ABSTRACT
The global demand for temporary structures is increasing in the wake of epidemics and wars. The need to build such structures in a prompt, effortless, and inexpensive manner has become a major focus of research. This paper addresses these issues through the use of weaving, an economical and historical construction technique, to achieve the time-cost and economic benefits of temporary construction required in the current environment. Innovative research on unitary and discrete structures was undertaken with the help of computer languages to explore the creation of such discrete structures using two methods: part-to-whole and whole-to-part. Typical design solutions were then selected for physical construction verification and structural optimisation to ensure feasibility and safety. This research attempts to define a complete workflow for this approach, package the relevant knowledge and processes into a digital method, and present them in a practical woven construction in order to fulfil the current demand for the timely and economic construction of temporary buildings.
Graphical Abstract
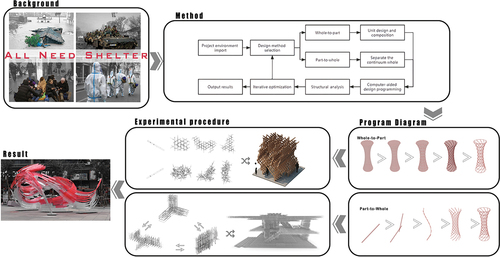
1. Introduction
Unpredictable situations require an adequate and immediate response in the wake of natural disasters, humanitarian and health emergencies, and armed conflicts that can occur anywhere in the world (Brown and Perkins Citation1992; Fried Citation2018). At the end of 2021, there were an estimated 89.3 million people worldwide who were forcibly displaced as a result of persecution, conflict, violence, human rights violations, and events seriously disrupting the public order (UNHCR Citation2022). Furthermore, ravaged by COVID-19, patients have no place to resettle globally. Thus, the need for an efficient shelter in a short period of time has become a problem that needs to be solved. For example, in the context of temporary buildings (Firrone Citation2007), emergency housing units are considered suitable solutions to meet the population’s housing needs (Paparella and Caini Citation2022). In addition, China completed the construction of the Wuhan Leishenshan and Huoshenshan hospitals in about 10 days; these buildings used pre-constructed modular composite building products to a large extent, thus reducing the workload of field operations and saving a lot of time (Chen, Yuan, and Ji Citation2021). However, whether it is for resettlement accommodation for refugees, hospitals for patients, or post-disaster reconstruction projects, the modular products available mostly focus on the project’s specific requirements. There exist few products focusing on recyclability, versatility, speed of construction, and affordability.
Traditional materials such as brick have a disadvantage as they cannot be easily recycled. This is one of the reasons that temporary construction was created: to overcome the time-restrictive construction system of bricks and achieve the effect of rapid construction within a certain period of time. Products with the same dimensions as bricks can be combined in a manner similar to bricks to create unexpected spaces. Woven early handicraft products can achieve a similar effect, as canes and branches can be woven to create a variety of artifacts that can be dismantled and reused. These products have the advantage of high efficiency, which meets the requirements of the current environment.
As the origin of architecture, weaving, through its development, appears in the skin of buildings (Martínez Calzón and Castañón Jiménez Citation2010), the demarcation of space (Reisner Citation2019), and the structure of buildings. Weaving encompasses not only the architecture but also the materials, textures, and colours of a building. The technique of weaving in architecture has made new advances in a variety of architectural contexts (Yan Citation2020; Liu, Hua, and Li Citation2022; Chen, Wang, and Chen Citation2021). The disadvantage of traditional woven architecture is that it takes more time to build, which conflicts with the principle of temporary architecture. However, in the context of digital architecture, the convenience and precision of digital tools provide a means of design and a way of thinking for weaving technology that is distinguishable from traditional techniques. Not only has it broadened the designer’s perspectives, it has also greatly improved the architect’s manipulation of design, provided new ways of dealing with woven forms, and most significantly, decreased the weaving time. “Digital design offers not just a style but a new approach to design” (Biao Citation2012). This new approach allows unitary weaving projects to be designed and built rapidly to fulfil ongoing requirements.
This paper will consider architectural weaving as an entry point and digital software as a tool to explore a diverse and variable temporary building design strategy for refugee shelters, temporary hospitals, and temporary special accommodation in response to the current demand for temporary buildings. Specifically, this research attempts to answer the following three questions:
What are woven buildings and unitary woven buildings and what are their characteristics? Furthermore, how can they be adapted to temporary buildings?
How should weaving be designed against the backdrop of war and epidemics to create a weaving form that fits the current era?
How should the rope and knot combination patterns of different weaving units be applied? Further, how is the digital model feasible in the actual production process?
