ABSTRACT
At present, people use a large amount of coal, oil and other energy while causing a series of environmental pollution problems. In response to the national 3060 policy, improving the utilization of solar energy is imminent. Solar photovoltaic air collector can not only collect heat efficiently but also reduce the surface temperature of solar cells to improve the photoelectric conversion efficiency. The research progress of solar photovoltaic air collector system improvement and application scenarios are reviewed. The theoretical and experimental research of solar photovoltaic air collector in improving photoelectric/photothermal conversion efficiency is analyzed, the current application scenarios and advantages of solar photovoltaic air collector are discussed, and the research direction of solar photovoltaic air collector is prospected.
1. Introduction
Nowadays, there are two main forms of solar energy utilization: solar photovoltaic power generation and solar thermal energy utilization. Among them, the photoelectric conversion efficiency of solar photovoltaic power generation is 10%–15%, and the photothermal conversion efficiency of solar photothermal utilization is 30%–40%. When the two systems operate independently, the utilization rate of solar energy is low. The technology combining solar photovoltaic power generation and solar photothermal utilization is called solar photovoltaic photothermal comprehensive utilization technology. The photoelectric/photothermal conversion efficiency of solar energy will be improved, and the comprehensive utilization rate of solar energy can reach more than 60% (Sultan and Efzan Citation2018). Solar photovoltaic photothermal comprehensive utilization technology can be roughly divided into solar photovoltaic air collector and solar photovoltaic hot water collector by medium (Assoa et al. Citation2007). Solar photovoltaic air collector (Choi and Choi Citation2020) has low pressure drop, simple structure, low operating cost and can be effectively integrated into the building (Barone et al. Citation2019). In the same environment, compared with the solar photovoltaic hot water system (Hazi et al. Citation2014), the photothermal conversion efficiency of the solar photovoltaic air collector is about 10% higher than that of the solar photovoltaic hot water system, while the photoelectric conversion efficiency is only 0.4% lower than that of the solar photovoltaic hot water system (Guo et al. Citation2017).
2. Numerical simulation of solar photovoltaic air collector
Most studies in this field focus on mathematical models and simulations, and the electrical, thermal and exergic properties of solar photovoltaic air collectors are evaluated by establishing physical models or simulations (Yazdanifard and Ameri Citation2018).
Cox and Raghuraman (Citation1985) used simulation software to study the performance of solar photovoltaic air collectors with photovoltaic cells with special coating, focusing on the influence of optical characteristics of the coating on photovoltaic cells on the collector’s photothermal conversion efficiency. They found that placing indium tin oxide (ITO) layers on photovoltaic cells increased the system’s photothermal conversion efficiency from 34% to 39%.
Sopian et al. (Citation1996) studied the performance of single-channel and double-channel solar photovoltaic air collectors by establishing a mathematical model. Due to the increased heat transfer area between the air and the photovoltaic cell, the photoelectric/photothermal conversion efficiencies of dual-channel collectors are 8–9% and 32–34%, while those of single-channel collectors are 6–7% and 24–28%, and the photoelectric/photothermal efficiency increases about 2% and 8%, respectively.
Anand and Tiwari (Citation1996) established a mathematical model of a flat-panel solar photovoltaic air collector through the climatic conditions of India (Srinagar) for 4 years (1998–2001). Results: The photothermal conversion efficiency and photoelectric conversion efficiency of the system are about 60% and 14.5%, respectively.
Sarhaddi, Farahat, and Ajam (Citation2010) studied exergic performance of a typical flat-panel solar photovoltaic air collector by establishing a mathematical model. Based on the structural parameters and climatic parameters, the thermal loss coefficient was corrected and the improved exergic efficiency formula was derived. By comparing the formula with the experimental data, the photothermal/photoelectric conversion efficiency and exergic efficiency of the collector were 17.18%, 10.01% and 10.75%, respectively.
Kamthania, Nayak, and Tiwari (Citation2010) established a mathematical model and solved it by MATLAB software to evaluate the performance of dual-channel and single-channel solar photovoltaic air collectors under quasi-steady-state conditions and the climate of New Delhi, India. The results show that the air outlet temperature of dual-channel solar photovoltaic air collector is 3–4°C higher than that of single-channel solar photovoltaic air collector.
Khelifa et al. (Citation2015) conducted a numerical study on the overall performance of solar photovoltaic air collector by using ANSYS software. The influence of climatic parameters such as solar radiation and wind speed on collector performance is analyzed. The photoelectric/photothermal conversion efficiency of the collector is highly dependent on the received solar radiation and the mass flow rate of the air.
Elsaf and Gandhidasan (Citation2015) established a mathematical model to study the influence of different fin designs on the overall efficiency of dual-channel solar photovoltaic air collectors. The simulation results show that when the number of fins increases from 10 to 50, the photovoltaic cell surface temperature decreases from 43°C to 36°C, and the photoelectric conversion efficiency increases about 2%.
Al-Damook et al. (Citation2018) used COMSOL Multiphysics software to analyze the electrical/thermal performance of a solar photovoltaic air collector with fins. Through simulation, it can be concluded that the fin has significant influence on the photoelectric conversion efficiency and the photothermal conversion efficiency of the system. The maximum comprehensive utilization rate of solar energy is 84.7% for the solar photovoltaic air collector with fins and 51.2% for the finless system.
Numan Özakin and Kaya (Citation2019) used ANSYS software to simulate the temperature field on the surface of a photovoltaic panel with a solar photovoltaic air collector with fins and the velocity field of heat transfer medium and compared with the experimental data. Through simulation, the photothermal conversion efficiency of the solar photovoltaic air collector with fins is increased by about 55%.
In summary, increasing the air mass flow can effectively improve the convective heat transfer effect, the air temperature at the collector outlet decreases, and the surface temperature of the photovoltaic panel also decreases, thus improving the photoelectric/photothermal conversion efficiency. The change of air flow section can effectively improve the heat transfer area and convective heat transfer disturbance and then improve the comprehensive utilization rate of solar energy of photovoltaic air collector.
