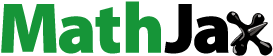
ABSTRACT
To investigate the mechanical properties of the eccentrically loaded steel reinforced recycled concrete filled square steel tube columns, 14 specimens of the columns were designed and tested. The failure modes, load-displacement curves, bearing capacity and deformation of specimens were obtained and analyzed in detail. In addition, the numerical model of columns considering the unfavorable effects of recycled coarse aggregate (RCA) and the restraint effect of square steel tube were also established by ABAQUS software. The results show that the deflection deformation on the mid-span section of columns was obvious and the failure mode of compression-bending occurred to the eccentrically loaded columns. The replacement percentage of RCA has some adverse effect on the eccentric compression performance of columns. Compared with ordinary concrete columns, the eccentric bearing capacity of columns fully using RCA is reduced by 9.53%. As the increasing eccentricity and slenderness ratio, the maximum reduction on the eccentric bearing capacity of columns was 46.14% and 25.86%, respectively. In addition, the increasing strength of RAC or steel effectively improves the eccentric bearing capacity of columns, but the ductile deformation of columns decreased gradually. Based on the above analysis, a modified calculation formula on the eccentric bearing capacity of columns was proposed.
1. Introduction
With the rapid improvement of social-economic level in recent decades, the concrete industry in the field of construction shows a high-speed development trend in the world. Meanwhile, a large number of old buildings need to be expanded or rebuilt, so as to meet the people’s living requirements. Unfortunately, as a result of the development of these large-scale construction activities, a large amount of construction waste (especially waste concrete) has been produced, which has caused serious pollution to the ecological environment. Therefore, how to deal with and utilize these large amounts of waste concrete reasonably and effectively has become one of the problems worldwide. Comfortingly, the application technologies of RAC provides an effective way to solve a large number of waste concrete (Xiao et al. Citation2012; Tam, Soomro, and Evangelista Citation2018; Tam et al. Citation2020; Isabel, Pablo, and Mirian Citation2020), which can consume a great quantity of RCA formed after the crushing of waste concrete. The difference between RAC and ordinary concrete is that the RCA adopting in RAC, which is an important factor affecting the mechanical properties of RAC. In fact, many scholars in the world had done lots of researches on the basic mechanical properties of RAC material (FAl et al. Citation2020; Bai et al. Citation2020; Adessina et al. Citation2019; Berredjem, Arabi, and Molez Citation2020), such as compression strength, tension strength, flexure strength, stress–strain relationship, elastic modulus and so on. These researches show that the basic mechanical properties of RAC are inferior to those of ordinary concrete in most cases, due to the defects and deficiencies of RCA. Therefore, how to enhance the mechanical properties of RAC and its structure is one of the hot spots in this research field.
In view of the advantages of composite structure, some scholars had replaced ordinary concrete with RAC in steel and concrete composite structures (Zhang and Gao Citation2019; Nour and Güneyisi Citation2019; Hongying et al. Citation2021; Jie et al. Citation2020; Liu et al. Citation2021), which can makes up for the shortage on the mechanical properties of RAC through the restraint action of steel tube or profile steel, and provides a new way to deal with the waste concrete effectively. It is also in line with the strategy of sustainable development and has important social and economic significance (Ma et al. Citation2016; Yang, Zhang, and Fu Citation2015). In particular, steel-reinforced recycled concrete (SRRC)-filled steel tube columns is a kind of structural form of steel and RAC composite members. The research literatures (Ma et al. Citation2018; Yu et al. Citation2019a) pointed out that SRRC-filled steel tube columns not only had the advantages of high strength, stiffness, and good deformation capacity, but also had the convenience of simple construction process and saving formwork. The restraint effect is jointly provided by the external steel tube and built-in profile steel, and the compressive strength of RAC in the columns is significantly improved, which effectively improves the bearing capacity and ductility of structural members, and is very beneficial to the popularization and application of RAC materials.
The authors’ research group (Ma et al. Citation2019) had done some experimental researches on the failure mechanism and ultimate bearing capacity of SRRC-filled square steel tube columns under axial compression loads. Especially, based on the unified strength theory, the calculation formulas on the nominal axial bearing capacity of SRRC-filled square steel tube columns was proposed. However, in the practical engineering, the ideal state of axially compressed columns are almost nonexistent due to the various accidental factors, such as the design defects, construction errors, and load action, which leads that the column has a certain eccentricity and bears the combined action of bending moments and vertical loads. Therefore, it is of great significance to investigate the mechanical properties of the eccentrically loaded columns. Up to now, the nonlinear analysis on the eccentric compression behaviors of SRRC-filled steel tube columns has hardly been reported in literatures. Therefore, it is necessary to carry out relevant research to provide the corresponding reference basis for the engineering application of SRRC-filled steel tube columns. In addition, some scholars had carried out a series of nonlinear analysis on the axial compression behavior of SRRC columns and RAC-filled steel tube columns. For example, the nonlinear numerical analysis on the mechanical behavior of SRRC columns under horizontal loads was carried out and the validity of calculated results were verified compare with the test results (Ma et al. Citation2016). Therefore, the numerical simulation method can provide a means for the nonlinear analysis on the mechanical performances of steel and RAC composite structural members adopting the reasonable constitutive relationship and failure criterion model of material.
