi.org/10.1017/sus.2018.11
- Croijmans, L., van Apeldoorn, D. F., Sanfilippo, F., Zangpo, T., & Poelman, E. H. (2024). Crop species diversity levels with attract and reward strategies to enhance pieris brassicae parasitism rate by cotesia glomerata in strip intercropping. Functional Ecology, 38(3), 654–667. https://doi.org/10.1111/1365-2435.14502
- Dai, J., Qiu, W., Wang, N., Wang, T., Nakanishi, H., & Zuo, Y. (2019). From Leguminosae/Gramineae intercropping systems to see benefits of intercropping on iron nutrition. Frontiers in Plant Science, 10, 605. https://doi.org/10.3389/fpls.2019.00605
- David, O. G. (2023). Metagenomic analysis of rhizospheric soil microbial communities associated with striga infested maize field [ Doctoral dissertation]. North-West University (South Africa).
- Davis, J. H. C., & Woolley, J. N. (1993). Genotypic requirement for intercropping. Field Crops Research, 34(3–4), 407–430. https://doi.org/10.1016/0378-4290(93)90124-6
- Dong, Q., Zhao, X., Zhou, D., Liu, Z., Shi, X., Yuan, Y., Jia, P., Liu, Y.Song, P.Wang, X.Jiang, C., Liu, X., Zhang, He, Zhong, C., Guo, F., Wan, S., Yu, H., Zhang, Z. (2022). Maize and peanut intercropping improves the nitrogen accumulation and yield per plant of maize by promoting the secretion of flavonoids and abundance of Bradyrhizobium in rhizosphere. Frontiers in Plant Science, 13, 957336. https://doi.org/10.3389/fpls.2022.957336
- Dupraz, C., Simorte, V., Dauzat, M., Bertoni, G., Bernadac, A., & Masson, P. (1999). Growth and nitrogen status of young walnuts as affected by intercropped legumes in a Mediterranean climate. Agroforestry Systems, 43, 71–80. https://doi.org/10.1023/A:1026499103899
- Eskandari, H., Ghanbari, A., & Javanmard, A. (2009). Intercropping of cereals and legumes for forage production. Notulae Scientia Biologicae, 1(1), 07–13. https://doi.org/10.15835/nsb113479
- Feng, L., Raza, M. A., Chen, Y., Khalid, M. H. B., Meraj, T. A., Ahsan, F., Fan, Y., Du, J., Wu, X., Song, C., Liu, C., Bawa, G., Zhang, Z., Yuan, S., Yang, F., Yang, W. (2019). Narrow-wide row planting pattern improves the light environment and seed yields of intercrop species in relay intercropping system. Public Library of Science One, 14(2), e0212885. https://doi.org/10.1371/journal.pone.0212885
- Fernandez-Aparicio, M., Sillero, J. C., & Rubiales, D. (2007). Intercropping with cereals reduces infection by orobanche crenata in legumes. Crop Protection, 26(8), 1166–1172. https://doi.org/10.1016/j.cropro.2006.10.012
- Gagné, G., Lorenzetti, F., Cogliastro, A., & Rivest, D. (2022). Soybean performance under moisture limitation in a temperate tree-based intercropping system. Agricultural Systems, 201, 103460. https://doi.org/10.1016/j.agsy.2022.103460
- Gao, X., Wu, M., Xu, R., Wang, X., Pan, R., Kim, H. J., & Liao, H. (2014). Root interactions in a maize/soybean intercropping system control soybean soil-borne disease, red crown rot. PLOS ONE, 9(5), e95031. https://doi.org/10.1371/journal.pone.0095031
- Gardarin, A., Celette, F., Naudin, C., Piva, G., Valantin-Morison, M., Vrignon-Brenas, S., Verret, V., Médiène, S. (2022). Intercropping with service crops provides multiple services in temperate arable systems: A review. Agronomy for Sustainable Development, 42(3), 39. https://doi.org/10.1007/s13593-022-00771-x
- Garrett, H. E., McGraw, R. L., & Walter, W. D. (2009). Alley cropping practices. Chapter 7. In H. Garrett (Ed.), North American agroforestry: an integrated science and practice (2nd ed.). Madison: American Society of Agronomy.
