ABSTRACT
This research offers valuable perspectives on the nexus between urbanization and the smart environment within the Najran city, Saudi Arabian context. Najran City, with its diverse districts and evolving urban landscape, is at the forefront of adopting advanced technologies and participatory governance models to create a resilient, environmentally conscious, and sustainable urban environment, likewise positioning itself as a model of smart urban development but is also fostering an innovation-driven community equipped to address the difficulties and exploit the prospects given by the 21st century. The study systematically analyzes the multifaceted factors of smart city variables in Saudi Arabia and their direct impact on urban sustainability through extensive quantitative investigations. This study reveals the positive correlations between smart city attributes, on the attainment of urban sustainability. In order words, the smart city’s attributes such as IoT (Internet of Things) devices, smart infrastructure, and data-driven solutions are linked to the creation of a resilient urban environment in the face of urbanization and sustainability challenges. On the other hand, the study noted that the integration of smart technologies might lead to unintended consequences in urban planning. For example, an overemphasis on technology may overshadow human-centric approaches, which might potentially affect the community well-being and social cohesion. Study’s insights provide policymakers, urban planners, and players with an in-depth knowledge of the pathways to harness smart city attributes for sustainable Najran urban development. The implications of this research extend beyond the borders of Saudi Arabia, serving as a valuable reference for regions worldwide grappling with similar urbanization and sustainability challenges in the 21st century. By harnessing the potential of smart cities, Saudi Arabia and other nations can pave the way for greener, more resilient urban futures.
1. Introduction
Globally, urbanization is critical to sustaining growth in the economy and industrialization (Henderson Citation2005; Pineo Citation2022). The growth of urban areas is accompanied by an agglomeration process in specific regions, thriving on enhanced infrastructure, an active workforce, and a varied range of products (Krugman Citation1991). Communities with better access to essential services are more appealing for economic development (Stepniak and Rosik Citation2018). Notwithstanding its good economic advantages, rapid population growth without parallel infrastructure can result in a variety of negative results, including increased urban expenditures and harmful environmental repercussions (Chatti Citation2021; Martin and Rogers Citation1995). Urban costs constitute a significant portion of household income in most countries worldwide, consequently impacting the quality of life in urban areas. Additionally, the escalating carbon emissions in large cities adversely affect the performance of companies (Tabuchi Citation1998). Acknowledging these potential negative impacts is crucial for developing a balanced and comprehensive understanding of the implications of smart city initiatives. It emphasizes the importance of carefully planning and managing the implementation of smart technologies to mitigate adverse effects on sustainability and ensure that the benefits are equitably distributed across the entire population. Thus, to ease and minimize the negative environmental impacts of urbanization, suitable government initiatives ought to regulate the migration of activities from rural to urban areas (Holl and Mariotti Citation2018; Pineo Citation2022).
Saudi Arabia has witnessed rapid urbanization in recent decades, driven by factors such as population growth, industrialization, and economic diversification (Alanazi Citation2023; Almulhim and Cobbinah Citation2023). The process of urbanization involves rural populations migrating to urban areas, which has been a global phenomenon occurrence over the past century. While urban transformation brings economic opportunities, improved access to services, and enhanced quality of life, it is also associated with a slew of issues, including a scarcity of reasonable housing and environmental concerns (Almulhim and Cobbinah Citation2023; Oke et al. Citation2020). To address these challenges, many cities are turning to the notion of smart cities, which utilize information and communication technology (ICTs) to stimulate sustainable growth, through effective resource management (Ismagilova et al. Citation2019; Pellicer et al. Citation2013). Among the visionary solutions emerging in this endeavor, the idea of Smart urban stands at the forefront, offering a transformative path towards environmentally conscious urban living. To solve this problem calls for ICT services through real-time data monitoring and analysis, which can help reduce downtime and ensure continuity of service delivery (Kaluarachchi Citation2022; Schewenius, McPhearson, and Elmqvist Citation2014).
Smart cities harness various technologies, including Internet of Things (IoT) devices, artificial intelligence (AI), and big data analytics, to gather and analyze from various sources, including sensors and citizen feedback, to guide decision-making and enhance service delivery (Ahad et al. Citation2020; Kaluarachchi Citation2022; Paroutis, Bennett, and Heracleous Citation2013). The primary aim of smart cities revolves around enhancing livability and functional efficiency, while also ensuring that the city is environmentally sustainable. It also tackles an array of urban issues, such as pollutants in the air, and ineffective energy utilization, optimizing urban services and infrastructure, improving accessibility and mobility, and promoting citizen engagement and participation. According to Mahapatra, et al., (Citation2017); Joshi, et al., (Citation2016); and Ahad, et al., (Citation2020), the ultimate objective of smart urban is to establish an urban environment that is more conducive to living, a sustainable, and inclusive that meets the needs of all residents, businesses, and visitors.
In partnership with UN-Habitat, the Minister of Municipal and Rural Affairs (MoMRA) launched the Future Saudi Cities Program (FSCP) to tackle urban challenges in Saudi Arabia (Borsekova, Vanova, and Vitalisova Citation2016; UN-Habitat Citation2019). This initiative is relatively recent and has a core mission of curbing urban sprawl, promoting balanced urbanization, and establishing a decentralized planning framework to foster sustainability in Saudi cities. FSCP’s business objectives are strategically aligned with enhancing the general standard of life, environmental protection, and bolstering rivalry in economic circumstances. The overarching aim is to elevate Saudi cities relating to social and environmental contributions. This aligns closely with the principles of Urban Sustainability Theory, which underscores sustainable urbanization. FSCP’s primary goals for business include enhancing the quality of life, improving business viability, and safeguarding the environment (Sharma et al. Citation2023; UN-Habitat Citation2019).
Urban sustainability refers to the concept of constructing and maintaining a city environment that satisfies the requirements of today without affecting future generations’ ability to satisfy their own needs (Spiliotopoulou and Roseland Citation2020; Zeng et al. Citation2022). It requires a comprehensive strategy for the development of cities that considers economic, social, and environmental factors to create cities that are resilient, inclusive, and environmentally responsible. The key component of urban sustainability involves residents’ community engagement and participation in decision-making processes (Horgan and Dimitrijević Citation2019). Participatory governance ensures that community members have a say in shaping the urban environment, contributing to a sense of ownership and responsibility. In addition, urban sustainability prioritizes social equity, ensuring that all residents have access to basic services, opportunities, and a high quality of life (Pineo Citation2022; Spiliotopoulou and Roseland Citation2020). Inclusive urban planning considers the needs of diverse populations, addressing issues of affordability, accessibility, and social cohesion.
In the framework of urban communities, resilience denotes a city’s ability to withstand disasters, and adapt to, and recover from various challenges and shocks (Cañavera-Herrera et al. Citation2022). Disasters caused by nature such as hurricanes, earthquakes, and floods, as well as man-made crises, can all pose significant obstacles. In the wake of relentless urbanization and the escalating global environmental crisis, the quest for more sustainable and resilient urban development has become an imperative shared by nations worldwide. Smart cities and resilience are closely interconnected. As Smart city technological advances and techniques are being adopted, they could contribute to building more resilient urban communities (Cañavera-Herrera et al. Citation2022; Gil-Garcia, Pardo, and Nam Citation2015; Oke et al. Citation2020). Smart city technologies and practices can enhance resilience in several ways. For example, advanced data analytics and real-time monitoring can improve early warning systems for natural disasters and other emergencies. Smart city infrastructure can also be designed to be more resilient, with features such as green roofs, permeable pavements, and underground storage systems that can mitigate the impacts of extreme weather events and other hazards. Smart city technologies can also improve the efficiency and responsiveness of urban services, such as transportation, energy, and water management, which are critical for ensuring the resilience of urban communities (Aldegheishem Citation2023; Ardito et al. Citation2019; Gil-Garcia, Pardo, and Nam Citation2015).
The increasing reliance on technology has introduced a spectrum of challenges associated with organizing data, safety, and the creation of digital platforms (Borsekova, Vanova, and Vitalisova Citation2016; Yigitcanlar et al. Citation2020). Smart city systems are susceptible to cybersecurity threats. A successful cyber-attack on critical infrastructure could have severe consequences, impacting both the functionality of the city and potentially causing harm to residents. Overreliance on technology may result in a loss of traditional skills and knowledge. In the event of technology failures or disruptions, the city’s functionality could be compromised. However, these challenges exhibit global variations; and have thrown additional costs on urban authorities in the Middle East region, particularly in cities; contending with limited resources and insufficient infrastructure for transitioning to smart urbanization. The current situation in Middle Eastern cities necessitates exploring solutions to address emerging urban challenges (Aldegheishem Citation2023; Borsekova, Vanova, and Vitalisova Citation2016). As a consequence, numerous cities in recent times have launched initiatives to enhance urban sustainability, with a specific focus on positively impacting their economies. Many of these solutions harness Information and Communication Technology (ICT) to facilitate the transformation of cities into smart ones. As opined by Pereira et al. (Citation2017), the innovative use of ICT, especially when combined with open data, holds opportunities to improve the effectiveness of urban infrastructure and services. Therefore, smart urban areas foster an open and collaborative setting that fosters the creation of novel ideas while actively engaging residents in procedures for service (Petrovic´, Milic´, and Prlincˇevic´ Citation2022). This approach not only provides access to government open data but also promotes transparency, furnishes new insights, and contributes to the optimization of public value delivery in various domains within the smart city context (Petrovic´, Milic´, and Prlincˇevic´ Citation2022).
This research embarks on a crucial exploration of this paradigm shift, with a focused lens on Najran City of the Kingdom of Saudi Arabia. As the nation rapidly urbanizes, grappling with the challenges and opportunities of a dynamic modernization process, understanding how smart cities can drive environmental sustainability becomes a paramount consideration. Saudi Arabia, characterized by its rich heritage, vast landscapes, and profound economic ambitions, is experiencing a profound urban transformation (AlQuhtani Citation2023; Najran Municipality Citation2019). The convergence of factors such as population growth, industrialization, and economic diversification has precipitated the swift evolution of its urban centers. However, with this growth comes an array of complex challenges, foremost among them being the urgent need for sustainable urbanization. As Saudi Arabia strides towards its ambitious Vision 2030 goals, the intersection of smart city initiatives and environmental sustainability becomes not only relevant but also a strategic necessity. This study aims to evaluate the following objectives in Najran City: (i) Assessing the Impacts of Smart Living on Urban Sustainability; (ii) Examining the Influence of Citizens’ Participation on Urban Sustainability; (iii) Investigating the Effects of Smart Building on Urban Sustainability; (iv) Analyzing the Relationship between Smart Economy and Urban Sustainability; (v) Understanding the Role of Smart Mobility and Smart Governance in Urban Sustainability; (vi) Exploring Interconnected Impacts on Smart Development.
The study employs quantitative research using primary and secondary data to investigate the objectives. It delves into the nexus between urbanization and environmental sustainability within the context of Saudi Arabia, offering a comprehensive examination of the opportunities, challenges, and insights that smart cities bring to the limelight. By scrutinizing the factors driving the transition towards smart urban environments and their tangible impact on the ecological balance, this research endeavors to illuminate a viable path that harmonizes the aspirations of modernity with the imperative of safeguarding the environment. This study contributes by bridging the gap between smart urbanization and environmental sustainability, offering practical insights from a real-world context, and providing a road map for lawmakers and city planners to build healthier and more resilient cities. Its potential impact reaches not only within Saudi Arabia but also urban development efforts worldwide.
The manuscript is of three sections. The second section informs the readers about the background of the study such as the environmental sustainability, smart city, smart buildings, and the urban sustainability Theory (UST). The third section shows the research methodology, while the last section four presents the study’s findings and conclusion. Our quest is not only to dissect the complexities of this dynamic paradigm, but also to offer beneficial perspectives that help improve policies, and decision-making and, eventually, lead to the development of sustainable, resilient, and environmentally responsible cities.
2. Background to the study
2.1. Urban sustainability through the smart city
The concept of sustainability is defined as a state of security, adaptability, and connectivity that allows the social system to meet its objectives (Bibri and Krogstie Citation2020; Ifere and Abim Citation2019; Zakka et al. Citation2017). The potential of smart cities to contribute to the attainment of Sustainable Development Goals (SDGs) has been documented by scholars (Schraven, Joss, and De Jong Citation2021; Sharifi et al. Citation2024). Regardless of the specific dynamics characterizing the relationship between smart cities and SDGs, Yigitcanlar et al. (Citation2020) stress the importance of recognizing the interconnectedness of sustainability and smartness in the urban development trajectory. Neglecting this association, they argue, may lead to risks such as an undue prioritization incentive over sustainability in the long run goals. Smart city initiatives are significant in enhancing urban sustainability, by leveraging technology to optimize urban services and infrastructure, improve resource management, and reduce environmental impact. For example, Smart energy management systems can enhance the efficiency of energy utilization within buildings; and public spaces, reducing energy consumption and associated greenhouse gas emissions. Smart transportation systems can promote sustainable mobility, by encouraging the use of public transportation, electric vehicles, and active modes of transportation such as cycling and walking (Ardito et al. Citation2019; Offiong and Dibie Citation2014). Development in the context of environmental considerations refers to the investigations, amendment, and modification of the environment to produce anticipated outcomes for raising human living standards, achieving economic or institutional objectives, or addressing issues within the context of modernization (Bassey Citation2019; Zubairu Citation2020).
