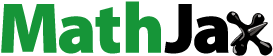
ABSTRACT
With a people-oriented principle, contemporary architectural design regards the users’ spiritual and emotional satisfaction as fundamental needs. However, it was tough for designers to collect and quantify the users’ accurate perception of a design scheme in the past. This research aims to propose a method incorporating virtual reality (VR) and sensors, and wearable technology to pre-evaluate the impact of spatial schemes on user experience. Specifically, it collected users’ physiological signals to detect emotions and eye-tracking data to understand visual attention during their roaming in a VR environment. An empirical design scheme for the International School of Design’s outdoor spaces in Harbin Institute of Technology was adopted as a study case. For this purpose, multiple groups of contrast schemes were established in VR for users to experience. Participants’ physiological signals were collected and analyzed using the paired-samples T-test method. Subjective questionnaire data were also collected to assist in the verification of objective physiological data. The results showed that designers could evaluate the experience of the designs by comparing user reactions through analysis of physiological signals. Simultaneously, this evaluation method explores a feasible way for designers to promote architecture designs to be more humanized and scientific.
GRAPHICAL ABSTRACT
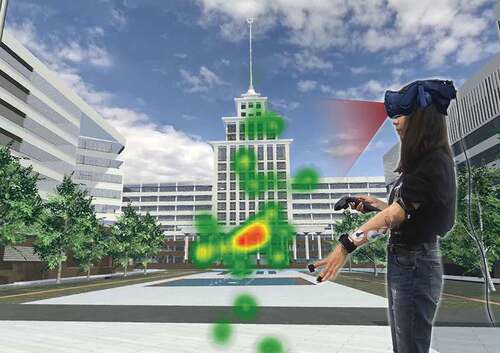
1. Introduction
The relationship between human perception and the physical environment is always a central issue for architecture design. Architects generally agree that the user experience, which can be used as feedback information, plays a crucial role in improving design schemes and realizing design concepts (Dias et al. Citation2014). The experiential architectural design looks at how users will interact with the space and builds the environment around human needs and experience. One of the proofs of good “Experiential architecture” is that it can convey the metaphor and concept of the design to users. That means the space users, who know little about the history, design intention, and construction techniques of the building can still perceive the information the designer wants to convey when experiencing the space. Merleau-Ponty’s Phenomenology of perception (Mooney Citation2012) grants a central role of the world to the human body because perception is a prerequisite for understanding the external environment (Cañas Citation2019). Since the 1960s, the decline of Modernism, research on the relationship between human and built environment has become further influential. Studies were concerned with the mutual interaction of individuals and the architectural spaces (Leavitt Citation1988; Rapoport Citation2016). The development direction of contemporary architecture design is gradually being transferred from designer-oriented to user-oriented (Cho and Kim Citation2017), which make the design prioritizes human perception and experience feeling of the building environment. One of the essential responsibilities of architects is designing experiences in spaces (Soltani and Kirci Citation2019). This means architects need to intentionally use design elements to elicit an emotional connection to space and immerse users in a narrative, using colour, light, and form composition to communicate spirit, quality, and character (Spence Citation2020).
However, too often, some buildings could not match the original aspirations of architects. Once the building space is built, changing or rebuilding will cause higher-than-expected resource consumption. To avoid the significant deviation between the users’ experience and the architect’s design objective, in the planning and design stages of building, the preliminary evaluation of the design scheme is essential to promote a sustainable and humanized building environment. During the design process of the architectural environment, one of the assignments of architects is Combining rationality and emotion and provide a positive spatial experience for users. Hence, architects need to get users’ accurate perceptions in the design spaces to evaluate and improve the schemes. The experiential user data collected by researchers allows architects to understand users’ needs and expectations and create a further harmonious relationship between humans and the built environment (Horayangkura Citation2012; Leavitt Citation1988). However, describing perception is more challenging than the description of physical environment. It always involves psychological characteristics, which are qualitative factors (Husserl Citation1997) and could be challenging to describe accurately (Li et al. Citation2020). It was tough for architects to collect and quantize users’ perception feedback in the past, especially before construction.
Heilig (1992) believed human attention could be influenced by various senses, including vision, audition, olfaction, touch (occupy 70%, 20%, 5%, 4% respectively), and so on (Rouch Citation1985). In addition, the users (with normal vision) mainly obtain spatial information, which is processed to recognize space through vision (Kiefer et al. Citation2017). Hence, previous research on the relationship between architectural spaces and human perception largely depends on visual perception and focused on surveying occupants after complete construction (Hedge et al. Citation1989). For example, the “Likert scale” and “Factor analysis” were used as visual evaluation tools to obtain users’ quantifiable psychological feelings and reveal their aesthetic preferences (Rogge, Nevens, and Gulinck Citation2007). At that time, the research used traditional techniques, such as questionnaires (Caicedo and Van Beuzekom Citation2006), scales or verbal protocols for self-report (Mauss and Robinson Citation2009), group discussion and interview (Annemans et al. Citation2014), video content analysis and behaviour observations. These survey methods were time-consuming and prone to errors introduced by the subjective feelings of the surveyed. While these traditional methods can provide valuable subjective insights, they are less effective in making objective comparisons between multiple designs schemes (Wingler et al. Citation2020). Other researchers have attempted to analyze users’ perceptions and emotions through “Overt behavior” such as facial expressions (Cowie et al. Citation2008; Li and Elmaghraby Citation2014) and body gesture recognition (Kapur et al. Citation2005). However, the accuracy and objectivity of “overt behavior” are still controversial. For example, people can hide their emotions by changing expressions. In recent years, perception tests combined with biosensing and information technology can provide an opportunity to detect and analyze cognition and feeling. Psycho-physiological measurements on the signals emitted from the central and peripheral nervous systems are currently several of the essential techniques to get users perception (Yadav et al. Citation2018). The sensors such as those collecting peripheral physiological signals, wearable devices and eye trackers present an opportunity to capture users’ physiologic parameters and cognitive states in a real-time and non-intrusive way. Compared with the former two kinds of evaluation methods, using bio-sensors to collect physiological data and recognize users’ perceptions is a generally accepted and objective measurement method at present (Li et al. Citation2015). Although the neurophysiological recording analysis would require even more time than the questionnaire survey, the collected physiological data are not easily controlled by people subject awareness because these data reflect the activity of the physiological systems caused by real emotion change.
The primary work of applying the perception test to the building environment is to prepare the experimental setting. The experimental environment could be divided into two categories. One is the natural environment, and the other is the experimental environment which can be constructed by adopting technologies such as VR and augmented reality (AR) etc. VR is a unique tool (Liu et al. Citation2020) that allows high-quality visualization of architectural space before construction. Besides, quantifying the human perception of architectural spaces need to create controlled testing environments (Franz, von der Heyde, and Bülthoff Citation2005) in which architects can alter and test the architectural design elements individually. The recent advancement of some technologies like VR provides opportunities to offer controlled environments with a high degree of realism for users and architects to replicate rapidly and alter testing conditions flexibly (Heydarian et al. Citation2015; Zou, Li, and Cao Citation2017). Some studies combined VR and bio-sensors to identify the spaces that may lead to negative emotional experiences (stress, anxiety and fear) (Dias et al. Citation2014; Engelniederhammer, Papastefanou, and Xiang Citation2019; Lee et al. Citation2020;) and compared the alterations between cognition and affect in real and virtual environments (Skorupka Citation2009; Westerdahl et al. Citation2006). Afterwards, proposed virtual reality technology as a strong potential psychological and architectural research tool (Kuliga et al. Citation2015). VR provides a controllable environment for testing unbuilt design schemes and provides the possibility to study various architectural spaces quickly (Jansen-Osmann Citation2002). Besides, virtual environments could awaken users’ emotions, such as relaxation or anxiety (Marín-Morales et al. Citation2018). The application of VR in buildings will enable users to interact more strongly with the design environment, eliciting emotional and psychological responses (López-Tarruella Maldonado et al. Citation2016). Many researchers have found that VR can assist in assessing users’ emotional reactions to gain a more precise understanding of the designed architecture spaces (Nikolic Citation2007; Paes, Arantes, and Irizarry Citation2017) and improve design decision-making. Integrating VR and psycho-physiological measurements () effectively quantify human perception and combines it into architectural design and evaluation.
Figure 1. Methods and techniques in human perception and the built environment (Source: adapted from Karakas and Yildiz Citation2020).