- Ghaffar, A., Rahman, M. H. U., Ahmed, S., Haider, G., Ahmad, I., Khan, M. A., Afzaal, M., Ahmed, S., Fahad, S., Hussain, J., & Ahmed, A. (2022). Adaptations in cropping system and pattern for sustainable crops production under climate change scenarios (p. 10). CRC Press. https://doi.org/10.1201/9781003286417-1.
- Glaze-Corcoran, S., Hashemi, M., Sadeghpour, A., Jahanzad, E., Afshar, R. K., Liu, X., & Herbert, S. J. (2020). Understanding intercropping to improve agricultural resiliency and environmental sustainability. Advances in Agronomy, 162, 199–256. https://doi.org/10.1016/bs.agron.2020.02.004
- Gollin, D., Hansen, C. W., & Wingender, A. M. (2021). Two blades of grass: The impact of the green revolution. Journal of Political Economy, 129(8), 2344–2384. https://doi.org/10.1086/714444
- Gou, F., van Ittersum, M. K., Simon, E., Leffelaar, P. A., van der Putten, P. E., Zhang, L., & van der Werf, W. (2017). Intercropping wheat and maize increases total radiation interception and wheat RUE but lowers maize RUE. The European Journal of Agronomy, 84, 125–139. https://doi.org/10.1016/j.eja.2016.10.014
- Haque, M. A., & Sakimin, S. Z. (2022). Planting arrangement and effects of planting density on tropical fruit crops—A review. Horticulturae, 8(6), 485. https://doi.org/10.3390/horticulturae8060485
- Harmanny, K. S., & Malek, Ž. (2019). Adaptations in irrigated agriculture in the Mediterranean region: An overview and spatial analysis of implemented strategies. Regional Environmental Change, 19(5), 1401–1416. https://doi.org/10.1007/s10113-019-01494-8
- Hatfield, J. L., & Dold, C. (2019). Water-use efficiency: Advances and challenges in a changing climate. Frontiers in Plant Science, 10, 103. https://doi.org/10.3389/fpls.2019.00103
- Homulle, Z., George, T. S., & Karley, A. J. (2021). Root traits with team benefits: Understanding belowground interactions in intercropping systems. Plant and Soil, 471(1–2), 1–26. https://doi.org/10.1007/s11104-021-05165-8
- Horwith, B. (1985). A role for intercropping in modern agriculture. BioScience, 35(5), 286–291. https://doi.org/10.2307/1309927
- Hu, F., Tan, Y., Yu, A., Zhao, C., Coulter, J. A., Fan, Z., Yin, W., Fan, H., & Chai, Q. (2018). Low N fertilizer application and intercropping increases N concentration in pea (Pisum sativum L.) grains. Frontiers in Plant Science, 9, 1763. https://doi.org/10.3389/fpls.2018.01763
- Huang, B., Zou, X., Xu, H., Xu, J., Liu, H., Sun, W., Gong, L., Niu, S., Feng, L., Yang, N., & Zhang, L. (2022). Seedling defoliation of cereal crops increases peanut growth and yield in an intercropping system. The Crop Journal, 10(2), 418–425. https://doi.org/10.1016/j.cj.2021.05.012
- Huang, D., Sun, M., Han, M., Zhang, Z., Miao, Y., Zhang, J., & Yao, Y. (2020). Volatile organic compounds (VOCs) regulate the spatial distribution of Lepidoptera insects in an orchard ecosystem. Biological Control, 149, 104311. https://doi.org/10.1016/j.biocontrol.2020.104311