2. Literature review
2.1. Architecture of woven structure
“The knot is the oldest and most optimal construction of the structural meaning of the universe” (Frampton Citation1985). In fact, the knot is a combination of a rope and a knot; if we consider the geometric perspective when exploring the weaving form, the knot can be summarised as a line and point through the interspersed point and line to build a two-dimensional or three-dimensional mesh form. Moreover, the line and point contain mechanical and morphological characteristics: the line has a certain volumetric dimension and mechanical load-bearing capacity with a certain compression resistance, while the point has a certain rigidity, which ensures that the overall form does not deform when the line is subjected to a reasonable force. Therefore, from the geometric perspective to the actual construction of the weave, the knot will have a specific meaning, whereas the rope represents the woven form of the linear components themselves. Thus, the focus of the discussion is the unit morphological pattern. The knot represents the pattern that connects the components of every part.
Weaving has developed over time, with Gottfried Semper being the first person to introduce the concept of weaving in architecture (Semper Citation1989). Frank Lloyd Wright created the theory of weaving on structure (Wright, Kaufmann, and Raeburn Citation1967), Kenneth Frampton extended weaving to tectonic culture (Frampton Citation1985), and in contemporary times, Jacques Herzog and Pierre de Meuron (CitationFloornature Herzog & de Meuron [EB/OL], Peter Zumthor (Zumthor Citation2014), and Jean Nouvel (Lee Citation2018) have referenced the diversity of weaving. Kengo Kuma (Kuma Citation2015) combines discrete elements using mortise and tenon connectors and builds a structural whole in which each element relies on the others, so that concrete architectural examples of unitary weaving emerge accordingly ().
With the continuous development of architectural weaving, there are currently many architectural works related to weaving. However, most of them are in the form of architectural skins (Simmonds, Self, and Bosia Citation2006), where the intricacy and variety of the patterns are mainly reflected through intertwining linear patterns. In this study, research on architectural weaving is conducted mainly from the perspective of combining graphics and structure; therefore, this study focuses on the role of structure in weaving, as it can present the most realistic form of the architectural aesthetic.
2.2. Temporary buildings
The term “temporary” is used in opposition to “permanent”. Temporary buildings focus on flexibility and the ease of transport and assembly (Cordescu and Kronenburg Citation2002). For example, nomadic tents and large 18th-century circus tents embody the concept of simple and systematic temporary constructions. After the emergence of modernism, temporary architecture with a variety of functional requirements gave rise to a wide range of experimental sites at the time. Furthermore, the concept of standardisation and modularity created multi-dimensional spatial forms. Later, represented by the “plug-in city” based on movable “cabin houses” proposed by Peter Cook in 1964, the Archigram group criticised and responded to what they considered dull and conservative modern architecture at the time (Kronenburg Citation2007). Mathias Schwartz-Clauss and Alexander von Vegesack’s summary of temporary architecture has driven the development of technology once again (Schwartz-Claus. Citation2002), in addition to Jennifer Siegal’s significant contribution to the development of the city (Gherardini and Leali Citation2017). Moreover, Japanese metabolism architect Kurokawa Kisho (Lin Citation2011) believed that buildings should be replaced like cells. Shigeru Ban’s work (Barrie Citation2014) on architecture explores the creation of new structural forms from ecological materials, and Kengo Kuma (Kuma Citation2015), inspired by the 2011 earthquake on the Pacific coast of Tōhoku, believes that small-scale temporary architecture can bring people closer to nature.
Meanwhile, the temporary construction industry has increasingly focused on sustainability, and Ryan E. Smith (Smith Citation2010) argues that off-site construction followed by on-site integration offers a higher level of convenience and recyclability. Further, owing to the slow pace of global urbanisation since the COVID-19 pandemic, temporary buildings (Ryan and Smith Citation2017) have become increasingly popular and of concern; the prefabricated strategy thus allows the fulfilment of a wide range of building requirements during isolation periods (Hatcher Citation2021).
Altogether, temporary architecture is gradually gaining attention as the best way to respond to the unexpected special needs of the architectural space. We analysed the characteristics and trends of the mainstream research on the rapid construction of temporary buildings in the construction industry. Accordingly, we believe that there is a demand for temporary buildings in the current environment in order to fulfil the demand of rapid construction, sustainability, convenience, economy, and low-tech constructability. These requirements have therefore become the focus of temporary building design, which is used as the design concept throughout this study.
2.3. Unit weave design
The unit design has been in existence for a long time in the development of architecture; the stacking of bricks and shaping of Chinese brackets are all closely related to the unit design. This discrete design pattern has different meanings for different designers. For example, Kengo Kuma referred to it as a “particularised design” (Carpo Citation2019), while Patrik Schumacher believed that design is a branch of parametric design (Schumacher Citation2009). However, it can be concluded that this form of design is characterised by a single-to-whole and less-to-more approach, and Mario Carpo summarises this discrete design pattern as a design style (Carpo Citation2017). In contrast to the continuity of the parametric design agenda, the notion of “discrete” breaks away from a tradition of mass-customisation of a singular building, thereby attempting to reconstruct the commons through distributed knowledge and design production (Sanchez Citation2019).