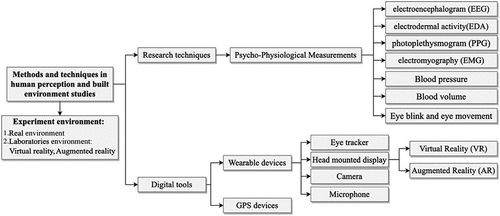
Physiological changes are the sympathetic nervous system’s response (SNS). The SNS will automatically activate physiological responses when the body perceives an environmental stimulus, including accelerated heart rate, dilated pupils, and increased sweat gland activity (Levenson Citation2014). This autonomous response can be triggered by many emotional reactions (such as surprise, fear, anger or stress) and is difficult to hide. In addition, the experimental result has shown that some autonomic nervous system activities are not culture-specific; that is, the emotional differences reflected by autonomic nervous system activities have cross-cultural consistency (Levenson et al. Citation1992). Hence, it is recently becoming prevalent in using ambulatory sensors to measure objective physiological signals and analyze the emotional reaction (Zeile et al. Citation2015) (). Skin conductance, heart rate, and blood volume were valid and extensively utilized emotional arousal measures (Dawson, Schell, and Filion Citation2017; Wac and Tsiourti Citation2014). For example, Radwan and Ergan used a data collection platform named Body Sensor Networks (BSN) to record the subjects’ physiological metrics (i.e., heart rate, facial muscle movement) in interior architectural spaces (Radwan and Ergan Citation2017). Some other studies collected some physiological data (i.e., skin conductance, skin temperature) to comprehend people’s emotional states in urban built environments (Engelniederhammer, Papastefanou, and Xiang Citation2019; Lee et al. Citation2020).
Figure 2. Research technologies: (a) ErgoLAB physiological sensors for EDA and PPG; (b) Tobii Pro VR eye-trackers.
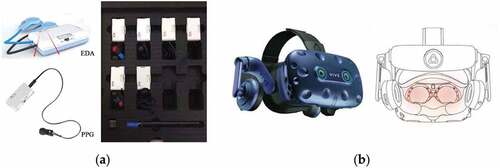
In addition to emotional responses, visual perception testing is one of the essential psycho-physiological measurement technologies in the research field of neuroarchitecture. Eye-tracking is an established technique standardly utilized in medicine, psychology, usability and User Experience (UX) (Junker and Nollen Citation2018). Eye-tracking was used to obtain visual evaluation information of spatial design in VR and fused with users’ location data (Jacob and Karn Citation2003, p.; Zhang, Jeng, and Zhang Citation2018). The main purpose of the eye-tracking application is to measure the users’ attention and identify the specific parts of a design (Yousefi et al. Citation2015) by monitoring the participants’ attention towards the Area of Interest (AOI). However, for architecture applications that need the immersive representation of a design model, this kind of set-up used not work, and few studies focused on using eye-tracking for architectural design (Aries et al., Citation2010; Hasanzadeh, Esmaeili, and Dodd Citation2016).
Recently, with the fast technology development, some software is compatible with HTC Vive Pro () and available to support the analysis and visualization of eye-tracking data gathered in VR studies. The most important thing is to create interactive virtual environments to measure the subjects’ attention when experiencing various architectural designs (Du et al. Citation2018; Radwan and Ergan Citation2017). Some researchers have begun to use eye-tracking to understand users’ visual perception in architectural design (Tang Citationn.d.; Zhang, Jeng, and Zhang Citation2018). Both the physiological test of emotion and eye-tracking measurement are not easily subjectively control by people, which is generally accepted as a suitable measurement method. These two methods reflect human perception from different aspects. Combining these two technologies will help understand a depth physiological detection mechanism. However, combining eye-tracking test and physiological test has not previously been considered by existed research especially on how to support the evaluation of building space design. Even though some previous research (Higuera-Trujillo, Llinares, and Macagno Citation2021) has used biometric sensors and VR as helpful technologies to quantify users’ perceptions in building space, little research has proposed applying the perceptual measurement to assist the pre-evaluation of design objectives and practical design stage. Besides, there is a lack of empirical research integrating objective physiological signals into an evaluation workflow to improve architectural schemes in the design stage. It would therefore be of interest to combine biometric sensors and eye trackers to get both emotional and visual perception feedback of users and support the improvement and evaluation of spatial design before construction.
This research integrally applied multidimensional physiological detection, including psycho-physiological and eye-movement measurements, which can reflect the users’ emotional state and the causes to evaluate the architectural spatial design. The combination of emotion and eye movement knowledge will contribute to constructing a more comprehensive perception evaluation method. The research presented in this paper aims to propose a method of integrating virtual reality and sensors and wearable technology to a pre-evaluate spatial scheme based on the design goals of the human experience.
The research objectives including:
(1) Proposing a method combining physiological signals to assist the pre-evaluation of the experiential space design by integrating VR and bio-sensor technologies;
(2) Applying this method in the design stage of a practical architectural project to verify its applicability of assisting the pre-evaluation and perception-based analysis of spaces;
This paper is structured as follows: Section 2 reviews existing literature and related works on using VR and bio-sensor technologies in the architecture spaces design. Section 3 presents the framework of the evaluation method combining with an empirical case, which includes the experimental design and its sub-components. Section 4 details the statistical analysis applied to our physiological signals and questionnaire data and the implications on the pre-evaluation of design using affective physiological signals. Finally, the conclusion and future directions of this work are described briefly in section 5.
2. Materials and Methods
The pre-evaluation method proposed in this research adopted the VR technology, eye-tracking signal acquisition and peripheral physiological signals to get human vision interest and arousal dimension of emotions when roaming in the pre-simulated VR environment. In this study, we chose physiological sensing (PPG and EDA) to detect the emotional arousal of the participants because the participants need to roam around the virtual environment using the VR controllers and the physiological sensing is more ease-of-use during exercise and other physical activities. Although more comprehensive emotional information could be obtained by means of EEG, the participants cannot move their limbs freely and need to minimize the number of blinks in order to reduce the interference of Electromyography (EMG) and Electrooculogram (EOG) signals with EEG. In this experiment, the participant’s head could not remain stationary and needed to look around during the roaming process, which made it difficult to meet the conditions for collecting effective EEG signals.
By collecting the physiological data and analysis combining with the experiential design intention, this assists method could provide the suggestion for assisting architects and decision-makers improve the design of the spaces. This part includes four sections to introduce the research method and experiment design. The first part proposes the construction of a pre-evaluation workflow. The second section is about the background information of the empirical case. The third section introduces the process of visualizing the tested space of the practical case. After finishing the construction of experimental spaces, the fourth part analyzed the method of extracting spatial elements and generated the comparison schemes. The design and details of the experiment are also introduced in the fourth part.
2.1. The pre-evaluation workflow
The pre-evaluation workflow mainly includes four stages (). This workflow constructed rely on the application of technologies mentioned before. The workflow construction was detailed introduced in the writers’ conference paper (Pei, Guo, and Lo Citation2021a). The workflow of evaluation mainly including four parts:
1. The researcher created the immersive spaces of design scheme through the use of virtual space based on VR.
2. The researchers extracted design factors from the design scheme and modified the design art to construct several different scenarios through l variables.
3. The researchers obtained eye-tracking and signal data peripheral physiological from subjects in the simulation space through VR eye-trackers and sensors.
4. The researchers analyzed physiological data to explore the human feeling of the spatial design environment and provide suggestions combined with design objectives.
2.2. The empirical case
To apply this pre-evaluation method to assist experiential space design and analysis the feasibility of this method. In this study, we chose an axis campus space design of Harbin Institute of Technology Shenzhen International School of Design (SISD) () as the sample to finish an empirical case study.
This experiential space scheme was in the stage of design optimization. Hence, it would be possible for us to construct a VR environment with efficient spatial details. SISD space has an essential campus location that connects the entrances and the main building. During the design optimization process, the university decision-makers, design units, construction units, and experts launched several rounds of discussion on the functional layout, public space, energy-saving issues, landscape design, facade design, etc. However, due to the significant differences in the aesthetic preferences, professional knowledge and concerns of the interest representatives of different parties, they had some other points of view about some spatial design objectives. At the same time, the architects did not get enough feedback from users to solve these design disputes.
2.3. The generation of the virtual environment
We firstly constructed SketchUp manual models and used Unity3D software to build the VR environment (). The data analysis tool, Tobii Pro VR Analytics, can collect and playback eye movement data in a Unity 3D environment. Hence, Unity3D meets the compatibility requirements of perceptual detection. At the same time, Unity3D provides a VR environment that can be experienced by wearing a head-mounted display. Quantitative data (including eye movement, skin electricity, electrocardiogram, etc.) of humans are collected by wearing physiological instruments in real-time for perception analysis and evaluation.
2.4. Spatial elements and comparison schemes
2.4.1. Spatial elements
The spatial components are the mainly stimulus for the users roaming in the spaces. To investigate and test the user’s perception feedback on the space design, the space components should be classified according to the exist criteria proposed. In 1959, Edward Hall summed up three types of space with different formal characteristics: fixed space, semi-fixed characteristic space and informal space (Zimmerman and Kitsantas Citation2005). Based on Hall’s theoretical point of view, Amos Rapport summarized the elements of the architecture in 1982 and further divided the space into three categories: fixed features, semi-fixed features and non-fixed features () (Rapoport Citation1990). Each element forms the final architectural space based on a certain combination.