In this study, on the basis of eccentric compression tests and numerical simulation of SRRC filled square steel tube columns, the mechanical properties such as the failure patterns, load-displacement curves, load values, deformation, and stress characteristics of columns were investigated in detail, as well as the simulation results were compared with the test results. The influence levels of design parameters on the mechanical properties of eccentrically loaded columns was also performed and further analyzed. Based on the superposition principle, the modified formulas on the nominal eccentric bearing capacity of columns were put forward. The results can provide some references for the application of SRRC-filled square steel tube columns.
2. Eccentric compression tests of columns
2.1. Design of specimens
14 specimens of SRRC-filled square steel tube columns were manufactured and tested under the strong-axis monotonic eccentric compression loading. In this study, the eccentricity, slenderness ratio, replacement percentage of RCA, width-to-thickness ratio of square steel tube, profile steel ratio, and RAC strength were taken as the main design parameters in the test specimns. describes the geometric sizes and cross-sectional shapes on the specimens of eccentrically loaded columns. shows the main design parameters on the specimens of eccentrically loaded columns. The RCA used in the specimens was manually crushed from the demolished building, and its basic mechanical properties met the use requirement of Class II RCA according to the Chinese standards of “Recycled coarse aggregate for concrete” (GB/T-25177-Citation2010 Citation2010). The mixture proportion design and basic mechanical properties of RAC are shown in . In addition, the strength grade of Q235 carbon steel was adopted to profile steel and steel tube in the specimens. In particular, the steel tubes used in the specimens were the butt welded square steel tubes and the section form of profile steel was I-shaped steel. The steel flange and steel web was made of the thickness of steel plate with 8 mm and 6 mm, respectively. To prevent the local buckling damage at the end of square steel tube, a square reinforcing ring with a height of 50 mm was welded around the two ends of square steel tube. shows the basic mechanical properties of steel materials adopted in the columns.
Table 1. Design parameters of the specimens.
Table 2. Basic mechanical properties of RAC material.
Table 3. Mechanical performance indexes of steel products in the specimens.
2.2. Test loading devices and measuring contents
The eccentric compression tests of specimnes was carried out by electro-hydraulic servo 500 t press machine, and the eccentric loads was applied by installing two sets of eccentric knife hinges with the adjustable positions at both ends of columns. Before the test, the thickness of cement mortar with 15 mm was applied to the top and bottom of specimens for leveling to prevent the local crushing and ensure the uniform force transmission of columns during the loading process. shows the test loading devices and loading photos on the eccentric compression tests of columns. The loading methods of specimens were the load-displacement mixed control by a computer, which was as follows: firstly, the specimens were preloaded with 50 kN, so as to the space gap between the specimens and test loading devices was eliminated as much as possible. The next step was to unload zero, and then the specimens were subjected to the graded monotonic loading with Pmax/15 as the load increment (Pmax was the estimated peak load). After reaching approximately 0.7 Pmax, the loading program of specimens was changed to the displacement controlled loading, and the loading rate was 1.0 mm/min until the load-displacement curves of specimen droped stably or it was not suitable to continue to bear the external loads. At this case, the eccentric compression tests of specimens can be terminated.
The load-displacement curves of specimens could be obtained through the automatic collection of electro-hydraulic servo testing machine system controlled by computer. In addition, the lateral deflection of specimens in the process of eccentric compression loading could be measured by evenly arranging three displacement meters on the tensile sides of specimens. Several strain gauges were also arranged at the appropriate positions of square steel tubes and profile steel in the columns to measure the development of vertical strains and transverse strains.
2.3. Failure process and modes of the columns
By observing the process of eccentric compression tests on the eccentrically loaded columns, the failure process and failure characteristics of all the specimens of SECS-1~SECS-14 was basically similar. In this study, several typical specimens of the eccentrically loaded columns were selected to describe the failure process, mainly as follows.
There was no obvious phenomenon in the initial stage of loading, and the lateral deflection deformation changed linearly with the increase of loads. When the loads increased to about 60% of the peak loads, a slight local bulge deformation occurred in the middle upper part of square steel tube, and the upper part of square steel tube had begun to yield. When the loads increased to about 90% of the peak loads, the bulge deformation began to appear in the middle of specimens. And when the loads increased to the peak loads, the bulging in the middle of specimens intensified and extended to both sides. It can be clearly seen that the specimens began to bend to the compression area. After the peak loads, the eccentric bearing capacity of specimens decreased and the lateral deflection developed relatively rapidly. After the test loading, cutting the square steel tube can clearly see that the bending direction inside the specimens was consistent with the overall bending direction of the specimens. The RAC at the corresponding part of the square steel tube with the local bulging was crushed, and several transverse cracks appeared on the surface of RAC in the middle of columns. After stripping the RAC from profile steel, it was found that the deformation of profile steel was consistent with the overall deformation of specimens. In addition, when the eccentricity of columns was greater, the bending degree of profile steel was greater, basically showing the failure phenomenon of symmetrical bending at the upper and lower ends.