Reconsidering part-to-whole relationships indicates a renewed interest in mereology (Köhler Citation2017). Two types of weaving patterns can be distinguished from the weaving perspective: unitary and continuous. The manner of distinguishing between these two forms can be found in the description of the spline diagram in Greg Lynn’s Animate Form (Lynn and Kelly Citation1999), as well as in Daniel Koehler’s (Köhler Citation2016) and Mario Carpo’s (Schumacher Citation2009) theories, which show that the digital “curve” of discrete blocks and discrete combinations of generic units result in an automated building that is both efficient and mass-produced, which describes the transition from continuous to discrete (G. Retsin Citation2019) (). The characteristics of the two weaves are also developed with reference to the above theories ().
Figure 2. The transformation from continuous to discrete by Greg Lynn’s NURBS curve shows the transition of continuous weaving to unit weaving.
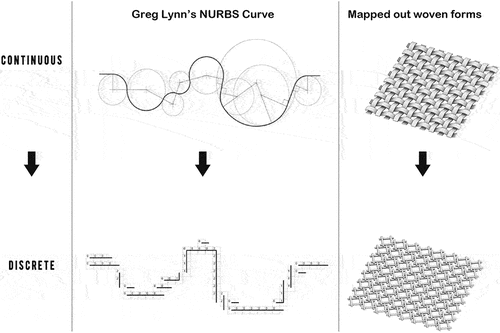
Table 1. Comparing the properties of the unit and intertwine weave forms.
At present, despite the impact of new technologies, such as collaborative construction using 3D printing, robotics, and artificial intelligence, research on different directions of weaving has emerged. However, on investigating the current works on woven architecture, we find that a more systematic theoretical system has not yet emerged for unit weave forms. Woven structures tend to be complex and do not fit the characteristics of temporary architecture because, for example, repeated installation and disassembly are difficult. Moreover, there is scarce research on the optimisation of unit components; for instance, in the unit weave projects of Kengo Kuma, to achieve a more reasonable structural form, some projects are not modified according to the mechanical environment in which the unitary elements reside. At the same time, some woven products are not designed completely according to the “knot and rope” theory of weaving, and there exists great potential for research on the form of connection between the units. The current construction of such discrete assemblies mostly considers factory prefabrication to on-site assembly as the overall workflow; thus, “design for manufacture and assembly” has become a basic principle of prefabricated construction design (Chen, Wang, and Chen Citation2021; Selvaraj, Radhakrishnan, and Adithan Citation2009). However, additional connections exist, and these auxiliary joints do not alleviate the difficulty in the assembly process. Therefore, understanding how to deal with the relationship between the unit parts and connectors – that is, the relationship between “rope” and “knot” – remains a crucial part of the research on unit weaving.
3. Unit weave structure design method and application
Ulrich Bogenstätter noted out that the design stage generally determines up to 80% of the building operational costs (Kovacic and Zoller Citation2015; Bogenstätter Citation2000). Therefore, a good design system (or process) is vital to prefabricated buildings. For unit weave, the unit part is the basis of the design; thus, the selection of the unit form is crucial and will have an impact on the overall process of the design. Additionally, for architecture design, two design ideas are common (Moussavi Citation2009), namely “bottom-up” and “top-down” (Leach and Yuan Citation2017; Schumacher Citation2011), the former represents a traditional design approach, using the overall project concept as a design strategy, while the latter represents generative design, often associated with computer-aided design, transforming architecture as an “outcome” into architecture as a “process”, and changing the design process of seeking a definitive solution into a design process of seeking an open system (Weiguo Citation2009). Nevertheless, as the object of this study is unit weaving, the word “unit” is of paramount significance; therefore, the bottom-up and top-down are further interpreted as part-to-whole (bottom-up) and whole-to-part (top-down) to better fit the definition of this design approach. Part-to-whole can be understood as the conceptualisation of the design from a macroscopic viewpoint, as well as a refinement of the design from the whole to the part. Whole-to-part is the process of forming the whole from the individual units under systematic organisation (). However, in this discussion of generation, there is no absolute part-to-whole or whole-to-part approach, and the experiment is based on the main operation of the algorithm or plug-in to classify the generation. The discrete structure with the overall form condition as the initial input condition is classified as whole-to-part generation, while the generation process with the unit form and operation rules as the main input condition is classified as part-to-whole generation.