3. Experimental study on structure optimization of solar photovoltaic air collector
At the same time of the numerical simulation of the solar photovoltaic air collector, a large number of experimental studies have been carried out to optimize the performance of the solar photovoltaic air collector. It is found that photovoltaic cell, glass cover plate, flow channel form, filling of multi-space medium and reasonable arrangement of fins are important structural parameters that affect the photoelectric/photothermal conversion efficiency of the system. Straight fin, V-shaped groove, baffle plate and other fin structures can change the air flow state and increase the heat transfer area, which can effectively improve the performance of solar photovoltaic air collector (Diwania et al. Citation2020; Schindler et al. Citation2017; Babu and Ponnambalam Citation2017). In this section, the experimental research on structural optimization of solar photovoltaic air collectors based on efficiency improvement is reviewed.
3.1. Photovoltaic cell
Photovoltaic cell is the core component of solar photovoltaic air collector, and photoelectric conversion is realized by photovoltaic cell. Photovoltaic cells absorb heat when working, and the efficiency of photoelectric conversion decreases by about 0.3% for every 1°C increase in temperature. Therefore, regulating the surface temperature of photovoltaic cells is the key to improve the photoelectric conversion efficiency of photovoltaic air collector.
Saygin et al. (Citation2017) conducted an experimental study on the effect of different spacing between glass cover plates and photovoltaic cells on performance in a two-channel solar photovoltaic air collector, as shown in . They set the intervals between the photovoltaic cells and the glass cover at 3 cm, 5 cm and 7 cm. It is found that when the distance between photovoltaic module and glass cover plate is 3 cm, the photovoltaic module has the highest photothermal conversion efficiency of 48%, followed by 39% for 7 cm, and the lowest time thermal conversion efficiency of 34% for 5 cm.
Figure 1. Improved PV/T solar air collector (Saygin et al. Citation2017).
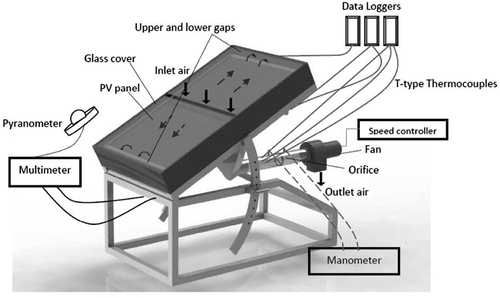
Wu et al. (Citation2019) studied the effect of photovoltaic cell location on the photoelectric/photothermal conversion efficiency of solar photovoltaic air collector. They placed photovoltaic cells on the surface of an insulated backplane (option 1) and on the back of a glass cover plate (option 2), as shown in . The results show that the photovoltaic panel temperature in scheme 2 is lower than that in scheme 1, the photoelectric conversion efficiency of scheme 2 is 14.8%, and that of Scheme 1 is 13.5%. The photothermal conversion efficiency of scheme 1 is 52%, and scheme 2 is 24%.
Figure 2. Two models of solar photovoltaic air collectors with different photovoltaic cell placement (Wu et al. Citation2019).
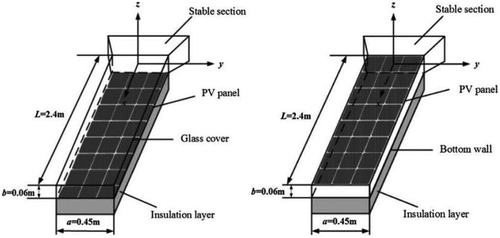
Hegazy et al. (Citation2020) experimentally studied three kinds of solar photovoltaic air collectors with different structures. They placed photovoltaic cells on an absorbing plate (Plan 1), on a glass cover plate (Plan 2) or separated them from the collector (Plan 3). The heat loss of scheme 3 is 7.5% and 29% higher than that of scheme 1 and Scheme 2, respectively, and the photothermal conversion efficiency of the three schemes is 53%, 27% and 64%, respectively.
In summary, analyzes and compares the effects of different photovoltaic cell positions and flow rates on the efficiency of solar photovoltaic air collectors. It can be concluded that the photovoltaic cell can be set above/below the air passage, and when the photovoltaic cell is placed below the air passage, the air will not only cool the photovoltaic panel, but also receive sunlight to get heat, and the photothermal conversion efficiency ratio can be increased by about 20% when set above. Different distances between photovoltaic cells, glass cover plates and insulation backplane will lead to changes in flow velocity and flow state (natural convection and forced convection) of convective heat transfer air, which will affect the photoelectric/photothermal conversion efficiency of collectors. However, the influencing mechanism needs to be further studied.
Table 1. Comparison table of the effects of different photovoltaic cell positions on efficiency.
3.2. Glass cover-plate
The glass cover plate is an integral part of the solar photovoltaic air collector, which can reduce the influence of the external environment on the system. Its performance parameters (surface condition, absorptivity, reflectivity and penetration) will affect the performance of the collector.
Riffat and Cuce (Citation2011) studied the influence of whether a glass cover plate is installed on collector performance. A flat-type solar photovoltaic air collector equipped with a single-layer glass cover plate was used in the experimental device. The study found that the installation of glass cover plates on photovoltaic panels would lead to an increase in surface temperature of photovoltaic cells of about 8°C.
Shahsavar, Ameri, and Gholampour (Citation2012) studied the performance difference of solar photovoltaic air collector with 5 mm thick glass cover plate and without glass cover plate, as shown in . It is found that the photothermal conversion efficiency of the collector increases by about 12%, but the photoelectric conversion efficiency of the photovoltaic cell will be affected by the temperature increase, and the operating voltage and current of the photovoltaic panel will decrease, and the photoelectric conversion efficiency will decrease by about 1.5%. The best photothermal conversion efficiency and total efficiency can be obtained by using glass cover plate, while higher photoelectric conversion efficiency can be obtained without using glass cover plate (Dupeyrat, Menezo, and Rommel Citation2011; Chow Citation2010).
Figure 3. Solar photovoltaic air collector with glass cover plate (Shahsavar, Ameri, and Gholampour Citation2012).