- Huss, C. P., Holmes, K. D., Blubaugh, C. K., & Adhikari, S. (2022). Benefits and risks of intercropping for crop resilience and pest management. Journal of Economic Entomology, 115(5), 1350–1362. https://doi.org/10.1093/jee/toac045
- Jamil, N., Kootstra, G., & Kooistra, L. (2022). Evaluation of individual plant growth estimation in an intercropping field with UAV imagery. Agriculture, 12(1), 102. https://doi.org/10.3390/agriculture12010102
- Janati, W., Mikou, K., El Ghadraoui, L., & Errachidi, F. (2023). Growth stimulation of two legumes (Vicia faba and Pisum sativum) using phosphate-solubilizing bacteria inoculation. Frontiers in Microbiology, 14. https://doi.org/10.3389/fmicb.2023.1212702
- Jensen, E. S., Carlsson, G., & Hauggaard-Nielsen, H. (2020). Intercropping of grain legumes and cereals improves the use of soil N resources and reduces the requirement for synthetic fertilizer N: A global-scale analysis. Agronomy for Sustainable Development, 40(1), 1–9. https://doi.org/10.1007/s13593-020-0607-x
- Jhariya, M. K., Meena, R. S., & Banerjee, A. (2021). Ecological Intensification of Natural Resources Towards Sustainable Productive System. In M. K. Jhariya, R. S. Meena, & A. Banerjee (Eds.), Ecological Intensification of Natural Resources for Sustainable Agriculture. Singapore: Springer. https://doi.org/10.1007/978-981-33-4203-3_1.
- Juventia, S. D., Norén, I. L. S., Van Apeldoorn, D. F., Ditzler, L., & Rossing, W. A. (2022). Spatio-temporal design of strip cropping systems. Agricultural Systems, 201, 103455. https://doi.org/10.1016/j.agsy.2022.103455
- Kahraryan, B., Farahvash, F., Mohammadi, S., Mirshekari, B., & Rashidi, V. (2021). Evaluation of yield, yield components and nutritive value in intercropping of barley with vetch. Plant Science Today, 8(2), 373–379. https://doi.org/10.14719/pst.2021.8.2.871
- Kaur, N., Bhullar, M. S., & Gill, G. (2016). Weed management in sugarcane-canola intercropping systems in northern India. Field Crops Research, 188, 1–9. https://doi.org/10.1016/j.fcr.2016.01.009
- Koohafkan, P., & Altieri, M. A. (2016). Forgotten agricultural heritage: Reconnecting food systems and sustainable development. Taylor & Francis.
- Kumar, S., Gopinath, K. A., Sheoran, S., Meena, R. S., Srinivasarao, C., Bedwal, S., Jangir, C K., Mrunalini, K.Jat, R., Praharaj, C S. (2023). Pulse-based cropping systems for soil health restoration, resources conservation, and nutritional and environmental security in rainfed agroecosystems. Frontiers in Microbiology, 13, 1041124. https://doi.org/10.3389/fmicb.2022.1041124
- Kumawat, A., Bamboriya, S. D., Meena, R. S., Yadav, D., Kumar, A., Kumar, S., Pradhan, G. (2022). Legume-based inter-cropping to achieve the crop, soil, and environmental health security. In R. S. Meena & S. Kumar (Eds.), Advances in legumes for sustainable intensification (pp. 307–328). Amsterdam, The Netherlands: Elsevier Inc.