3.1. Unit weaving design process
As mentioned above, the current unit weave designs need to primarily resolve the following issues: recyclability, low construction cost, rapid assembly and multi-environmental adaptation. Therefore, a set of systematic processes is needed to accomplish the above design principles and avoid the issues related to the isolated design solutions. For example, a particular solution is only applicable to a single site that is inconsistent with the original design intent of unitary weaving. This design strategy is undoubtedly accomplished with the help of digital technology and both part-to-whole and whole-to-part designs are universally applicable with the help of digital tools, thereby allowing for optimal solutions for different spatial needs. Based on the above conditions, shows the workflow of the design.
3.2. Design approach
3.2.1. Part-to-whole
Part-to-whole is a common technique of discrete design, which can be seen in the construction of fences and ancient Chinese wooden buildings, similar to the bracket system in wooden buildings where the units are gradually stacked using mortise and tenon joints to form a poetic structural whole.
We used two basic types of linear unit pieces to complete the part-to-whole design. In the linear unit, We designed the linear unit using mortise and tenon joints. First, the angle of the tenon opening on the unit piece determines the shape and orientation of the structure as a whole. For example, setting the tenon opening at 90° results in an orthogonal combination of linear units, which will also result in a regular matrix-like frame. However, rotating these tenons by a certain angle will result in a structural system that appears as a chaotic frame. Thus, this is a design system similar to a patchwork toy where a variety of results will be formed by adjusting the most basic unit blocks at different angles, and if regularised by simple logic, structural blocks that are structurally, spatially, and functionally valuable will be formed.
Inspired by Kengo Kuma’s Yure Pavilion (Hatcher Citation2021) prototype design, based on the principle of discretisation, a simple pavilion can be obtained fairly quickly using the Grasshopper software by opening a 60° cut in the vertical plane at the top and bottom of the same unit piece, connecting the unit pieces together, and repeating this mortise and tenon connection over and over again to meet the aesthetic and functional requirements (). Moreover, linear unit has the following characteristics: 1) recyclable (all components are combined with discrete components without any external connectors which can be easily disassembled and reassembled into other shapes); 2) easy to construct (because there are no extra connectors, the whole shape can be constructed in a short span of time as if it were a patchwork); 3) extremely low cost (regardless of the material chosen for construction, as all the modular components are the same, they can be constructed in a factory).
In the curved module, the above-mentioned mortise and tenon connection is no longer sufficient for normal weaving owing to the shape of the curve and the way it is stressed. Therefore, we need to find a new way to complete the weaving of the curved unit.
For the general curved unit, the irregular connection will display the chaos without the design sense, which not only deviates from the essence of the discrete design (discrete buildings are continuously repetitive and consist of reconfigurable universal discrete components)(Köhler Citation2016) but is also time-consuming and labour-intensive in terms of the manufacturing and construction process. Therefore, conceiving the basic unit module becomes the first step of design. We can consider a unit cube as the prototype, extract the centre point of the edge line as the curve control point, obtain a total of 12 points, arrange and set three or four of these points as a group, and filter for differentiation and standardisation to get the following 12 curves, from which three representative curves are selected for further design ().
After completing the construction of the unitary form, the connection between the units will be considered. The heterogeneous aggregated morphology is gradually created under the controllability of the connection rules. In this way, the connections are finite and controllable, thereby creating a complete set of connection rules between several units. The connection behaviour of the units can be considered in two separate ways: 1) the “end” connection, where the unit is connected by a rectangular face frame at the end, while ensuring the point-to-point docking (this approach ensures the overall uniformity of the unit body after several iterations of growth and is a means to ensure the controllability of the form); 2) connection through the “middle” of the unit body. Compared with the end connection, this approach increases the expansion of the woven form, so that the unit body has not only unidirectional linear development but also multi-dimensional expansion, which increasing the possibility of each discrete unit becoming an architectural weave ().
Although such an approach can achieve a discrete distribution of the weave as a whole without breaking the connection logic, it gives rise to an infinite number of permutation patterns because each unit can be connected to random points of other units, and thus, additional rules and constraints need to be introduced. This phase of the iteration focuses more on the geometry of the units and the combinatorial laws. To make the connection form more operational, further attempts to expand the unit body are made based on the unit morphology of the previous stage. B2’/D1’/D2’ is obtained by stretching the B2/D1/D2 base unit cell in the Z-axis direction. To make the two types of unitary bodies morphologically distinct, B2/D1/D2 was used as the unitary transformation structure of the woven whole and B2’/D1’/D2’ was used as the main structure, which means that we individualized a central spindle bar in each unit and the point connections were made only through these rods (). With this constraint, the possible connections are greatly reduced, which making it easier for the designer to control the aggregation process.