Hence, basically all kinds of architectural spaces components could be divided into three categories. Firstly, the fixed-feature elements (Rapoport Citation1990) refer to the standard components like walls, ceilings, and floors fixed or changed slowly. In most cases, they are supplemented by other elements to convey the meaning of spaces. Secondly, semifixed-feature elements are the arrangement and type of furniture, plants, facility and other elements that change pretty quickly and easily. Semifixed-feature elements are essential in current research on spatial meaning. Thirdly, nonfixed-feature elements are related to the human behaviours in the spaces, including their body positions and postures, hand and arm gestures, facial expressions, eye contact.
Since the pre-evaluation method proposed by this research is developed for the design optimization stage for the practical projects (after completing the design and before conducting the construction). Hence, the fixed-feature elements like the plane layout and section form have been determined in the early stages of design formation. The nonfixed-feature elements are related to the using patterns of users when they use the physical space which are usually be considered and observed after finishing construction. Therefore, the main object of discussion is the semifixed-feature elements.
To identify the semifixed-feature elements that need to be evaluated in the design of SISD campus space. We reviewed the recordings of the discussion sessions () attended by decision makers from various parties. Through reviewing the sessions’ recording, we concluded the most mentioned and controversial semifixed-feature elements during the process of design optimization (). The university decision-makers (party A) proposed these spatial elements and design issues, which could not be answered by designers (party B) with absolute certainty. We will focus on testing these spatial factors by designing contrast schemes.
Figure 7. The recording of the controversial elements during (a) the discussion sessions and (b) the site survey.
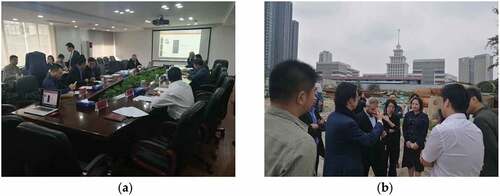
Table 1. The point at issues of the controversial semifixed-feature elements of SISD design.
2.4.2. Comparison schemes
According to the design scheme from Ateliers 234 architecture office, this SISD axis campus space mainly composed of three parts: the entrance space, the square space and the axis space. The program designers defined these three spatial nodes with the themes: “Origin”, “Development” and “Transcendence” to show the development of the university over the past century. Therefore, starting from the entrance of the campus, the designers designed a series of spatial nodes along the walking sequence and wish to let the users have a gradually emotional arousal during the process of roaming and eventually reach the core area of the campus (the main building). Each of the three spatial nodes (entrance, square, axis) on the path had many spatial design elements. To point out the elements most worthy evaluation and form comparison schemes, this research analyzed the controversial elements and the elements designed to have a significant impact on human feeling by the architect for the spatial nodes. Finally, we identified three elements: “school gate stone”, “palm trees group”, and “Corridor bridge” in each spatial node as the variable elements.
To fully understand people’s physiological feedback to various spatial elements on the roaming path, this experiment set two kinds of comparison (): one was the “horizontal comparison”, the other was “vertical comparison” (Pei, Guo, and Lo Citation2021b). The “horizontal comparison” is applied to evaluate the influence on users’ perception caused by the three most essential elements when they experience the three spatial nodes separately. Meanwhile, the “vertical comparison” is designed to test the users’ visual attention to the other spatial elements appeared during the roaming process as well as the change of emotional arousal level during roaming process. Combining the horizontal and vertical comparison, we could collect the feedback data required for the design scheme evaluation. At the same time, the users only need to roam two times in the experimental group and control group virtual environments separately.
The horizontal comparison including “experimental group” and “control group”. Along the “experimental group” path, the three essential elements were set and appeared gradually with the roaming process. On the contrary, along the “control group” path, these three elements were removed. Comparing spatial nodes along the path removes these elements. Through analyzing the two groups’ perception results, we can know the influence of these spatial elements on the user’s and whether the design of these elements can truly reflect the design objectives of architect. The “vertical comparison” including the contrast of users’ perception change when roaming different spatial nodes and their visual perception of the other spatial elements including gate stone, palm trees, building elevation landscape belt corridor bridge and signage system etc. along the path.
2.4.3. Participants
To eliminate or reduce the errors caused by differences between subjects, this experiment was a within-subjects (within-groups) design test. Each participant has to conduct experiments with all conditions. In this study, through the distribution of recruitment information on social platforms and online platforms, a total of 30 participants (16 female, 14 male) were eventually recruited, ranging in age from 18 to 40 (7 was 18–25 years old, 18 was 26–30 years old, and five was 31–40 years old). Of the 30 participants, 10 people had VR devices at home or workplaces and used them regularly. 12 people said they had limited experience using VR before, and 8 people had never used it. Besides, the subject pool included people from different educational and occupational backgrounds. In this study, the 30 subjects had different occupational backgrounds, including students, financial workers, salespeople, technical engineers, translators, and product developers. Only two of them worked in architectural design-related jobs, so the spatial perception bias would be mitigated. The experiment’s participants’ conditions were normal vision and no diseases such as heart disease or vertigo. Participants did not join in the design process of this project or experience this space sample before the experiment. They were told all the possible risks of the investigation before the experiment and agreed to participate in this research study.
To eliminate accumulative error caused by experiment order from one condition to another, 15 subjects will first roam the control group and then the experimental group; the other 15 participants will wander in reverse order. Because this will be an open campus space that welcomes the public, the subjects’ educational background and occupation are not restricted in this experiment.
2.4.4. Experimental equipment and environment
This research used the ErgoLAB man-machine synchronization platform (“ErgoLAB 3.0” King far International Inc.) (). This platform has the function of real-time synchronous acquisition, processing and analysis of multimodal human-machine-environment data. Since 2013, the platform has been used as an academic scientific research tool in relevant research units or institutes. The test sample groups (subjects) cover a wide range of research fields such as built environment, safety engineering, user experience, military defense, traffic and driving behavior, industrial innovation design, sports, psychology, etc. The search terms of the outcomes using this platform is “ErgoLAB & kingfar”
In this study, ErgoLAB 3.0 was used to synchronize the HTC Vive Pro VR eye-tracking system (recording frequencies: 120 Hz, Resolution: 1440 × 1600 pixels per eye, the trackable field of view: 110°) in real time with an eye movement sampling frequency of 120 Hz. The velocity-threshold (I–VT) algorithm was used to extract gaze points and further analyze eye movement indexes such as gaze count, gaze duration, pupil diameter, eye beat, and blink count. Meanwhile, the eye movement data are synchronized in real time with the ErgoLAB wearable wireless physiological recording system. Among them, the skin resistance sensor (EDA) and ear tip pulse sensor (PPG-HR/HRV) were sampled at 64 Hz (EDA sampling accuracy 0.01 μs; HR sampling accuracy 1 bpm) to further analyze heart rate (HR) and heart rate variability (HRV) in the time domain (standard deviation of RR intervals-SDNN and root mean square difference of adjacent RR interval sequences-RMSSD) with frequency domain indicators (energy ratio LF/HF) and physiological indicators such as skin conductance (SC), some of which were calculated as follows (1) (2):
For the data analysis, the ErgoLAB includes signal processing modules, which can preprocess data, and select data fragments to filter and extract meaningful data results. The data of preprocessed physiological signals collected by wearable sensors can be exported for statistical analysis after being processed.
The experiment was carried out in the laboratory of Beijing Jinfa Technology Co., Ltd. The laboratory is an independent room that includes the experiment area and the analysis area to reduce the environmental interference in the experiment. Through strictly controls experimental variables, this research minimized the real environmental influence on the participates’ sense of presence when experience VR. The researchers synchronously monitored the data collected and conveyed experimental instructions in real-time.
2.4.5. Experimental process
The formal experimental process () design mainly includes the following five stages:
1. The preparation stage: explaining experiments and the method to wear physical sensors. Subjects first understand the experiment content, the path of roaming and wear the related equipment () and VR eye movement device. The eye movement equipment needed calibration, after which the subjects calmed down for about 5 minutes until ready to start the experiment.
2. The first stage of the experiment: the subjects completed the roaming in the experimental space. The subjects wore Tobii Pro VR eye tracker and wandered along the path of the tested spatial sequence. Their physiological devices recorded was collected by the ErgoLAB platform, and the first stage lasted about 5 minutes.
3. Rest: Let participants rest for about 10 minutes. The researcher switched the VR scene (remove the space elements) and checked whether the collected physiological and eye movement data were complete, regular and exportable.
