ABSTRACT
Green and energy-saving reforms, which use protection as a precondition, can, not only reduce a building’s energy use but also improve its level of comfort. Such reforms can also adapt to the new demands of modern society while extending the life of traditional architecture. Therefore, this research chose a classical, traditional house in Hangzhou Zhejiang, China, as a transformation object – attempting to implement low-cost, energy-saving technology. The building’s energy consumption and level of comfort before and after the transformation have been simulated and measured to compare the effects of the transformation. The authors hope this research will provide insights into green and energy-saving reform technology and aid the sustainable development of traditional houses.
Graphical Abstract
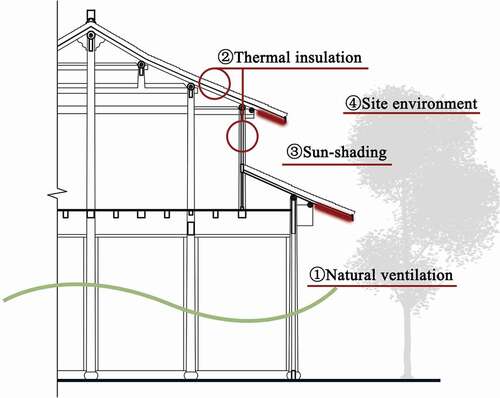
1. Introduction
The world’s global dependence on energy has been increasing at an alarming rate (Martínez-Molina et al. Citation2016). According to the International Energy Agency, from 1971 to 2014, world-wide energy consumption increased by 92% (IEA Citation2014). Buildings are the largest sector and will continue to be a source of increasing energy demand in the future (Sesana and Salvalai Citation2018). The international community – for example the United States, the European Union, and China – has taken definitive steps to curtail these global trends (Martínez-Molina et al. Citation2016). Due to the difficulty of implementing energy efficiency measures that do not compromise the architectural and other core values sought to be preserved, most building energy efficiency laws exclude historic buildings (European Parliament and the Council Citation2012). However, historic and traditional buildings are estimated to make up between 10% and 40% of the building stock, depending on the region and age threshold (Webb Citation2017). At the same time, with economic and social development and the improvement of people’s living standards, the low thermal comfort and high energy consumption of traditional buildings can no longer meet the new needs of modern society. Therefore, balancing energy efficiency, architectural heritage conservation requirements, and users’ thermal comforts is an inevitable consideration in historic buildings renovation research.
Early research on traditional buildings mainly focused on spatial composition, cultural background, and construction experience, etc., with less consideration given to energy-saving performance and thermal comfort. Since 2010, the thermal comfort and energy consumption of traditional buildings have attracted more and more scholarly attention all over the world (Wang, Wei, and He Citation2004). The current research is mainly concentrated in Europe, especially in Italy (Cardinale et al. Citation2011; Alongi et al. Citation2015; Ascione, Rossi, and Vanoli Citation2011; Cardinale, Rospi, and Stefanizzi Citation2013), with earth (Li et al. Citation2013) or stone buildings (Cardinale et al. Citation2011; Alongi et al. Citation2015; Ascione, Rossi, and Vanoli Citation2011; Cardinale, Rospi, and Stefanizzi Citation2013) as the research object, rather than wooden buildings. Cardinale et al. (Citation2011) quantified the thermo-hygrometric performance of the base materials in Italian historic buildings through in situ measurements. Alongi et al. (Citation2015) examined occupant thermal comfort in a historic castle, concluding that a mechanical cooling system needed to be installed as part of the retrofit project. Ascione, Rossi, and Vanoli (Citation2011) assessed occupant thermal comfort under new temperature setpoints. Li et al. (Citation2013) conducted a field investigation of indoor environmental quality (IEQ) in traditional rural buildings (Tulou) and found that, regarding the luminous environment and indoor air quality, the sensations for residents in Tulou and normal rural buildings were of no obvious distinction. Cardinale, Rospi, and Stefanizzi (Citation2013) also studied two types of buildings, which are the examples of vernacular architecture in Southern Italy. The substantially constant values of indoor seasonal temperatures affirmed that these buildings do not require air conditioning during summer. The seasonal heat storage can assure near comfort levels by using simple heating systems during the cold season.