Within the aforementioned frameworks, urban sustainability and development, promote the idea of exploring, adventuring, altering, and manipulating the environment to raise the standard of living and coexistence of humans and other living things without causing harm to the environment or disrupting the ecosystem (Agboola, Zakka, and Zango Citation2015; Bibri and Krogstie Citation2020; Zakka et al. Citation2017). Researchers claimed that urban planning is a critical factor to consider in a bid to reduce catastrophe risk and worldwide response to climate change adaptation (Moglia, Podkalicka, and McGregor Citation2018; Pelling and Manuel-Navarrete Citation2011). Urban sustainability is a crucial consideration in the growth and development of smart cities (AlQuhtani Citation2023; Sharma et al. Citation2023). It could improve the use of smart city growth; which allows residents’ quality of life and a city’s efficiency and sustainability (Gil-Garcia, Pardo, and Nam Citation2015, Sharifi, Kawakubo, and Milovidova Citation2020; Gonzalez, et al., (Citation2020). Smart cities therefore utilize cutting-edge technologies and data-driven solutions to elevate the quality of life for their inhabitants, enhance urban services and infrastructure, and champion sustainable development as shown in .
Figure 1. Hub of the Smart sustainable cities. Source: Adapted from Bibri, and Krogstie (Citation2020) ; Alamoudi et al. (Citation2023).
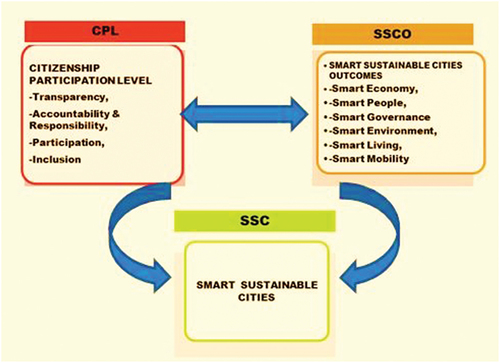
However, as cities continue to grow and expand, the impact of urbanization becomes a pressing issue, with concerns over energy usage, the release of greenhouse gases, the generation of waste, as well as air and water pollution. In addition to these specific initiatives, the adoption of smart city technologies can promote broader sustainability goals, by enhancing citizen engagement and participation, promoting sustainable economic development, and improving the overall livability of urban environments (Martina et al. Citation2019; Spiliotopoulou and Roseland Citation2020). The concept of Smart Sustainable Cities has recently emerged in academic discourse as a concerted effort to advance urban sustainability through the integration of smart city principles (Bibri Citation2021; Gil-Garcia, Pardo, and Nam Citation2015; Sharifi, Kawakubo, and Milovidova Citation2020). Central to this discourse is the notion of incorporating citizens’ participation levels into decision-making processes, sparking extensive global deliberation. As elucidated by Narayan (Citation2002), the promotion of public participation, heightened transparency, capacity building within marginalized communities and socio-cultural dimensions serve as both mechanisms and indicators for evaluating public engagement. The governance of participation encompasses the mechanisms of involvement and dialogue, facilitating public input in the policy formulation and implementation phases, rectifying deficiencies in policy design, and fostering social inclusion (Petesch, Smulovitz, and Walton Citation2005; Simonofski et al. (Citation2019). In this context, the comprehensive framework for smart cities encompasses six primary domains: Smart Economics, Smart Environment, Smart Governance, Smart People, Smart Living, and Smart Mobility.
The Smart cities are designed with the ambitious goal of improving efficiency, sustainability, and overall quality of life. However, the implementation of smart city initiatives comes with a set of negative aspects and trade-offs that necessitate acknowledgment for a comprehensive understanding of potential downsides (Gil-Garcia, Pardo, and Nam Citation2015; Sharifi et al. Citation2024; van Zoonen Citation2016). Several challenges exist in the context of sustainability and sustainable development. One significant concern is the extensive reliance on surveillance and data collection mechanisms in smart cities. Utilizing various sensors and devices, these systems raise substantial privacy issues, leaving citizens feeling constantly monitored (Edge et al. Citation2020; Jay Citation2013; Talari et al. Citation2017). Additionally, the implementation of smart technologies may inadvertently deepen existing social and economic inequalities, creating a digital divide where not everyone has equal access to technological benefits.
The rapid deployment of technology in smart cities contributes to electronic waste, posing environmental challenges in terms of disposal and recycling. Furthermore, the infrastructure requirements for smart cities, including data centers and constant connectivity, can result in increased energy consumption, potentially undermining the intended sustainability benefits (Xie et al. Citation2019, Gonzalez, et al., (Citation2020). The interconnected nature of smart city systems introduces cybersecurity threats, with the vulnerability to data breaches that could compromise sensitive information and disrupt essential city services. Heavy reliance on technology creates dependencies, making systems susceptible to failure or compromise, leading to widespread and severe consequences for various aspects of city functioning.
Another concern is the overreliance on automation, risking the loss of traditional skills and potentially causing unemployment and a decline in cultural practices. The substantial initial costs associated with implementing smart city infrastructure can divert financial resources from other essential social services and projects (Edge et al. Citation2020; Gil-Garcia, Pardo, and Nam Citation2015). In some cases, smart city initiatives may lack citizen engagement, failing to involve residents adequately in decision-making processes. This can result in a lack of understanding and acceptance among the population. Moreover, the rapid pace of technological advancements may render smart city solutions obsolete quickly, leading to a continuous cycle of upgrades and replacements, contributing to electronic waste. To address these challenges, it is crucial for smart city initiatives to prioritize inclusivity, transparency, and long-term sustainability (Gil-Garcia, Pardo, and Nam Citation2015; Thornbush and Golubchikov Citation2019). Incorporating feedback from citizens and considering the broader societal implications of these technologies can contribute to more effective and socially responsible smart city development.
2.2. Urbanization and development of smart cities in Saudi Arabia
Ensuring sustained economic growth and global industrialization hinges on the significance of urbanization (Henderson Citation2005; Pineo Citation2022). Urbanization is pivotal in providing individuals and businesses with improved access to knowledge and information (Henderson Citation2005; Ochoa et al. Citation2018). The ongoing rise in urbanization is accompanied by an agglomeration process in urban areas, driven by enhanced public services, a robust labor market, and diverse goods (Krugman Citation1991; Pineo Citation2022). However, this expanding urbanization also brings about a heightened ecological footprint in economic activities. Consequently, the contemporary world faces the dual challenge of managing the surge in urbanization while ensuring environmental sustainability. In response to this scenario, the attention of academic researchers and policymakers has shifted towards recognizing the importance of smart urbanization.
Amidst rapid urbanization and a growing imperative for sustainable and efficient urban development, smart city initiatives have garnered considerable global attention. According to Al Jaafreh and Allouzi (Citation2023); the Kingdom of Saudi Arabia, standing as one of the fastest-growing economies in the Middle East, has discerned the transformative potential of smart cities in elevating the quality of life, optimizing resource management, and fostering economic growth. Given this, acknowledging the pressing need for sustainable urban development, the Saudi Arabian government has strategically positioned smart cities as a pivotal element within its Vision 2030 plan, designed to diversify the economy and enhance the well-being of its citizens (World Bank, Citation2017). This underscores a deliberate effort to align with global trends and leverage technological advancements to address urban challenges. As highlighted by Al Jaafreh, and Allouzi (Citation2023); a comprehensive understanding of the drivers, challenges, and opportunities associated with smart city adoption in Saudi Arabia is indispensable. This knowledge serves as a crucial foundation for informed policy-making and the effective implementation of smart city initiatives, aligning with the overarching goals of Vision 2030.
The notion of smart cities has garnered international recognition as a prospective remedy for the difficulties arising from swift urbanization and limited resources (Al Jaafreh and Allouzi Citation2023; Gonzalez et al. Citation2020). Smart cities signify a fundamental change in urban development, incorporating information and communication technologies (ICT) to amplify efficiency, sustainability, and the overall quality of life within these urban centers (Abdullahi, Pradhan, and Mojaddadi Citation2018; Mawkhlieng and Debbarma Citation2018). These cities utilize digital technologies, data analytics, and connectivity to enhance diverse facets of life and the environment, including improved transportation, energy management, waste management, healthcare, governance, and increased engagement of residents. However, the implementation of advanced technologies may widen the digital divide, leading to unequal access to smart city services (Ardito et al. Citation2019; Gil-Garcia, Pardo, and Nam Citation2015; Sharifi et al. Citation2024). This disparity could result in marginalized communities being left behind, exacerbating social and economic inequalities.
Saudi Arabia has seen the birth of the notion of a smart city in recent decades, which includes essential features such as a smart economy, smart environment, smart governance, smart people, and smart lifestyle. This evolution of the smart city idea in the countless pieces in Saudi literature can be dated back to e-government, which marked the initial steps towards introducing digital tools and smart approaches within Saudi cities. Some years ago, the concept of a smart city began to gain prominence in Saudi literature, especially in papers highlighting smart development. These documents stressed the critical importance of urban intelligence in boosting many economic elements such as commerce, the tourism industry, entrepreneurial efforts, and energy consumption (Alaidroos and Krarti Citation2015; Aldegheishem Citation2023; Komninos Citation2015; Shiraishi and Yabe Citation2014). Notably, the deployment of smart infrastructure and IoT devices might increase overall energy consumption. Data centers, sensors, and other components of a smart city can contribute to a higher demand for energy, potentially offsetting gains in sustainability.
The Saudi Vision 2030 is a significant turning point rooted in e-government principles. This catalyzed a surge in studies delving into the concept of smart cities. Scientific papers began exploring the fundamental characteristics of smart cities and delved into discussions on smart mega projects in Saudi cities, dissecting their impact on the urban landscape (Alanazi Citation2023; Almulhim et al. Citation2022; Doheim, Farag, and Badawi Citation2019). These endeavors fostered a deeper technical comprehension of the smart urban concept, accompanied by the exploration of smart technologies and their application in various urban domains (Bibri Citation2021; Bibri and Krogstie Citation2020). Also, the emphasis on highlighting the challenges and opportunities associated with transforming Saudi cities into smarter entities was given thorough consideration. The focus of the literature has recently shifted towards assessing smart initiatives to effectively understand the smart urban concept. Consequently, numerous studies have focused on individual elements of smart cities within the broader urban context. This includes in-depth examinations of smart environments, mobility solutions, governance structures, lifestyles, and economic facets (Bibri and Krogstie Citation2020; Borsekova, Vanova, and Vitalisova Citation2016).
3. Theoretical framework and hypothesis development
3.1. Theoretical framework
The smart urban is a novel arrangement of urban environments based on a set of interconnected qualities to promote inhabitants’ quality of life. Similarly, Smart urban ideas possess the required comfortability index that would make living in the cities worthwhile (Borsekova, Vanova, and Vitalisova Citation2016; Grossi and Pianezzi Citation2017; Agboola, et. al., Citation2023). Notably, achieving the smart city concept requires an integrated planning approach (Aldegheishem Citation2023; Jones Citation2018). ICTs are now widely used in municipal administration and administration, where they are used as assets and instruments to improve living standards, attain sustainable development, and protect the environment with the help of a variety of players (Agboola et al. Citation2022; Anthopoulos, Janssen, and Weerakkody Citation2015). The recently created city technology evolved from this perspective. When studying the literature, it is evident that ICT represents the infrastructures and new technologies, constituting smart growth principles within the governance and administration of urban regions (Agboola, Bashir, et al. Citation2023; Bifulco, Amitrano, and Tregua Citation2014; Feldman and Audretsch Citation1999), conceptualisations (Anttiroiko, Valkama, and Bailey Citation2013; J. H. Lee, Hancock, and Gand Hu Citation2013). Conventional cities are becoming smart cities all around the world by utilizing the potential of IoT. The smart city indicators and the significance of Information and communication technology (ICTs) in management are essential in creating urban sustainability ().
Figure 2. ICT Inclusion in Urban Management. Source: Wlodarczak (Citation2017).
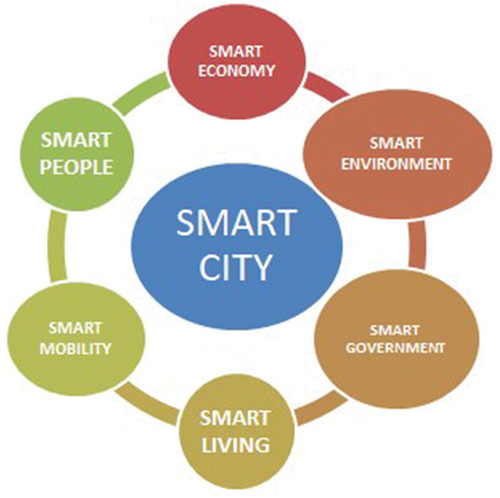
The linkages between sustainability and the smartness of cities are integral components in shaping urban development for the future. The implementation of smart city solutions presents both co-benefits and trade-offs in the pursuit of Sustainable Development Goals (SDGs). For instance, Sharifi et al. (Citation2024) highlight that the responsible development and deployment of smart city technologies have the potential to contribute significantly to SDG progress. In addition, Smart cities offer advantages such as fostering economic growth, enhancing operational efficiency, fostering innovation, and increasing citizen awareness. These positive aspects suggest that smart cities can play a pivotal role in driving the shift toward sustainable development and effectively addressing environmental challenges. However, realizing these benefits involves navigating various trade-offs; such as privacy and cybersecurity issues, the associated financial burden for infrastructural upgrades, the digital divide, and the misuse of artificial intelligence (Ismagilova, et. al., Citation2020; van Zoonen Citation2016). Thus, a holistic and responsible approach is essential to harness the positive potential of smart city solutions while proactively mitigating the negative consequences associated with these complex urban technologies.