The final failure patterns of the eccentrically loaded SRRC-filled square steel tube columns are described in . In general, the failure patterns of the eccentrically loaded columns can be summarized as a compression bending failure subjected to the eccentric compression loads. The main failure characteristics of eccentrically loaded columns can be described as the profile steel first yielded, then internal RAC was crushed, and finally the square steel tube yielded under eccentric compression loading. Moreover, the external square steel tube has a good restraining effect binding force on the internal RAC. In addition, square steel tube and profile steel also effectively delayed the cracking of RAC and improved the stiffness and bearing capacity of eccentrically loaded columns.
2.4. Load-displacement curves of columns
Obviously, it can be seen from the section 2.3 that the specimens had experienced the elastic stage, plastic development stage, and decline stage in the whole loading process. The load-displacement curves of test design parameters on the eccentric compression behavior of columns are shown in .
(1) (a) shows the influence levels of different replacement percentages of RCA on the mechanical properties of eccentrically loaded columns. According to (a), all the specimens of columns are in the elastic stage at the initial stage of loading. When the loads increases to about 80% of the peak loads, the specimens enter the elastic-–plastic stage, and external square steel tube and internal profile steel of columns have yielded gradually, as well as the stiffness of columns also begins to decrease. Especially, with the increase of replacement percentage of RCA, the bearing capacity and stiffness of each specimen gradually decrease, with the maximum decrease of 9.53%. After peak loads, the displacement increases rapidly, and the bearing capacity of specimens begins to decrease, but the falling section of curves is relatively flat, indicating that the square steel tube and profile steel play a good restrictive role on the internal RAC, and the eccentrically loaded columns also have a good deformation capacity.
(2) The effect of different profile steel ratio on the mechanical properties of eccentrically loaded columns is described in (b). According to the curves in (b), the load-displacement relationship of all specimens basically increases linearly at the initial stage of loading. When the load increases to about 70% of the peak load, the specimen enters the elastic-plastic stage. The greater the profile steel ratio, the greater the bearing capacity and stiffness of the eccentrically loaded columns. Among them, the maximum increase of profile steel ratio on the eccentric bearing capacity of columns is 9.75%. On the premise of the same cross-sectional area of the eccentric compression columns, increasing the profile steel ratio can increase the section moment of inertia of profile steel, so as to improve the flexural stiffness of eccentrically loaded columns. After the peak loads, the eccentric bearing capacity of columns begins to decrease gradually. The larger the profile steel ratio is, the gentler the falling section of curves is. Therefore, properly increasing the profile steel ratio can improve the mechanical behaviors of eccentrically loaded columns.
(3) (c) shows the effect of width-to-thickness ratio of square steel tube on the mechanical properties of eccentrically loaded columns. Based on (c), the eccentric bearing capacity of columns increases with the increasing the wall thickness of square steel tube. It is mainly because the restraint effect of square steel tubes on the internal RAC gradually increases with the increase of wall thickness of steel tube. The maximum decrease of width-to-thickness ratio on the eccentrically loaded bearing capacity is 17.31%. After the peak loads, the eccentric bearing capacity of specimens begins to decrease gradually. The smaller the width-to-thickness ratio of specimens, the more gentle the falling section of curves, and the better the deformation of specimens. Therefore, the reasonably increasing wall thickness of square steel tubes is beneficial to enhance the eccentric bearing capacity and deformation of columns.
(4) Based on (d), it can be seen from that the eccentricity has an obvious impact on the mechanical performance of eccentrically loaded columns. The larger the eccentricity, the lower the bearing capacity of the specimens, and the maximum decrease is 46.14%. When the vertical loads drops to about 80% of the peak loads, the bearing capacity of columns begins to decline slowly and shows certain ductility. In addition, the eccentricity of SECS-8 specimen is zero, that is, it is an axially compressed column in the test and its bearing capacity and stiffness are significantly higher than those of the eccentrically loaded columns. Therefore, the eccentricity of columns should be reduced as much as possible to improve the mechanical performance of columns in the practical engineering.
(5) (e) shows the effect of different RAC strength on the mechanical performance of eccentrically loaded columns. It can be seen from (e) that the eccentric bearing capacity of specimen increases slightly with the increasing RAC strength, with a maximum increase of 5.35%. After the peak load, it is observed that the higher the RAC strength, the more obvious the downward trend of curves. The reason may be that the strength of RAC increases, the brittleness of RAC increases, and RAC is entirely crushed under eccentric compression loads, resulting in a faster decline rate of bearing capacity of columns.
(6) The effect characteristics of slenderness ratio on the mechanical performance of eccentrically loaded columns are described in (f). It can be seen from (f) that the eccentric bearing capacity and stiffness of specimens decrease with the increase of slenderness ratio, and the maximum decrease of bearing capacity is 25.86%. After the peak loads, the larger the slenderness ratio is, the faster the eccentric bearing capacity of columns decreases. It may be because the second-order effect has a significant effect on the specimens with a large slenderness ratio under eccentric compression loads. Therefore, the slenderness ratio of the eccentrically loaded columns should be reasonably controlled to avoid the stability of columns.
In a word, the bearing capacity and ductility of eccentrically loaded columns decreases with the increase of slenderness ratio, width-to-thickness ratio, eccentricity and replacement percentage of RCA respectively. In addition, the slenderness ratio and eccentricity have a significant impact on the mechanical performance of eccentrically loaded columns. The maximum decreases of slenderness ratio, width-to-thickness ratio of steel tube, eccentricity and replacement percentage of RCA on the eccentric bearing capacity of columns are 25.86%, 17.31%, 46.14%, and 9.53% respectively. The maximum increases of RAC strength and profile steel ratio on the eccentric bearing capacity are 5.35% and 9.75%. respectively. In general, this kind of eccentrically loaded column has high bearing capacity and good deformation capacity.