Existing research on thermal comfort and the energy-saving renovation of traditional buildings has mainly focused on single measures, such as increasing the heat preservation performance of walls (Bianco et al. Citation2015; Walker and Pavía Citation2015), improving the thermal insulation performance of windows (Frey et al. Citation2012; Heath and Baker Citation2013), adjusting air conditioning equipment (Schibuola, Scarpa, and Tambani Citation2018), etc. However, due to the limitations set by traditional architectural heritage protection requirements, comprehensive renovation mostly adopts the simulation method to analyse the energy-saving potential of buildings, but there are a few cases of traditional buildings undergoing energy-saving renovations. For example, Ascione et al. (Citation2015) analysed energy efficiency measures by hourly energy simulations of an educational building in the ancient centre of Benevento. They found that the most suitable combination of energy efficiency measures in this kind of building is replacing windows with low-emissive versions, applying thermal plaster, and insulating the roof slab with the new thermal insulation. Galatioto et al. (Citation2019) applied some permitted retrofit actions to a public historic building to analyse, by simulating, the effectiveness of national measures in four different climatic zones. The results showed that the same applied action that reduces primary energy consumption in some zones will significantly increase consumption in other zones. Alev et al. (Citation2014) assembled a package of retrofits aimed at improving occupant thermal comfort in traditional, single-family dwellings, while Geva (Citation1998) evaluated total discomfort degree hours under the original design conditions, current conditions, and several potential retrofits.
Chinese architecture originated in 4000 BC, the middle of the Neolithic period, with wood and brick being the main building materials and wood frame structures being the main structural mode (Liang Citation2011). Chinese architecture is completely different from Western architecture in terms of building materials, building structure, spatial layout, and artistic aesthetic. Indoor thermal comfort performance and energy consumption are also very different from that found in Western architecture.
This paper took the traditional houses in Hangzhou as the research object. Hangzhou, Zhejiang, China, is a thousand-year-old city located in the middle and lower reaches of the Yangtze River in southeast China. It belongs to the subtropical monsoon climate zone and has hot summers and cold winters. In 2018, the average temperature of Hangzhou in summer and winter is 28.4°C and 5.9°C, respectively. It has an extreme high temperature of 39.4°C and an extreme low temperature of −7.5°C. And the average annual relative humidity in Hangzhou is 76%-81%. Jiangnan traditional houses are a typical historical building type in Hangzhou and are also one of the most important types of traditional Chinese buildings, with distinct national, and rich local, characteristics. Hangzhou, with its more than 280 historical buildings, 15 historic blocks, and nearly 1.8 million square meters of traditional buildings, is one of the first ten pilot cities, chosen by the Ministry of Housing and Urban-Rural Development of China, for the protection and use of historical buildings. In 2018, the Hangzhou Municipal Government promulgated the “Pilot Work Plan for the Protection and Utilization of the Historic Building in Hangzhou,” which intends to promote the effective protection and sustainable use of historic buildings by opening up cultural exhibitions, leisure spaces, hotels, homestays, and other forms of use. Therefore, how to reduce the energy consumption of a traditional building, improve its comfort level, make it adapt to the needs of modern society, and encourage people to voluntarily use and protect it, without affecting its traditional style and cultural value, is an important issue, which must urgently be solved.
This research chose Luo’s house, a classical, traditional house in Hangzhou, as the transformation object. First, its indoor thermal environment and thermal comfort level were evaluated to explore existing problems and analyse their causes. Then, intending to retain the building’s original style while solving key thermal environment problems, suitable low-cost, energy-saving technologies – such as site design, ventilation treatment, sun-shading measures, and enclosure structure reconstruction – were used to renovate the indoor thermal environment of Luo’s house. The energy consumption and the comfort of the building before and after transformation have been simulated and measured to compare the effects of the transformation.