4. The second stage of the experiment: the subjects completed the roaming in the other space. The subjects wore Tobii Pro VR eye tracker and wandered along the path of the tested spatial sequence. Their physiological devices recorded was collected by the ErgoLAB platform, and the second stage lasted about 5 minutes.
5. Finish subjective questionnaire: help the subjects remove the devices they wore and let them take a short rest to complete the subjective questionnaire survey, which was used to verify and analyze the physiological signals and collect the subjects’ suggestions on the spatial schemes. This process took about 10 minutes. At the same time, the researcher sorted out the stored data and prepared the next subject for the experiment.
3. Result
The data analysis in this study is based on objective data collection and a subjective questionnaire survey. The physiological signal includes the physiological indicators and eye-tracking data. This research used the data analysis modules of the ErgoLAB platform to do the data analysis. This study used ErgoLAB EDA analysis software which has the function of smoothing data to automatically extract the Skin conductance (SC) data (μs) varied with time. Besides, we used ErgoLAB PPG analysis software
3.1. Objective data
3.1.1. Emotion model and physiological signals
Although human emotions are complex, several standard models are used in psychological research to define and measure emotions. There are two types of models for emotions measurement: one is the discrete emotion model; the other is the dimensional emotions model. Compared with the discrete emotion model, the dimensional emotion model is more suitable for emotion recognition based on physiological signals, which does not need to judge the emotion type precisely. The Circumplex Model () is a widely used dimensional emotion model (Russell Citation1980) that has two such dimensions: Valence and Arousal, and can quantitatively describe emotion. The valence (X-axis) is used to describe the degree of liking, from liking (positive) to disliking (negative); Emotional arousal (Y-axis) is used to describe the degree of arousal from low arousal (low arousal) to high arousal (high arousal). Since 1980, the emotion mode tool has begun to be applied in environmental psychology research and was widely accepted in architectural studies (Plutchik Citation1980).
Figure 12. Wearable physical sensors were used in this experiment (Braithwaite et al. Citation2013).
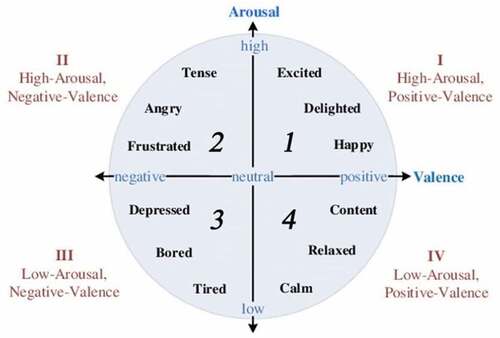
The key to measure emotion based on physiological response patterns is collecting reliable physiological signals. In this study, EDA and PPG, the two most commonly used and stable physiological indicators in physiological and emotional analysis, are selected to conduct physiological sensing detection and emotion recognition for users who have roaming experiences in architectural space.
Electrodermal activity (EDA) describes autonomic changes in the skin’s electrical properties which is decomposed into Skin Conductance Level (SCL) and Skin Conductance Responses (SCRs) (Park Citation2009). The most widely studied property of EDA is the Skin Conductance (SC). Skin Conductance (SC) is the time-domain indicator that has been suggested by preliminary evidence which is an important index of respondents’ arousal levels. Since there is a positive linear correlation between SC and arousal level, it is often used to study psychological and emotional states (Porges Citation1995). The SC has been shown to be higher during activated states, such as states of high emotional arousal level. The SCRs is the phasic data of SC which represents the fast-changing signal res ponding to a stimulus. SCRs usually last for a few seconds and is caused by a particular stimulus or event. The SCL is the tonic data of SC which stands for continuous, slowly-changing Skin Conductance Level that could last for at least 30s (Braithwaite et al. Citation2013). The SCL refers to respondents’ long-term reaction to stimuli and general changes in autonomic arousal which is used in this research to indicate moment-to-moment (roaming process in the built space) arousal level change.
PPG is mainly used to measure the user’s heart rate variability (HRV), which can give objective indicators of sympathetic (SNS) and parasympathetic nerve activity (PNS) balance in the autonomic nervous system (Electrophysiology Citation1996). Descriptive values (mean ± sd) of HRV in time, including i) the mean of Heart Rate (Mean HR); ii) standard deviation regular RR interval (SDNN) and iii) root mean square of successive differences squared (RMSSD). In the frequency domain variables, the following was considered of the spectral components: i) very low-frequency component (VLF); ii) low-frequency component (LF); iii) high-frequency component (HF), and iv) LF/HF ratio.
The most commonly used time-domain measures for short-term recordings are RMSSD and SDNN. RMSSD metric is thought to primarily represent the indicator of beat-to-beat variations related to PNS (Kreibig Citation2010); SDNN indicates overall variability. Subjects who had high SDNN exhibited greater activation of other autonomic nervous (including PNS and SNS). Frequency-domain main variables: LF/HF ratio reveals the balance between SNS and PNS. Heart rate is slowed due to increased PNS activity when people have a rest. When people have an emotional episode, the PNS and SNS influence would be reduced and increased, respectively, increasing heart rate. The PNS activity can be measured as the RMSSD, while SNS activity can be indicated by heart rate (Daniel and Kamioka Citation2017). The higher the emotional arousal (positive or negative), the higher the Mean HR and the lower the RMSSD.
3.1.2. Area of interest and eye movement indicators
To analyze the visual attention of spatial elements that occurred along this roaming path, Area of Interest (AOI) tools should be used to select regions of a displayed element in advance () so that the area by which metrics are calculated. For analysis, AOI Fixation Count (N) and AOI Fixation Duration (s) were selected as the eye-movement index. These two indexes can reflect users’ attention to spatial scene factors. (i) AOI Fixation Count represents how the subject processes and distributes attention to the spatial elements. The more Fixation times, the more interested the subjects are in the area of interest. (ii) AOI Fixation Duration denotes the duration of fixation when the subject’s viewpoint stays in the AOI. The longer the AOI Fixation Duration, the longer the subject spent fixing the AOI during the roaming, which means the subjects may be more interested in these elements. Besides, (iii) Pupil diameter has been proved to be a reliable physiological feature that can reflect subjects’ emotional state and degree of focus. Pupil dilation is determined by emotional arousal, independent of whether visual stimulations are pleasant or unpleasant. (iv) The more blink counts the user has, to some extent, the more nervous or anxious the user is; The average absolute distance reflects the degree of openness and narrowness of space.
3.1.3. Experimental result
As mentioned above, this experiment adopted a within-group design. This research used statistical methods for the experiment data analysis, including paired-sample t-test and Wilcoxon Signed Rank Test. Meanwhile, before data analysis, the descriptive statistic function of SPSS (version 20.0.0) software was used to check the outliers of collected data. The value of more than 3 standard deviations above or below the mean can be regarded as extreme values. When the extreme value has information, the normal processing method uses the edge value corresponding to the upper and lower limits instead. We extracted the physiological, eye movement and subjective questionnaires data through horizontal and vertical comparison analysis, which has significant differences (P < 0.05). We finally obtained feedback on spatial design and factors using multivariate data to conduct integrated statistics and mutual verification. All Statistical calculations are performed in SPSS.
3.1.3.1. Horizontal comparison
The horizontal comparison mainly includes the comparison of the paired comparison of “entrance”, “square”, and “axis” three spaces and some comparison according to design intent. Firstly, we used SPSS to check data outliers (). After processing extreme value data, we conducted Normality tests in the statistical software “SPSS”. Paired sample t-test can be used when our data follow a normal distribution; otherwise, the Wilcoxon Sign-Rank test should be used to analyze whether the difference of each physiological index between two sample means is “statistically significant”. In addition, we analyzed the mean value physiological signals of the 30 subjects were to obtain the central tendency of their physiological feedback.
Figure 14. Check outliers of physiological feedback data of the whole roaming path (generated by SPSS). (a) Mean HR difference. (b) SDNN difference (c) RMSSD difference (d) HF/LF difference (e) SC difference.

1. Comparison of whole roaming path
According to the physiological data statistical analysis of the whole path (), we found that the subjects’ physiological data have no significant difference when roaming the experimental path and control path (p > 0.05). The average level of 30 subjects’ physiological indexes shows that () the subjects’ autonomic nervous is more active when roaming the control path, in the process of path roaming. These 30 subjects may have higher emotional arousal level when roaming in the “control group” path. However, there was no statistical significance of users’ perception when roaming in the “control group” and “experiment group” paths which means the presence or absence of these three spatial elements would not have a statistically significant effect on the participants’ emotional arousal level during the process of roaming the whole path.
Table 2. Physiological feedback data of the whole roaming path.