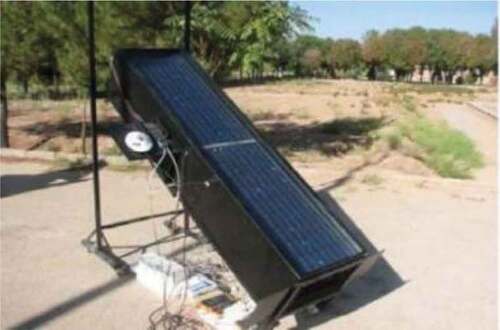
Combined with the relevant research on glass cover plate of solar photovoltaic air collector (Jeong et al. Citation2020; Panchal Citation2016), it can be seen that the number, number of layers and specific position of glass cover plate can directly affect the collector performance output due to its high penetration rate and high absorption rate of photoelectric conversion spectrum in sunlight. The setting of multi-layer glass cover plate has less influence on the photoelectric conversion rate than on the photothermal conversion rate. Under the condition of effectively reducing the external influence (reducing heat loss), the ratio of photothermal and photoelectric output should be comprehensively measured and the number of layers of glass cover plate should be set reasonably to obtain higher energy output. At the same time, it is also one of the future research directions to study the influence of different parameters (surface condition, absorption rate, reflectance and penetration rate) of glass cover plate on the system performance.
3.3. Port form
The air passage in solar photovoltaic air collector is divided into single passage and double passage. The air in the flow passage passes over the surface of photovoltaic panels and heat absorption panels, taking away the heat energy for use. Different flow passage forms have different photothermal conversion efficiency.
Ooshaksaraei et al. (Citation2017) conducted experimental studies on four kinds of solar photovoltaic air collectors. They made four devices, namely single-channel flow, double-channel co-flow, double-channel reverse flow and single-channel backflow, as shown in . The total energy efficiency is 28%–49%, 51%–67%, 47%–62% and 42%–56%, respectively. It can be seen that the total energy efficiency of the structure with two-channel flow in the same direction is the highest.
Figure 4. Four kinds of solar photovoltaic air collectors (Ooshaksaraei et al. Citation2017).
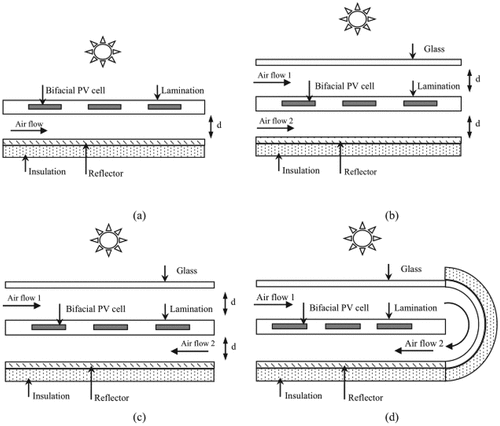
Slimani et al. (Citation2017) analyzed the influence of different flow channel forms on solar photovoltaic air collectors. They set up four systems with different structures, as shown in : Scheme 1 has no flow channels; Scheme 2 is single flow channel; Scheme 3 is single flow channel, but air gap is set between solar cell and glass cover plate; Scheme 4 is single-channel return flow, in which air enters through the lower flow passage and is sent out through the upper flow passage. The solar cell temperatures of the four solar photovoltaic air collectors were 54°C, 48°C, 60°C and 49°C, respectively. Scheme 4 had the highest photothermal conversion efficiency, and Scheme 2 had the highest photoelectric conversion rate.
Figure 5. Solar photovoltaic air collectors with different flow channel forms (Slimani et al. Citation2017).
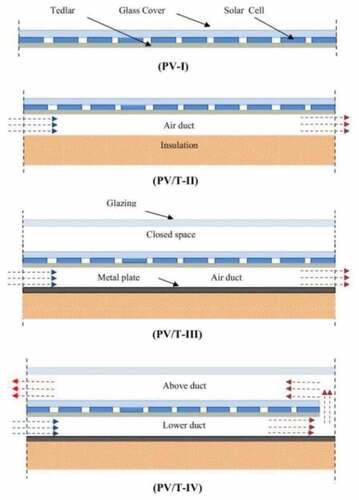
analyzes and compares the effects of different flow channel forms and flow rates on the efficiency of solar photovoltaic air collectors. To sum up, the flow channel forms of solar photovoltaic air collectors can be divided into single flow channel, double flow channel in the same direction, double flow channel reverse flow and single flow channel reflux. The main influencing factors of different flow channel forms on the photothermal conversion efficiency are that the heat transfer area is increased and the average temperature difference of heat transfer is larger. The strengthening of heat transfer enables the temperature of photovoltaic cells to be effectively controlled, and the photoelectric conversion efficiency is also improved synchronously. Since the heat transfer area of the latter three channels is much larger than that of the single channel, it will have higher photoelectric/photothermal conversion efficiency, and its comprehensive utilization rate of solar energy can be improved by 10–20%. Compared with the flow in the same direction and reverse direction of the double flow channel, the temperature difference between the upper and lower flow channels increases due to the reverse flow, and the temperature distribution in the flow channel is uneven, the average air temperature increases, and the heat transfer temperature difference decreases, which ultimately affects the heat transfer performance and photovoltaic cell temperature. Different flow channel structures can improve the air heat transfer area and heat transfer temperature difference in the flow channel, so the optimization of flow channel structure and its heat transfer mechanism will become one of the research hotspots in the future.
Table 2. Comparison of the effects of different flow channel forms on efficiency.
3.4. Porous media
The porous medium has the advantages of low relative density, large surface area, light weight, good permeability and high nominal thermal conductivity. It can be filled into the solar photovoltaic air collector to increase the heat transfer area.
Sopian et al. (Citation2009) studied a dual-channel solar photovoltaic air collector, in which porous media with different porosity were set in the lower side channel of the solar cell. The results show that the photothermal conversion efficiency of the solar photovoltaic air collector with porous media can be increased by 10%, and the higher the porosity of porous media, the higher the photothermal conversion efficiency.
Ahmed and Mohammed (Citation2017) studied the effect of porous media on the performance of dual-channel solar photovoltaic air collector. They placed porous media (glass spheres) in the lower flow channel of the collector, and the porosity was 0.437, as shown in . The results show that the pressure drop increases with the adoption of porous media, and the photothermal conversion efficiency of the collector using porous media is 80.23%, while that of the collector without porous media is only 51.25%.