3. Finite element models of the eccentrically loaded columns
3.1. Constitutive relationship of steel material
In this study, square steel tube and profile steel of eccentrically loaded columns were made of Q235 carbon steel with the obvious yield strength. Therefore, in the process of numerical modeling, the steel material used in the eccentrically loaded columns can be assumed to obey the Von Mises yield criterion. The equation expression on the constitutive model of steel material can be shown in EquationEq. (1)(1)
(1) (Zhong Citation2003).
In the above formula, σs is the equivalent stress of steel material; Es is the elastic modulus of steel material and εs is the equivalent strain of steel material; εe, εe1, εe2, and εe3 are the different values on the equivalent strains of steel material, respectively.
In addition, in the above symbols of EquationEq. (1)(1)
(1) :
The relevant parameters could be obtained from the properties test of steel products in the specimens.
3.2. Constitutive relationship of RAC material
In fact, the eccentrically loaded column had both the tension zone and compression zone under the combined action of vertical load and bending moment, and internal RAC in the columns was obviously restrained by square steel tube. To make the simulation process of columns accord with the actual situations, the constitutive model of RAC in the numerical model included the stress-strain relationship of compression and tension state, respectively. And the restraint effect of square steel tube on RAC was also considered in this simulation, as shown in EquationEq. (11)(11)
(11) .
(1) Compressive stress–strain relation of RAC
For the compressive stress-strain relation of RAC confined by the square steel tube, Yang (Yang, Zhang, and Fu Citation2015) had further revised the formulas of stress–strain relation proposed by Han (Han, Yao, and Tao Citation2007), which included the adverse influence of RCA replacement percentage, and proposed a revised formula on the equivalent uniaxial stress–strain relationship of RAC confined by square steel tube in the column, as shown in EquationEq. (2(2)
(2) ) ~ (11).
In the above formulas, A and B are the parameters related to the constraint effect coefficient. σ and σ0 are the stress value and peak stress of RAC, respectively; fck is the standard compressive strength of RAC; ζ is the constraint effect coefficient of square steel tube (i.e. ζ = As fy/Acfck); As and Ac are the section area of profile steel and RAC, respectively; fy is the yield strength of square steel tube; and θ is the coefficient related to the adverse effect of RCA replacement percentage; ɛc is the peak strain corresponding to the peak stress of RAC.
(2) Tensile stress–strain relation of RAC
Up to now, there were few literatures on the uniaxial tensile stress–strain of RAC material. Xiao (Xiao Citation2008) proposed the rising section of tensile stress–strain curve of RAC, but the mathematical equations on the falling section of the tensile curve of RAC had almost not been reported in the literatures. For all that, some researches show that the tensile failure characteristics of RAC were basically similar to that of ordinary concrete. Therefore, some scholars (Yang Citation2007; Wang and Xiao Citation2018; Luo et al. Citation2018) adopted a combination of the tensile rising section of RAC and the tensile falling section of ordinary concrete in the numerical simulation, and the computed results were satisfactory. In view of this, the tensile stress–strain relationship of RAC in the columns was composed of two parts in this study, of which the rising section of tensile stress–strain model adopted the tensile relation of RAC proposed by Xiao (Xiao Citation2008), and the falling section of tensile stress–strain model could be given with reference to ordinary concrete (GB50010-Citation2010 Citation2010). On this basis, combined with the mechanical properties of RAC, and modifying the relevant parameters of tensile model, the tensile stress–strain equations of RAC were obtained as follows:
In EquationEq. (12)(12)
(12) , x = ε/εtr, y = σ/ftr, εtr is the tensile peak strain of RAC, ftr is the axial tensile strength of RAC, c is the initial elastic modulus of RAC, d is the control parameter on the descending section of uniaxial tensile stres–strain curve of RAC, and usually takes 0.3.
In addition, the damage effect and failure criterion of RAC in this simulation had also been considered when establishing the stress–strain constitutive model of RAC. The calculated formulas on the compressive and tensile damage factors of RAC can be referred to the literatures (Guo and Wang Citation1991; Yu et al. Citation2019b), and the failure criteria of RAC were also given in references (Hibbit K Citation2007; He and Zhang Citation2014).
3.3. Element types and mesh generation
In the numerical model of eccentrically loaded columns, square steel tube adopted the shell element of S4R, and RAC, profile steel, stiffener as well as the steel end plates adopted the C3D8R element. The mesh sizes on the finite element model of columns can be as follows: the element size of square steel tube, RAC and profile steel were all 20 mm. Besides, the element size of stiffener and the steel end plates were 30 mm in the columns. describes the mesh generation on the each part of eccentrically loaded columns.