Besides, we analyzed the user’s eye movements data, including Pupil Diameter (mm), Absolute Distance (Px) and Blink Count (N/s). From the statistical analysis result (), there was still no significant difference when subjects were roaming in two different paths (p > 0.05). The average analysis of the 30 subjects’ eye movement indexes () showed that their average Absolute distance was larger when they were roaming the control group path. Daniel and Kamioka (Citation2017) compared their research and showed saccade distance was larger under complex content conditions [80]. The analysis result showed that there is more spatial information in the control path.
Table 3. Statistics of the eye movement feedback data of the whole path.
In the horizontal comparison, after statistical data processing, no significant differences were found in physiological indicators. To some extent, these three spatial factors have no significant effect on users’ emotional experience and visual perception when roaming on the whole path.
2. Horizontal comparison of three space nodes
We replayed the experimental videos of the subjects and marked three time periods on the whole roaming path according to the participant’s position in the 3D virtual space. Then, the data were derived in sections to obtain the physiological perception data of the participants in these three spatial nodes. The data of these three spatial nodes in the experimental group and control group were compared, respectively.
According to the results of statistical analysis (), there was no significant difference in emotional arousal among all the subjects when experiencing different space nodes (p < 0.05). This means that the design of three essential elements in each spatial node did not cause different emotional arousal level. Partly explains, the existing essential design elements did not have a substantial impact on constructing experiential space. For example, the designer wants to use a large school stones gate to form an atmosphere of the entrance space and impress the users. However, through the experiment, we found that this design did not have a significant effect on users’ emotional arousal. Therefore, the form of the school gate still needs to be further optimized to enhance the effect on users’ perception.
Table 4. Statistics of the physiological feedback data of three space nodes.
In the axis space design, one of the designs aims to use the horizontal corridor to enhance the depth of space and users’ attention to the facade of the main building, located at the end of the axis. Therefore, in the horizontal comparison, we also set the main building as an AOI area and collected the subjects’ eye movement data on the main building when roaming in the “axis space” with and without corridors (). According to the statistical analysis result (), the axis space with and without horizontal corridors had approached close to a significant impact on the users’ Fixation Count on the facade of the main building (p = 0.094 < 0.1). There was no difference in fixation duration, and the average happens to be the same.
Figure 17. Heat map of fixation count of the main building (schematic diagram). (a) Fixation count of the main building (experiential group); (b) Fixation count of the main building (control group).
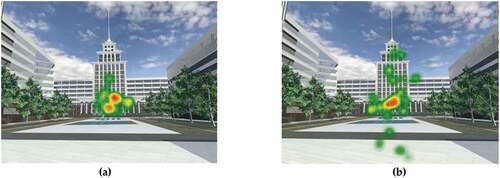
Table 5. Statistics of the physiological feedback data of three space nodes.
The experimental group has the axis space with the horizontal corridors. The scenery created by corridors will make users unable to see the full view of the main building when they just enter the axis space and gradually see the whole facade of the main building moving. It can be seen from the analysis results of eye movement data that the attention of the subjects to the main building can be enhanced to a certain extent. However, the difference of existing visual attention is not significant enough. Designers can further strengthen this effect by optimizing the horizontal corridor’s design or adjusting the corridors’ location.
3.1.3.2. Vertical comparison
In the vertical comparison, the physiological and emotional feedback of users when roaming “entrance”, “square”, and “axis” three spaces in turns will be analyzed. One-way repeated-measures ANOVA was used. In addition, the spatial elements seen in sequence along the path are set as AOI areas to explore the visual feedback.
1. Physiological signals of three spaces nodes
According to the statistical analysis (), there were significant differences in some physiological indexes of the subjects during precepting the three spaces. For example, when the three spaces were compared as a whole, it was found that the Mean HR, LF/HF and SC indexes of users were significantly different (p < 0.05). Hence, the emotional arousal states of users in these three spatial scenes are other.
Table 6. Vertical comparison of physiological feedback data in the three spaces.
To further analyze the differences of emotional arousal in the space, we conducted paired sample t-test for every two spatial nodes. It can be seen from the results that:(i) Mean HR and SC indexes of users in the “square” and “axis” space are significantly different; (ii) There are significant differences in the p values of LF/HF and SC indicators of users in the process of roaming in the “entrance” and “square” space respectively; (iii) Significant differences were found in the mean HR, LF/HF and SC of users during their roaming in the “entrance” and “axis” space.
According to the vertical comparison (), in general, the emotional arousal degree of the subjects in the three spatial nodes is as follows: entrance >square >axis. The users in the roaming process of “axis” did not have high emotional arousal. However, “entrance” is also the starting point of roaming. The roaming perception experiment has just started, which will cause some users to be relatively nervous (8 people in this experiment have never been exposed to VR environment at all), which may be one of the reasons for the high average emotional arousal of the participants in the entrance space.
2. Eye movement situation of three spaces nodes
We found that only average Pupil diameter were significant differences (). When subjects roaming in the square space (B), their average pupil diameter was larger. According to the previous studies, the pupil diameter larger means the space may be more attractive. The subjects were likely to be happier when roaming the square space. To verify the result, this study also used the subjective questionnaire to assist.
Table 7. Analysis of eye movement data of three spatial nodes in longitudinal comparison.
Another essential comparison is to analyze the visual attention of spatial elements in this testing path in the vertical comparison. According to the eye-movement data analysis result of the subjects in each AOI below (), it can be concluded that, during the roaming process, the 30 participants:
Had the most frequent fixation count on the facade of the main building (N = 79.95). This indicates that the main building has a substantial degree of attention.
Had a more frequent fixation count (N = 49.92). Even though the participants had been informed of the roaming path in advance, they still spent a long time reading the guide system and bulletin board (Mean value = 0.24s), which indicated that their design was relatively distinct and easy to attract users.
Had less attention to the entrance of the building. According to the recordings experiment, most of the subjects did not notice the entrances. The fixation count and duration were few and very short. On the one hand, the design of the entrance was not clear enough; on the other hand, the subjects were told that they could not enter the building to ignore the entrance.
Had the most prolonged attention to the stone carving on the ground, on which the designer has carved famous quotes to reflect the cultural nature of the campus. Since it was on the floor, many of the subjects ignored them while roaming. But the participants would stop and read the stone inscriptions word by word once they saw them. This shows that this design element has a specific role in cultural metaphor, but its appearance needs to be designed more eye-catching.
3.2. Subjective questionnaire data
After completing the physiological sensing test, this study used the subjective questionnaire to verify the objective physiological detection results. The subjective questionnaire mainly consists of two parts: The first part is the primary information survey of the subjects, including their age, gender, education level, occupation, VR experience, etc. The second part is about their spatial perception and feeling after completing the roaming. The questionnaire design mainly adopts the Semantic Differential Technique. Subjective survey for each space specifically includes two main contents. The first is to give a semantic rating on spatial elements and obtain the user’s preference. The second is to score the semantic meaning of the emotion scale for the emotional experience. This part is to get the information of emotional valence (positive/negative).
1. Entrance space
As the semantic differential chart shows (), on the whole, the emotions of the 30 subjects in this space are positive. Most of the subjects feel relaxed and happy with positive arousal. However, the participants generally did not feel the apparent emotional fluctuation or surprise in the entrance space. Besides, the scale of the entrance space gives people the impression of being relatively open and uncrowded. However, it should be noted that the subjects generally believe that the school gate stone was not very eye-catching, which is consistent with the eye movement analysis results. The school gate stones’ AOI had fewer fixation counts overall.
2. Square space
The square is a transition space for users from the entrance space to the axis space. According to the chart (), (i) on the whole, the square spatial scale is big to users, but most of the users did not find apparent geographical features through design; (ii) palm trees give users a positive feeling on the whole without delivery the ritual sense of the university that different from the designer expectation and needs to be improved. (iii) The facade design of the building, the skin of the building, will not produce a too dense visual impression. As for the emotional survey, users thought their emotional state was relatively happy and relaxed. At the same time, users generally believe that their emotions in the square space are more stable than in the entrance space. This is consistent with what the physiological feedback tells us.
3. Axis space
Axis space includes landscape, markers (guide system and billboard), rest seats, corridors, building facade (entrance) and other spatial elements. It can be found from the subjective questionnaire results that the users felt more relaxed and pleasant in this space. Therefore, regarding the degree of emotional arousal, the subjective questionnaire () and physiological data results show a similar trend; the entrance space will make users produce higher emotional arousal. As roaming deepens, users’ emotions level off.
For spatial elements analysis, the subjective feedback was consistent with the results of eye movement result to some extent. (i) The subjects generally believed that the content of the guiding system and the billboard was relatively explicit, with strong visual attraction and distinct location for easy viewing; (ii) The scores of the building entrance obviousness were low, indicating that the users thought that the design of the building entrance had not attracted their attention; (iii) the corridors was an essential factor in this space. Some users thought they blocked the line of sight. However, they give the space a sense of hierarchy. Users cannot see the whole of the main building at a glance. This design enhances the degree of attention paid to the facade of the main building.