With this approach, a large number of aggregates are generated based on the number of connected units. Each generated solution relies on the structural ability, spatial quality and aesthetic qualities of the designer. On this basis, these units, whether discarded or retained, can be formed into aggregates with controllability. The larger, more complex forms created by the simple units are where the beauty of unitary weaving lies, and the reuse and disassembly of units can be adapted to different environments and spatial needs at the same time ().
Unitary masses have the ability to form aggregations on three axes under different stacking strategies, thus creating volumes in space to aggregate into complete woven forms. In addition, the need for a building system can be addressed by accumulating the unitary parts, which can be converted into floor slabs, structural columns or a complete building system that, when aggregated with other parts with specific behaviours, can form complete building bodies ().
As mentioned above, part-to-whole is a bottom-up process, where in we set the base unit and add the corresponding “knots” to form the design rules to finalise the overall building design. This method is based on the emergent theory and the theory of swarming, which makes it possible to obtain diverse results by adjusting the shape of the base unit and algorithm very conveniently. Then, the unit body, which tends to be of the same shape, can be manufactured in practice with much less time and cost. However, the disadvantage of this approach is that it is difficult to adjust one or more of the units to the nuances of the process. Further, as the combination of shapes is mechanically generated by the process, the end result may not be what the designer had in mind in terms of the outcome. Conversely, this procedural approach is a good option for temporary buildings when there are significant financial and time constraints.
3.2.2. Whole-to-part
The major difference between whole-to-part and part-to-whole is that the latter is a discrete combination of units, which aims to turn the continuous paradigm upside down by shifting formal complexity from the design of the whole to the assembly logic of the parts.
The whole-to-part is not completely separated from the continuum; to some extent, it is designed as a continuous whole as the foundation of discrete units, which can be summarised as voxelisation or grid design. In this way, whole-to-part units are not identical in morphology, there is a topological relationship between the units, and the units need to be combined into a continuous structure by deformation. In this process, the border area that needs to be filled by the unit is first formulated, and from the overall morphology as the initial condition of the design, the entities that are originally in different spatial locations are abstracted into a grid in 3D space. In this whole-to-part generation process, the independent variable is set to the resolution when a uniform overall form with a smaller resolution is able to restore the detailed design, and a larger resolution is usually used for those parts of the design object that have structural implications while having a more abstract representation (G Retsin Citation2019).
For example, in Grasshopper, we can use the nursery particle simulation to extrapolate the form and build a continuous surface (). With this as the field, we can set the rules for any identical or different units and put them in, so that a top-down design logic is created, and the process of arbitrarily adjusting the form, size, and sparsity of the units is also a continuous-to-discrete and concrete-to-abstract transformation process ().
What is different in this stage from the above-mentioned part-to-whole is the design step of the “knot”. In whole-to-part, the “knot” is no longer the guider of the unit module, that is it does not have the function of guiding the connection of the unit body. Instead, it plays more of an auxiliary role, which is the function of “connection”. At the same time, for this type of combination of units, the choice of “knots” generally requires a classification of the materials used. For example, traditional materials, such as bamboo and wood, are often used to bind, while metal components, such as aluminium and steel, are welded. If acrylonitrile butadiene styrene (ABS) is used as the unit construction material, then connectors and snaps are the best choice.
From a design approach perspective, both approaches can be effective. However, the whole-to-part approach, which has played a pivotal role in design practice in the past, provides more flexibility and freedom to translate inspiration directly into graphics. However, for unit weaving, it is difficult to separate the whole-to-part or part-to-whole design approaches, as either approach, even in its purest design, cannot avoid a mixture of the other approach. Therefore, the two strategies proposed in this study are merely considerations for the initial stage of design, and it is not possible to determine which is superior to the other. Among the above-mentioned design ideas, part-to-whole provides a more scientific and systematic solution and a basis for inspiration, while whole-to-part provides a clear design guideline and leads the way.
3.3. Mechanical performance verification and manufacture
In the end, owing to time constraints and the ongoing pandemic, only one of the weaving forms was selected for the actual construction to verify the practicality of this research. After the comparison, the more complex and difficult to construct whole-to-part grid surface unit weave became the experimental target.
We grouped the original curves so that each group of curves formed a larger discrete module, thus combining the 22 groups of module items to create a lightweight framework (). The arrangement retains bending forces in the structure, thereby maximising its strength. The modular elements were designed for their interconnectivity, allowing the shift from small-scale elements to larger ones and vice versa.