Figure 6. Set up the device model for the porous media (Ahmed and Mohammed Citation2017).
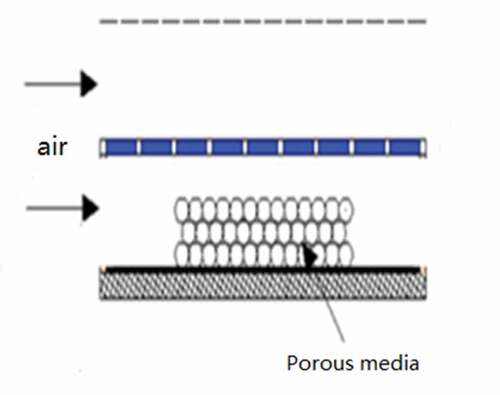
In conclusion, the use of porous media can effectively increase the heat transfer area and improve the photothermal conversion efficiency of the solar photovoltaic air collector. However, the existing research on the key factors affecting the performance output is not thorough enough. The research can be mainly carried out in the following aspects: research on the influence of different thermal conductivity and porosity of filled media; Optimization of the relationship between filling medium and flow channel structure under the balance of air flow resistance and heat transfer enhancement; Study on the low thermal resistance of the interconnecting part between the filling medium and the collector.
3.5. Fin
The results show that the fin structure can change the air flow state and increase the heat transfer area, which can effectively improve the performance of solar photovoltaic air collector.
Bambrook and Sproul (Citation2012) designed a solar photovoltaic air collector with a parallel runner to ensure uniform distribution of airflow in the runner (). The experimental results show that the operating temperature of the solar cell can be kept at 38°C, and the photoelectric conversion efficiency can reach 12.5%. When the mass flow rate of air exceeds 0.055 kg/s, the photoelectric/photothermal conversion efficiency does not change.
Figure 7. Solar photovoltaic air collector with parallel runner (Bambrook and Sproul Citation2012).
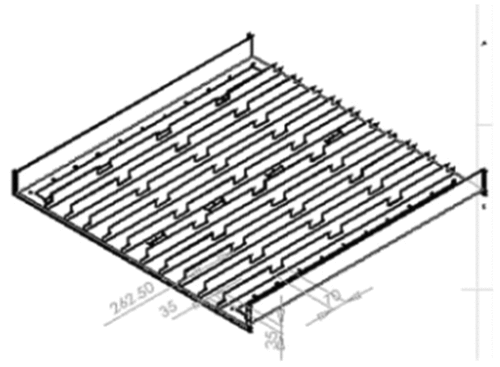
Mojumder et al. (Citation2016) proposed a single-channel solar photovoltaic air collector with rectangular fins in the flow channel, which were in the same direction as the air flow direction. The experiments recorded the temperature of the upper and back surfaces of photovoltaic modules, the rear wall of the system and the inlet and outlet of the system under different fin number (0–4), mass flow rate (0.02 kg/s – 0.14 kg/s) and solar radiation (200 w/m2-700 w/m2). It was found that adding rectangular fins could improve the performance of photovoltaic air collector. Its photoelectric/photothermal conversion efficiency is 13.75% and 56.19%, respectively.
Touafek et al. (Citation2018) carried out simulation and experimental study on a new type of solar photovoltaic air collector. The photovoltaic cells inside the collector are arranged on a metal plate painted black to increase the solar radiation absorption capacity of the heat absorption plate. Rectangular fins are also provided on the heat absorption plate as air channels. The experimental results show that the current intensity generated by the photovoltaic cell in the collector is 7–30% higher than that of the simple photovoltaic system.
Franklin and Chandrasekar (Citation2019) added vertical fins to the runner of a solar photovoltaic air collector. The results show that the maximum air outlet temperatures of the collectors with and without fins are 34°C and 30.7°C, respectively, in the climate of Tamil Nadu (India).
Jha, Das, and Gupta (Citation2019) conducted an experimental comparison of solar photovoltaic air collectors with flat and wave plates as heat absorption panels. By setting different flow rates, it was found that the photothermal conversion efficiency of wave plate solar photovoltaic air collector was 3–5.5% higher than that of plate type, and the exergic efficiency was 1–1.2% higher.
Choi and Choi (Citation2020) made a solar photovoltaic air collector with dual channels, and they set a set of unequal section fins on the back of the photovoltaic module, as shown in . With the increase of air mass flow rate, the photothermal conversion efficiency of solar photovoltaic air collector increases from 35.2% to 56.72%, and the photoelectric conversion efficiency increases from 14.23% to 14.81%.
Figure 8. Schematic diagram of solar photovoltaic air collector with unequal section fins (Choi and Choi Citation2020).
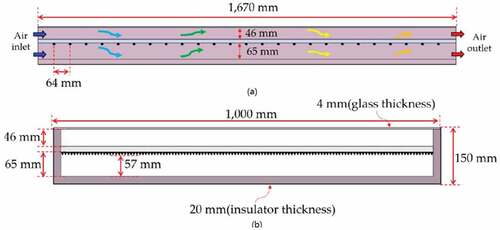
Yu, Kim, and Kim (Citation2020) verified the influence of triangular baffle arrangement on solar photovoltaic air collector by staggered triangular baffle arrangement in the flow passage, as shown in . The results show that the thermal performance of solar photovoltaic air collectors increases with the increase of horizontal distance between triangular baffles and the decrease of vertical distance. The more baffles there are, the higher the photothermal conversion efficiency of solar photovoltaic air collectors. Compared with ordinary collectors, the outlet temperature of the solar photovoltaic air collector with triangular baffle plate increases by 3.6–3.9°C, and the photothermal conversion efficiency increases by 31%.
Figure 9. Solar photovoltaic air collectors with triangular baffles (Yu, Kim, and Kim Citation2020).