4. Discussion
4.1. The findings of the empirical case experiment
4.1.1. Horizontal comparison
According to the analysis of physiological detection results and subjective survey, the most critical elements in these three spatial nodes have little influence on users’ perception. Compared with the designers’ design concepts, the existing design could not fully reflect the design objectives (). Based on this, this study puts forward the following optimization suggestions:
Table 8. Analysis of eye movement data of three spatial nodes in longitudinal comparison.
1. The design of the school gate stone in the entrance space needs to be further optimized to improve the effect of emotional contagion. Basing on the experience of architects, on the one hand, the material selection and form design could be used to improve the cultural nature of the school gate stone. On the other hand, the combination of school gate stone and landscape design would be a method to foil the campus cultural atmosphere of the entrance space. In addition, considering the tension of users when they first enter the unfamiliar campus environment, the designers could appropriately design a guiding system for entrance space and help users quickly understand the walking path.
2. The design of the palm trees group in the square did not achieve the architect’s design goals. While most users felt peace and comfort and did not have high emotional arousal, reflecting the effect on campus culture’s emotional contagion was somewhat insufficient. The architect could combine landscape design, such as setting statues between the palm trees to enhance the emotional appeal of the square;
3. The horizontal corridor in axis space is suggested to be preserved here because the comprehensive results can better enhance the sense of sequence of the axis space and improve their attention to the main building. We infer that this result is that when users enter the axis space, the horizontal corridor blocks the view of seeing the main building. The horizontal facade texture of the buildings on both sides guides users to walk forward. This design can cause the association and curiosity of the users to the distant main building.
4.1.2. Vertical comparison
According to the vertical comparison result, there are significant differences in the emotional changes of users when exploring three spaces. After analyzing the impact of space on users’ emotions and architect’s design intention (), this study puts forward the following optimization suggestions:
Table 9. Comparison of design objectives and feedback results.
1. The emotional arousal of users decreases gradually as they enter the campus. This is different from the designer’s design goal, which wanted the user to have emotional change as the sequence unfolds, at least, the design effect was not significant. Hence, the designer needs to optimize the space design to enhance the impact of emotional contagion to better convey the campus culture atmosphere and spirit.
2. According to the feedback results of eye-movement data, users do not pay enough attention to the building entrance. The guides system could be helpful to solve this problem and help users find the entrance more easily.
3. At present, the guide system, bulletin boards and ground stones landscape are used to convey campus culture. According to the eye-tracking data, the ground carving stones were important landscape design. However, through watching the experiment recording, we found that they were challenging for users to find in the process of roaming which they need to walk into and lower the head to see them on the ground. It is suggested that the design can optimize to form a certain angle with the ground so that users can read and watch it easily and give full play to its role in cultural communication.
4.2. The findings of pre-evaluation method application
This study extracted the spatial elements that need to be evaluated according to the design scheme text, the designer’s design concept and the viewpoints of all interested parties during the design optimization process. The different spatial elements were used to form the comparison schemes in next step to test the emotional and visual perception of users. The perception results standing for the users’ feedback provided objective suggestion and made the design optimization process more effective and scientific. In addition, this pre-evaluation method is based on the extraction and test of controversial spatial elements mentioned in the practical project. Hence, this method is expected could to be applied to the evaluation of experiential building spaces with different types and functions such as monumental, commercial, iconic and transportation spaces which emphasis the user’ experience. At the same time, the method could help to improve the process and results of scheme optimization especially when there are controversial factors or difficult to predict the spatial design effect by providing more scientific suggestion and feedback for optimization and comparison.
There are still some deficiencies of this research and result. First of all, collecting physiological signals need sensing devices and a controlled environment. Hence, it was difficult to widely collect the users’ perceptions to analyze combing design objectives. Besides, the physiological signals such as PPG and EDA used in this research could only reflect arousal dimension but not valence dimension of emotions. Among them, the valence dimension is used to describe the degree of (un)pleasantness, from pleasantness (positive) to unpleasantness (negative); the arousal dimension of emotion is used to describe the degree of arousal, from low arousal to high arousal. One of the limitations of this research is the lack of measuring valence dimension using physiological signals. Through physiological detection results, this research could only obtain information on the arousal dimension, without able to judge specific types of emotions. Hence, this research used questionnaires to verify the physiological detection result and got some information related to the emotional types. In the following research stage, some other physiological detection methods such as Electroencephalogram (EEG) and FER could be used to obtain the user’s emotional type. The existed research (Horlings, Datcu, and Rothkrantz Citation2008) proved that the EEG signals contained enough information on both the valence and arousal dimension. Besides, developing an algorithm to build a direction between the psychological and neurophysiological results is an expected research direction. Meanwhile, the pre-evaluation method proposed in this research requires a simulation of the schemes in a VR environment with high quality.
5. Conclusion
In this paper, we describe a workflow to pre-evaluate the design of experiential architecture combined with VR and physiological detection. Firstly, the architectural spaces that need to be tested were constructed in the VR environment, which could quickly add, subtract or change the spatial elements and create comparison schemes. Secondly, through physiological sensors and eye trackers, users’ emotional and visual perception data were recorded when roaming in the spaces. Thirdly, the users’ subjective feelings were collected using questionnaires to verify the objective physiological test result. In the end, the users’ perception data were statistically analyzed to compare the users’ different reactions to the spaces and elements. In this way, this research found that the existing three spatial features, which are most controversial in discussions between school decision-makers and designers, have little influence on the perception and need to be improved based on the design objectives. This research proposed a pre-evaluation workflow which combine biometric sensors and eye trackers to get both emotional and visual perception feedback of users and could be used to improve architectural schemes in the design stage before construction in a more objective and scientific way. In addition, this method is based on dividing building space elements to construct comparison schemes for testing which could be applied to the pre-evaluation of many types of building spaces as well as their design elements. The research result shows that this proposed workflow could help architects to real-time iterate or compare designs and enhance the existing evaluation process of participatory design in some respects. On the one hand, physiological data and detection provided more scientific and objective evidence for scheme optimization. On the other hand, the evaluation flow did not require users to understand professional knowledge or imagine 3D space based on 2D drawings. In further work, we expect to aggregate the perception data and spatial elements using big data information interaction technologies to provide direct guidance for experiential architect design. This evaluation method is expected to provide evidence-based recommendations to designers and make the architectural design process more humane and scientific.
Institutional review board statement
This study involves no greater than minimal risk to the participants and informed consent were obtained in advance. This research did not involve a prospective evaluation, did not involve interventional studies (human or animal) and only involved non-invasive physiological detecting and devices. The rights and welfare of the participants will not be adversely affected. Hence this research did not obtain ethical approval.
Informed consent statement
Informed consent was obtained from all subjects involved in the study.
Acknowledgments
Thanks to the “King far Research Support Program” of King far International Inc. for supporting this study and providing the necessary equipment.
Disclosure statement
No potential conflict of interest was reported by the author(s).
Data availability statement
The data that support the findings of this study are available from the corresponding author: LO T.upon reasonable request.
Additional information
Funding
Notes on contributors
Wanyu Pei
Wanyu PEI was an architecture master student who graduated from Harbin Institute of Technology (Shenzhen). Currently, she is a PhD student in the Department of Architecture, College of Design and Engineering, National University of Singapore. Her main research areas are architectural evaluation and design in VR Environments; human environmental perception; VR+architectural education, urban information modeling, 3D urban information mapping, circular urban design.
Xiangmin Guo
Guo Xiangmin is currently an associate professor at HITsz, a member of the Foreign Urban Planning Committee of the Urban Planning Society of China, a member of the editorial board of authoritative international SCI, and A&HCI journals, and an expert in rewriting international SCI journals. His main research interests include urban and architectural design methods supported by virtual digital technology, decision-making evaluation of urban and rural development, urban operations, and strategic planning. He has presided over and participated in a number of national funds and projects.
Tiantian Lo
Tiantian Lo is an Assistant Professor at the HITsz. During his doctoral study, he was awarded a full doctoral scholarship by the Chinese University of Hong Kong and Victoria University of Wellington. His main research area is the bottom-up human-centered architectural design concept. Using VR, BIM, and gamification to create an interactive environment. At present, he has published nearly 30 international journal and conference papers with some published and distributed by Springer. He is also the organizing committee member and reviewer of computer-aided Architectural Design Research in Asia (CAADRIA), Architectural Science Association (ANZAScA), and other international well-known conferences.
References
- Annemans, M., C. Van Audenhove, H. Vermolen, and A. Heylighen. 2014. “How to Introduce Experiential User Data: The Use of Information in Architects’ Design Process.” Paper presented at Design’s Big Debates. The Design Research Society’s 2014 Conference, Umeå, Sweden, 1626–1637.