3.4. Material interaction and loading program
In the eccentric compression process of columns, the various components in the columns will deform differently, so the interaction between the interfaces of various components must be considered in this nonlinear analysis. In order to facilitate the analysis, the interaction between profile steel and core RAC adopted the binding constraint. While the bond-slip between RAC and steel tubes were mainly considered in this study. The surface-to-surface contact in ABAQUS software was selected for normal contact among the square steel tube and RAC, and the bond-slip was also considered in the tangential direction, which was suitable for Coulomb friction model (Balaty and Gjelsyilk Citation1990). Obviously, when the shear stress transferred by the interface exceeds the critical value τcrit, the relative sliding will occur between the interaction interfaces. During the sliding process, the shear stress of interface maintains at the critical value τcrit. Therefore, the equation expression of the relationship between the critical shear stress τcrit and normal pressure P of the interface can be as follows:
Where τbond is the average interfacial bonding force, D is the external diameter of square steel tube; t is the wall thickness of steel tube; μ is the friction coefficient, which was between 0.2 and 0.6 (Yu, Cheng, and Xu Citation2015). Referring to the literature (GB 50936-Citation2014 Citation2014) and combining with the trial calculations, the value μ in this model could be taken as 0.6.
By the way, the eccentrically loaded columns in an ideal state is calculated and analyzed in the simulation process. In order to make the simulation closer to the test situations, the eccentricity of columns under eccentric compression loads was added to the Y axis in this model. Therefore, the degree freedom of UR1 was fixed at the upper and lower ends of eccentrically loaded columns to ensure that the columns can only rotate around the X axis. And the vertical loads were applied at the upper end of eccentric compression columns along the Z axis. To facilitate the extraction of calculation results and prevent the local damage caused by the stress concentration of columns, the action point of displacement was coupled to the reference points XRP1 and XRP2 in the numerical model of columns, as shown in .
4. Results on the numerical calculation of eccentrically loaded columns
4.1. Stress nephogram of columns
The numerical calculation on the finite element model of eccentrically loaded columns was carried out and the stress nephogram of columns was obtained. ~ show the stress nephogram on the some typical specimens.
In and , the specimens of SECS-3 and SECS-7 were the typical short columns. At the initial stage of loading, the deformation and stress of each component in the columns increased linearly with the increase of vertical loads. With the increasing loads, the deformation of column was smaller, but the growth rate of stress on the compression zone of square steel tube and profile steel in the middle of column was faster. When the vertical loads increased to about 80% of peak loads (i.e. 80%Pmax), the slight local buckling occurred in the compression zone of the middle of columns. At this time, the profile steel basically reached the yield state, which the yield stress was about 326 MPa. Meanwhile, the strain of each component in the columns varied nonlinearly with the increase of loads. When the loads increased to about 90% Pmax, the expansion deformation in the middle of columns became more significant. The stress distribution of square steel tube mainly changed from the two ends of to the position of the compression zone in the middle of columns. Meanwhile, the cross section of the compression zone of square steel tube basically reached yield and its stress was about 304 MPa. In addition, Core RAC in the columns had been crushed seriously, which led to the expansion of compression zone in the middle of columns to increase. After peak loads, the bearing capacity and stiffness of eccentrically loaded column decreased gradually, while its lateral bending deformation increases obviously. Finally, the eccentrically loaded column was damaged due to the compression yield of profile steel, local buckling of steel tube, and crushing of RAC.
In , the specimen of SECS-14 was the typical long columns. It had the similar failure process and mode compared with the short columns, but the lateral bending deformation of long columns is more obvious under the combined action of compression and bending. The yield stresses of profile steel and steel tube in the specimen of SECS-14 were about 325 MPa and 298 MPa, respectively. Compared with the short columns, the difference was that the specimen of SECS-14 had a large slenderness ratio, which was obviously affected by the second-order effect under eccentric compression loads. When the same vertical loads were applied, the lateral deflection of SECS-14 developed more rapidly than that of the short columns. It caused that the bending phenomenon of SECS-14 was more obvious, and the stress concentration areas were mainly in the middle of compression zone and tension zone. When the loads increased to about 90% of peak load, the internal RAC in the column was collapsed at the corresponding part of square steel tube. At this time, the stress of internal RAC reached about 42.1 MPa. Subsequently, the eccentric bearing capacity of column gradually decreased after reaching peak loads until the column was damaged.
4.2. Comparison between the calculation curves and test curves of columns
To further verify the rationality on the finite element model of eccentrically loaded columns, the calculation load-displacement curves and load characteristic values of eccentrically loaded columns were obtained, which were used to compare with the test results. shows the comparison on the numerical curves and test curves of eccentrically loaded columns.
Figure 10. Comparison between the test curves and calculation curves of eccentrically loaded columns.