- Lai, H., Gao, F., Su, H., Zheng, P., Li, Y., & Yao, H. (2022). Nitrogen distribution and soil microbial community characteristics in a legume–cereal intercropping system: A review. Agronomy, 12(8), 1900. https://doi.org/10.3390/agronomy12081900
- Lamichhane, J. R., Alletto, L., Cong, W. F., Dayoub, E., Maury, P., Plaza-Bonilla, D., Reckling, M., Saia, S., Soltani, E., Tison, G., Debaeke, P. (2023). Relay cropping for sustainable intensification of agriculture across temperate regions: Crop management challenges and future research priorities. Field Crops Research, 291, 108795. https://doi.org/10.1016/j.fcr.2022.108795
- Lanker, M., Bell, M., & Picasso, V. D. (2020). Farmer perspectives and experiences introducing the novel perennial grain Kernza intermediate wheatgrass in the US midwest. Renewable Agriculture and Food Systems, 35(6), 653–662. https://doi.org/10.1017/S1742170519000310
- Latati, M., Aouiche, A., Tellah, S., Laribi, A., Benlahrech, S., Kaci, G., Ouarem, F., Ounane, S M. (2017). Intercropping maize and common bean enhances microbial carbon and nitrogen availability in low phosphorus soil under Mediterranean conditions. European Journal of Soil Biology, 80, 9–18. https://doi.org/10.1016/j.ejsobi.2017.03.003
- Latati, M., Blavet, D., Alkama, N., Laoufi, H., Drevon, J. J., Gerard, F., Pansu, S. M., Ounane, S M. (2014). The intercropping cowpea-maize improves soil phosphorus availability and maize yields in an alkaline soil. Plant and Soil, 385(1–2), 181–191. https://doi.org/10.1007/s11104-014-2214-6
- Li, C., Stomph, T. J., Makowski, D., Li, H., Zhang, C., Zhang, F., & van der Werf, W. (2023). The productive performance of intercropping. Proceedings of the National Academy of Sciences, 120(2), e2201886120. https://doi.org/10.1073/pnas.2201886120
- Li, N., Gao, D., Zhou, X., Chen, S., Li, C., & Wu, F (2020). Intercropping with potato-onion enhanced the soil microbial diversity of tomato. Microorganisms, 8(6), 834. DOI: 10.3390/microorganisms8060834
- Li, Q., Chen, J., Wu, L., Luo, X., Li, N., Arafat, Y., Lin, S., Lin, W. (2018). Belowground interactions impact the soil bacterial community, soil fertility, and crop yield in maize/peanut intercropping systems. International Journal of Molecular Sciences, 19(2), 622. https://doi.org/10.3390/ijms19020622
- Li, X. W., Lu, X. X., Zhang, Z. J., Huang, J. M., Zhang, J. M., Wang, L. K., Hafeez, M., Fernández-Grandon, G M., Lu, Y. B. (2021). Intercropping rosemary (Rosmarinus officinalis) with sweet pepper (Capsicum annum) reduces major pest population densities without impacting natural enemy populations. Insects, 12(1), 74. https://doi.org/10.3390/insects12010074
- Lionello, P., Malanotte-Rizzoli, P., & Boscolo, R. (Eds.). (2006). Mediterranean climate variability. Elsevier.
- Liu, Y., Wu, L., Baddeley, J. A., Watson, C A. (2011). Models of biological nitrogen fixation of legumes. A review. Sustainable Agriculture, 31(1), 155–172. https://doi.org/10.1051/agro/2010008
- Lv, Q., Chi, B., He, N., Zhang, D., Dai, J., Zhang, Y., & Dong, H. (2023). Cotton-based rotation, intercropping, and alternate intercropping increase yields by improving root–shoot relations. Agronomy, 13(2), 413. https://doi.org/10.3390/agronomy13020413
- Ma, H., Zhou, J., Ge, J., Nie, J., Zhao, J., Xue, Z., Hu, Y., Yang, Y., Peixoto, L., Zang, H., Zeng, Z. (2022). Intercropping improves soil ecosystem multifunctionality through enhanced available nutrients but depends on regional factors. Plant and Soil, 480(1–2), 71–84. https://doi.org/10.1007/s11104-022-05554-7
- Maina, J. M. (1997). Effects of Intercropping on weeds and weed management in maize growing in Kenya [ PhD Thesis]. University of Reading.