Urban Sustainability Theory (UST) underpins the concept of sustainable urbanization, as exemplified by the Future Saudi Cities Program (FSCP), with a specific focus on three primary areas as core business objectives, as outlined by UN-Habitat (Bibri and Krogstie Citation2020; UN-Habitat Citation2019). These three key aspects are i) quality of life, ii) economic competitiveness, and iii) environmental protection. Social sustainability, as it relates to enhancing the quality of life, centers on the promotion of high-quality urban design (UN-Habitat Citation2019). This approach strives to create cities that are not only prosperous but also productive, equitable, socially inclusive, and environmentally sustainable. Additionally, it underscores the importance of establishing adequate and efficient infrastructure to support these aspirations effectively. Environmental protection, another integral dimension of Urban Sustainability Theory, entails a commitment to curtailing urban sprawl and championing spatially balanced development (Bibri and Krogstie Citation2020; UN-Habitat Citation2019).
This endeavor aims to safeguard the environment from the adverse effects of unchecked urban expansion. Furthermore, the third pillar of “economic competitiveness” within this framework is devoted to the generation of improved financing mechanisms, elevated well-being for residents, and the creation of enhanced employment opportunities (Bibri and Krogstie Citation2020; UN-Habitat Citation2019). In essence, it seeks to bolster the economic vitality of urban areas while ensuring that these benefits are equitably distributed among the populace. Smart buildings are revolutionizing the way we design, construct, and manage real estate properties (Olsthoorn, Haghighat, and Mirzaei Citation2016). Meanwhile, the rapid evolution of technology can lead to a significant amount of electronic waste. The disposal of outdated or non-functional smart devices may contribute to environmental pollution and sustainability challenges if not managed properly. On a positive note, these innovative structures leverage cutting-edge technology and data-driven systems to enhance efficiency, sustainability, comfort, and security for occupants and owners alike. They represent a significant shift in the real estate industry towards more intelligent and responsive infrastructure. presents the essentials of the building system and its functionalities.
Table 1. Essentials of the Smart Building System and Functionalities.
3.2. Hypothesis development
While numerous studies have employed diverse elements to gauge the smart city paradigm, a comprehensive examination of these assessment frameworks underscores the inherent heterogeneity of this concept (Anthopoulos, Janssen, and Weerakkody Citation2015; Gil-Garcia, Pardo, and Nam Citation2015). Despite this diversity, a common thread runs through these frameworks, emphasizing the utilization of various information and communication technologies (ICT) across six dimensions such as economy, mobility, environment, people, living, and governance as depicted in , with the overarching goal of enhancing the sustainability of urban environments. Various models have emerged to quantify the smartness of urban areas. Carli et al. (Citation2013), for instance, proposed a conceptual framework centered on smart city performance indicators, bifurcated into two key areas. The first area scrutinizes the physical infrastructure, urban services, and environmental aspects of a city, while the second delves into residents’ satisfaction and well-being. This delineation reflects an attempt to capture the multifaceted nature of smart city initiatives and their impact on both the urban environment and the quality of life for residents.
3.2.1. Hypothesis (H1): the impacts of smart living on urban sustainability
Smart living refers to the idea of living in a way that leverages technology and innovation to enhance the quality of life (Chen and Chang Citation2009; Saeedi et al. Citation2023). It often involves using advanced technologies to make daily life more convenient, efficient, and sustainable. Smart living can encompass various aspects of life, including smart homes, transportation, healthcare, and more. On the other hand, Urban Sustainability pertains to the sustainability of urban environments, particularly in cities (Bibri Citation2021; Sharifi, Kawakubo, and Milovidova Citation2020). Urban sustainability involves implementing strategies and technologies to make cities more environmentally friendly, economically viable, and socially inclusive. It often includes initiatives like energy-efficient infrastructure, waste reduction, green transportation, and social equity programs. Studies have shown elements of smart city development in a broader context of urban life, such as smart living (Almulhim et al. Citation2022; Gil-Garcia, Pardo, and Nam Citation2015); for urban sustainability (Mohamed, ALSurf, and AL-Kesmi Citation2022), and Smart living (Chen and Chang Citation2009; Latif et al. Citation2020). Thus, hypothesis (H1) is generated, testing the impacts of smart living on urban sustainability.
3.2.2. Hypothesis (H2): the impacts of citizens’ participation on urban sustainability
Public participation is a fundamental component of smart urban development. It not only ensures that development initiatives fit with the necessities and goals of the community; but also fosters transparency, inclusivity, and accountability. By proactively engaging the public in the process of making choices, cities can work towards more sustainable, resilient, and habitable urban surroundings that benefit all their residents. In a separate investigation conducted by Jiang et al. (Citation2020), and Alamoudi et al. (Citation2023). The study emphasized the crucial significance of public participation as a primary goal in achieving smart urban development. Within the framework of smart cities, public participation through the use of technology plays a vital role in promoting transparency through the online accessibility of information while ensuring privacy protection and improving transparency at every stage of the decision-making process (Priano and Guerra Citation2014; Spiliotopoulou and Roseland Citation2020). Thus, citizen participation is directly related to smart urban development, hypothesis (H2).
3.2.3. Hypothesis (H3): the impacts of smart building on urban sustainability
Dirks and Keeling (Citation2009); concentrate on the harmonization of diverse systems within a city; such as buildings and physical infrastructure, in sustaining a smart city. The integration of buildings and physical infrastructure, plays a pivotal role in sustaining a smart city (Alamoudi, Abidoye, and Lam Citation2022; Gil-Garcia, Pardo, and Nam Citation2015). A smart city uses technology and information-driven strategies to improve the quality of life of its citizens, increase operational effectiveness and encourage sustainability. The integration of buildings and physical infrastructure allows for more effective maintenance and optimization (Ghaffarianhoseini et al. Citation2016; Keshavarzi, Yildirim, and Arefi Citation2021. For instance, sensors within buildings can monitor structural integrity and report any issues in real-time, ensuring that infrastructure remains safe and well-maintained. The interconnectivity of various systems within the urban landscape is a fundamental concept in achieving these goals. Hence, smart building is directly related to smart urban development, hypothesis (H3).
3.2.4. Hypothesis (H4): the impacts of smart economy on urban sustainability
Smart economy according to Grab and Ilie (Citation2019) scrutinized how innovative digitalization of business management contributes to shaping the economic landscape of smart cities. Additionally, Ardito et al. (Citation2019) and Asmyatullin et al. (Citation2020) define a smart economy as having a symbiotic relationship with urban sustainability. Economic growth, job creation, innovation, and prosperity are intricately linked to the sustainable development of urban areas. By adopting practices and policies that prioritize sustainability, cities can not only improve the quality of life for their residents but also strengthen their economic foundations, positioning themselves for long-term success in an increasingly interconnected and environmentally conscious world. Thus, the smart economy is directly related to urban sustainability, hypothesis (H4).
3.2.5. Hypothesis (H5): the impacts of smart mobility on urban sustainability
As illustrated by Alanazi (Citation2023) and Aljoufie and Tiwari (Citation2022), improving smart mobility should be accompanied by improvements in smart services such as vehicle data, applications for mobile devices, and web-based platforms. The relationship between smart mobility and smart urban development is synergistic. By implementing intelligent transportation solutions, cities can reduce congestion, improve environmental sustainability, enhance the quality of life, stimulate economic growth, and promote social inclusivity (Bamwesigye and Hlavackova Citation2019; Chatti Citation2021; Maldonado Silveira Alonso Munhoz et al. Citation2020). These elements are essential for creating more efficient, livable, and sustainable urban environments. Thus, smart mobility is directly related to smart urban development, hypothesis (H5).
3.2.6. Hypothesis (H6): the impacts of smart governance on urban sustainability
Smart urban governance according to Alamoudi et al. (Citation2023), and Alswedani et al. (Citation2022); is a fundamental requirement for achievement in shifting to smart cities; and is defined by civic engagement and public-private cooperation, as well as various interest groups. Smart urban governance is an essential component in sustaining a smart city. It involves the efficient and effective management of city resources, infrastructure, and services while leveraging technology, data, and citizen engagement to create a sustainable and prosperous urban environment. Thus, smart urban governance is directly related to smart urban development, hypothesis (H6).
3.2.7. Hypothesis (H7): assessing the impacts of smart living on smart economy
Smart living has far-reaching impacts on the smart economy by creating an environment where technological innovation, economic opportunities, urban sustainability, and social inclusivity are prioritized (Anthopoulos, Janssen, and Weerakkody Citation2015; Gonzalez, et al., (Citation2020). These factors, combined, lead to an improved quality of life, convenience, and overall well-being for residents, making smart living a desirable and achievable goal in such economies. Smart living has several significant impacts on the smart economy, creating a synergistic relationship between economic prosperity and the overall quality of life (Ahvenniemi et al. Citation2017; Gonzalez et al. Citation2020). Hence, smart living is directly related to the smart economy, hypothesis (H7).
3.2.8. Hypothesis (H8): examining the impacts of citizens’ participation on smart living
Citizen participation has a profound impact on smart living, fostering a dynamic and inclusive environment in which residents actively engage in shaping their communities (Alamoudi, Abidoye, and Lam Citation2022. Citizen participation is a driving force behind the success of smart living. It empowers citizens to shape their communities, promotes transparency and accountability in governance, and ensures that smart living initiatives are aligned with the needs and preferences of the residents (Chen and Chang Citation2009; de Melo Cartaxo Citation2021). By actively engaging in the process of building and sustaining smart cities, citizens become key stakeholders in creating vibrant, inclusive, and technologically innovative urban settings improve everyone’s quality of life. Therefore, citizen participation is directly related to the smart living, hypothesis (H8).
3.2.9. Hypothesis (H9): investigating the impacts of citizens’ participation in smart building
Citizen participation can have significant impacts on the development and operation of smart buildings, contributing to a more sustainable, efficient, and user-friendly built environment (Narayan Citation2002; Paulussen et al. Citation2007). Citizen participation plays a vital role in shaping the future of smart buildings. By actively engaging in the planning, design, and operation of these buildings, citizens can help create user-centric, environmentally friendly, and inclusive environments (Ghaffarianhoseini et al. Citation2016; Kim and Lee Citation2019). This enhances the quality of life and contributes to the broader goals of smart, sustainable, and resilient urban development. Thus, citizen participation is directly related to smart building, hypothesis (H9).
4. Methodology
4.1. Study area and study sample
Najran City, situated in the southwestern region of Saudi Arabia, occupies an expansive area of approximately 885 square kilometers (Najran Municipality Citation2019). It comprises a network of 78 residential districts, as visualized in . As of 2019, the city’s population stood at 454,035 inhabitants (Najran Municipality Citation2019). It is important to note that population density across Najran City exhibited variations among its districts. Near the city center, the population density reached around 174 individuals per hectare. However, as one moves away from these core areas, population density gradually decreases to roughly 48 persons per hectare in other parts of the city. Notably, in a substantial portion of Najran’s city, particularly those situated in the eastern region, population density plummeted to less than five persons per hectare. Najran has embarked on a forward-looking trajectory, leveraging advanced technologies and data-driven solutions to transform its urban landscape into a model of resilience and environmental consciousness (AlQuhtani Citation2023; Cañavera-Herrera et al. Citation2022).
Figure 4. Najran city districts. Satellite image. Source: AlQuhtani (Citation2023).
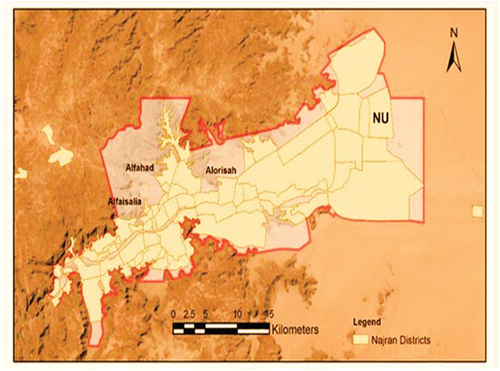
This transformation is in line with global sustainability objectives, emphasizing the city’s commitment to a sustainable future. A pivotal aspect of Najran’s strategy lies in its recognition of the paramount importance of involving its residents in shaping the city’s trajectory. To achieve this inclusive approach, Najran according to AlQuhtani (Citation2023), has established digital platforms and communication channels that facilitate active community participation and engagement. By providing residents with the means to voice their perspectives and ideas, the city not only empowers individuals but also cultivates a sense of ownership and co-creation in decisions about urban development. This collaborative ethos is instrumental in forging a dynamic and responsive urban ecosystem. Najran’s endeavors in urbanism and smart city initiatives do not merely reflect a commitment to innovation but actively contribute to fostering an innovation ecosystem within the city. This ecosystem serves as a nurturing ground for creativity and technological advancement. By nurturing local talent, supporting entrepreneurship, and fostering partnerships with educational institutions and the private sector, Najran is poised to cultivate cutting-edge solutions that will further enhance its smart city capabilities.