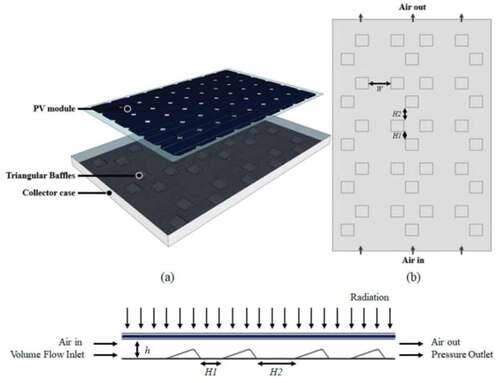
analyzes and compares the effects of fin form and flow rate on the efficiency of solar photovoltaic air collector. To sum up, the finned structures of different structures in the flow passage can increase the convective heat transfer area on the one hand, change the air flow state on the other hand, and affect the flow boundary layer morphology (boundary layer thinning, strengthen the disturbance in the boundary layer), so as to improve the heat transfer capacity. In addition, the addition of objects such as fins will lead to increased flow resistance, reducing air flow and increasing power consumption of the fan. Therefore, it is necessary to balance the contradiction between the finned structure (form and position) and the combination of finned and runner to enhance heat transfer and increase resistance and study a better finned structure to effectively increase boundary layer disturbance, reduce boundary layer thickness, reduce air resistance, and effectively improve the heat transfer effect of solar photovoltaic air collector.
Table 3. Comparison of the effects of different fin forms on efficiency.
At present, a large number of literatures have studied the structure of solar photovoltaic air collector. Photovoltaic cells, glass cover plates, flow channel forms, filling media, fins and other factors will affect the photoelectric/photothermal conversion efficiency of solar photovoltaic air collector. However, the coupling optimization among these factors still needs further research.
4. Applied research
In the past 20 years of development, photovoltaic photothermal technology has made some achievements in energy saving and improving the comprehensive utilization of solar energy, and photovoltaic photothermal technology has been applied in some scenes.
4.1. Dry crop
Solar photovoltaic air collectors have certain advantages in crop drying (Chauhan, Kumar, and Gupta Citation2017), and scholars have carried out some experimental studies on different crop drying scenarios.
Tiwari, Agrawal, and Tiwari (Citation2018) added a drying box after a solar photovoltaic air collector and studied potato chips of different thickness as drying objects. The results show that the integrated solar photovoltaic air collector drying system has better temperature controllability than other drying systems, and the forced convection mode has better drying performance for high moisture content crops, while the natural convection mode has better drying performance for low moisture content crops.
Hu et al. (Citation2018) proposed a solar photovoltaic air collector drying system, which was combined with a heat pump to dry 50 kg Lycium barbarum. The results show that the combined system saves 2.9 kW·h energy compared with the heat pump alone, and the energy saved accounts for 29.5% of the energy consumed by the heat pump alone. At the same time, the combined system has a higher dehumidification energy consumption ratio, and the maximum difference between them is 0.71 kg/(kW·h).
Fterich et al. (Citation2018) developed a forced convection solar photovoltaic air dryer and conducted an experimental study on its performance. The system consists of a solar photovoltaic air collector (PV/T) and a drying chamber. By drying tomatoes in the equipment and comparing with naturally dried samples, they found that after 44 h of drying, the water content of tomatoes in the equipment was 8.17% lower than that in natural drying.
Kong et al. (Citation2020) built a solar hot air drying system based on solar photovoltaic air collector, as shown in . The collector is equipped with two flow channels, on which are crystal silicon solar cells (Scheme 1) and amorphous silicon solar cells (Scheme 2). The results show that the maximum air outlet temperature of scheme 1 is 69.72°C, and that of Scheme 2 is 75.56°C. The photothermal conversion efficiency of scheme 1 and Scheme 2 is 40.7% and 46.8%, and the photoelectric conversion efficiency is 6.8% and 5.7%, respectively.
Figure 10. Solar hot air drying system (Tiwari, Agrawal, and Tiwari Citation2018).
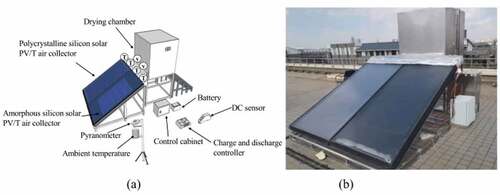
In summary, solar photovoltaic air collector’s energy output characteristics of “combined heat and power generation” and drying characteristics of different crops are combined. Solar photovoltaic air collector’s drying of crops is not only limited to the above research scenarios but also can continue to carry out research work in combination with its advantages. First, good temperature control performance. Adjust air flow by changing fan power and accurately control drying temperature. Second, good moisture control performance. It is easy to combine a variety of air treatment forms, such as electric drive wheel dehumidification, electric drive refrigeration table cooler dehumidification, electric heating and other forms to achieve accurate humidity control. Third, the energy efficiency ratio is high, the electric energy can be used for the fan, but also can drive the heat pump, improve the quality and quantity of heat energy output.
4.2. The building integrates air photovoltaic/thermal (A-BIPV/T) systems
The system combining solar photovoltaic air collector and building is called building integrated air PHOTOVOLTAIC/thermal system, short for A-BIPV/T system (Tian and Zhao Citation2013). Usually, the collector is placed on the exterior wall or roof. Without affecting the lighting and ventilation functions of the building, increasing the irradiation area of the collector is also conducive to improving the photoelectric/photothermal conversion efficiency of the collector.
Ahn, Kim, and Kim (Citation2015) discussed the feasibility of integrating solar photovoltaic air collectors on building roofs. The BIPV/T system is combined with a ventilation system to improve air quality inside the building.
Rounis, Athienitis, and Stathopoulos (Citation2016) compared the performance of BIPV/T systems with single and multiple entrances. The study used summer and winter data and adjusted system air volume to evaluate system performance. The results show that the multi-inlet BIPV/T system can improve the electrical efficiency by 1%. This increase increases the overall power output of the 120 kW pv system by 7% and the photothermal conversion efficiency by 24%.
Agathokleous, Kalogirou, and Karellas (Citation2018) carried out theoretical analysis and experimental studies on integrated air photovoltaic/thermal systems in buildings. They placed photovoltaic panels on the exterior walls and left a thick air gap between them as air channels. The efficiency of the system is 26.5%–33.5%, exergic efficiency is 13%–16%. The air flows through the gap to exchange heat with the surface of the photovoltaic panel to reduce the temperature of the photovoltaic panel and improve the photovoltaic conversion efficiency of the solar cell. Part of the air will be sent into the room by the fan powered by the photovoltaic panel to bear part of the indoor heat load.