- Maitra, S., Hossain, A., Brestic, M., Skalicky, M., Ondrisik, P., Gitari, H., Brahmachari, K., Shankar, T., Bhadra, P., Palai, J. B., Jena, J., Bhattacharya, U., Duvvada, S K., Lalichetti, S., Sairam, M. (2021). Intercropping—A low input agricultural strategy for food and environmental security. Agronomy, 11(2), 343. https://doi.org/10.3390/agronomy11020343
- Maitra, S., & Ray, D. P. (2019). Enrichment of biodiversity, influence in microbial population dynamics of soil and nutrient utilization in cereal-legume intercropping systems: A review. International Journal of Bioresource Science, 6(1), 11–19. https://doi.org/10.30954/2347-9655.01.2019.3
- Mazzafera, P., Favarin, J. L., & Andrade, S. A. L. D. (2021). Editorial: Intercropping systems in sustainable agriculture. Frontiers in Sustainable Food Systems, 5, 634361. https://doi.org/10.3389/fsufs.2021.634361
- Mickelbart, M. V., Hasegawa, P. M., & Bailey-Serres, J. (2015). Genetic mechanisms of abiotic stress tolerance that translate to crop yield stability. Nature Reviews Genetics, 16(4), 237–251. https://doi.org/10.1038/nrg3901
- Monti, M., Pellicanò, A., Pristeri, A., Badagliacca, G., Preiti, G., & Gelsomino, A. (2019). Cereal/grain legume intercropping in rotation with durum wheat in crop/livestock production systems for Mediterranean farming system. Field Crops Research, 240, 23–33. https://doi.org/10.1016/j.fcr.2019.05.019
- Morales-Rosales, E. J., & Franco-Mora, O. (2009). Biomass, yield and land equivalent ratio of Helianthus annus L. in sole crop and intercropped with Phaseolus vulgaris L. in high valleys of Mexico. Tropical and Subtropical Agroecosystems, 10(3), 431–439.
- Mortensen, M., Bastiaans, B., & Sattin, S. (2000). The role of ecology in the development of weed management systems: An outlook. Weed Research, 40(1), 49–62. https://doi.org/10.1046/j.1365-3180.2000.00174.x
- Mousavi, S. R., & Eskandari, H. (2011). A general overview on intercropping and its advantages in sustainable agriculture. Journal of Applied Environmental and Biological Sciences, 1(11), 482–486.
- Nicholls, C. I., & Altieri, M. A. (2013). Plant biodiversity enhances bees and other insect pollinators in agroecosystems. A review. Agronomy for Sustainable Development, 33(2), 257–274. https://doi.org/10.1007/s13593-012-0092-y
- Nyawade, S. O., Karanja, N. N., Gachene, C. K., Gitari, H. I., Schulte-Geldermann, E., & Parker, M. L. (2019). Intercropping optimizes soil temperature and increases crop water productivity and radiation use efficiency of rainfed potato. American Journal of Potato Research, 96(5), 457–471. https://doi.org/10.1007/s12230-019-09737-4
- Olowe, V. I. O., & Adebimpe, O. A. (2009). Intercropping sunflower with soyabeans enhances total crop productivity. Biological Agriculture & Horticulture, 26(4), 365–377. https://doi.org/10.1080/01448765.2009.9755095
- Pélissier, R., Violle, C., & Morel, J. B. (2021). Plant immunity: Good fences make good neighbors? Current Opinion in Plant Biology, 62, 102045. https://doi.org/10.1016/j.pbi.2021.102045
- Pickett, J. A., & Khan, Z. R. (2016). Plant volatile‐mediated signalling and its application in agriculture: Successes and challenges. New Phytologist, 212(4), 856–870. https://doi.org/10.1111/nph.14274
- Pierre, J. F., Latournerie-Moreno, L., Garruña, R., Jacobsen, K. L., Laboski, C. A., Us-Santamaría, R., & Ruiz-Sánchez, E. (2022). Effect of maize–legume intercropping on maize physio-agronomic parameters and beneficial insect abundance. Sustainability, 14(19), 12385. https://doi.org/10.3390/su141912385
- Pourali, S., Aghayari, F., Ardakani, M. R., Paknejad, F., & Golzardi, F. (2023). Benefits from intercropped forage sorghum–red clover under drought stress conditions. Gesunde Pflanzen, 75(5), 1769–1780. https://doi.org/10.1007/s10343-023-00833-4