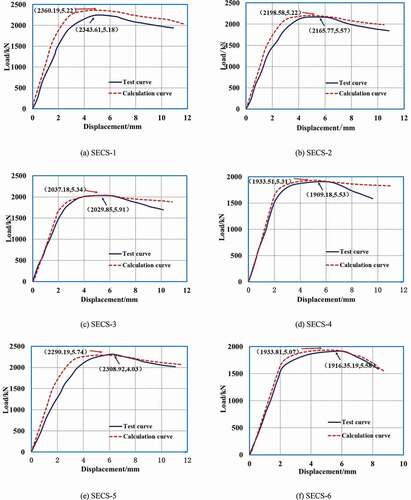
As can be seen from , the calculated curves of eccentrically loaded columns was in good agreement with the test curves in the elastic working stage, and the overall trend of the curves was basically similar. also lists the comparison between the calculated values and test values of eccentrically loaded columns. From , it can be seen that the maximum error between the calculated peak values and the experimental peak values of specimens were approach to 9.9%. In general, the relative error on the numerical calculation of columns is relatively small. At the same time, the secant stiffness on the calculated curves of some specimens is larger than that of the test curves, which is due to the fact that the eccentrically loaded columns are in a relatively ideal state during the finite element simulation, resulting in the less damage to the eccentrically loaded columns than in the test case. In addition, the peak loads on the numerical computation of the eccentrically loaded columns was generally larger than the test values, which may be due to the following reasons: (1) when ABAQUS software was used for the modeling, the initial defects such as the nonuniformity and damage or initial cracks inside RAC materials were not fully considered; (2) the simulation loading process was divided into several loading steps to carry out the monotonic loading, while the actual test was the continuous loading action with a certain damage accumulation. As a whole, the calculation results verified the rationality on the numerical model of eccentrically loaded columns to a certain extent.
Table 4. Comparison between the numerical values and test values of eccentrically loaded columns.
5. Calculation formulas on nominal eccentric bearing capacity of columns
Obviously, the eccentric bearing capacity of SRRC-filled square steel tube columns was provided by the square steel tube, profile steel, and internal RAC. Based on the superposition principles, and referring to the Chinese’s technical code for concrete-filled steel tube structure (GB 50936-Citation2014 Citation2014; CECS 28:Citation2012 Citation2012), this study put forward the modified calculation formulas on the eccentric bearing capacity of columns which consider the constraint effect of square steel tube and the contribution of profile steel. Especially, the adverse effect coefficients of replacement percentage of RCA, slenderness ratio, eccentricity on the eccentric bearing capacity of columns was also regressed by the test data to obtain the relevant reduction formulas. The calculation formulas on the eccentric bearing capacity of SRRC-filled square steel tube columns were as follows;
When the cross section of columns is rectangular:
In the above formulas, Nu is the eccentric bearing capacity of columns; N0 is the axial bearing capacity of columns; φr is the reduction coefficient of replacement percentage of RCA;φl is the reduction coefficient of slenderness ratio;φe is the reduction coefficient of the eccentricity; Asc is the cross-sectional area of columns; fsc is the design values of compressive strength of columns; B and C are the influence coefficients of cross-sectional shape on the confinement effect; θ is the hoop coefficient of square steel tube; fs is the design values of steel yield strength; fc is the design values on the compressive strength of RAC; αsc is the steel ratio of columns; As is the sum of the cross-sectional area of square steel tube and profile steel; and Ac is the sectional area of RAC.
To make EquationEq. (15)(15)
(15) reliable and extensive, this study makes the detailed statistics on the test data by the consulting relevant literature (Jie et al. Citation2020; Zeng et al. Citation2018; Liu et al. Citation2020), the influence levels on the replacement percentage of RCA, eccentricity, and slenderness ratio on the eccentric bearing capacity of columns was analyzed by the regression analysis. The reduction formulas on the replacement percentage of RCA, slenderness ratio, and eccentricity on the eccentric bearing capacity of columns were finally obtained as follows:
In the formulas, r is the replacement percentage of RCA; λ is the slenderness ratio of columns; e is the eccentricity of columns.
The comparison between the thecalculation and experimental results of eccentric bearing capacity of columns was shown in . It was found that the average value on the ratio of the calculation values to the test values was 1.08, and the standard deviation was 0.114. The comparison results show that the calculation results are in good agreement with the experimental results and meet the requirements of calculation accuracy, which can provide a calculation method for the nominal eccentric bearing capacity of SRRC-filled square steel tube columns.
Table 5. Comparison of calculation results and test results on the eccentric bearing capacity of columns.
6. Conclusions
The eccentric compression behaviors of SRRC-filled square steel tube columns were investigated in detail by experimental tests and numerical simulation in this study. The main conclusions can be summarized as follows:
(1) The columns show a typical bending failure pattern under eccentric compression loading, in which the bulging deformation was obvious in the middle compression area of columns. When the vertical loads reached 80% of the peak loads, the profile steel in the columns yielded first and the yield stress was about 326 MPa. When the vertical loads continued to increase to 90% of the peak loads, the square steel tubes of columns reached the yield state successively and the yield stress was about 304 MPa. Finally, core RAC in the middle compression area of columns was crushed seriously, which indicated that the columns were damaged.
(2) The numerical calculation results of columns were basically close to the test results, which verified the validity on the numerical model of eccentrically loaded columns. The calculated curves of columns overlapped with the test curves in the early stage of loading, but the calculated stiffness of some columns was larger than that of test values. With the increase of loads, the two curves began to deviate from the straight line. The difference of peak loads was not obvious, and the error between calculated values and test values of each column was within 10%. In addition, due to the fact that the initial defects of materials could not be fully considered during modeling process and the simulated column was in a relatively ideal state during loading, which resulted in the calculation values of peak loads being larger than the test values.