Shahsavar and Rajabi (Citation2018) analyzed the performance of a building integrated air photovoltaic/thermal system, . They installed solar photovoltaic air collectors on the roof and an all-air air-conditioning system in the attic, which combined to regulate indoor temperatures. The system can save 3038.83 kW·h of heat energy and 2259.64 kW·h of electric energy, reduce 5.94 tons of carbon dioxide emissions, and bear 32.52% of the heat load of ventilation heat load. On the one hand, the collector generates hot air into the all-air air conditioning system and plays a role of pre-heating the heat source. On the other hand, the COP (energy efficiency ratio) of the system can be effectively improved by combining the all-air air-conditioning system with the solar photovoltaic air collector.
Figure 11. Building integrated photovoltaic/thermal system (Shahsavar and Rajabi Citation2018).
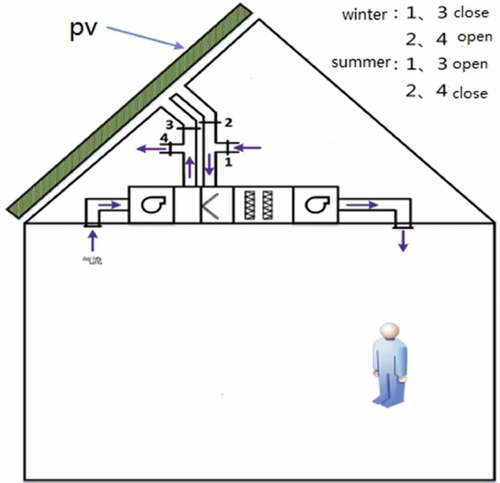
Fuentes (Citation2018) installed building integrated air PV/thermal systems and PV systems on the roofs of commercial buildings. The results show that the single photovoltaic system and the building integrated air photovoltaic/thermal system have good photoelectric conversion efficiency, with the efficiency range of 16.1–19.1% and 15.3–18.2%, respectively. Due to the lack of optimization between the building integrated air photovoltaic/thermal system and the building structure, the heat transfer efficiency of air flowing in the lower side of the photovoltaic panel is low, and the temperature drop of the photovoltaic cell surface is small. The researchers point out that the system can be improved by increasing the air flow or increasing the heat transfer area.
Ji-Suk, Kim, and Kim (Citation2019) discussed the performance of a BIPV/T system installed on the facade of an educational research facility in South Korea and used to heat the air in the building classrooms.
To sum up, the building integrated air photovoltaic/thermal system organically combines the building design with the solar photovoltaic air collector, which not only solves the problem of the collector site but also provides a new scene mode for building energy saving and energy supply. Future research will focus, on the one hand, how to efficiently and rationally use the building space, flexible use of natural ventilation and heat insulation and other means to reduce building energy consumption. On the other hand, the reasonable arrangement of solar photovoltaic air collectors can improve the utilization rate of solar energy, enhance the photoelectric/photothermal conversion efficiency, and increase the proportion of renewable energy in the building.
5. Conclusion
The mathematical model and experimental research progress of solar photovoltaic air collector in recent years are reviewed. After summarizing the research results of some application scenarios, there is still a lot of space for further research on application scenarios. The author thinks that, in the research on the key structure theory and experimental foundation at the same time, the future research should be around of photovoltaic solar air collector solar quality, natural environment characteristics of the specific application scenario, can use intensity, time and quality characteristics (such as energy output is given priority to with heat, or is given priority to with electricity, mainly hot, summer or winter is given priority to with electricity, etc.). For the purpose of building energy conservation, multiple means should be taken into account to integrate the surrounding building structure conditions and carry out the application research of structural optimization with consideration of practicality and economy oriented to improve the photothermal/photoelectric conversion efficiency.
Disclosure statement
No potential conflict of interest was reported by the author(s).
Additional information
Funding
Notes on contributors
Liang Pan
Liang Pan: Associate professor, Master supervisor, Changchun Institute of Technology
Rundong Zhang
Rundong Zhang: Master student in Changchun Institute of Technology
Xinping Wang
Xinping Wang: Doctoral candidate in Jilin University
References
- Agathokleous, R. A., S. A. Kalogirou, and S. Karellas. 2018. “Exergy Analysis of a Naturally Ventilated Building Integrated Photovoltaic/Thermal (BIPV/T) System.” Renewable Energy 128: 541–552. doi:10.1016/j.renene.2017.06.085.
- Ahmed, O. K., and Z. A. Mohammed. 2017. “Influence of Porous Media on the Performance of Hybrid PV/Thermal Collector.” Renewable Energy 112: 378–387. doi:10.1016/j.renene.2017.05.061.
- Ahn, J.-G., J.-H. Kim, and J.-T. Kim. 2015. “A Study on Experimental Performance of Air-Type PV/T Collector with HRV.” Energy Procedia 78: 3007–3012. doi:10.1016/j.egypro.2015.11.705.
- Al-Damook, M., D. Dixon-Hardy, P. J. Heggs, M. Al Qubeissi, J. Cottom. (2018). “CFD Analysis of a one-pass photovoltaic/thermal Air System with and without Offset Strip Fins.” MATEC Web of Conferences, 2018, 240, Cracow, POLAND.
- Anand, S. J., and A. Tiwari. 1996. “Energy and Exergy Efficiencies of a Hybrid photovoltaic–thermal (PV/T) Air Collector.” Renewable Energy 32 (14): 2223–2241.
- Assoa, Y. B., C. Menezo, G. Fraisse, R. Yezou, and J. Brau. 2007. “Study of a New Concept of photovoltaic-thermal Hybrid Collector.” Solar Energy 81 (9): 1132–1143. doi:10.1016/j.solener.2007.04.001.
- Babu, C., and P. Ponnambalam. 2017. “The Role of Thermoelectric Generators in the Hybrid PV/T Systems: A Review.” Energy Convers and Manag 151: 368–385. doi:10.1016/j.enconman.2017.08.060.
- Bambrook, S. M., and A. B. Sproul. 2012. “Maximising the Energy Output of a PVT Air System.” Solar Energy 86 (6): 1857–1871. doi:10.1016/j.solener.2012.02.038.