4.2. Data collection and measurement of variables
4.2.1. Data collection
A quantitative study approach was utilized to analyze the influences of a smart environment on its sustainability. In previous works, Ajibade et al. (Citation2018), Agboola et al. (Citation2022) and Creswell (Citation2012) employed this approach to document the relationship between the many variables of a scientific data gathering instrument. As a result, our research focused on a few cutting-edge ways to improve Najran’s development of smart cities and structures. Quantitative data collection methods in this study were conducted online and through traditional means from 22 June 2022, to 28 August 2022. These methods are crucial for gathering numerical data that can be analyzed statistically and to draw conclusions and make evidence-based decisions. These methods are associated with diverse advantages such as reaching wider respondents; achieving higher response rates, and in-depth data collection is made possible.
The respondent’s sample of the study was selected from the professionals residing in Najran city and its environment, such as the Town planners 48 (9.81%) of respondents, while contract managers, civil engineers, and architects make up 88 (17.99%); 62 (12.67%); and 51 (10.42%) of respondents, respectively. Project managers 68 (13.90%) and Quantity surveyors each make up 63 (12.88%) of the respondents, Telecommunication and electrical engineers make up 53 (10.83%), and Data and information technicians make up 56 (11.45%). These were selected experts; who already have an understanding of smart cities and buildings. Due to a lack of accurate professional lists, convenience sampling techniques were applied to contact the respondents. Convenience sampling is often more time-efficient and cost-effective compared to other sampling methods. It allows researchers to quickly gather data without the need for an extensive recruitment process or significant financial resources. This is particularly beneficial when conducting surveys or assessments with limited time and budget constraints. This sampling method according to Creswell (Citation2012); is a non-probability sampling technique often employed in research studies, especially when it is challenging to access a specific population or when the population of interest is not well-defined. The choice of convenience sampling was appropriate as corroborated by previous studies by Akinola, et al., (Citation2020); Huck and Monstadt (Citation2019), and Kaluarachchi (Citation2021). This research requires insights from individuals who are directly impacted by local policies, initiatives, and developments. Convenience sampling is appropriate when seeking the perspectives of residents within a specific city or community, where the primary focus is on understanding local dynamics and experiences.
4.2.2. Measurement of variables
A comprehensive questionnaire survey was undertaken to gain a well-informed perspective from respondents who possess expertise in the execution of smart environment initiatives, as outlined in the study by Pratama and Imawan (Citation2019), Agboola et al. (Citation2022) and Sharifi et al. (Citation2020). This survey also facilitated an exploration of the research objectives within the specific context of Saudi Arabia. Questionnaire distributions were carried out in the study’s locations during April and May of 2023. A closed-ended questionnaire that addressed the study’s predetermined objectives was used to solicit respondents’ opinions in this investigation. To verify content validity, we used measuring scales from prior studies (Pratama and Imawan Citation2019; Sharifi, Kawakubo, and Milovidova Citation2020). Some of the variables were slightly modified to fit our research environment. Urban sustainability assessment looks at the broader aspects of a city’s sustainability in terms of environment, society, and economy, smart city assessment hones in on the integration of technology to enhance efficiency, connectivity, and urban services. Both assessments play crucial roles in shaping the future of cities, ensuring they are both sustainable and technologically advanced. After the changes, all of the items adopted displayed positive statements on five-point Likert-style scales.
The survey was segmented into three sections. The very first segment discussed the participants’ backgrounds. Section two explored the 6 dimensions of factors influencing smart cities, such as smart living (3 items measurement); urban sustainability (5-item measurement); smart governance (3-items measurement); smart mobility (3-item measurement); smart economy (3-Items measurement); and citizen participation (3-Items measurement) as previously measured by researchers. The third section focused on smart buildings (5-item measurement) as presented in . For respondents’ agreement, a ”5-point” Likert scale of “5”-strongly agree, “4”-agree, “3”-neutral, “2”-disagree, and “1”-strongly disagree was used (see Appendix A for the questionnaire measurement items). For the appraisal of the smart buildings, respondents were asked about their knowledge of the following case studies shown in : i), the Park Inn Radisson Najran, ii), the Florida Inn Hotel, and iii) the Najran University. All the case studies buildings and environment were appraised by the respondents, and all have smart features; and provide a higher quality of life for residents and tourists as reinstated by Frost and Sullivan (Citation2023).
Table 2. Measurement of variables.
Table 3. Selected Case Studies Structures in Najran city.
4.2.3. Data analysis and data screening
The data screening and analysis involved the application of both descriptive and inferential statistics, utilizing SPSS and AMOS software tools. As a result, a comprehensive analysis using simple descriptive statistics was carried out. Hair et al. (Citation2020) considered a sample size of 100 or above to be appropriate for variance-based structural equation modeling. As a result, a sample size of 489 was computed using the Raosoft sample size calculator (Citation2023), assuming a 95% confidence level, a 5% margin of error, and a 50% response distribution. Examining missing data is of utmost importance as it can lead to a reduction in the available sample size for analysis. This, in turn, may impact the generalizability of the study’s results. Numerous studies have underscored the significance of addressing missing data, as its neglect by researchers can detrimentally influence the outcomes of empirical research (Cavana, Delahaye, and Sekeran Citation2001). Recognizing the potential negative effects of missing data, this survey proactively implemented preventive measures in the field to mitigate their occurrence. The researcher meticulously reviewed submitted questionnaires to ensure proper completion. Following data entry into SPSS, a preliminary descriptive statistics analysis was conducted to identify the extent of missing data prevalence. It is noteworthy that the missing data in this study was found to be less than 5%.
To address missing data, mean substitution was employed as a remedy due to its ease of execution and time efficiency. The importance of checking and replacing missing data is emphasized, especially since Structural Equation Modeling (SEM) is highly sensitive to any missing values, preventing its proper execution (Hair et al. Citation2019). Another critical aspect of data screening involves identifying and addressing outliers. Outliers are observations that significantly deviate numerically from the rest of the dataset (Sheridan, Lyndall, and Clara Citation2010). Various methods for outlier detection have been proposed in the literature, with one approach being the classification of data points based on their Mahalanobis distance (D2) relative to the research’s expected values (Hair et al. Citation2019). Advocates of outlier treatment based on Mahalanobis distance argue that it is an effective method for outlier detection. The Mahalanobis distance is calculated using predetermined thresholds to determine whether a point should be classified as an outlier (Hair et al. Citation2019).
The data analysis was evaluated in two stages, via measurement and structural modeling. All variables were incorporated in the development of a measurement model and the first synchronized CFA. The second technique considered the use of structural modeling to analyze the connections between the various elements, as shown in the hypothetical framework of the regression of the variables (). The hypothesized relationships according to Sarstedt and Cheah (Citation2019); could be evaluated using structural equation modeling based on the parameter estimation of partial least squares (PLS-SEM) with the aid of Smart Partial Least Square (SmartPLS) software version 3. The hypothesized relationships explore the research objectives in terms of analyzing the contribution of smart city programs to environmental sustainability within urban areas of Saudi Arabia, and exploring the contribution of the smart city and buildings to environmental sustainability in Najran; Saudi Arabia. According to Creswell (Citation2012) and Fetters et al. (Citation2013), this quantitative research technique is ideal for minimizing biased appraisal and discussion. This method is particularly useful when trying to explain complex behavior by reasoning about the interaction of multiple factors Ringle et al. (Citation2015).
First, the validity and reliability of the measurement model were evaluated. The structural model was then tested to ensure that the hypotheses were correct, including the model fit test (Hair et al. Citation2019; Henseler, Hubona, and Ray Citation2016). In addition, the relevance of the path coefficient and load was determined using a bootstrapping approach with 5,000 replicate samples. For the statistical analysis, a normality test was also performed to ensure that the data did not exceed the normality criterion (Henseler, Hubona, and Ray Citation2016; Ringle, Wende, and Becker Citation2015). In any multivariate analysis, the assumption of normality holds significant importance, with researchers typically assuming that the variables follow a normal distribution (Sheridan, Lyndall, and Clara Citation2010; Hair et al. Citation2019). A variable deviating from normal distribution can exhibit high skewness, potentially distorting the relationship between the variables of interest and affecting the significance of test results (Hulland Citation1999). When the overarching goal of the research is inference, screening for normality becomes a crucial step in almost all multivariate analyses (Tabachnick and Fidell Citation2007). The initial examination for normality indicated signs of non-normal data, revealed through visual inspections using stem-and-leaf plots, normal Q-Q plots, and box plots to assess data skewness and kurtosis. Further evidence of non-normality surfaced when calculating Z-score values for each item, with a few cases having Z-values exceeding ± 2 and spanning across variables. To mitigate the presence of abnormal data in this study, the researcher opted for data transformation. Subsequently, after transformation, the skewness and kurtosis values for all items fell within the acceptable range of < 2 and < 7, respectively. For instance, all skewness values are below 1, and similarly, the kurtosis values are below 2, indicating that the data is now considered normal.
5. Results and discussions
5.1. Respondents’ demographics
Following the previously outlined selection criteria, a total of 612 responses were gathered. Subsequently, a sample of 489 valid responses was meticulously collected and subjected to analysis. This gives a total of 79.90% response rate. Based on the analyzed data the results in , revealed that the gender sample of 268 (54.8%) of male, and 221 (45.19%) of female. The respondents’ age showed that 18–30 years at 49 (10.02%); 31–40 years at 156 (31.90%); 41–50 years at 187 (38.20%); and 51 years and above having 97 (19.80%). Similarly, 102 (20.85%) of respondents held a Higher Diploma or certificate; 189 (38.65%) of respondents held a bachelor’s degree, 110 (22.49%) of respondents held a Master’s degree, and 88 (17.99%) had a doctorate. The majority of respondents 142 (29.03%) work as principal contractors, followed by 128 (26.17%) who work for consulting firms, 88 (17.99% who work for information technology companies, 112 (22.90% who work for government agencies, and 73 (14.92%) who work for academic institutions. According to the analysis of respondents’ years of experience 125 (25.56 %) of participants have between one and five years of construction experience, 106 (21.67 %) have six to ten years of experience, 119 (24.33%) have between eleven and fifteen years of experience, and 139 (28.42 %) has sixteen of experience or more.
Table 4. Respondents-demographics.
5.2. Data reliability
We chose partial least squares (PLS) as the estimation approach, which is becoming more popular in smart city literature. Because the model contains both reflecting and formative constructs at the same time, PLS is a suitable approach for testing the hypotheses (Agboola, Bashir, et al. Citation2023; Chin Citation1998). It makes no assumptions about the data’s underlying distribution and therefore does not require huge sample sizes (Henseler, Hubona, and Ray Citation2016). SmartPLS software version 3.0 was used to analyze the data (Ringle et al., Citation2005). The reliability and validity were tested using Cronbach’s alpha on the data and the result showed a value of 0.935. Cronbach’s alpha is a statistical measure that ranges between 0 and 1.0. When Cronbach’s alpha exceeds the threshold of 0.7, it signifies a high level of consistency in participants’ opinions concerning the variables associated with Smart Cities and Environmental Sustainability, as indicated by the research of (Gliem and Gliem Citation2003). Moreover, following the reliability standards set forth by (Nunnally Citation1978), a scale is considered reliable when its Cronbach’s alpha coefficient surpasses 0.5. In our study, all the variables consistently demonstrate elevated reliability values, affirming their dependability in assessing the concepts of Smart City and Environmental Sustainability.
The results as summarized in , present the descriptive analysis of the variables of the smart city and the environmental sustainability. On average, respondents express strong agreement with various variables encompassing Smart City: Smart living (M = 5.5, SD = 1.45); Urban sustainability (Mean = 5.7, SD = 1.72); Smart building and environment (Mean = 5.63, SD = 1.79); and citizen participation (Mean = 5.68, SD = 1.16). A moderate agreement was shown in Smart Governance (Mean = 4.68, SD = 1.76); Smart Mobility (Mean = 4.13, SD = 1.54); Smart Economy (Mean = 4.67, SD = 1.17). All these results collectively reflect a robust consensus among the participants regarding both Smart City and Environmental Sustainability, emphasizing the reliability and consistency of the measurement scales employed in our study.
Table 5. Descriptive Statistics of Variables of Smart City and Environmental Sustainability.
5.3. Results of the measurement model
To test the measurement model, this study used a confirmatory composite analysis (CCA) approach described by Hair et al. (Citation2020). First, the analytical measurements were evaluated for their reliability and convergent validity. As shown in , all metrics for internal consistency reliability (CA, rho_A, and CR) were greater than 0.7. As a result, the measurement’s reliability was satisfactory (Hair et al. Citation2019). Furthermore, all of the constructs had average variance extracted (AVE) values greater than 0.5 (Fornell and Larcker Citation1981), indicating that all of the assessments were convergent. All reflective constructions have composite reliabilities greater than 0.9, indicating their internal consistency. Two criteria were evaluated to confirm discriminant validity. First, as indicated in , good discriminant validity is recognized when the loading of each measurement item on its assigned construct is greater than its loading on any other construct (Chin Citation1998). Second, as indicated in , the square roots of a construct’s AVEs are greater than the correlations between the construct and other constructs in the model (Fornell and Larcker Citation1981). Overall, convergent and discriminant validity are empirically supported, suggesting that the scales have sufficient construct validity.