To create this spatially curved unit, several materials were used, namely ABS, rebar, expanded polystyrene (EPS), wood, and bamboo. First, natural raw materials such as bamboo were excluded because they could not meet the precision requirements of standardisation. Moreover, wood was eliminated owing to its poor bending and shear resistance. Additionally, based on the scheduling and cost control of ABS, the use of injection moulding or 3D printing is not advisable as it will result in a wastage of time and money. Furthermore, EPS is not suitable for shaping into linear forms owing to its own material properties. In summary, we compared the material cost, unit manufacturing time, material strength, manufacturing difficulty, and precision of these materials. Local manufacturing levels and transportation costs were taken into account to rate each performance (). Steel was finally selected as the material for creating the curve module.
The corresponding “knot” method was then determined. The connection method of reinforcing steel was classified into tying lap, welding, and mechanical connection, and welding was selected as the most suitable “knot” owing to its timeliness and convenience.
Prior to the actual fabrication, we performed a finite element analysis (FEA) on this project. Before the structural optimisation, all the curves were made using 8 mm steel, which led to a maximum displacement of 86.5 cm and a highly unstable structure. For better optimisation, three different diameters of steel pipes (6 mm/8 mm/10 mm) were selected for the replacement according to the load strength at the location. After the optimisation, the maximum displacement of the structure was reduced to 5.3 cm, which greatly improved the overall strength of the structure and met the project construction requirements ().
Figure 15. Comparison of the structural displacement before and after replacing different sizes of the rebar tubes.
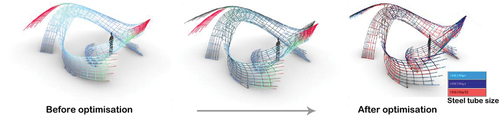
The finished products displayed outstanding structural performance. It is worth mentioning that during the manufacturing process, we made use of augmented reality devices (Hololens) for the localisation of the units. Although we lacked the help of large machines in production and manufacturing, this low-tech approach improved the accuracy of the construction to a great extent. The final research team of six people completed the whole process from manufacturing to assembly in seven days ().
In the final result, we added two colours of polycarbonate board to the maintenance structure of the skeleton, which is also an excellent solution to the long-standing problem of sealing and waterproofing the temporary structure of the unitary weave. The polycarbonate boards are also presented in a discrete form, similar to the shingles used on roofs. After the overall frame was completed, the polycarbonate boards were placed in the correct places to meet both the functional and aesthetic requirements ().
This case strictly follows the actual construction and usage requirements as well as implements the core concept of unit woven construction. The low cost, ease of assembly, and flexible and robust product characteristics of such construction determine its potential in the field of temporary construction where rapid construction is required. The final presentation shows that the woven pavilion fully inherits the knotted concept of woven architecture and combines both the part-to-whole and whole-to-part concepts, reflecting the result of the combination of the two design approaches. In the final experimental phase, the project as a whole was further optimised according to the actual situation, resulting in the construction of a unit woven pavilion that achieves a balance between aesthetics and stability.
4. Discussion
This study discussed the use of the unit weave form in temporary architectural pavilions to assess the relationship between discrete geometric elements and temporary construction. While continuous and monolithic structures have been the mainstay of architectural research in the past, unitary and discrete architectural forms have become increasingly popular as humans need change. Thus, in the traditional design process, the node is often overlooked; for example, it is difficult to find a clear node for concrete pouring or brick masonry. However, for the unitary weave discussed in this study, the node is essential, as it symbolises the integrity of the architecture as well as its logic; each node foresees the direction and position of the next unit. Simultaneously, expressed in the morphology of weaving, the node is transformed into a knot. Therefore, we summarised the characteristics of this structure from the starting point of “rope” and “knot”, and it is obvious that choosing the right “knot” and “rope” is important in temporary construction so that it can cope with different situations and lead to diverse results. For example, Jose Sanchez used the principles of a voxel space frame constructed from standardised timber elements with steel nodes planning of the site (Sanchez Citation2019), and Gilles Retsin’s Diamonds House (Retsin Citation2020) embodies such characteristics.
In terms of results, the unit weave system has intense discrete characteristics. It is obvious that the stacking of a series of units achieves efficiency that is difficult to accomplish by traditional construction means. This confirms the view of digital architect Greg Lynn (Lynn Citation1993), that imaginative and complex forms can be created through constant repetition of discrete units. Thus, the unit weave system, like other discrete architecture, can be manufactured in large volumes through modular manufacturing. Simultaneously, the enhancement of either the part-to-whole or whole-to-part approach allows for the adaptation of multiple solutions through a very low cost and rapid construction strategy. This has wide social value as it can be applied to temporary housing for low-income and homeless people, short-term exhibition halls and mobile stores, and landscape artifacts. Most of the materials can be recycled after use, which greatly enhances the environmental and economic value. However, the shortcomings of the unit weave include the lack of airtightness and containment owing to its own structural characteristics, which makes it unsuitable for a fully enclosed isolation ward or infectious temporary hospital in the event of a pandemic; if it is necessary to include this type of function, adding an outer skin over the unit components is one possible way to address this.