2. Methodology
2.1. Case study description
Luo’s house was built during the Qing Dynasty (1830) and was originally the residence of the rich Luo family. It is located at no. 79–88, Chating Pian, Pengbu Town, Jianggan District, Hangzhou. The building covers an area of 748 square meters, with a building area of 809 square meters. It is a traditional, wooden structure house facing south. The gate building, front yard, main building, and back yard are arranged from south to north, and there are wing rooms on the east and west sides of the front and back yards. The main building is divided into five rooms and two floors, while the gate building and the wing rooms are all single-floor, and the original wooden stairs and floors are preserved. The building has a complete pattern, rigorous structure, and complete functions – a typical, large-scale house with a courtyard pattern, exhibiting the structural characteristics of traditional houses in the Jiangnan region during the late Qing Dynasty (). For various historical reasons, 17 families lived together in the old house before it was renovated. The east wing, west wing, and main rooms were divided into several sections, each of which was very cramped, and the building’s style was damaged to a certain extent. The residents generally stated that the indoor environment could no longer meet the requirements of modern society.
2.2. Indoor environment measurement
In early March 2012 and mid-July 2013, the JT-IAQ indoor thermal environment comfort instrument was used to monitor indoor air temperature, relative humidity, thermal comfort, and other environmental conditions before the transformation of Luo’s house. Three measuring points were set up in the main space of the outdoor courtyard and inside the house (): Measuring Point 1 (outdoor eaves on the first floor), Measuring Point 2 (bedroom on the first floor), and Measuring Point 3 (bedroom on the second floor).
To assess the indoor thermal comfort of the building, this research used the PMV (Predicted Mean Vote) – PPD (Predicted Percentage Dissatisfied) indices (including six variables: metabolic rate, clothing insulation, air temperature, average radiation temperature, relative air velocity, relative humidity), which P. O. Fanger of Denmark has proposed for evaluating indoor thermal environments. The relationship between PMV and PPD values and human thermal sensation is shown in .
Table 1. The relationship between PMV and PPD values and human thermal sensation
Referring to China’s national standard – GB/T 18,049–2000 “Moderate thermal Environments – Determination of the PMV and PPD Indices and Specification of the Conditions for Thermal Comfort” – the thermal comfort parameters in this paper were as follows:
Assume that people are mainly seated at home, so the metabolic rate is 1.0 met (58.15 W/㎡).
Clothing insulation in winter is 2.2clo (0.341㎡·°C/W), and clothing insulation in summer is 0.3clo (0.045㎡·°C/W).
The heat consumed by external work is 0, which is negligible for most activities.
2.2.1. The measured temperatures
The hourly temperatures of the three measuring points on a typical winter day are shown in . The temperatures of the outdoor eaves fluctuated to a certain extent, with the minimum temperature being 4.6°C and the maximum temperature being 7°C. The indoor temperature remained relatively stable, without obvious fluctuations, showing that the building’s envelope has a certain level of heat preservation performance. The average temperature of the outdoor eaves was 5.4°C; the average temperature of the bedroom on the first floor was 6.2°C; and the average temperature of the bedroom on the second floor was almost the same as that of the outdoor eaves at approximately 5.4°C.
The indoor and outdoor temperature differences on a typical winter day are shown in . From 2:00 pm to 10:00 am, the indoor and outdoor temperature difference on the first floor was about 1°C, and the indoor and outdoor temperature difference on the second floor was 0–0.5°C. At noon, the indoor and outdoor temperatures on the first floor were very similar, while the indoor temperature on the second floor was 0.2–1.1°C lower than the outdoor temperature. This shows that the thermal insulation performance of the building envelope is poor, due to the lack of insulation measures on the roof and the small window area on the second floor. The insulation performance at night and the direct solar heat gain capacity of the second floor are not as good as that of the first floor.