Table 6. Assessment of Loading, Full Collinearity, Reliability and Convergent Validity.
5.4. Final structural model and hypotheses testing
In total eleven (7) standards constructs of the models include smart living, smart citizens participation, urban sustainability, smart building, smart mobility, smart governance, and smart economy. The stated hypotheses were investigated using Chin’s (Citation1998) bootstrap re-sampling estimation of PLS. The structural model was tested using path coefficients (b) and coefficients of determination (R-square value). R-square values revealed that the independent constructs explained the majority of the variation. Using 1000 bootstrap runs, the significance of all pathways was determined. Path coefficients demonstrated the strength of the relationships between the dependent and independent constructs. The results of the path coefficients with related measures such as standardized deviation, sample means, t-report & p-values after bootstrapping were presented in , whıle the Hypothesıs path coeffıcıents were presented ın . shows the final computed values of the structural model path analysis of PLS estimation; showing the R-squares and the model direction coefficients achieved.
Table 7. Correlation of constructs and the square root of AVEs Confirming the discriminant validity of reflective constructs.
The structural model shows that all the VIF values were well below the threshold of 3.33; with the values ranging from 1.180 to 2.75; as a result, it can be concluded that this model did not exhibit multicollinearity (Hair et al. Citation2019). Next, also shows the hypotheses for each path tested via a bootstrapping technique with 5,000 subsamples (Streukens and Leroi-Werelds Citation2016). The results for the entire dataset reveal that the majority of the proposed hypotheses were statistically significant. For instance, as shown in and Figure 8; for Smart living on Urban Sustainability (H1: β = 0.523; t value = 4.427; p < 0.001); Smart living on Smart economy (H7: β = 0.465; t-value = 0.729; p < 0.001); Citizens participation on Urban Sustainability (H2: β = 0.405; t-value 4.034; p < 0.002); Smart Building & Environment on Urban Sustainability (H3: β = 0.512; t-value 7.753; p < 0.001); Smart economy on Urban Sustainability (H4: β = 0.415; t-value 11.406; p < 0.050); Smart mobility on Urban Sustainability (H5: β = 0.345; t-value 6.918; p < 0.030); Smart Governance on Urban Sustainability (H6: β = 0.307; t-value 5.735; p < 0.010); Smart living on Smart economy (H7: β = 0.465; t value = 7.291; p < 0.001); Smart people on Smart living (H8: β = 0.605; t-value = 6.391; p < 0.003; Citizens participation on smart building (H9: β = 0.406; t-value = 5.241; p < 0.003).
The result in shows the factor loadings and cross-loadings for the measurement model. Meanwhıle, , indıcated the coefficient determinations (R2) of 0.729 for Urban Sustainability, 0.415 for Smart living, and 0.648, for citizen participation. In other words, all of the variables’ R-square values above 10%, show significant predictive ability (Bock, Kankanhalli, and Sharma Citation2006; Hsu and Chang Citation2014). Finally, a blinding procedure was executed to assess the model’s predictive relevance (Q2). Finally, the predictive relevance was tested in SmartPLS using the blindfolding procedure with an omission distance of 7. All of the cross-validated redundancy Q2 values were greater than zero (Tenenhaus et al. Citation2005), indicating that the elements of our model were predictively relevant. The Q2 values for the endogenous variable ranged from 0.287 to 0.825, showing that the model had an adequate level of predictive quality (Hair et al. Citation2019). In assessing the Model fit; the standardized root mean square residual (SRMR) and normed fit index (NFI) are the fit measures adopted in this study to demonstrate model fit in PLS-SEM as suggested by Hair et al. (Citation2019). As equally presented in ; the SRMR value of 0.051, is less than the threshold value of 0.08, and hence can be regarded as a good fit and suitable for PLS path models (Hu and Bentler Citation1999). The NFI value is 0.812, which is likewise considered a good match because it is close to 1 (Bentler and Bonett Citation1980).
Table 8. Factor loadings and cross-loadings for the measurement model.
Table 9. Hypothesis Path coefficients.
Table 10. Coefficients of determination (R2), Predictive Power (Q2), SRMR, and NFI.
6. Discussion and implications
6.1. Assessing the Impacts of Smart Living on Urban Sustainability
The study delves into the assessment of the impacts of smart living on urban sustainability, with the first objective focusing on exploring the relationship between smart city development and urban sustainability. This objective is grounded in the acknowledgment of the existing body of literature that has established a link between modern lifestyles and the sustainability of urban environments (Bibri Citation2021; Mohamed, ALSurf, and AL-Kesmi Citation2022; Latif et al., Citation2022). Building on this foundation, the study seeks to test a specific hypothesis, as outlined in , aiming to determine whether the adoption of smart living practices, such as utilizing energy-efficient appliances in smart homes, directly and positively influences the overall sustainability of urban areas.
The study’s findings support the hypothesis (H1), indicating that promoting smart living practices can indeed contribute to the broader objective of enhancing the sustainability of cities. This aligns with the conclusions drawn in similar studies conducted by Saeedi et al. (Citation2023) and Latif et al. (Citation2022). Drawing connections with these prior studies, it becomes evident that the current research reinforces and extends the understanding of the positive impact of smart living practices on urban sustainability. However, it is crucial to acknowledge potential trade-offs and nuances in the relationship between smart living and urban sustainability. While the study establishes a positive correlation, it is essential to consider contextual factors, varying levels of smart city development, and diverse urban environments that may influence the extent of this relationship. For example, the effectiveness of smart living practices may vary in densely populated urban areas compared to more suburban settings. Findings from other studies are instrumental in gaining a comprehensive perspective. Comparisons with studies by Bibri (Citation2021), Mohamed et al. (Citation2022), and Latif et al. (Citation2022) not only validate the current results but also provide a broader context for interpretation. Exploring potential trade-offs, such as increased reliance on technology leading to potential environmental consequences, would contribute to a more nuanced understanding of the complex dynamics between smart living and urban sustainability.
6.2. Examining the influence of citizens’ participation on Urban sustainability
In addition, Hypothesis 2 affirmed the positive relationships between public participation in achieving smart urban development; about objective two. Hence, this study revealed that public participation plays a crucial role in achieving smart urban development. This is in line with the previous studies by Jiang et al. (Citation2020), and Alamoudi et al. (Citation2023). Public participation is the active involvement of the public, including residents, businesses, and other stakeholders, in the planning, decision-making, and implementation of urban development initiatives. Public participation ensures that local knowledge, needs, and perspectives are considered in the planning and design of smart urban projects (Agboola, Zakka, and Zango Citation2015; Priano and Guerra Citation2014; Sharifi, Kawakubo, and Milovidova Citation2020).
Residents frequently possess valuable insights into the specific challenges and opportunities within their communities, providing a foundation for the creation of more intelligent, contextually relevant solutions. Public participation plays a pivotal role in fostering social inclusivity by amplifying the voices of marginalized or underrepresented groups, thereby addressing issues of social equity and ensuring that the advantages of smart initiatives are accessible to all residents. The involvement of the public in decision-making processes not only enhances transparency and accountability but also diminishes the risks associated with corruption and mismanagement when residents actively contribute to the allocation of resources and project implementation. This collaborative approach builds trust between the government and the public. Additionally, public participation serves as a feedback mechanism, enabling city planners to collect community input on ongoing projects, comprehend their impact, and make necessary adjustments. This iterative process contributes to the continual enhancement of smart urban development strategies.
When citizens, businesses, and local governments collaboratively engage in efforts, co-created solutions emerge that are more attuned to the community’s unique needs and challenges, potentially leading to the development of innovative, context-specific smart solutions. The involvement of residents in resource allocation decisions often results in the directing of resources towards projects aligned with their priorities, such as sustainability, infrastructure improvement, or community services. Beyond the tangible benefits, engaging the public in smart urban development projects serves an educational purpose by raising awareness about the advantages of sustainability, technology, and innovation in urban living. This empowerment of residents with knowledge enables them to make informed choices in their daily lives.
The importance of public participation in smart urban development aligns with findings from past studies that underscore the significance of involving residents in decision-making processes. Bibri (Citation2021) and Nam and Pardo (Citation2011) emphasize the role of citizen engagement in the successful implementation of smart city initiatives. One potential trade-off to consider is the challenge of balancing inclusivity with efficiency. While public participation promotes social inclusivity by giving voice to marginalized groups, it may also lead to longer decision-making processes and potential delays in project implementation. Studies by Caragliu et al. (Citation2011); Castelnovo et al. (Citation2015) and Anthopoulos et al. (Citation2015) have discussed the need for efficient governance structures in smart cities, and a potential tension exists between ensuring inclusivity and maintaining the agility required for swift urban development. Benchmarking the current findings against research by Joshi, et al., (Citation2016) and Cardullo et al. (Citation2018), the discussion should consider the varying degrees of success in achieving social inclusivity through public participation in different urban contexts. Understanding the contextual factors influencing the effectiveness of public engagement is essential for guiding policymakers in diverse environments.
Moreover, potential trade-offs may arise in terms of conflicting priorities. While involving residents in resource allocation decisions aligns with principles of democratic governance, it may lead to competing interests and challenges in reaching a consensus. Giffinger et al. (Citation2007) and Hollands (Citation2008) discuss the complexities of balancing diverse stakeholder interests in smart city projects, emphasizing the need for effective conflict resolution mechanisms. To further benchmark results, the study draws parallels with studies byBoudjelida et al. (Citation2016), and McNeill et al. (Citation2021), which explore the role of public participation in promoting transparency and accountability. Examining the mechanisms used in different cities to ensure accountability in resource allocation and project implementation provides valuable insights into best practices. While public participation in smart urban development has undeniable benefits, it is essential to recognize potential trade-offs related to efficiency, conflicting priorities, and the need for transparent governance. Hence, this study allows for a more nuanced understanding of the complexities involved in citizen engagement and provides valuable lessons for designing effective and context-specific public participation strategies in smart city projects.
6.3. The impacts of smart building on urban sustainability
This study’s Hypothesis (H3) affirmed that smart building is directly related to smart urban development of objective three. The integration of a city’s different systems, including buildings and physical infrastructure, is a cornerstone of smart urban development. This is in line with the study of Keshavarzi et al. (Citation2021) as it facilitates the efficient use of resources, data-driven decision-making, environmental sustainability, enhanced public services, and urban resilience. By seamlessly connecting various elements within the urban landscape, cities can sustainably evolve into smart, efficient, and livable spaces that benefit both residents and the environment. The integrated systems approach holds particular significance in the optimal management of vital resources such as energy, water, and transportation. Drawing parallels with the study by Dirks and Keeling (Citation2009), the integration of energy management systems in buildings, capable of communication with the city’s power grid, exemplifies how smart technology can optimize energy usage and curtail wastage. Likewise, the integration of transportation systems, contributes to the reduction of traffic congestion and fuel consumption, emphasizing the tangible benefits of interconnected urban systems.
Building on the concept of data sharing and analysis, the discussion aligns with the insights from past studies. The generation of substantial data by different city systems becomes more meaningful when these systems are interconnected. This resonates with the findings of studies like those conducted by Kitchin (Citation2014) and Caragliu et al. (Citation2011), emphasizing the transformative potential of data-driven approaches in urban planning. The interconnected systems allow for comprehensive data sharing and analysis, empowering city planners to make informed decisions, adapt to evolving circumstances, and formulate future-oriented plans with greater efficacy. The current study expands upon the role of integrated systems in enhancing urban resilience and sustainability. The insights gleaned from Agboola et al. (Citation2015) and Keshavarzi et al. (Citation2021) provide a contemporary context for understanding the multifaceted advantages of integrated systems. As urban environments evolve, the integration of city systems emerges as not only a technological necessity but a strategic imperative for fostering sustainable, resilient, and forward-thinking cities. The integration of systems, therefore, stands as a linchpin in the ongoing transformation of urban landscapes into smart and adaptive entities.
6.4. Analyzing the relationship between smart economy and Urban sustainability
About research objective four; Hypothesis (H4), smart economy is directly related to urban sustainability. As the smart economy is directly related to urban sustainability; it highlights the strong and interconnected relationship between economic prosperity and the sustainability of urban areas. This replicates the views of the past studies Grab and Ilie (Citation2019) and Le Duc (Citation2022). Urban sustainability, as highlighted in the discussion, resonates with findings from past studies that emphasize the efficient use of resources as a key component. Sharifi et al. (Citation2020) provide insights into the potential cost savings for city governments and businesses through resource efficiency measures. The current studies align with their observations, emphasizing the economic benefits that can accrue to both stakeholders when resource use is optimized. Drawing from Sharifi et al. (Citation2020), potential trade-offs could be explored concerning the initial costs of adopting sustainable practices and technologies. While the long-term benefits are evident, cities may face challenges in the short term, particularly in terms of upfront investments required for the deployment of advanced technologies and infrastructure. Comparative studies by Nam and Pardo (Citation2011) can offer benchmarks to assess the financial implications and trade-offs associated with adopting sustainable urban practices.