- Aries, M. B., J. A. Veitch, and G. R. Newsham. 2010. “Windows, View, and Office Characteristics Predict Physical and Psychological Discomfort.” Journal of Environmental Psychology 30 (4): 533–541. doi:10.1016/j.jenvp.2009.12.004.
- Braithwaite, J. J., D. G. Watson, R. Jones, and M. Rowe. 2013. “A Guide for Analysing Electrodermal Activity (EDA) & Skin Conductance Responses (Scrs) for Psychological Experiments.” Psychophysiology 49 (1): 1017–1034.
- Caicedo, D. G., and M. Van Beuzekom. 2006. “How Do You Feel? An Assessment of Existing Tools for the Measurement of Emotions and Their Application in Consumer Products Research.“ Delft, Netherlands, Delft University of Technology, Department of Industrial Design.
- Cañas, P. M. 2019. “The Understanding of the Body and Movement in Merleau-Ponty.” Trans/Form/Ação 42 (1): 201–226. doi:10.1590/0101-3173.2019.v42n1.10.p201.
- Cho, M. E., and M. J. Kim. 2017. “Measurement of User Emotion and Experience in Interaction with Space.” Journal of Asian Architecture and Building Engineering 16 (1): 99–106. doi:10.3130/jaabe.16.99.
- Cowie, R., E. Douglas-Cowie, K. Karpouzis, G. Caridakis, M. Wallace, and S. Kollias. 2008. “Recognition of Emotional States in Natural human-computer Interaction.” In Multimodal User Interfaces, 119–153. Berlin/Heidelberg, Germany: Verlag Berlin Heidelberg.
- Daniel, K. N., and E. Kamioka. 2017. “Detection of Learner’s Concentration in Distance Learning System with Multiple Biological Information.” Journal of Computer and Communications 5 (4): 1. doi:10.4236/jcc.2017.54001.
- Dawson, M. E., A. M. Schell, and D. L. Filion. 2017 “The electrodermal system“ Cacioppo, J. T., Tassinary, L. G., Berntson, G. G. eds. Handbook of psychophysiology, Singapore: Cambridge University Press .
- Dias, M. S., S. Eloy, M. Carreiro, E. Vilar, S. Marques, A. Moural, P. Proênça, J. Cruz, J. d’Alpuim, and N. Carvalho. 2014. “Space Perception in Virtual Environments-On How Biometric Sensing in Virtual Environments May Give Architects Users' Feedback.” In 32nd ECAADe Conference, Newcastle upon Tyne, United Kingdom.
- Du, J., Y. Shi, Z. Zou, and D. Zhao. 2018. “CoVR: Cloud-based Multiuser Virtual Reality Headset System for Project Communication of Remote Users.” Journal of Construction Engineering and Management 144 (2): 04017109. doi:10.1061/(ASCE)CO.1943-7862.0001426.
- Electrophysiology, T. F. O. T. E. S. O. C. T. N. A. S. O. P. 1996. “Heart Rate Variability: Standards of Measurement, Physiological Interpretation, and Clinical Use.” Circulation 93 (5): 1043–1065. doi:10.1161/01.CIR.93.5.1043.
- Engelniederhammer, A., G. Papastefanou, and L. Xiang. 2019. “Crowding Density in Urban Environment and Its Effects on Emotional Responding of Pedestrians: Using Wearable Device Technology with Sensors Capturing Proximity and Psychophysiological Emotion Responses while Walking in the Street.” Journal of Human Behavior in the Social Environment 29 (5): 630–646. doi:10.1080/10911359.2019.1579149.
- Franz, G., M. von der Heyde, and H. H. Bülthoff. 2005. “Predicting Experiential Qualities of Architecture by Its Spatial Properties.“ In 8th International Association for People-Environment Studies (IAPS 2004), Vienna, Austria, 157–166.
- Hasanzadeh, S., B. Esmaeili, and M. D. Dodd. 2016. “Measuring Construction Workers’ real-time Situation Awareness Using Mobile eye-tracking.” Construction Research Congress 2016: 2894–2904.
- Hedge, A., P. S. Burge, A. S. Robertson, S. Wilson, and J. Harris-Bass. 1989. “Work-related Illness in Offices: A Proposed Model of the “Sick Building Syndrome”.” Environment International 15 (1–6): 143–158. doi:10.1016/0160-4120(89)90020-2.
- Heydarian, A., J. P. Carneiro, D. Gerber, B. Becerik-Gerber, T. Hayes, and W. Wood. 2015. “Immersive Virtual Environments versus Physical Built Environments: A Benchmarking Study for Building Design and user-built Environment Explorations.” Automation in Construction 54: 116–126. doi:10.1016/j.autcon.2015.03.020.
- Higuera-Trujillo, J. L., C. Llinares, and E. Macagno. 2021. “The cognitive-emotional Design and Study of Architectural Space: A Scoping Review of Neuroarchitecture and Its Precursor Approaches.” Sensors (Basel, Switzerland) 21 (6): 2193. doi:10.3390/s21062193.
- Horayangkura, V. 2012. “Incorporating environment-behavior Knowledge into the Design Process: An Elusive Challenge for Architects in the 21st Century.” Procedia-Social and Behavioral Sciences 50: 30–41. doi:10.1016/j.sbspro.2012.08.013.
- Horlings, R., D. Datcu, and L. J. Rothkrantz. 2008. “Emotion Recognition Using Brain Activity.” In Proceedings of the 9th International Conference on Computer Systems and Technologies and Workshop for PhD Students in Computing, Gabrovo, Bulgaria, II–1.
- Husserl, E. 1997. Thing and Space: Lectures of 1907. Vol. 7. Berlin/Heidelberg, Germany: Springer Science & Business Media.
- Jacob, R. J., and K. S. Karn. 2003. “Eye Tracking in human-computer Interaction and Usability Research: Ready to Deliver the Promises.” In The Mind’s Eye, 573–605. New York City, New York, U.S.: Knopf.
- Jansen-Osmann, P. 2002. “Using Desktop Virtual Environments to Investigate the Role of Landmarks.” Computers in Human Behavior 18 (4): 427–436. doi:10.1016/S0747-5632(01)00055-3.
- Junker, D., and C. Nollen. 2018.“ Mobile Eye Tracking in Landscape Architecture: Discovering a New Application for Research on Site.“ Landscape Architecture. The Sense of Places, Models and Applications; Almusaed, A., Ed, London: IntechOpen, 45–66.
- Kapur, A., A. Kapur, N. Virji-Babul, G. Tzanetakis, and P. F. Driessen. 2005. “Gesture-based Affective Computing on Motion Capture Data.” In International Conference on Affective Computing and Intelligent Interaction, Beijing, China, 1–7.
- Karakas, T., and D. Yildiz. 2020. “Exploring the Influence of the Built Environment on Human Experience through A Neuroscience Approach: A Systematic Review.” Frontiers of Architectural Research 9 (1): 236–247. doi:10.1016/j.foar.2019.10.005.
- Kiefer, P., I. Giannopoulos, M. Raubal, and A. Duchowski. 2017. “Eye Tracking for Spatial Research: Cognition, Computation, Challenges.” Spatial Cognition & Computation 17 (1–2): 1–19. doi:10.1080/13875868.2016.1254634.
- Kreibig, S. D. 2010. “Autonomic Nervous System Activity in Emotion: A Review.” Biological Psychology 84 (3): 394–421. doi:10.1016/j.biopsycho.2010.03.010.
- Kuliga, S. F., T. Thrash, R. C. Dalton, and C. Hölscher. 2015. “Virtual Reality as an Empirical Research tool—Exploring User Experience in a Real Building and a Corresponding Virtual Model.” Computers, Environment and Urban Systems 54: 363–375. doi:10.1016/j.compenvurbsys.2015.09.006.
- Leavitt, J. 1988. Creating Architectural Theory: The Role of Behavioral Sciences in Environmental Design. New York: JSTOR.
- Lee, G., B. Choi, H. Jebelli, C. R. Ahn, and S. Lee. 2020. “Wearable Biosensor and Collective sensing–based Approach for Detecting Older Adults’ Environmental Barriers.” Journal of Computing in Civil Engineering 34 (2): 04020002. doi:10.1061/(ASCE)CP.1943-5487.0000879.
- Levenson, R. W. 2014. “The Autonomic Nervous System and Emotion.” Emotion Review 6 (2): 100–112. doi:10.1177/1754073913512003.
- Levenson, R. W., P. Ekman, K. Heider, and W. V. Friesen. 1992. “Emotion and Autonomic Nervous System Activity in the Minangkabau of West Sumatra.” Journal of Personality and Social Psychology 62 (6): 972. doi:10.1037/0022-3514.62.6.972.
- Li, Y., and A. S. Elmaghraby. 2014. “A Framework for Using Games for Behavioral Analysis of Autistic Children.“ In 2014 Computer Games: Al, Animation, Mobile, Multimedia, Educational and Serious Games (CGAMES), Louisville, KY, USA, 1–4.