The hourly temperatures of the three measuring points on a typical summer day are shown in . The temperature of the outdoor eaves fluctuated significantly, varying from 29.1°C to 35.8°C, with the average temperature being 32.8°C. The temperature of the bedroom on the first floor fluctuated between 28.6°C and 32°C, with the average temperature being 30°C. The average temperature of the bedroom on the second floor was 31.7°C.
The indoor and outdoor temperature differences on a typical summer day are shown in . Except for 1:20 pm-5:00 pm, the temperature difference between the first floor and the outdoors was 2–4°C, and the temperature difference between the second floor and the outdoors was 1–2°C. This shows that this type of traditional house has certain insulation effects in summer, but, due to the lack of insulation measures on the roof, the thermal environment of the second floor is not as good as that of the first floor.
2.2.2. Measured humidity
The humidity monitoring results are shown in below. The indoor humidity was obviously affected by the outdoors, but the change was relatively stable. It was rainy during the winter testing, and the humidity was generally high. The outdoor relative humidity even reached more than 100%, with an average value of 97.2% and a range of 85.3–100%. The average relative humidity of the first floor was approximately 95%; the maximum value was 97.1%, and the minimum value was 88.11%, with variation being relatively stable. The relative humidity of the second floor was obviously high, with an average of 99.51%.
In summer, the average outdoor relative humidity was 53.7%. The humidity fluctuated significantly, ranging from 25% to 77.4%. The average relative humidity of the first floor was about 68%, and that of the second floor was lower, at 56%.
2.2.3. Thermal comfort evaluation
The indoor and outdoor PMV values in winter and summer are shown in below. In winter, the average PMV value for the bedroom on the first floor was −1.563. It reached −1.472 at the highest and −1.650 at the lowest, far from the thermal comfort standard of −0.75–0.75. Over a 24-h period, the PMV value in the daytime was slightly higher than that in the evening, and it reaches its lowest value in the early morning. The indoor thermal comfort of the bedroom on the second floor was worse than the outdoors, and the average PMV value was −2.071. Therefore, when there is no heating equipment in winter, the indoor comfort level cannot meet the current national standard or thermal comfort requirements, and residents generally feel cold.
In summer, without any cooling equipment, the indoor PMV value changed greatly over 24 hours. The average PMV value in the bedroom on the first floor was 0.82. It reached 1.55 at its highest and 0.17 at its lowest. From 10:00 pm to 8:00 am, this room can basically meet the national PMV standard of −0.75–0.75, but people will feel a little hot in the bedroom at other times. The indoor thermal comfort of the bedroom on the second floor was worse than that on the first floor, with an average PMV value of 1.41, but it can still meet the national standard between 12:00 am and 8:00 am; at other times, people may feel hot. Therefore, when there is no cooling equipment in summer, the indoor comfort of the building during the day cannot meet the current national standard. Users generally feel hot during the day but comfortable at night.
2.2.4. Indoor thermal environment characteristics and their causes
According to the results of the thermal and humidity environment monitoring, as well as the indoor thermal comfort evaluation, the indoor thermal environment characteristics of traditional houses in the Jiangnan region, as represented by Luo’s house, may be defined as follows.
In winter (both day and night), the indoor temperature is almost the same as the outdoor temperature. Users generally feel cold; the thermal comfort is far lower than the current national standard; and the indoor thermal comfort of the second floor is even lower than the outdoors. Therefore, measures, such as improving the thermal insulation performance of the structure and improving the airtightness of doors and windows, should be taken. At the same time, because traditional houses in the Jiangnan region are mostly less than two stories high, the large, sloping roofs make roof insulation particularly important.
The indoor temperature in summer is slightly lower than the outdoor temperature. Benefiting from natural ventilation, thermal comfort at night can basically meet the national standard. However, indoor comfort during the day cannot do so, and users generally feel hot. Therefore, it is necessary to carry out thermal insulation and sun-shading measures and to improve the natural ventilation, bolstering thermal comfort at all times, especially during the day.