The link between sustainability goals and job creation, as discussed, aligns with the findings of Asmyatullin et al. (Citation2020). However, to better elaborate on potential trade-offs, it is crucial to consider the skills gap and potential displacement of certain jobs due to the adoption of green technologies. A comparative analysis with studies by Acemoglu and Restrepo (Citation2018) and Chetty et al. (Citation2020) can shed light on the broader economic implications of transitioning to sustainability-focused job markets. The connection between sustainable urban planning and economic stability in the face of natural disasters and climate change, as mentioned in the discussion, is supported by the work of Asmyatullin et al. (Citation2020) and Le Duc (Citation2022). To better benchmark the results, further exploration of case studies from cities that have experienced resilience challenges and the economic aftermath, such as New Orleans post-Hurricane Katrina, can provide valuable insights into the effectiveness of sustainability-focused planning.
The deployment of advanced technologies in pursuing urban sustainability, including IoT sensors and data analytics, aligns with Sharifi et al. (Citation2020). However, to better discuss potential trade-offs, attention should be given to privacy concerns, cybersecurity risks, and the digital divide that may result from uneven technology adoption. Comparative studies by Moraes, and Cunha (Citation2022) and Kitchin, and McArdle (Citation2016) can offer insights into the challenges and benchmarks associated with the technological aspects of sustainable urban development. While the economic benefits of urban sustainability are evident, a nuanced understanding of potential trade-offs and a thorough benchmarking against a range of studies will contribute to a more comprehensive and actionable perspective. Considering various urban contexts and challenges, coupled with insights from past research, will guide future strategies for sustainable urban development.
6.5. Understanding the role of smart mobility and smart Governance in Urban sustainability
Research objective five captured the impacts of smart mobility on urban sustainability (H5) and the impacts of smart governance on urban sustainability (H6). Meanwhile, smart mobility is directly related to smart urban development which affirms Hypothesis H5. The concept of smart mobility is indeed directly related to smart urban development. Smart mobility refers to the intelligent, efficient, and sustainable movement of people and goods within urban areas (Bamwesigye and Hlavackova Citation2019; Maldonado Silveira Alonso Munhoz et al. Citation2020). This relationship is critical in the context of creating more livable, efficient, and sustainable cities. Smart mobility initiatives, such as integrated public transportation systems and real-time traffic management, help reduce traffic congestion in cities. This not only saves commuters time and frustration but also has economic implications by reducing fuel consumption and productivity losses due to traffic delays (Aljoufie and Tiwari Citation2022; Porru et al. Citation2020). Smart mobility often prioritizes environmentally friendly transportation options, such as electric buses, and pedestrian-friendly infrastructure (Alanazi Citation2023; Aljoufie and Tiwari Citation2022). By reducing carbon emissions and promoting cleaner transportation, cities contribute to a healthier environment and reduce healthcare costs related to air pollution. Efficient and sustainable mobility options lead to a better quality of life for urban residents (Maldonado Silveira Alonso Munhoz et al. Citation2020). Access to reliable public transportation, safe cycling infrastructure, and pedestrian-friendly streets enhance accessibility and convenience, ultimately making cities more attractive places to live and work. Smart mobility planning often involves optimizing existing infrastructure rather than building new roads or expanding highways (Alanazi Citation2023; Ghaffarianhoseini et al. Citation2016). This can lead to cost savings for the city, which can then be allocated to other essential services or infrastructure improvements. Smart mobility initiatives aim to provide accessible transportation options for all residents, including those with disabilities or limited access to private vehicles. Inclusive transportation systems enhance social equity by ensuring that everyone can participate in the economic and social life of the city.
Smart urban governance contributes to sustaining a smart city to Hypothesis H6. Smart urban governance relies on data analytics to make informed decisions about resource allocation, infrastructure development, and service provision. By utilizing data, city leaders can identify trends, predict future needs, and optimize city operations for sustainability (Agboola, Alotaibi, et al. Citation2023; Alamoudi, Rotimi, and Terence Citation2023). Effective governance promotes the effective administration of natural assets such as energy, water, and land (Castelnovo, Misuraca, and Savoldelli Citation2015). Cities may reduce waste and environmental effect by putting in place sustainable strategies like energy-efficient lighting and water conservation. Smart urban governance involves long-term infrastructure planning that prioritizes sustainability. This includes the development of green infrastructure, intelligent transportation systems, and resilient buildings to address environmental challenges and improve the overall quality of life for residents (Agboola, Alotaibi, et al. Citation2023; Alswedani et al. Citation2022). Smart urban governance aims to create inclusive policies that consider the needs of all residents. By addressing social equity, cities ensure that smart city benefits are accessible to everyone, regardless of their socioeconomic status or background. Effective governance involves disaster preparedness and resilience planning. Smart city leaders consider the potential impact of natural disasters and climate change and develop strategies to mitigate risks and respond to emergencies promptly. Smart urban governance often includes collaborative partnerships with private sector organizations, academic institutions, and community groups. These partnerships can drive innovation and create opportunities for sustainable initiatives.
6.6. Exploring interconnected impacts on smart development
Research objective six, captured the hypothesis assessing the impacts of smart living on smart economy (H7), examining the impacts of citizens’ participation on smart living (H8), and investigating the impacts of citizens’ participation on smart building (H9) respectively. First is Hypothesis H7 in which the impact of a smart economy on smart living is supported. The supported Hypothesis H7, affirming the impact of a smart economy on smart living, underscores a significant interconnection between economic dynamics and the lifestyle choices and practices embraced within a smart living framework. The supported Hypothesis H7 aligns with research by Caragliu et al. (Citation2011) and Giffinger et al. (Citation2007), which emphasize the transformative impact of smart technologies on various aspects of urban life. The study was enriched by drawing comparisons with studies that investigate the specific pathways through which a smart economy influences smart living. For example, research by Komninos (Citation2015) and Albino et al. (Citation2015) delves into the intricate relationships between technological advancements, economic structures, and the quality of life in urban environments. This finding aligns with the evolving landscape of smart urban development where the integration of technological advancements into economic structures has profound implications for residents’ daily lives. The notion of a smart economy encompasses the infusion of innovative technologies, data-driven decision-making, and digital connectivity into various economic sectors. As this smart economy takes shape, it has a ripple effect on the way individuals experience and engage with their living environments. The positive correlation between a smart economy and smart living suggests that the advancements and efficiencies achieved in economic activities translate into tangible benefits for residents, influencing their behaviors, choices, and overall quality of life. This finding resonates with the idea that a robust and technologically advanced economy can create an environment that facilitates smart living practices. For instance, a smart economy may stimulate the development and adoption of technologies that enhance energy efficiency, connectivity, and accessibility within residential spaces. The availability of smart infrastructure, coupled with economic prosperity, can contribute to a seamless integration of technology into daily life, fostering a more connected, efficient, and sustainable living experience.
To elaborate on potential trade-offs associated with this impact, one could explore questions related to inclusivity, privacy concerns, and the potential digital divide. Examining the experiences of different demographic groups within the smart living framework can provide insights into whether the benefits of a smart economy are equitably distributed. Comparative studies by Chowdhury, et al. (Citation2018) and Schaffers et al. (Citation2011) may offer benchmarks for understanding the social implications and potential challenges associated with the convergence of a smart economy and smart living. A smart economy often leads to increased income levels and job opportunities, which can enhance the overall quality of life for residents (Anthopoulos, Janssen, and Weerakkody Citation2015; Ahvenniemi et al. Citation2017). Higher-income allows people to access better education, healthcare, housing, and leisure activities. A smart economy supports the growth of advanced services in areas like healthcare, education, and transportation. Residents can benefit from innovative healthcare solutions, high-quality education, and efficient transportation systems, all of which contribute to a higher standard of living. This results in the availability of cutting-edge technologies and products that make daily life more convenient and efficient. Smart homes, connected devices, and automation improve convenience and comfort (Anthopoulos, Janssen, and Weerakkody Citation2015). Smart economies often prioritize environmental sustainability, leading to cleaner air, better waste management, and reduced environmental pollution. This contributes to a healthier living environment and a higher quality of life for residents. A smart economy typically includes measures to ensure that the benefits of economic growth are distributed fairly. Policies aimed at social inclusivity help reduce income inequality and provide opportunities for all residents to participate in the smart living experience. In a smart economy, there is an emphasis on developing smart infrastructure, such as high-speed internet, efficient public transportation, and renewable energy sources. These investments in infrastructure improve daily life by enabling faster connectivity, easier commutes, and cleaner energy sources. Smart living benefits from advancements in safety and security technologies. These may include smart surveillance systems, emergency response networks, and technologies that enhance personal safety, contributing to peace of mind and well-being.
Citizen’s participation influences and enhances smart living affirmed Hypothesis H8. Active citizen participation contributes to more informed decision-making processes. By providing input, citizens offer valuable insights and local knowledge that can lead to smarter, more contextually relevant policies and initiatives (Alamoudi, Abidoye, and Lam Citation2022; Castelnovo, Misuraca, and Savoldelli Citation2015). When citizens participate in governance, it holds public officials and institutions accountable. Elected representatives are more likely to respond to the needs and concerns of engaged citizens, ensuring that government actions align with the best interests of the community. Citizen participation empowers individuals and communities to be proactive in addressing their own needs. When residents are involved in decision-making, they have a sense of ownership over the outcomes and are more likely to take responsibility for the well-being of their communities. Smart living often involves advanced urban planning and design. Therefore, citizen participation ensures that these plans align with the preferences and needs of the community, leading to better-designed, sustainable, and livable urban environments (de Melo Cartaxo Citation2021). Engaged citizens can influence resource allocation decisions, directing funds and resources toward projects that promote smart living. This can lead to investments in smart infrastructure, sustainable practices, and community services. Citizen participation provides ongoing feedback on policies and initiatives, allowing for continuous improvement and adaptation. This iterative approach ensures that policies remain relevant and effective in addressing evolving smart living needs.
Hypothesis H9 of the impacts of citizen participation on smart building initiatives is significant in this study. Active citizen involvement in smart building projects ensures that designs prioritize the needs and preferences of the building’s occupants (Narayan Citation2002; Paulussen et al. Citation2007). This can lead to more user-friendly features, layouts, and amenities that enhance comfort and convenience. Engaged citizens can influence the adoption of energy-efficient practices and technologies within smart buildings. This includes the selection of energy-saving appliances, lighting systems, and HVAC solutions, ultimately reducing energy consumption and operational costs. Citizens concerned about environmental impact can advocate for sustainable building materials, green construction practices, and renewable energy sources. These initiatives align with smart building principles and contribute to environmental sustainability (Agboola, Zakka, and Zango Citation2015; Kim and Lee Citation2019). Citizen participation promotes the inclusion of universal design features in smart buildings, ensuring accessibility for individuals with disabilities and diverse needs. These inclusive designs benefit all occupants and promote social equity. Engaged citizens can provide feedback on the functionality and performance of smart building systems. This feedback can inform ongoing improvements and refinements in building operations, maintenance, and user experience. Citizen involvement can lead to the incorporation of enhanced safety and security features, such as advanced fire prevention systems, emergency response plans, and robust access control, to ensure the well-being of building occupants (Ghaffarianhoseini et al. Citation2016; Narayan Citation2002). Smart buildings often collect and process personal data. The collection and analysis of vast amounts of data in smart cities raise privacy concerns. Citizens may feel uncomfortable with the constant monitoring and data sharing, potentially leading to resistance against smart city initiatives. Notwithstanding, citizen participation can help set policies and guidelines to protect privacy and security, ensuring that data is handled responsibly and transparently. Engaged citizens can advocate for resilience planning within smart buildings, ensuring they are equipped to handle unexpected events, such as power outages, extreme weather, or natural disasters, and continue to function effectively. Citizen’s participation in smart building initiatives can raise public awareness about the benefits and opportunities of smart technology adoption in daily life (Ghaffarianhoseini et al. Citation2016; Gonzalez et al. Citation2020; Paulussen et al. Citation2007). This awareness can encourage responsible and efficient use of building features. Citizens can advocate for policies that support the development and maintenance of smart buildings. This can include incentives for energy-efficient construction, subsidies for green technologies, and regulations that ensure safety and sustainability.
7. Conclusion and recommendation
In light of the rapid urbanization sweeping across Saudi Arabia Cities and the pressing challenges associated with urban sustainability, the paradigm shift towards the development of smart cities has emerged as a strategic imperative. As we conclude our exploration within the unique context of Najran City, Saudi Arabia, it becomes evident that Najran stands as a remarkable exemplar of this transformative journey, poised at the intersection of urban evolution and technological innovation. In Saudi Arabia, the embrace of smart cities presents substantial prospects for bolstering economic growth, promoting sustainability, and elevating the overall quality of life. The government’s dedication to digital transformation, coupled with substantial investments in smart city endeavors, is poised to establish a robust groundwork for the creation of technologically advanced and sustainable urban landscapes. Najran City, with its diverse districts and dynamic urban landscape, has taken bold strides toward harnessing advanced technologies and adopting participatory governance models. These endeavors have been undertaken with the overarching goal of sculpting a resilient, environmentally conscious, and sustainable urban environment. Our research, employing a rigorous mixed-methods approach, has delved into the intricate web of factors underpinning the emergence of smart cities in Saudi Arabia and the direct consequences of these initiatives on the critical issue of environmental sustainability.