(3) The increasing replacement percentage of RCA was little disadvantageous to the eccentric bearing capacity of columns. Especially, compared with ordinary concrete columns, the eccentric bearing capacity of columns with the replacement percentage of RCA 100% decreased by 9.53%, and it had a little effect on the deformation capacity of columns. Besides, the bearing capacity of eccentrically loaded columns decreased obviously with the increasing eccentricity, slenderness ratio and the width-to-thickness ratio of steel tube, respectively, so the above parameters shall be should be reasonably controlled to avoid negative effects in the engineering application.
(4) The properly increasing RAC strength and profile steel ratio could effectively improve the eccentric bearing capacity of columns and the largest increases of bearing capacity were 5.35% and 9.75%, respectively. However, the deformation capacity of columns decreased gradually with the increase of strength of RAC. Conversely, the increasing profile steel ratio could improve the ductile deformation of eccentrically loaded columns.
(5) The modified calculation formulas on the nominal eccentric bearing capacity of SRRC-filled square steel tube column were proposed in this study. And the calculation values of bearing capacity were in good agreement with the test values, which verified the validity of the modified calculation formulas.
Acknowledgments
The research was financially supported by National Natural Science Foundation of China P.R. (No. 5140 8485), The Project Supported by Natural Science Basic Research Plan in Shaanxi Province of China (No. 2021JM-332 and 2021JM-333), which is gratefully acknowledged.
Disclosure statement
No potential conflict of interest was reported by the author(s).
References
- Adessina, A., A. B. Fraj, J. F. Barthélémy, C. Chateau, and D. Garnier. 2019. “Experimental and Micromechanical Investigation on the Mechanical and Durability Properties of Recycled Aggregates Concrete.” Cement and Concrete Research 126 (12): 105900. doi:10.1016/j.cemconres.2019.105900.
- Bai, G., C. Zhu, C. Liu, and B. Liu. 2020. “An Evaluation of the Recycled Aggregate Characteristics and the Recycled Aggregate Concrete Mechanical Properties.” Construction and Building Materials 240 (4): 117978. doi:10.1016/j.conbuildmat.2019.117978.
- Balaty, P., and A. Gjelsyilk. 1990. “Coefficient of Friction for Steel on Concrete at High Normal Stress.” Journal of Materials in Civil Engineering 2 (1): 46–49. doi:10.1061/(ASCE)0899-1561(1990)2:1(46).
- Berredjem, L., N. Arabi, and L. Molez. 2020. “Mechanical and Durability Properties of Concrete Based on Recycled Coarse and Fine Aggregates Produced from Demolished Concrete.” Construction and Building Materials 246 (6): 118421. doi:10.1016/j.conbuildmat.2020.118421.
- CECS 28:2012. 2012. Technical Specification for Concrete-filled Steel Tubular Structures. Beijing: China Planning Press. in Chinese.
- FAl, M., R. Boissiere, C. Mercier, and A. Khelil. 2020. “Shear Behavior of Reinforced Concrete Beams Made from Recycled Coarse and Fine Aggregates.” Structures 25 (6): 660–669. doi:10.1016/j.istruc.2020.03.015.
- GB 50936-2014. 2014. Technical Specification for Concrete-filled Steel Tubular Structures. Beijing: China Architecture and Building Press. (in Chinese).
- GB50010-2010. 2010. Code for Design of Concrete Structures. Beijing: China Architecture and Building Press. (in Chinese).
- GB/T-25177-2010. 2010. Recycled Coarse Aggregate for Concrete. Beijing: Standards Press of China. (in Chinese).
- Guo, Z., and C. Wang. 1991. “Investigation of Strength and Failure Criterion of Concrete under Multi-axial Stresses.” China Civil Engineering Journal 24 (3): 1–14. in Chinese.
- Han, L., G. Yao, and Z. Tao. 2007. “Performance of Concrete-filled Thin- Walled Steel Tubes under Pure Torsion.” Thin-Walled Structures 45 (1): 24–36. doi:10.1016/j.tws.2007.01.008.
- He, Z., and J. Zhang. 2014. “Strength Characteristics and Failure Criterion of Plain Recycled Aggregate Concrete under Triaxial Stress States.” Construction and Building Materials 54 (3): 354–362. doi:10.1016/j.conbuildmat.2013.12.075.
- Hibbit K, S. 2007. “ABAQUS/standard Analysis User’s Manual.”
- Hongying, D., L. Yanna, C. Wanlin, and G. Yanli. 2021. “Seismic Behavior of Full-scale Steel Reinforced Recycled Concrete Columns under High Axial Compression Ratio.” Structures 29 (2): 1882–1897. doi:10.1016/j.istruc.2020.12.037.
- Isabel, M. L., V. B. Pablo, and V. L. Mirian. 2020. “Sustainability Evaluation of Concretes with Mixed Recycled Aggregate Based on Holistic Approach: Technical, Economic and Environmental Analysis.” Waste Management 104 (3): 9–19. doi:10.1016/j.wasman.2019.12.044.
- Jie, C., Z. Sumei, W. Yuyin, and Y. Geng. 2020. “Axial Compressive Behavior of Recycled Concrete Filled Steel Tubular Stub Columns with the Inclusion of Crushed Brick.” Structures 26 (8): 271–283. doi:10.1016/j.istruc.2020.03.045.
- Liu, Z., Y. Lu, S. Li, and L. Xiao. 2021. “Enhanced Bond-slip Behavior between Recycled Aggregate Concrete and Steel Tubes under Repeated Loading.” Structures 33 (10): 1263–1282. doi:10.1016/j.istruc.2021.05.022.