2.3. Retrofit measures
According to the results from the above analysis, this study renovated four aspects of Luo’s house: natural ventilation, thermal insulation, component sunshade, and site environment. It also applied appropriate low-tech, energy-saving technology to improve and balance the current situation of the building’s thermal environment.
Natural ventilation
Due to protection requirements, the windows in the external walls, which users originally created for ventilation, had to be completely closed. Although this restores the historical design of the building, it objectively weakens indoor ventilation for the summer. Therefore, according to the traditional form of Jiangnan houses, it was suggested to increase the areas of the landscape windows on the south, west, and east courtyard walls (red portions of ). At the same time, the researchers ensured that there was enough area to open the doors and windows of the rooms adjacent to the inner courtyard, thus enhancing natural ventilation in the summer. Tall trees were also planted in the north of the building to slow down the wind speed in winter.
(2) Thermal insulation
The weather in Hangzhou is hot in summer, cold and humid in winter, so the thermal insulation of the enclosure is very important. The original walls of Luo’s house were cavity walls, commonly used in traditional wooden buildings (). The middle cavity in these walls, filled with air, had a certain thermal insulation effect, but it could not meet the thermal comfort requirements of modern life. According to the requirements of “repairing as old,” the original cavity walls were retained, and a layer of 30 mm inorganic thermal insulation mortar was added outside the walls () to strengthen the thermal insulation performance of the external walls.
Due to the large, sloping roofs of traditional houses, the thermal insulation of the roof is very important. Before the renovation, Luo’s house had a conventional roof structure of purlins, roof boarding, and green tiles. However, the above results show that the thermal comfort performance of the second story was very poor. Therefore, the researchers added a layer of 50 mm extruded polystyrene board to the roof, hoping to improve its thermal insulation performance and the thermal comfort of the second floor ().
The brick walls facing the patio have been replaced by traditional wooden doors and windows in the renovation, just like what it used to be during the Qing Dynasty (1830). So the areas of the hollow windows on the south, west, and east courtyard walls were increased (). While restoring the traditional architectural style, the natural ventilation in summer was enhanced. However, the thermal insulation and airtightness of the hollow windows were very poor in winter. Therefore, double-layer fir board with double-deck glass was adopted, which greatly improved the thermal insulation performance of the doors and windows ().
(3) Sun-shading measures
In the project, the building’s cornice was simulated and optimized for sunshade in summer. The depth of the cornice was increased locally (red portions of ), which not only blocks excessive sunlight but also serves as a transition space, organizing ventilation, improving the comfort of the indoor environment, and acting as an air buffer layer. The east and west walls of the building were given three-dimensional greening technology, such as creepers, which require no maintenance. This not only enriched the landscape but also provided good thermal insulation performance.
(4) Site environment
Broad-leaved plants (such as loquat, plantain, etc.) were planted in the courtyard to increase the transpiration of plants, reduce ground temperature, reduce radiant ground heat, and create a more comfortable indoor environment. Laying bluestone slabs on the roads in the courtyard and paving traditional stone parquet floors on the other activity sites not only created a better traditional architectural atmosphere but also made rainwater quickly penetrate into the ground surface, effectively supplementing groundwater, alleviating the “urban heat island” effect, and protecting the city’s natural water system from damage. At the same time, a rain pipe was set at the corner of the eaves gutter on the patio to collect rainwater into a water tank or collector on the ground. The vaporization of this water was used to cool the house’s environment, so as to regulate the local microclimate.
3. Results and analysis
In September 2014, the renovation project of Luo’s house was completed (), which has restored the architectural pattern and original style of the building. And now the building is operated as a small museum for people to experience the traditional feeling. Heating and cooling equipment is continuously operated during winter and summer. So the project team measured the building’s indoor thermal environment and assessed its indoor thermal comfort in transition season, and simulated energy consumption to compare the effects of the transformation.