- Barone, G., A. Buonomano, C. Forzano, A. Palombo, and O. Panagopoulos. 2019. “Experimentation, Modelling and Applications of a Novel low-cost air-based Photovoltaic Thermal Collector Prototype.” Energy Conversion and Management 195 (95): 1079–1097. doi:10.1016/j.enconman.2019.04.082.
- Chauhan, P. S., A. Kumar, and B. Gupta. 2017. “A Review on Thermal Models for Greenhouse Dryers.” Renewable & Sustainable Energy Reviews 75: 548–558. doi:10.1016/j.rser.2016.11.023.
- Choi, H.-U., and K.-H. Choi. 2020. “Performance Evaluation of PV/T Air Collector Having a Single-Pass Double-Flow Air Channel and Non-Uniform Cross-Section Transverse Rib.” Energies 13 (9): 2203. doi:10.3390/en13092203.
- Chow, T. T. 2010. “A Review on photovoltaic/thermal Hybrid Solar Technology.” Applied Energy 87 (2): 365–379. doi:10.1016/j.apenergy.2009.06.037.
- Cox, C. H., III, and P. Raghuraman. 1985. “Design Considerations for flat-plate-photovoltaic/thermal Collectors.” Solar Energy 35 (3): 227–241. doi:10.1016/0038-092X(85)90102-1.
- Diwania, S., S. Agrawal, A. S. Siddiqui, and S. Singh. 2020. “Photovoltaic-thermal (PV/T) Technology: A Comprehensive Review on Applications and Its Advancement.” International Journal of Energy and Environmental Engineering 11 (1): 33–54. doi:10.1007/s40095-019-00327-y.
- Dupeyrat, P., C. Menezo, and M. Rommel. 2011. “Efficient Single Glazed Flat Plate photovoltaic-thermal Hybrid Collector for Domestic Hot Water System.” Solar Energy 85 (7): 1457–1468. doi:10.1016/j.solener.2011.04.002.
- Elsaf, A., and P. Gandhidasan. 2015. “Performance of a Photovoltaic or Thermal double-pass Solar Air Heater with Different Fin Configurations.” Clean Energy Technol 3 (1): 28–33. doi:10.7763/JOCET.2015.V3.163.
- Franklin, J. C., and M. Chandrasekar. 2019. “Performance Enhancement of a Single Pass Solar Photovoltaic Thermal System Using Staves in the Trailing Portion of the Air Channel.” Renewable Energy 135: 248–258. doi:10.1016/j.renene.2018.12.004.
- Fterich, M., M. Fterich, H. Bentaher, and A. Maalej. 2018. “Experimental Parametric Study of a mixed-mode Forced Convection Solar Dryer Equipped with a PV/T Air Collector.” Solar Energy 171: 751–760. doi:10.1016/j.solener.2018.06.051.
- Fuentes, M. 2018. “An Experimental Comparison between Commercial Hybrid PV-T and Simple PV Systems Intended for BIPV.” Renewable and Sustainable Energy Reviews 93: 110–120. doi:10.1016/j.rser.2018.05.021.
- Guo, C. H., J. Ji, W. Sun, and Y. Q. Wang. 2017. “Performance Study of multi-function Solar PV/T Collector.” Journal of Solar Energy 38 (2): 372–377.
- Hazi, A., G. Hazi, R. Grigore, and S. Vernica. 2014. “Opportunity to Use PVT Systems for Water Heating in Industry.” Solar Energy 63 (1): 151–157.
- Hegazy, M. M., A. El-Sebaii, M. R. Ramadan, S. Aboul-Enein, and A. Khallaf. 2020. “Comparative Study of Three Different Designs of a Hybrid PV/T double-pass Finned Plate Solar Air Heater.” Environmental Science and Pollution Research 27 (26): 32270–32282. doi:10.1007/s11356-019-07487-8.
- Hu, Y. D., W. He, S. C. Zhang, S. Lv, Z.T. Hu, H.C. Yu, M.H. Qin, J. Ji. 2018. “Design of a Solar-Air Source Heat Pump Drying System and Experimental Research on Drying Lycium Barbarum.” Advances In New And Renewable Energy 6 (2): 83–89.
- Jeong, H. J., Y. C. Kim, S. T. Kim, M. H. Choi, Y. H. Song, J.-H. Yun, M.-S. Park, et al. 2020. “Cu(In,Ga)Se-2 Solar Cells Integrated with Subwavelength Structured Cover Glass Fabricated by One-Step Self-Masked Etching.” Micromachines 11 (9): 877. DOI:10.3390/mi11090877.
- Jha, P., B. Das, and R. Gupta. 2019. “An Experimental Study of A Photovoltaic Thermal Air Collector (PVTAC): A Comparison of A Flat and the Wavy Collector.” Applied Thermal Engineering 163: 114344. doi:10.1016/j.applthermaleng.2019.114344.
- Ji-Suk, Y., J.-H. Kim, and J.-T. Kim. 2019. “A Study on the Thermal Performance of Air-Type BIPVT Collectors Applied to Demonstration Building.” Energies 12 (16): 3120. doi:10.3390/en12163120.
- Kamthania, D., S. Nayak, and G. N. Tiwari. 2010. “Energy and Exergy Analysis of a Hybrid Photovoltaic Thermal Double Pass Air Collector.” Applied Solar Energy 47 (3): 199–206. doi:10.3103/S0003701X11030066.
- Khelifa, A., K. Touafek, H. Ben Moussa, Ι. Tabet, H. Ben Cheikh Ei Hocine, H. Haloui. 2015. “Analysis of a Hybrid Solar Collector Photovoltaic Thermal (PVT)”. Energy Procedia 74: 835–843. doi:10.1016/j.egypro.2015.07.819.
- Kong, D. C., Y. F. Wang, M. Li, V. Keovisar, M. X. Huang, Q.F. Yu. 2020. “Experimental Study of Solar photovoltaic/thermal (PV/T) Air Collector Drying Performance”. Solar Energy 208: 978–989. doi:10.1016/j.solener.2020.08.067.