- Liu, Z., Y. Lu, S. Li, and S. Yi. 2020. “Behavior of Steel Tube Columns Filled with Steel-fiber-reinforced Self-stressing Recycled Aggregate Concrete under Axial Compression.” Thin-Walled Structures 149 (4): 106521. doi:10.1016/j.tws.2019.106521.
- Luo, S., S. Ye, J. Xiao, J. Zheng, and Y. Zhu. 2018. “Carbonated Recycled Coarse Aggregate and Uniaxial Compressive Stress-strain Relation of Recycled Aggregate Concrete.” Construction and Building Materials 188 (11): 956–965. doi:10.1016/j.conbuildmat.2018.08.159.
- Ma, H., J. Dong, G. Hu, and Y. Liu. 2019. “Axial Compression Performance of Composite Short Columns Composed of RAC-filled Square Steel Tube and Profile Steel.” Journal of Constructional Steel Research 153 (2): 416–430. doi:10.1016/j.jcsr.2018.10.018.
- Ma, H., J. Dong, Y. Liu, and T. Guo. 2018. “Compressive Behaviour of Composite Columns Composed of RAC-filled Circular Steel Tube and Profile Steel under Axial Loading.” Journal of Constructional Steel Research 143 (4): 72–82. doi:10.1016/j.jcsr.2017.12.020.
- Ma, H., J. Xue, Y. Liu, and J. Dong. 2016. “Numerical Analysis and Horizontal Bearing Capacity of SRRC Columns.” Steel and Composite Structures 22 (4): 797–820. doi:10.12989/scs.2016.22.4.797.
- Nour, A. I., and E. M. Güneyisi. 2019. “Prediction Model on Compressive Strength of Recycled Aggregate Concrete Filled Steel Tube Columns.” Composites Part B: Engineering 173 (9): 106938. doi:10.1016/j.compositesb.2019.106938.
- Tam, V. W. Y., A. Butera, K. N. Le, and W. G. Li. 2020. “Utilising CO2 Technologies for Recycled Aggregate Concrete: A Critical Review.” Construction and Building Materials 250 (7): 118903. doi:10.1016/j.conbuildmat.2020.118903.
- Tam, V. W. Y., M. Soomro, and A. C. J. Evangelista. 2018. “A Review of Recycled Aggregate in Concrete Applications (2000–2017).” Construction and Building Materials 172 (5): 272–292. doi:10.1016/j.conbuildmat.2018.03.240.
- Wang, C., and J. Xiao. 2018. “Evaluation of the Stress-strain Behavior of Confined Recycled Aggregate Concrete under Monotonic Dynamic Loadings.” Cement and Concrete Composites 87 (3): 149–163. doi:10.1016/j.cemconcomp.2017.12.012.
- Xiao, J. 2008. Recycled Concrete. Beijing: China Architecture & Building Press. (in Chinese).
- Xiao, J., W. Li, Y. Fan, and X. Huang. 2012. “An Overview of Study on Recycled Aggregate Concrete in China (1996–2011).” Construction and Building Materials 31 (6): 364–383. doi:10.1016/j.conbuildmat.2011.12.074.
- Yang, Y. 2007. “Theoretical Research on Load-deformation Relations of Recycled Aggregate Concrete-filled Steel Tubular Members.” Industrial Construction 12: 1–6+124.
- Yang, Y., Z. Zhang, and F. Fu. 2015. “Experimental and Numerical Study on Square RACFST Members under Lateral Impact Loading.” Journal of Constructional Steel Research 111 (8): 43–56. doi:10.1016/j.jcsr.2015.04.004.
- Yu, F., L. Chen, Y. Fang, J. Jiang, S. Wang, and Z. Kong. 2019a. “Study on Load-deflection Relationship of Recycled Self-compacting Concrete Filled Steel Tubular Columns Subjected to Eccentric Compression.” Adv Steel Constr 15 (3): 252–258.
- Yu, F., A. Cheng, and G. Xu. 2015. “Nonlinear Finite Element Analysis on Seismic Behavior of PVC-CFRP Confined Reinforced Concrete Columns.” Chinese Journal of Computational Mechanics 32 (6): 781–788. in Chinese.
- Yu, Z., R. Tang, P. Cao, Q. Huang, F. Shi, and F. Shi. 2019b. “Multi-axial Test and Failure Criterion Analysis on Self-compacting Lightweight Aggregate Concrete.” Construction and Building Materials 215 (8): 786–798. doi:10.1016/j.conbuildmat.2019.04.236.
- Zeng, L., L. Li, Z. Su, and F. Liu. 2018. “Compressive Test of GFRP-recycled Aggregate Concrete-steel Tubular Long Columns.” Construction and Building Materials 176 (7): 295–312. doi:10.1016/j.conbuildmat.2018.05.068.
- Zhang, X., and X. Gao. 2019. “The Hysteretic Behavior of Recycled Aggregate Concrete-filled Square Steel Tube Columns.” Engineering Structures 198 (11): 109523. doi:10.1016/j.engstruct.2019.109523.
- Zhong, S. 2003. Concrete-filled Steel Tube Structure. Beijing: Tsinghua University Publishing House. (in Chinese).