3.1. Thermal comfort evaluation
From 12:25 pm on 18 September 2014 to 14:15 pm on 19 September 2014, the researchers monitored the indoor temperature, relative humidity, and thermal comfort of Luo’s house for 24-h periods. Five measuring points were used: Measuring Point 1 (outdoor eaves on the first floor), Measuring Point 2 (the first room on the first floor), Measuring Point 3 (the west wing room on the first floor), Measuring Point 4 (the second room on the first floor), and Measuring Point 5 (the room on the second floor). Among these, Measuring Points 1, 2, and 5 corresponded to the three measuring points used before the transformation, and the thermal comfort results according to the PMV-PPD formula are shown in .
In late summer and early autumn, it was hot during the day and cold at night, so the outdoor PMV value changed greatly. The minimum PMV value reached −1.87, and the dissatisfaction rate reached 70%. Without any heating and cooling equipment, the PMV value in the room changed little over 24 hours. The average PMV value in the room on the first floor was about −0.7; the highest value was close to 0; and the lowest value was about −1.2. The national standard for indoor thermal comfort is a PMV value between – 0.75 and 0.75. shows that the thermal comfort of the building was poor from 11:30 pm to 10:30 am, but, at all other times, it was relatively comfortable. After the transformation, the PMV value of the room on the second floor changed greatly compared with that on the first floor; but the overall comfort was high, and the dissatisfaction rate was maintained around 20%. Generally speaking, after the energy-saving transformation, the thermal comfort of the building can basically meet national standards in late summer and early autumn. However, in summer and winter, it still requires cooling or heating equipment to improve comfort levels.
3.2. Energy consumption simulation
To analyse changes in the building’s energy consumption before and after the thermal environment transformation, this study used PKPM software to simulate the energy consumption of Luo’s house in its unrestored situation, in the preliminary design scheme (without energy-saving measures), and in the thermal environment transformation design scheme. PKPM is an energy-saving design software developed according to China’s national and local energy-saving standards, the energy consumption calculation is based on DOE-2 software (Chen Citation2008). The main structural and annual total heating and cooling energy consumptions per unit area, under the three working conditions, are shown in . According to the design requirements of public buildings, the total energy consumption per unit area for cooling and heating was 121.02 (kWh/m2·a) for the building’s unrestored situation and 156.10 (kWh/m2·a) for the preliminary design plan, which was higher than energy consumption before the transformation. This is because the preliminary design scheme restored the original style of the traditional houses in the Jiangnan region, increased the areas of the landscape windows in the south, west, and east courtyard walls, and greatly increased the envelope’s window-wall ratio. The annual total energy consumption per unit area of cooling and heating simulated in the thermal environment transformation design scheme was 103.72 (kWh/m2·a), which was 14% lower than the unrestored situation and 34% lower than the preliminary design scheme. Thus, the energy-saving effect was significant.
Table 2. The main structure and annual energy consumption per unit area
4. Conclusion
With economic and social development, alongside the improvement of people’s living standards, the low thermal comfort and high energy consumption of traditional houses cannot meet the needs of modern society. It is an inevitable trend for the sustainable development of traditional houses to carry out green, energy-saving transformations, reduce building energy consumption, and improve building comfort.
This study selected Luo’s house, a typical, traditional house in the Jiangnan region, as the research object. Through measuring the indoor thermal environment and evaluating thermal comfort, the researchers found that the indoor temperature of the building in winter was almost the same as that outdoors and that the average PMV value was −1.563. The thermal comfort was far lower than the current national standard of −0.75–0.75, and the indoor thermal comfort of the second floor was even lower than that outdoors, at only −2.071. In summer, the indoor thermal comfort basically met the national standard at night, but it could not meet requirements during the day. The thermal comfort of the second floor was, again, lower than that of the first floor, and the average PMV value was 1.41. In view of the abovementioned thermal environment problems, and based on the premises of protection, this research put forwards a thermal environment transformation plan for four aspects of the structure: natural ventilation, thermal insulation, component sunshade, and site environment. Due to the large sloping roofs of traditional houses in the Jiangnan region, the thermal insulation of the roof was improved. Measuring the post-transformation indoor thermal environment, the researchers found that the thermal comfort of the building was greatly improved in late summer and early autumn. The average PMV value in the room on the first floor was about −0.7, and the average PMV value in the room on the second floor was about −0.4. The daytime indoor thermal comfort thus met national standards, but some rooms required heating equipment at night to improve comfort levels. Energy consumption simulation software was also used to simulate the building’s energy consumption both before and after transformation. These simulations found that the annual total energy consumption of cooling and heating per unit area in the thermal environment transformation design scheme was 103.72 (kWh/m2·a), which reduced the energy consumption of the unrestored situation by 14% – a significant energy-saving effect.