- Li, Y., A. S. Elmaghraby, A. El-Baz, and E. M. Sokhadze. 2015. “Using Physiological Signal Analysis to Design Affective VR Games.” In 2015 IEEE International Symposium on Signal Processing and Information Technology (ISSPIT), Abu Dhabi, United Arab Emirates, 57–62.
- Li, J., Y. Jin, S. Lu, W. Wu, and P. Wang. 2020. “Building Environment Information and Human Perceptual Feedback Collected through a Combined Virtual Reality (VR) and Electroencephalogram (EEG) Method.” Energy and Buildings 224: 110259. doi:10.1016/j.enbuild.2020.110259.
- Liu, Y., F. Castronovo, J. Messner, and R. Leicht. 2020. “Evaluating the Impact of Virtual Reality on Design Review Meetings.” Journal of Computing in Civil Engineering 34 (1): 04019045. doi:10.1061/(ASCE)CP.1943-5487.0000856.
- López-Tarruella Maldonado, J., J. L. Higuera Trujillo, S. Iñarra Abad, M. A. Carmen Llinares Millán, J. Guixeres Provinciales, and M. A. Raya. 2016. “Virtual Reality as a Tool for Emotional Evaluation of Architectural Environments.“ In Congreso Internacional de Expresión Gráfica Arquitectónica, Alcalá de Henares, Spain, 889–903.
- Marín-Morales, J., J. L. Higuera-Trujillo, A. Greco, J. Guixeres, C. Llinares, E. P. Scilingo, M. Alcañiz, and G. Valenza. 2018. “Affective Computing in Virtual Reality: Emotion Recognition from Brain and Heartbeat Dynamics Using Wearable Sensors.” Scientific Reports 8 (1): 1–15. doi:10.1038/s41598-018-32063-4.
- Mauss, I. B., and M. D. Robinson. 2009. “Measures of Emotion: A Review.” Cognition and Emotion 23 (2): 209–237. doi:10.1080/02699930802204677.
- Mooney, T. 2012. Phenomenology of Perception. Milton Park, Abingdon-on-Thames, Oxfordshire, England, UK: Routledge.
- Nikolic, D. 2007. “Evaluating Relative Impact of Virtual Reality Components Detail and Realism on Spatial Comprehension and Presence.” PhD Thesis, Pennsylvania State University.
- Paes, D., E. Arantes, and J. Irizarry. 2017. “Immersive Environment for Improving the Understanding of Architectural 3D Models: Comparing User Spatial Perception between Immersive and Traditional Virtual Reality Systems.” Automation in Construction 84: 292–303. doi:10.1016/j.autcon.2017.09.016.
- Park, B. 2009. “Psychophysiology as a Tool for HCI Research: Promises and Pitfalls.” International Conference on Human-Computer Interaction, Springer, Berlin, Heidelberg, 5610: 141–148. doi:https://doi.org/10.1007/978-3-642-02574-7_16.
- Pei, W., X. Guo, and T. Lo. 2021a. “Biofeedback in the Dynamic VR Environments: A Method to Evaluate the Influence of Architectural Elements on Human Spatial Perception.” 2021 Workshop on Algorithm and Big Data, Fuzhou China, 27–33.
- Pei, W., X. Guo, and T. Lo. 2021b. “Detecting Virtual Perception Based on Multi-Dimensional Biofeedback-A Method to Pre-Evaluate Architectural Design Objectives.“ In The 26th CAADRIA Conference, Chinese University of Hong Kong and Online, Hong Kong, 2: 183–192 .
- Plutchik, R, Kellerman, H. 1980. “A General Psychoevolutionary Theory of Emotion.” In Theories of Emotion, 3–33. Cambridge, Massachusetts, United States: Academic Press.
- Porges, S. W. 1995. “Cardiac Vagal Tone: A Physiological Index of Stress.” Neuroscience & Biobehavioral Reviews 19 (2): 225–233. doi:10.1016/0149-7634(94)00066-A.
- Radwan, A., and S. Ergan. 2017. “Quantifying Human Experience in Interior Architectural Spaces . “In Computing in Civil Engineering 2017 33: 373–380. doi:10.1061/(ASCE)CP.1943-5487.0000812.
- Rapoport, A. 1990. “The Meaning of the Built Environment: A Nonverbal Communication Approach.“ Tucson, Arizona, United States: University of Arizona Press.
- Rapoport, A. 2016. “Human Aspects of Urban Form: Towards a man—environment Approach to Urban Form and Design.“ Oxford, United Kingdom: Pergamon press.
- Rogge, E., F. Nevens, and H. Gulinck. 2007. “Perception of Rural Landscapes in Flanders: Looking beyond Aesthetics.” Landscape and Urban Planning 82 (4): 159–174. doi:10.1016/j.landurbplan.2007.02.006.
- Rouch, J. 1985. “The Cinema of the Future?” Studies in Visual Communication 11 (1): 30–37. doi:10.1111/j.2326-8492.1985.tb00015.x.
- Russell, J. A. 1980. “A Circumplex Model of Affect.” Journal of Personality and Social Psychology 39 (6): 1161. doi:10.1037/h0077714.
- Skorupka, A. 2009. “Comparing Human Wayfinding Behavior in Real and Virtual Environment.” Proceedings of the 7th International Space Syntax Symposium 104: 1–7.
- Soltani, S., and N. Kirci. 2019. “Phenomenology and Space in Architecture: Experience, Sensation and Meaning.” International Journal of Architectural Engineering Technology 6: 1–6. doi:10.15377/2409-9821.2019.06.1.
- Spence, C. 2020. “Senses of Place: Architectural Design for the Multisensory Mind.” Cognitive Research: Principles and Implications 5 (1): 1–26. doi:10.1186/s41235-019-0201-4.
- Tang, M. 2021. “Visual Perception: Eye-tracking and Real-time Walkthroughs in Architectural Design.” International Journal of Architecture, Engineering and Construction 10 (1): 1–9. doi:10.7492/IJAEC.2021.001.
- Wac, K., and C. Tsiourti. 2014. “Ambulatory Assessment of Affect: Survey of Sensor Systems for Monitoring of Autonomic Nervous Systems Activation in Emotion.” IEEE Transactions on Affective Computing 5 (3): 251–272. doi:10.1109/TAFFC.2014.2332157.
- Westerdahl, B., K. Suneson, C. Wernemyr, M. Roupé, M. Johansson, and C. M. Allwood. 2006. “Users’ Evaluation of a Virtual Reality Architectural Model Compared with the Experience of the Completed Building.” Automation in Construction 15 (2): 150–165. doi:10.1016/j.autcon.2005.02.010.
- Wingler, D., A. Joseph, S. Bayramzadeh, and A. Robb. 2020. “Using Virtual Reality to Compare Design Alternatives Using Subjective and Objective Evaluation Methods.” HERD: Health Environments Research & Design Journal 13 (1): 129–144. doi:10.1177/1937586719851266.
- Yadav, M., T. Chaspari, J. Kim, and C. R. Ahn. 2018. “Environment.” In Proceedings of the Workshop on Human-Habitat for Health (H3): Human-Habitat Multimodal Interaction for Promoting Health and Well-Being in the Internet of Things Era, New York, NY, United States, 1–8.
- Yousefi, M. V., E. Karan, A. Mohammadpour, and S. Asadi. 2015. “Implementing Eye Tracking Technology in the Construction Process.” In 51st ASC Annual International Conference Proceedings, College Station, TX: Associated Schools of Construction, 752–759.
- Zeile, P., B. Resch, J.-P. Exner, and G. Sagl. 2015. “Urban Emotions: Benefits and Risks in Using Human Sensory Assessment for the Extraction of Contextual Emotion Information in Urban Planning.” Geertman, S., Ferreira, J., Goodspeed, R., Stillwell, J. eds. In Planning Support Systems and Smart Cities, 209–225. Switzerland: Springer.
- Zhang, L.-M., T.-S. Jeng, and R.-X. Zhang. 2018. “Integration of Virtual Reality, 3-D eye-tracking, and Protocol Analysis for re-designing Street Space.” In the 23rd CAADRIA Conference, Tsinghua University, Beijing, China, 431–440.
- Zimmerman, B. J., and A. Kitsantas . 2005. “The Hidden Dimension of Personal Competence: Self-Regulated Learning and Practice.”Handbook of competence and motivation, 509–526.
- Zou, H., N. Li, and L. Cao. 2017. “Emotional response–based Approach for Assessing the Sense of Presence of Subjects in Virtual Building Evacuation Studies.” Journal of Computing in Civil Engineering 31 (5): 04017028. doi:10.1061/(ASCE)CP.1943-5487.0000679.