In summary, this study discussed the generative design of different forms of units including the combination of bottom-up and top-down design logic. Such design involves various combinations of linear elements and the generation of complex structures. The design approach is similar to the construction of a honeycomb, gradually extending from a single honeycomb form to the overall form. This provides the design logic of weaving forms under the aggregation of multiple units of architectural design, and explores the demand for temporary architecture in contemporary society with a modular way of thinking.
5. Conclusions
The unit weave system is a design strategy for temporary buildings to respond to the need for rapid construction in a short period of time. The first priority is to overcome the complexity of the work, with short timescales and high design accuracy. Second, the units are rapidly manufactured during the design process using Grasshopper for comparison and rapid modification of multiple solutions, as well as during the construction process, which benefits from augmented reality assistance and a robotic arm machining assistance system for construction organisation. The design and construction solutions were also pre-simulated and assembled with modular structures to complete the woven structure as a whole in a considerably short period of time. Based on this design strategy and the actual construction situation, the following conclusions were obtained:
Unitary woven architecture in the digital age is highly malleable and, with the help of digital tools, can be shaped into a diversity of semi-enclosed spaces. The assembly of the units not only constitutes the main structure of the building but also contains the aesthetics of the architecture.
Based on research on the design and construction phases, temporary buildings fit well with the unit weaving system, in terms of both timeliness and economy, as this system meets most needs in the current environment. This design strategy aims to solve the problem of the efficient and low-cost construction of temporary buildings. The extant research also indicates that this design effectively solves the problem of low-tech digital manufacturing.
In the early stage of the unit weave project design, a 3D information model was constructed using Grasshopper, the relationship between the building and the site was visualised using Hologram program, and site levelling and spatial positioning was carried out based on the analysis. Augmented reality technology was used for site layout planning and design-efficient design solutions. This provided technical support for rapid construction.
Standardising the design is the foundation and prerequisite for promoting the industrialisation of temporary buildings. Simplifying the design process while coordinating construction is necessary, and the structural design of unit weave systems should fully consider unit connections (knots) to prevent accidents during practical use.
Disclosure statement
No potential conflict of interest was reported by the author(s).
Additional information
Funding
References
- Barrie, A. 2014. “Shigeru Ban: Cardboard Cathedral[J].” Interstices 107 (14): 107–110.
- Biao, L. 2012. “Generative Design of architecture[M].” Southeast University. 35–46. (in Chinese).
- Bogenstätter, U. 2000. “Prediction and Optimization of life-cycle Costs in Early design[J].” Building Research & Information 28 (5–6): 376–386.
- Brown, B. B., and D. D. Perkins. 1992. Disruptions in Place Attachment, 279–280. New York: Plenum Press.
- Carpo, M. 2017. The Second Digital Turn: Design beyond intelligence[M], 134–137. Cambridge, MA: MIT press.
- Carpo, M. 2019. “Particlised: Computational Discretism, or the Rise of the Digital discrete[J].” Architectural Design 89 (2): 86–93.
- Chen, D., G. Wang, and G. Chen. 2021. “Lego Architecture: Research on a Temporary Building Design Method for post-disaster emergency[J].” Frontiers of Architectural Research 10 (4): 758–770.
- Chen, L. K., R. P. Yuan, X. J. Ji . 2021. “Modular Composite Building in Urgent Emergency Engineering Projects: A Case Study of Accelerated Design and Construction of Wuhan Thunder God Mountain/Leishenshan Hospital to COVID-19 pandemic[J].” Automation in Construction 124: 103555.
- Cordescu, A., and R. Kronenburg. 2002. Mobile: The Art of Portable architecture[M], 186–190. Princeton, NJ: Princeton Architectural Press.
- Firrone, T. R. L. 2007. “Sistemi abitativi di permanenza temporanea[M].” Aracne Editrice 159–160.
- Floornature Herzog & de Meuron [EB/OL]. (2001-0707) [2022-0903]. https://www.floornature.com/herzog-de-meuron-78/
- Frampton, K. 1985. Studies in Tectonic culture[M], 10–30. Cambridge: Harvard University Graduate School of Design.
- Fried, M. 2018. Grieving for a Lost home[M], 335–359. London; New York: Routledge.
- Gherardini, F., and F. Leali. 2017. “Reciprocal Frames in Temporary Structures: An Aesthetical and Parametric investigation[J].” Nexus Network Journal 19 (3): 741–762.
- Hatcher. 2021. “Modular Buildings in the Time of Covid-19[J].” Smart Buildings Magazine 124 (4): 1–10.
- Köhler, D. 2016. “The Mereological City: A Reading of the Works of Ludwig Hilberseimer[M].” Transcript Verlag 86–87.