The green and energy-saving transformation of traditional houses based on the premises of protection can not only reduce energy consumption but also improve the buildings’ comfort levels. Such transformations adapt to the new demands of modern society, while extending the life of traditional architecture, which is the inevitable trend of sustainable development for traditional houses. The authors hope this research can provide ideas for protecting and developing traditional houses in Hangzhou and throughout the Jiangnan region, while providing a reference for future green and energy-saving reform technology research to aid the sustainable development of traditional houses.
Acknowledgments
The study has been supported by the China National Key R&D Program “Research on the energy efficiency and health performance improvement of building operations based on lifecycle carbon emissions reduction (Grant No. 2018YFE0106100)“ and the Zhejiang Construction Scientific Research Program “Research on green energy-saving transformation and thermal environment improvement technology of historic buildings in Zhejiang Province (2019K036)“.
Disclosure statement
The authors declared no potential conflicts of interest to the research, authorship, or publication of this article.
Additional information
Funding
Notes on contributors
Xiaoyu Luo
Xiaoyu Luo, Ph.D., College of Civil Engineering and Architecture, Zhejiang University, Hangzhou, China.
Jiapan Lu
Jiapan Lu, College of Civil Engineering and Architecture, Zhejiang University, Hangzhou, China.
Jian Ge
Jian Ge, Professor, Ph.D., College of Civil Engineering and Architecture, Zhejiang University, Hangzhou, China.
References
- Alev, Ü., L. Eskola, E. Arumägi, J. Jokisalo, A. Donarelli, K. Siren, T. Broström, and T. Kalamees. 2014. “Renovation Alternatives to Improve Energy Performance of Historic Rural Houses in the Baltic Sea Region.” Energy and Buildings 77: 58–66. doi:https://doi.org/10.1016/j.enbuild.2014.03.049.
- Alongi, A., R. Scoccia, M. Motta, and L. Mazzarella. 2015. “Numerical Investigation of the Castle of Zena Energy Needs and a Feasibility Study for the Implementation of Electric and Gas Driven Heat Pump.” Energy and Buildings 95: 32–38. doi:https://doi.org/10.1016/j.enbuild.2014.11.012.
- Ascione, F., F. D. Rossi, and G. P. Vanoli. 2011. “Energy Retrofit of Historical Buildings: Theoretical and Experimental Investigations for the Modelling of Reliable Performance Scenarios.” Energy and Buildings 43 (8): 1925–1936. doi:https://doi.org/10.1016/j.enbuild.2011.03.040.
- Ascione, F., N. Bianco, R. F. De Masi, F. de’Rossi, and G. P. Vanoli. 2015. “Energy Retrofit of an Educational Building in the Ancient Center of Benevento. Feasibility Study of Energy Savings and Respect of the Historical Value.” Energy and Buildings 95: 172–183. doi:https://doi.org/10.1016/j.enbuild.2014.10.072.
- Bianco, L., V. Serra, S. Fantucci, M. Dutto, and M. Massolino. 2015. “Thermal Insulating Plaster as a Solution for Refurbishing Historic Building Envelopes: First Experimental Results.” Energy and Buildings 95: 86–91. doi:https://doi.org/10.1016/j.enbuild.2014.11.016.