- Qiao, X., Gao, Y., Xiao, L., Lyu, X., Zhang, C., Zhang, Y., Sai, L., Xue, L., Lei, J. (2021). Intercropping wheat between walnut trees reduced yield and improved quality. Agronomy Journal, 113(2), 1058–1070. https://doi.org/10.1002/agj2.20498
- Qu, Q., Zhang, Z., Peijnenburg, W. J. G. M., Liu, W., Lu, T., Hu, B., Chen, J., Chen, J., Lin, Z., Qian, H. (2020). Rhizosphere microbiome assembly and its impact on plant growth. Journal of Agricultural and Food Chemistry, 68(18), 5024–5038. https://doi.org/10.1021/acs.jafc.0c00073
- Rahman, M. K., Wang, X., Gao, D., Zhou, X., Wu, F. (2021). Root exudates increase phosphorus availability in the tomato/potato onion intercropping system. Plant and Soil, 464(1–2), 45–62. https://doi.org/10.1007/s11104-021-04935-8
- Raza, M. A., Cui, L., Qin, R., Yang, F., & Yang, W. (2020). Strip-width determines competitive strengths and grain yields of intercrop species in relay intercropping system. Scientific Reports, 10(1), 21910. https://doi.org/10.1038/s41598-020-78719-y
- Raza, M. A., Yasin, H. S., Gul, H., Qin, R., Mohi Ud Din, A., Khalid, M. H. B., Hussain, S., Gitari, H., Saeed, A., Wang, J., & Rezaei-Chiyaneh, E. (2022). Maize/soybean strip intercropping produces higher crop yields and saves water under semi-arid conditions. Frontiers in Plant Science, 13, 1006720. https://doi.org/10.3389/fpls.2022.1006720
- Ren, Y. Y., Wang, X. L., Zhang, S. Q., Palta, J. A., & Chen, Y. L. (2017). Influence of spatial arrangement in maize-soybean intercropping on root growth and water use efficiency. Plant and Soil, 415, 131–144. https://doi.org/10.1007/s11104-016-3143-3
- Riemens, M., Sønderskov, M., Moonen, A. C., Storkey, J., & Kudsk, P. (2022). An integrated weed management framework: A pan-European perspective. European Journal of Agronomy, 133, 126443. https://doi.org/10.1016/j.eja.2021.126443
- Ripoche, A., Rellier, J. P., Martin-Clouaire, R., Paré, N., Biarnès, A., & Gary, C. (2011). Modelling adaptive management of intercropping in vineyards to satisfy agronomic and environmental performances under Mediterranean climate. Environmental Modelling & Software, 26(12), 1467–1480. https://doi.org/10.1016/j.envsoft.2011.08.003
- Roder, W., Anderhalden, E., Gurung, P., & Dukpa, P. (1992). Potato intercropping systems with maize and faba bean. American Potato Journal, 69(3), 195–202. https://doi.org/10.1007/BF02856552
- Saad, A. H., El Naim, A. M., Ahmed, A. A., Ibrahim, K. A., Islam, M. S., Al-Qthanin, R. N., & Idris, A. M. (2022). Response of sesame to intercropping with groundnut and cowpea. Communications in Soil Science & Plant Analysis, 53(17), 2285–2296. https://doi.org/10.1080/00103624.2022.2071438
- Saia, S., Urso, V., Amato, G., Frenda, A. S., Giambalvo, D., Ruisi, P., & DiMiceli, G. (2016). Mediterranean forage legumes grown alone or in mixture with annual ryegrass: Biomass production, N2 fixation, and indices of intercrop efficiency. Plant and Soil, 402(1–2), 395–407. https://doi.org/10.1007/s11104-016-2837-x
- Sarto, M. V. M., Borges, W. L. B., Bassegio, D., Rice, C., & Rosolem, C. A. (2021). Maize and sorghum root growth and yield when intercropped with forage grasses. Agronomy Journal, 113(6), 4900–4915. https://doi.org/10.1002/agj2.20920
- Shrestha, J., Subedi, S., Timsina, K. P., Subedi, S., Pandey, M., Shrestha, A., Shrestha, S. Hossain, M A. (2021). Sustainable intensification in agriculture: An approach for making agriculture greener and productive. Journal of Nepal Agricultural Research Council, 7, 133–150. https://doi.org/10.3126/jnarc.v7i1.36937
- Stomph, T., Dordas, C., Baranger, A., de Rijk, J., Dong, B., Evers, J., van Der Werf, W. (2020). Designing intercrops for high yield, yield stability and efficient use of resources: Are there principles? Advances in Agronomy, 160(1), 1–50.