- Mojumder, J. C., W. T. Chong, H. C. Ong, K. Y. Leong, and Abdullah-Al-Mamoon. 2016. “An Experimental Investigation on Performance Analysis of Air Type Photovoltaic Thermal Collector System Integrated with Cooling Fins Design.” Energy and Buildings 130: 272–285. doi:10.1016/j.enbuild.2016.08.040.
- Numan Özakin, A., and F. Kaya. 2019. “Effect on the Exergy of the PVT System of Fins Added to an air-cooled Channel: A Study on Temperature and Air Velocity with ANSYS Fluent.” Solar Energy 184: 561–569. doi:10.1016/j.solener.2019.03.100.
- Ooshaksaraei, P., K. Sopian, S. H. Zaidi, and R. Zulkifli. 2017. “Performance of Four air-based Photovoltaic Thermal Collectors Configurations with Bifacial Solar Cells.” Renewable Energy 102: 279–293. doi:10.1016/j.renene.2016.10.043.
- Panchal, H. 2016. “Performance Investigation on Variations of Glass Cover Thickness on Solar Still: Experimental and Theoretical Analysis.” Technology and Economics of Smart Grids and Sustainable Energy 1 (1). doi:10.1007/s40866-016-0007-0.
- Riffat, S. B., and E. Cuce. 2011. “A Review on Hybrid photovoltaic/thermal Collectors and Systems.” International Journal of Low-Carbon Technologies 6 (3): 212–241. doi:10.1093/ijlct/ctr016.
- Rounis, E. D., A. K. Athienitis, and T. Stathopoulos. 2016. “Multiple-inlet Building Integrated Photovoltaic/Thermal System Modelling under Varying Wind and Temperature Conditions.” Solar Energy 139 (1): 157–170. doi:10.1016/j.solener.2016.09.023.
- Sarhaddi, F., S. Farahat, and H. Ajam. 2010. “Exergetic Performance Assessment of a Solar Photovoltaic Thermal (PV/T) Air Collector.” Energy and Buildings 42 (11): 2184–2199. doi:10.1016/j.enbuild.2010.07.011.
- Saygin, H., R. Nowzari, N. Mirzaei, L. B. Y. Aldabbagh, and Abdullah-Al-Mamoon. 2017. “Performance Evaluation of A Modified PV/T Solar Collector: A Case Study in Design and Analysis of Experiment.” Solar Energy 141: 210–221. doi:10.1016/j.solener.2016.11.048.
- Schindler, F., B. Michl, P. Krenckel, S. Riepe, J. Benick, R. Müller, A. Richter, et al. 2017. “How to Achieve Efficiencies Exceeding 22% with Multicrystalline n-type Silicon Solar Cells”. Energy Proced 124: 777–780. doi:10.1016/j.egypro.2017.09.086.
- Shahsavar, A., M. Ameri, and M. Gholampour. 2012. “Energy and Exergy Analysis of a Photovoltaic-Thermal Collector with Natural Air Flow.” Journal of Solar Energy Engineering-Transactions of the Asme 134 (1). doi:10.1115/1.4005250.
- Shahsavar, A., and Y. Rajabi. 2018. “Exergoeconomic and Enviroeconomic Study of an Air Based Building Integrated photovoltaic/thermal (BIPV/T) System.” Energy 144: 877–886. doi:10.1016/j.energy.2017.12.056.
- Slimani, M. E., M. Amirat, I. Kurucz, S. Bahria, A. Hamidat, W.B. Chaouch. 2017. “A Detailed thermal-electrical Model of Three photovoltaic/thermal (PV/T) Hybrid Air Collectors and Photovoltaic (PV) Module: Comparative Study under Algiers Climatic Conditions”. Energy Conversion and Management 133: 458–476. doi:10.1016/j.enconman.2016.10.066.
- Sopian, K., S. Yigi, H. T. Liu, S. Kakaç, and T. N. Veziroglu. 1996. “Performance Analysis of Photovoltaic Thermal Air Heaters.” Energy Conversion and Management 37 (11): 1657–1670. doi:10.1016/0196-8904(96)00010-6.
- Sopian, K., M. A. Alghoul, E. M. Alfegi, M. Y. Sulaiman, and E. A. Musa. 2009. “Evaluation of Thermal Efficiency of double-pass Solar Collector with porous-nonporous Media.” Renewable Energy 34 (3): 640–645. doi:10.1016/j.renene.2008.05.027.
- Sultan, S. M., and M. N. E. Efzan. 2018. “Review on Recent Photovoltaic/Thermal (PV/T) Technology Advances and Applications.” Solar Energy 173: 939–954. doi:10.1016/j.solener.2018.08.032.
- Tian, Y., and C. Y. Zhao. 2013. “A Review of Solar Collectors and Thermal Energy Storage in Solar Thermal Applications.” Applied Energy 104: 538–553. doi:10.1016/j.apenergy.2012.11.051.
- Tiwari, S., S. Agrawal, and G. N. Tiwari. 2018. “PVT Air Collector Integrated Greenhouse Dryers.” Renewable & Sustainable Energy Reviews 90: 142–159. doi:10.1016/j.rser.2018.03.043.
- Touafek, K., A. Khelifa, L. Boutina, I. Tabet, and S. Haddad. 2018. “Theoretical Study and Experimental Validation of Energetic Performances of Photovoltaic/Thermal Air Collector.” International Journal of Photoenergy 1. doi:10.1155/2018/2794068.
- Wu, S. Y., T. Wang, L. Xiao, and Z. G. Shen. 2019. “Effect of Cooling Channel Position on Heat Transfer Characteristics and Thermoelectric Performance of air-cooled PV/T System.” Solar Energy 180: 489–500. doi:10.1016/j.solener.2019.01.043.
- Yazdanifard, F., and M. Ameri. 2018. “Exergetic Advancement of photovoltaic/thermal Systems (PV/T): A Review.” Renewable and Sustainable Energy Reviews 97: 529–553. doi:10.1016/j.rser.2018.08.053.
- Yu, J. S., J. H. Kim, and J. T. Kim. 2020. “Effect of Triangular Baffle Arrangement on Heat Transfer Enhancement of Air-Type PVT Collector.” Sustainability 12 (18): 7469. doi:10.3390/su12187469.