- Cardinale, N., G. Rospi, and P. Stefanizzi. 2013. “Energy and Microclimatic Performance of Mediterranean Vernacular Buildings: The Sassi District of Matera and the Trulli District of Alberobello.” Building and Environment 59: 590–598. doi:https://doi.org/10.1016/j.buildenv.2012.10.006.
- Cardinale, N., G. Rospi, P. Stefanizzi, and V. Augenti. 2011. “Thermal Properties of the Vernacular Buildings Envelopes: The Case of The” Sassi Di Matera” And” Trulli Di Alberobello.” International Journal of Energy and Environment 2 (4): 605–614. doi:https://doi.org/10.1016/j.buildenv.2012.10.006.
- Chen, J. 2008. “Thermal Environment and Energy Efficiency Research of Atrium.” MA diss., Zhejiang University.
- European Parliament and the Council. 2012. Directive 2012/27/EU.
- Frey, P., R. Harris, M. Huppert, K. Spataro, J. F. McLennan, J. Heller, and M. Heater. 2012. Saving windows, saving money: Evaluating the energy performance of window retrofit and replacement. Project Report. National Trust for Historic Preservation (NTHP) / Preservation Green Lab.
- Galatioto, A., R. Ricciu, T. Salem, and E. Kinab. 2019. “Energy and Economic Analysis on Retrofit Actions for Italian Public Historic Buildings.” Energy 176: 58–66. doi:https://doi.org/10.1016/j.energy.2019.03.167.
- Geva, A. 1998. “Energy Simulation of Historic Buildings: St. Louis Catholic Church, Castroville, Texas.” Association for Preservation Technology International Bulletin 29 (1): 36–41.
- Heath, N., and P. Baker. 2013. Historic Scotland Technical Paper 20: Slim-profile double-glazing in listed buildings-Re-measuring the thermal performance. Historic Scotland.
- International Energy Agency (IEA). 2014. Key World Energy Statistics. International Energy Agency: Paris, France.
- Li, Q., R. You, C. Chen, and X. Yang. 2013. “A Field Investigation and Comparative Study of Indoor Environmental Quality in Heritage Chinese Rural Buildings with Thick Rammed Earth Wall.” Energy and Buildings 62: 286–293. doi:https://doi.org/10.1016/j.enbuild.2013.02.057.
- Liang, S. 2011. History of Chinese Architecture. 1st ed. China: Joint Publishing.
- Martínez-Molina, A., I. Tort-Ausina, S. Cho, and J. Vivancos. 2016. “Energy Efficiency and Thermal Comfort in Historic Buildings: A Review.” Renewable and Sustainable Energy Reviews 61: 70–85. doi:https://doi.org/10.1016/j.rser.2016.03.018.
- Schibuola, L., M. Scarpa, and C. Tambani. 2018. “Innovative Technologies for Energy Retrofit of Historic Buildings: An Experimental Validation.” Journal of Cultural Heritage 30: 147–154. doi:https://doi.org/10.1016/j.culher.2017.09.011.
- Sesana, M. M., and G. Salvalai. 2018. “A Review on Building Renovation Passport: Potentialities and Barriers on Current Initiatives.” Energy and Buildings 173: 195–205. doi:https://doi.org/10.1016/j.enbuild.2018.05.027.
- Walker, R., and S. Pavía. 2015. “Thermal Performance of a Selection of Insulation Materials Suitable for Historic Buildings.” Building and Environment 94: 155–165. doi:https://doi.org/10.1016/j.buildenv.2015.07.033.
- Wang, Z., Q. Wei, and Y. He. 2004. “From Original to Sustainable development—Regional Architectural Analysis and Construction of Green Loess Plateau.” Architectural Journal 3: 34–37.
- Webb, A. L. 2017. “Energy Retrofits in Historic and Traditional Buildings: A Review of Problems and Methods.” Renewable and Sustainable Energy Reviews 77: 748–759. doi:https://doi.org/10.1016/j.rser.2017.01.145.