- Su, K., Mu, L., Zhou, T., Kamran, M., & Yang, H. (2024). Intercropped alfalfa and spring wheat reduces soil alkali-salinity in the arid area of northwestern China. Plant and Soil, 499(1–2), 275–292. https://doi.org/10.1007/s11104-022-05846-y
- Ullah, M. A., Hussain, N., Schmeisky, H., Rasheed, M., Anwar, M., & Rana, A. S. (2018). Fodder quality improvement through intercropping and fertilizer application. Pakistan Journal of Agricultural Sciences, 55(3), 549–554. https://doi.org/10.21162/PAKJAS/18.4579
- UNFPA. (2023). https://www.un.org/en/global-issues/population
- Wang, G., Bei, S., Li, J., Bao, X., Zhang, J., Schultz, P. A., Li, H., Li, L., Zhang, F., Bever, J D., Zhang, J. (2021). Soil microbial legacy drives crop diversity advantage: Linking ecological plant–soil feedback with agricultural intercropping. Journal of Applied Ecology, 58(3), 496–506. https://doi.org/10.1111/1365-2664.13802
- Wang, L., Yu, B., Ji, J., Khan, I., Li, G., Rehman, A., Liu, D., & Li, S. (2023). Assessing the impact of biochar and nitrogen application on yield, water-nitrogen use efficiency and quality of intercropped maize and soybean. Frontiers in Plant Science, 14, 1171547. https://doi.org/10.3389/fpls.2023.1171547
- Wang, S., Chen, G., Yang, Y., Zeng, Z., Hu, Y., & Zang, H. (2021). Sowing ratio determines forage yields and economic benefits of oat and common vetch intercropping. Agronomy Journal, 113(3), 2607–2617. https://doi.org/10.1002/agj2.20647
- Wasaki, J., & Dissanayaka, D. M. S. B. (2021). Intercropping to Maximize Root–Root Interactions in Agricultural Plants. In Z. Rengel & I. Djalovic (Eds.), The Root Systems in Sustainable Agricultural Intensification. https://doi.org/10.1002/9781119525417.ch11
- Willey, R. (1990). Resource use in intercropping systems. Agricultural Water Management, 17(1–3), 215–231. https://doi.org/10.1016/0378-3774(90)90069-B
- Workayehu, T., & Wortmann, C. S. (2011). Maize–bean intercrop weed suppression and profitability in Southern Ethiopia. Agronomy journal, 103(4), 1058–1063. https://doi.org/10.2134/agronj2010.0493
- World Bank. (2023). Arable land (hectares per person) [CC BY-4.0]. https://data.worldbank.org/indicator/AG.LND.ARBL.HA.PC
- Wu, J., Liu, W., & Chen, C. (2016). Below-ground interspecific competition for water in a rubber agroforestry system may enhance water utilization in plants. Scientific Reports, 6(1), 19502. https://doi.org/10.1038/srep19502
- Yang, H., Zhang, W., & Li, L. (2021). Intercropping: Feed more people and build more sustainable agroecosystems. Frontiers of Agricultural Science and Engineering, 8(3), 373–386.