One of the central tenets that have emerged from our study is the pivotal role played by smart technologies in achieving sustainable resource management and elevating urban livability in Saudi Arabia and indeed, nations globally. These significant steps ensure the creation of a greener, more resilient, and ultimately, more livable urban future. Amid a rapidly changing urban landscape, the integration of smart solutions has proven instrumental in enhancing the quality of life for residents. The intelligent deployment of digital infrastructure, alongside innovative governance models, has acted as a linchpin in steering Cities towards achieving their environmental sustainability goals. Our findings resoundingly underscore the significance of specific smart city attributes. In the attainment of environmental sustainability objectives which shed light on the transformative power of smart urbanization. It is clear that the synergy between these components not only fosters sustainable resource management but also contributes to the overall well-being and prosperity of the residents.
The implications of our research are not confined to the boundaries of Najran or Saudi Arabia alone. They reverberate as a resonant reference point for regions across the globe grappling with parallel challenges of urbanization and environmental sustainability. The journey embarked upon by Najran serves as an invaluable case study, offering insights, best practices, and inspiration to policymakers, urban planners, and stakeholders worldwide. As nations collectively navigate the intricate terrain of urban development, Najran’s experiences and successes provide a guiding light. In closing, our research has illuminated the profound impact of smart cities on the pursuit of environmental sustainability. It underscores the transformative potential of advanced technologies, participatory governance, and holistic urban planning.
Nonetheless, realizing the full potential of smart cities necessitates overcoming various difficulties. These include developing an extensive legislative and regulatory structure, securing adequate funding, increasing capacity and competencies, encouraging citizen engagement, encouraging collaboration, facilitating reliable governance of data and confidentiality, achieving adaptability and seamless integration, focusing on resilience and sustainable development, promoting digital access and fairness, supporting continuous creativity, and assessing the financial and social effects. In accordance with the outcomes of this study, a major potential direction for future research is identified. In this vein, it would be valuable to explore the potential trade-offs and unintended consequences of smart cities and building development. For example, the rapid adoption of new technologies and automation could lead to job losses and other socio-economic challenges, while also potentially exacerbating existing inequalities. Understanding these trade-offs and their implications for different stakeholders will be critical to ensuring that smart city and building development is both sustainable and equitable. In conclusion, the smart integration of cities and buildings can significantly contribute to achieving the UN Sustainable Development Goals and combatting climate change. However, successful implementation requires collaboration between stakeholders, effective policies, and sufficient financial resources.
CRediT authorship contribution statement
Badr Saad Alotaibi, Rana Elnaklah & Oluwagbemiga Paul Agboola: Conceptualization, Formal analysis, Writing – original and final draft. Mohammed Awad Abuhussain, Yakubu Aminu Dodo & Mustafa Tunay: Data curation, Software & editing. Ammar Maghrabi & Mana Alyami: Data curation; editing & visualization.
Disclosure statement
No potential conflict of interest was reported by the author(s).
Additional information
Funding
Notes on contributors
Badr Saad Alotaibi
Badr Saad Alotaibi, Ph.D., is an Assistant Professor at the Architectural Engineering Department College of Engineering, Najran University, Najran, Saudi Arabia.
Rana Elnaklah
Rana Elnaklah, Ph.D., is an Assistant Professor at the Faculty of Architecture and Design, Al-Ahliyya Amman University, Amman Jordan.
Oluwagbemiga Paul Agboola
Oluwagbemiga Paul Agboola, Ph.D., is an Assistant Professor at the Department of Architecture, Faculty of Engineering and Architecture, Istanbul Gelisim University, Istanbul, Turkey.
Mohammed Awad Abuhussain
Mohammed Awad Abuhussain, Ph.D., is an Assistant Professor at the Architectural Engineering Department College of Engineering, Najran University, Najran, Saudi Arabia.
Mustafa Tunay
Mustafa Tunay, Ph.D., is an Assistant Professor at the Cyprus Science University Computer Engineering, Girne, Northern Cyprus, Mersin 10, Turkey.
Yakubu Aminu Dodo
Yakubu Aminu Dodo, Ph.D., is an Assistant Professor at the Architectural Engineering Department College of Engineering, Najran University, Najran, Saudi Arabia.
Ammar Maghrabi
Ammar Maghrabi, Ph.D., is an Assistant Professor at the Urban and Engineering Research Department, The Custodian of the Two Holy Mosques Institute for Hajj and Umrah Research, Umm Al-Qura University, Saudi Arabia.
Mana Alyami
Mana Alyami, Ph.D., is an Assistant Professor at the Department of Civil Engineering, College of Engineering, Najran University, Najran, Saudi Arabia.
References
- Abdullahi, S., B. Pradhan, and H. Mojaddadi. 2018. “City Compactness: Assessing the Influence of the Growth of Residential Land Use.” Journal of Urban Technology 25 (1): 21–46. https://doi.org/10.1080/10630732.2017.1390299.
- Acemoglu, D., and P. Restrepo. 2018. “Artificial Intelligence, Automation, and Work.” InThe Economics of Artificial Intelligence: An Agenda, 197–236. University of Chicago Press. http://www.nber.org/books/agra-1.
- Agboola, O. P., B. S. Alotaibi, Y. A. Dodo, M. A. Abuhussain, and M. Abuhussain. 2023. “Built Environment Transformation in Nigeria: The Effects of a Regenerative Framework.” Journal of Asian Architecture and Building Engineering 1–24. https://doi.org/10.1080/13467581.2023.2238045.
- Agboola, O. P., F. M. Bashir, Y. A. Dodo, M. A. S. Mohamed, and I. S. R. Alsadun. 2023. “The Influence of Information and Communication Technology (ICT) on stakeholders’ Involvement and Smart Urban Sustainability.” Environmental Advances 13:100431. https://doi.org/10.1016/j.envadv.2023.100431.
- Agboola, O. P., S. Moveh, K. Yahya, H. Attar, and A. Amer. 2022. “The Role of Smart Environment Initiatives on Environmental Degradation: Consolidating the Resilient Built Landscape.” In 2022 International Engineering Conference on Electrical, Energy, and Artificial Intelligence (EICEEAI), Zarqa, Jordan, November, 1–5. IEEE. https://doi.org/10.1109/EICEEAI56378.2022.10050481.
- Agboola, O. P., and M. Tunay. 2023. “Urban Resilience in the Digital Age: The Influence of Information-Communication Technology for Sustainability.” Journal of Cleaner Production 428. https://doi.org/10.1016/j.jclepro.2023.139304.
- Agboola, O. P., S. D. Zakka, and M. S. Zango. 2015. “Towards Sustainability of Open Space Planning and Management in Nigeria: The Role of Science and Technology.” Journal Teknologi 27 (14): 51–56. https://doi.org/10.11113/jt.v77.6446.
- Ahad, M. A., S. Paiva, G. Tripathi, and N. Feroz. 2020. “Enabling Technologies and Sustainable Smart Cities.” Sustainable Cities and Society 61:102301. https://doi.org/10.1016/j.scs.2020.102301.
- Ahvenniemi, H., A. Huovila, I. Pinto-Seppä, and M. Airaksinen. 2017. “What Are the Differences Between Sustainable and Smart Cities?” Cities 60:234–245. https://doi.org/10.1016/j.cities.2016.09.009.
- Ajibade, T. B., E. T. Ajibade, and W. O. Oloyede. 2018. “Economic Appraisal of Formal and Informal Credit Sources Usage in Livestock Financing Among Women in South-Western Nigeria.” PAT 14 (1) : 106–112. www.patnsukjournal.net/currentissue.
- Akinola, A. O., A. P. Opoko, E. O. Ibem, H. I. Okagbue, and A. O. Afolabi. 2020. “Climate Change Adaptation and Mitigation Strategies in Lagos, Nigeria: Built Environment professionals’ Perspective.” International Journal of Engineering and Advanced Technology 9 (3): 1273–1282. https://doi.org/10.35940/ijeat.B2630.029320.
- Alaidroos, A., and M. Krarti. 2015. “Optimal Design of Residential Building Envelope Systems in the Kingdom of Saudi Arabia.” Energy and Buildings 86:104–117. https://doi.org/10.1016/j.enbuild.2014.09.083.
- Alamoudi, A. K., R. B. Abidoye, and T. Y. Lam. 2022. “Critical Review of Citizens’ Participation in Achieving Smart Sustainable Cities: The Case of Saudi Arabia.” In International Summit Smart City 360◦, edited by S. Paiva, X. Li, S. I. Lopes, N. Gupta, D. B. Rawat, A. Patel, and H. R. Karimi, 434–454. Vol. 442. Cham, Switzerland: Springer.
- Alamoudi, A. K., B. A. Rotimi, and Y. M. L. Terence. 2023. “The Impact of Citizens’ Participation Level on Smart Sustainable Cities Outcomes: Evidence from Saudi Arabia.” Buildings 13 (2): 343. https://doi.org/10.3390/buildings13020343.
- Alanazi, F. 2023. “Development of Smart Mobility Infrastructure in Saudi Arabia: A Benchmarking Approach.” Sustainability 15 (4): 3158. https://doi.org/10.3390/su15043158.
- Albino, V., U. Berardi, and R. M. Dangelico. 2015. “Smart Cities: Definitions, Dimensions, Performance, and Initiatives.” Journal of Urban Technology 22 (1): 3–21. https://doi.org/10.1080/10630732.2014.942092.
- Aldakheel, J., M. Bahrar, and M. El Mankibi. 2023. “Indoor Environmental Quality Evaluation of Smart/Artificial Intelligence Techniques in Buildings–A Review.” E3S Web of Conferences, 396:01101. EDP Sciences. https://doi.org/10.1051/e3sconf/202339601101.
- Aldegheishem, A. 2023. “Assessing the Progress of Smart Cities in Saudi Arabia.” Smart Cities 6 (4): 1958–1972. https://doi.org/10.3390/smartcities6040091.
- Al Jaafreh, M. B., and M. A. Allouzi. 2023. “Smart Cities Adoption in Saudi Arabia: A Comprehensive Review and Future Drivers.” British Journal of Multidisciplinary and Advanced Studies 4 (5): 20–39. https://doi.org/10.37745/bjmas.2022.0300.
- Aljoufie, M., and A. Tiwari. 2022. “Citizen Sensors for Smart City Planning and Traffic Management: Crowdsourcing Geospatial Data Through Smartphones in Jeddah, Saudi Arabia.” Geo Journal 87 (4): 3149–3168. https://doi.org/10.1007/s10708-021-10423-4.
- Almulhim, A. I., S. E. Bibri, A. Sharifi, S. Ahmad, and K. M. Almatar. 2022. “Emerging Trends and Knowledge Structures of Urbanization and Environmental Sustainability: A Regional Perspective.” Sustainability 14 (20): 13218. https://doi.org/10.3390/su142013195.
- Almulhim, A. I., and P. B. Cobbinah. 2023. “Urbanization-Environment Conundrum: An Invitation to Sustainable Development in Saudi Arabian Cities.” International Journal of Sustainable Development & World Ecology 30 (4): 359–373. https://doi.org/10.1080/13504509.2022.2152199.
- AlQuhtani, S. 2023. “Factors Affecting Active Commuting to School in Sprawled Cities: The Case of Najran City, Saudi Arabia.” Sustainability 15 (12): 9562. https://doi.org/10.3390/su15129562.
- Alswedani, S., I. Katib, E. Abozinadah, and R. Mehmood. 2022. “Discovering Urban Governance Parameters for Online Learning in Saudi Arabia During COVID-19 Using Topic Modeling of Twitter Data.” Frontiers in Sustainable Cities 4:751681. https://doi.org/10.3389/frsc.2022.751681.
- Anthopoulos, L., M. Janssen, and V. Weerakkody. 2015. “Comparing Smart Cities with Different Modeling Approaches.” In Proceedings of the 24th International Conference on World Wide Web, 525–528. https://doi.org/10.1145/2740908.2743920.
- Anttiroiko, A. V., P. Valkama, and S. J. Bailey. 2013. “Smart Cities in the New Service Economy: Building Platforms for Smart Services.” AI & Society 29 (3): 1–12. https://doi.org/10.1007/s00146-013-0464-0.
- Ardito, L., A. Ferraris, M. A. Petruzzelli, S. Bresciani, and M. Del Giudice. 2019. “The Role of Universities in the Knowledge Management of Smart City Projects.” Technological Forecasting and Social Change 142:312–321. https://doi.org/10.1016/j.techfore.2018.07.030.
- Asmyatullin, R. R., K. V. Tyrkba, and E. I. Ruzina. 2020. “Smart Cities in GCC: Comparative Study of Economic Dimension.” In IOP Conference Series: Earth and Environmental Science, 459:062045. https://doi.org/10.1088/1755-1315/459/6/062045.
- Bamwesigye, D., and P. Hlavackova. 2019. “Analysis of Sustainable Transport for Smart Cities.” Sustainability 11 (8): 2140. https://doi.org/10.3390/su11072140.
- Bassey, S. 2019. “‘Anthropoholism’ as an Authentic Tool for Environmental Management.” International Journal of Environmental Pollution and Environmental Modelling 2 (3): 160–168.
- Bentler, P. M., and D. G. Bonett. 1980. “Significance Tests and Goodness of Fit in the Analysis of Covariance Structures.” Psychological Bulletin 88 (3): 588. https://doi.org/10.1037/0033-2909.88.3.588.