- Köhler, D. 2017. “Large City Architecture: The Mereological Mode of the Quantified city[J].” International Journal of Parallel 32 (1): 163–172.
- Kovacic, I., and V. Zoller. 2015. “Building Life Cycle Optimization Tools for Early Design phases[J].” Energy 92: 409–419.
- Kronenburg, R. 2007. Portable architecture[M], 50–53. London; New York: Routledge.
- Kuma, K. 2015. “Small Architecture: Natural Architecture[M].” Architectural Association 35–36.
- Leach, N., and P. Yuan. 2017. Computational design[M], 68–75. Shanghai: Tongji University Press.
- Lee, J. Y. 2018. “The Scenography of Nature in the Work of Jean nouvel[J].” Journal of Landscape Architecture 13 (1): 68–77.
- Lin, Z. 2011. “Nakagin Capsule Tower: Revisiting the Future of the Recent past[J].” Journal of Architectural Education 65 (1): 13–32.
- Liu, Y., H. Hua, and B. Li. 2022. “Exploration and Design of Knitted Composites for Architectural Application: The MeiTing project[J].” Frontiers of Architectural Research 594–608.
- Lynn, G. 1993. “Folding in architecture[M].” Academy Editions Limited. 28–47.
- Lynn, G., and T. Kelly. 1999. Animate form[M], 35–50. New York: Princeton Architectural Press.
- Martínez Calzón, J., and C. Castañón Jiménez. 2010. “Weaving Architecture: Structuring the Spanish Pavilion, Expo 2010, Shanghai[J].” Architectural Design 80 (4): 52–59.
- Moussavi, F. 2009. “The Function of Form[M].” Actar and the Harvard University Graduate School of Design 20–50.
- Paparella, R., and M. Caini. 2022. “Sustainable Design of Temporary Buildings in Emergency situations[J].” Sustainability 14 (13): 8010.
- Reisner, Y. 2019. “Architecture and Beauty: A Symbiotic relationship[J].” Architectural Design 89 (5): 6–13.
- Retsin, G. 2019. “Bits and Pieces: Digital Assemblies: From Craft to automation[J].” Architectural Design 89 (2): 38–45.
- Retsin, G. 2019. “In Part Whole: The Aesthetics of the Discrete[J].” Architectural Design 89 (5): 120–127.
- Retsin, G. 2020. Discrete Timber assembly[C], 264–271. London: UCL Press.
- Ryan, E., and Q. Smith. 2017. Offsite Architecture: Constructing the future[M], 200–205. Abingdon, UK: Taylor & Francis.
- Sanchez, J. 2019. “Architecture for the Commons: Participatory Systems in the Age of platforms[J].” Architectural Design 89 (2): 22–29.
- Schumacher, P. 2009. “Parametricism: A New Global Style for Architecture and Urban design[J].” Architectural Design 79 (4): 14–23.
- Schumacher, P. 2011. The Autopoiesis of Architecture, Volume I: A New Framework for Architecture[M], 35–43. Chichester: John Wiley & Sons .
- Schwartz-Claus, M. 2002. “Living in Motion: Design and Architecture for Flexible dwelling[M].” Vitra Design Museum. 20–22.
- Selvaraj, P., P. Radhakrishnan, and M. Adithan. 2009. “An Integrated Approach to Design for Manufacturing and Assembly Based on Reduction of Product Development Time and cost[J].” The International Journal of Advanced Manufacturing Technology 42 (1): 13–29.
- Semper, G. 1989. The Four Elements of Architecture and Other writings[M], 56–94. Cambridge: Cambridge University Press.
- Simmonds, T., M. Self, and D. Bosia. 2006. “Woven Surface and form[J].” Architectural Design 76 (6): 82–89.
- Smith, R. E. 2010. Prefab Architecture: A Guide to Modular Design and construction[M], 86–90. Hoboken, New Jersey: John Wiley & Sons.
- UNHCR. 2022 . “Global Trends Forced Displacement in 2020 [EB/OL].” (2022-0616) [2022-0903]. https://www.unhcr.org/hk/about-us/figures-at-a-glance
- Weiguo, X. 2009. “Fold Theory, Nomadic Space: Interview on Parametric Architectural design[J].” World Architecture, no. 8: 16–17.
- Wright, F. L., E. Kaufmann, and B. Raeburn. 1967. Frank Lloyd Wright: Writings and buildings[M], 25–50. Thiruvalla: Meridian Books.
- Yan, L. 2020. Woven Arch Bridge: Histories of Constructional Thoughts[M], 132–140. London; New York: Routledge.
- Zumthor, P. 2014. “Peter Zumthor: Buildings and Projects[M].” Scheidegger & Spiess 20–25.