- Yang, T., Siddique, K. H., & Liu, K. (2020). Cropping systems in agriculture and their impact on soil health-a review. Global Ecology and Conservation, 23, e01118. https://doi.org/10.1016/j.gecco.2020.e01118
- Ye, L., Zhao, X., Bao, E., Li, J., Zou, Z., & Cao, K. (2020). Bio-organic fertilizer with reduced rates of chemical fertilization improves soil fertility and enhances tomato yield and quality. Scientific Reports, 10(1), 177. https://doi.org/10.1038/s41598-019-56954-2
- Yin, W., Chai, Q., Zhao, C., Yu, A., Fan, Z., Hu, F., Fan, H., Guo, Y., Coulter, J. A. (2020). Water utilization in intercropping: A review. Agricultural Water Management, 241, 106335. https://doi.org/10.1016/j.agwat.2020.106335
- Yin, W., Fan, Z., Hu, F., Fan, H., Yu, A., Zhao, C., & Chai, Q. (2019). Straw and plastic mulching enhances crop productivity via optimizing interspecific interactions of wheat–maize intercropping in arid areas. Crop Science, 59(5), 2201–2213. https://doi.org/10.2135/cropsci2019.02.0082
- Yu, J., Rezaei, E. E., Thompson, J. B., Reckling, M., & Nendel, C. (2024). Modelling crop yield in a wheat–soybean relay intercropping system: A simple routine in capturing competition for light. European Journal of Agronomy, 153, 127067. https://doi.org/10.1016/j.eja.2023.127067
- Yuvaraj, M., Pandiyan, M., & Gayathri, P. (2020). Role of legumes in improving soil fertility status. In Legume Crops-Prospects, Production and Uses (pp. 16–27). IntechOpen.
- Zakir, I., Wahab, A. A., Abbas, T., Haider, S. T. A., Irum, S., Sabir, S., Hussain, S., & Ahmad, S. (2023). Cereal–vegetable intercropping: Hindrances and strategies to increase intercropping. Turkish Journal of Agriculture and Forestry, 47(6), 1115–1129. https://doi.org/10.55730/1300-011X.3151
- Zhang, J., Yin, B., Xie, Y., Li, J., Yang, Z., & Zhang, G., Cheng, Z. (2015). Legume-cereal intercropping improves forage yield, quality and degradability. Public Library of Science One, 10(12), e0144813. https://doi.org/10.1371/journal.pone.0144813
- Zhao, X., Dong, Q., Han, Y., Zhang, K., Shi, X., Yang, Xu, Yuan, Y., Zhou, D., Wang, K., Wang, X., Jiang, C., Liu, X., Zhang, He, Zhang, Z., Yu, H. (2022). Maize/peanut intercropping improves nutrient uptake of side-row maize and system microbial community diversity. BMC Microbiology, 22(1), 14. https://doi.org/10.1186/s12866-021-02425-6
- Zhao, X., Hao, C., Zhang, R., Jiao, N., Tian, J., Lambers, H., Liang, C., Cong, W. F., Zhang, F. (2023). Intercropping increases soil macroaggregate carbon through root traits induced microbial necromass accumulation. Soil Biology and Biochemistry, 185, 109146. https://doi.org/10.1016/j.soilbio.2023.109146
- Zhao, Y., Guo, S., Zhu, X., Zhang, L., Long, Y., Wan, X., & Wei, X. (2024). How maize-legume intercropping and rotation contribute to food security and environmental sustainability. Journal of Cleaner Production, 434, 140150. https://doi.org/10.1016/j.jclepro.2023.140150
- Zhou, Y., Chen, C., Franck, W. L., Khan, Q., Franck, S., Crutcher, F. K., McVay, K., McPhee, K. (2023). Intercropping chickpea–flax for yield and disease management. Agronomy Journal, 115(2), 726–743. https://doi.org/10.1002/agj2.21280
- Ziaie-Juybari, H., Pirdashti, H., Abo-Elyousr, K. A., & Mottaghian, A. (2021). Abiotic benefits of intercropping legumes and maize to reduce pests. Archives of Phytopathology and Plant Protection, 54(17–18), 1539–1552. https://doi.org/10.1080/03235408.2021.1919592
- Zou, X. X., Shi, P. X., Zhang, C. J., Si, T., Wang, Y. F., Zhang, X. J., & Yu, X-N., Wang, H-X., Wang, M-L. (2021). Rotational strip intercropping of maize and peanuts has multiple benefits for agricultural production in the northern agropastoral ecotone region of China. European Journal of Agronomy, 129, 126304. https://doi.org/10.1016/j.eja.2021.126304