- Bibri, S. E. 2021. “Data-Driven Smart Sustainable Cities of the Future: Urban Computing and Intelligence for Strategic, Short-Term, and Joined-Up Planning.” Computational Urban Science 1 (1): 1–29. https://doi.org/10.1007/s43762-021-00001-2.
- Bibri, S. E., and J. Krogstie. 2020. “Environmentally Data-Driven Smart Sustainable Cities: Applied Innovative Solutions for Energy Efficiency, Pollution Reduction, and Urban Metabolism.” Energy Informatics 3 (1): 1–59. https://doi.org/10.1186/s42162-020-00130-8.
- Bifulco, F., C. C. Amitrano, and M. Tregua. 2014. “Driving Amortization Through Intelligent Transport.” Chinese Business Review 13 (4): 243–259.
- Bock, G. W., A. Kankanhalli, and S. Sharma. 2006. “Are Norms Enough? The Role of Collaborative Norms in Promoting Organizational Knowledge Seeking.” European Journal of Information Systems 15 (4): 357e367. https://doi.org/10.1057/palgrave.ejis.3000630.
- Borsekova, K., A. Vanova, and K. Vitalisova. 2016. “The Power of Communities in Smart Urban Development.” Procedia-Social and Behavioral Sciences 223:51–57. https://doi.org/10.1016/j.sbspro.2016.05.289.
- Boudjelida, A., S. Mellouli, and J. Lee. 2016. “Electronic Citizens Participation: Systematic Review.” In Proceedings of the 9th international conference on theory and practice of electronic governance, March, 31–39. https://doi.org/10.1145/2910019.2910097.
- Cañavera-Herrera, J. S., J. Tang, T. Nochta, and J. M. Schooling. 2022. “On the Relation Between ‘Resilience’ and ‘Smartness’: A Critical Review.” International Journal of Disaster Risk Reduction 75:102970. https://doi.org/10.1016/j.ijdrr.2022.102970.
- Caragliu, A., C. Del Bo, K. Kourtit, and P. Nijkamp. 2011. “Comparative Performance Assessment of Smart Cities Around the North Sea Basin.” Network Industries Quarterly 13 (3): 15–17.
- Cardullo, V. M., N. S. Wilson, and V. I. Zygouris-Coe. 2018. “Enhanced Student Engagement Through Active Learning and Emerging Technologies.” Student Engagement and Participation: Concepts, Methodologies, Tools, and Applications 399–417. https://doi.org/10.4018/978-1-5225-2584-4.ch019.
- Carli, R., M. Dotoli, R. Pellegrino, and L. Ranieri. 2013. “Measuring and Managing the Smartness of Cities: A Framework for Classifying Performance Indicators.” In Proceedings of the 2013 IEEE International Conference on Systems, Man, and Cybernetics, Manchester, UK, 1288–1293. https://doi.org/10.1109/SMC.2013.223.
- Castelnovo, W., G. Misuraca, and A. Savoldelli. 2015. “Smart Cities Governance: The Need for a Holistic Approach to Assessing Urban Participatory Policy Making.” Social Science Computer Review 34 (6): 724–739. https://doi.org/10.1177/0894439315611103.
- Cavana, R., B. L. Delahaye, and U. Sekeran. 2001. Applied Business Research: Qualitative and Quantitative Methods, 83–87. Singapore: Markono Print Media Ltd.
- Chatti, W. 2021. “Moving Towards Environmental Sustainability: Information and Communication Technology (ICT), Freight Transport, and CO2 Emissions.” Heliyon 7 (10): 1–8. https://doi.org/10.1016/j.heliyon.2021.e08190.
- Chen, S.-Y., and S.-F. Chang. 2009. “A Review of Smart Living Space Development in a Cloud Computing Network Environment.” Computer-Aided Design and Applications 6 (4): 513–527. https://doi.org/10.3722/cadaps.2009.513-527.
- Chetty, R., J. N. Friedman, N. Hendren, M. Stepner, and The Opportunity Insights Team. 2020. “How Did COVID-19 and Stabilization Policies Affect Spending and Employment? A New Real-Time Economic Tracker Based on Private Sector Data.” 1689–1699. Vol. 91. Cambridge, MA: National Bureau of Economic Research. https://doi.org/10.3386/w27431.
- Chin, W. W. 1998. “The Partial Least Squares Approach to Structural Equation Modeling.” Modern Methods for Business Research 295 (2): 295e336.
- Chowdhury, S., D. Haftor, and N. Pashkevich. 2018. “Smart Product-Service Systems (Smart PSS) in Industrial Firms: A Literature Review.” Procedia Cirp 73:26–31. https://doi.org/10.1016/j.procir.2018.03.333.
- Creswell, J. W. 2012. Educational Research Planning, Conducting, and Evaluating Quantitative and Qualitative Research. 4th ed. Boston: Pearson Education Inc.
- de Melo Cartaxo, T. 2021. “Digitalization and Smartening Sustainable City Development: An Investigation from the High North European Cities.”
- Dirks, S., and M. Keeling. 2009. A Vision of Smarter Cities: How Cities Can Lead the Way into a Prosperous and Sustainable Future. 8. IBM Institute for Business Value. https://www.foresightfordevelopment.org/sobipro/55/1042-a-vision-of-smarter-cities-how-cities-can-lead-the-way-into-a-prosperous-and-sustainable-future.
- Doheim, R. M., A. A. Farag, and S. Badawi. 2019. “Smart City Vision and Practices Across the Kingdom of Saudi Arabia—A Review.” Smart Cities: Issues, Challenges, and Mapping of Political, Social, Economic, Risks, Threats 309–332.
- Edge, S., K. Boluk, M. Groulx, and M. Quick. 2020. “Exploring Diverse Lived Experiences in the Smart City Through Creative Analytic Practice.” Cities 96:102478. https://doi.org/10.1016/j.cities.2019.102478.
- Feldman, M. P., and D. B. Audretsch. 1999. “Innovation in Cities: Science-Based Diversity, Specialization, and Localized Competition.” European Economic Review 43 (2): 409–429. https://doi.org/10.1016/S0014-2921(98)00047-6.
- Fetters, M. D., L. A. Curry, and J. W. Creswell. 2013. “Achieving Integration in Mixed Methods Designs—Principles and Practices.” Health Services Research 48 (Pt 2): 2134–2156. https://doi.org/10.1111/1475-6773.12117.
- Fornell, C., and D. F. Larcker. 1981. “Evaluating Structural Equation Models with Unobservable Variables and Measurement Error.” Journal of Marketing Research 18 (1): 39e50. https://doi.org/10.1177/002224378101800313.
- Frost & Sullivan. 2023. “Smart Cities to Create Business Opportunities Worth $2.46 Trillion by 2025.” https://www.frost.com/news/press-releases/automotive-transportation/smart-cities-to-create-business-opportunities-worth-2-46-trillion-by-2025-says-frost-sullivan/.
- Ghaffarianhoseini, U. Berardi, A. Ghaffarianhoseini, H. AlWaer, S. Chang, E. Halawa, A. Ghaffarianhoseini, and D. Clements-Croome. 2016. “What Is an Intelligent Building? Analysis of Recent Interpretations from an International Perspective.” Architectural Science Review 59 (5): 338–357. https://doi.org/10.1080/00038628.2015.1079164.
- Giffinger, R., C. Fertner, H. Kramar, R. Kalasek, N. Pichler-Milanovic, and E. Meijers. 2007. “Smart Cities Ranking of European Medium-Sized Cities. Retrieved from source: Accessed August 25, 2023. www.smart-cities.eu/download/smart_cities_final_report.pdf.
- Gil-Garcia, J. R., T. A. Pardo, and T. Nam. 2015. “What Makes a City Smart? Identifying Core Components and Proposing an Integrative and Comprehensive Conceptualization.” Information Polity 20 (1): 61–87. https://doi.org/10.3233/IP-150354.
- Gliem, J. A., and R. R. Gliem. 2003. “Calculating, Interpreting, and Reporting Cronbach’s Alpha Reliability Coefficient for Likert-Type Scales.” In Midwest Research-to-Practice Conference in Adult, Continuing, and Community Education, Columbus, 82–88. Available online: https://hdl.handle.net/1805/344.
- Gonzalez, E. L., C. K. Chinelli, A. L. Azevedo Guedes, E. G. Vazquez, A. W. Hammad, A. N. Haddad, and C. A. Pereira Soares. 2020. “Smart, and Sustainable Cities: The Main Guidelines of City Statute for Increasing the Intelligence of Brazilian Cities.” Sustainability 12 (3): 1025. https://doi.org/10.3390/su12031025.
- Grab, B., and C. Ilie. 2019. “Innovation Management in the Context of Smart Cities Digital Transformation.” Proceedings of the 37th International Scientific Conference on Economic and Social Development—“Socio Economic Problems of Sustainable Development, Baku, Azerbaijan, 14–15; 165–174.
- Grossi, G., and D. Pianezzi. 2017. “Smart Cities: Utopia or Neoliberal Ideology?” Cities 69:79–85. https://doi.org/10.1016/j.cities.2017.07.012.
- Hair, J. F., M. C. Howard, and C. Nitzl. 2020. “Assessing Measurement Model Quality in PLS-SEM 14, Using Confirmatory Composite Analysis.” Journal of Business Research 109:101–15. 110. https://doi.org/10.1016/j.jbusres.2019.11.069.
- Hair, J. F., J. J. Risher, M. Sarstedt, and C. M. Ringle. 2019. “When to Use and How to Report the 18 Results of PLS-SEM.” European Business Review 31 (1): 2–24. https://doi.org/10.1108/EBR-11-192018-0203.
- Henderson, J. V. 2005. “Urbanization and Growth.”In Handbook of Economic Growth, edited byP. Aghion and S. N. Durlauf, 1543–1591. Vol. 1. Elsevier. https://doi.org/10.1016/S1574-0684(05)01024-5.
- Henseler, J., G. Hubona, and P. A. Ray. 2016. “Using PLS Path Modeling in New Technology Research: Updated Guidelines.” Industrial Management & Data Systems 166 (1): 2–20. https://doi.org/10.1108/IMDS-09-2015-0382.
- Hollands, R. 2008. “Will the Real Smart Culture, Theory, Policy, Action Please Stand Up? Creative, Progressive or Just Entrepreneurial? City: Analysis of Urban Trends.” City 12 (3): 303–320. https://doi.org/10.1080/13604810802479126.
- Holl, A., and I. Mariotti. 2018. “The Geography of Logistics Firm Location: The Role of Accessibility.” Networks and Spatial Economics 18 (2): 337–361. https://doi.org/10.1007/s11067-017-9347-0.
- Horgan, D., and B. Dimitrijević. 2019. “Frameworks for citizen’s Participation in Planning: From Conversational to Smart Tools.” Sustainable Cities and Society 48:101550. https://doi.org/10.1016/j.scs.2019.101550.
- Hsu, M. H., and C. M. Chang. 2014. “Examining Interpersonal Trust As a Facilitator and Uncertainty As an Inhibitor of Intra-Organizational Knowledge Sharing.” Information Systems Journal 24 (2): 119e142. https://doi.org/10.1111/isj.12000.
- Hu, L.-T.-T., and P. M. Bentler. 1999. “Cutoff Criteria for Fit Indexes in Covariance Structure Analysis: 33- 34, Conventional Criteria versus New Alternatives.” Multidisciplinary Journal 35. https://doi.org/10.1080/10705519909540118.
- Huck, A., and J. Monstadt. 2019. “Urban and Infrastructure Resilience: Diverging Concepts and the Need for Cross-Boundary Learning.” Environmental Science & Policy 100:211–220. https://doi.org/10.1016/j.envsci.2019.05.008.
- Hulland, J. 1999. “Use of Partial Least Squares (PLS) in Strategic Management Research: A Review of Four Recent Studies.” Strategic Management Journal 20 (2): 195–204. https://doi.org/10.1002/(SICI)1097-0266(199902)20:2<195:AID-SMJ13>3.0.CO;2-7.
- Ifere, N. B., and E. D. Abim. 2019. “Development and Environmental Sustainability in Nigeria: An African Perspective.” An Interdisciplinary Journal of Human Theory and Praxis 2 (1). DOI: https://doi.org/10.5281/zenodo.3534015.
- Ismagilova, E., L. Hughes, Y. K. Dwivedi, and K. R. Raman. 2019. “Smart Cities: Advances in Research—An Information Systems Perspective.” International Journal of Information Management 47:88–100. https://doi.org/10.1016/j.ijinfomgt.2019.01.004.
- Ismagilova, E., E. Slade, N. P. Rana, and Y. K. Dwivedi. 2020. “The Effect of Characteristics of Source Credibility on Consumer Behaviour: A Meta-Analysis.” Journal of Retailing & Consumer Services 53:101736.
- Jay, J. 2013. “Navigating Paradox as a Mechanism of Change and Innovation in Hybrid Organizations.” Academy of Management Journal 56 (1): 137–159. https://doi.org/10.5465/amj.2010.0772.
- Jia, M., A. Komeily, Y. Wang, and R. S. Srinivasan. 2019. “Adopting Internet of Things for the Development of Smart Buildings: A Review of Enabling Technologies and Applications.” Automation in Construction 101:111–126. https://doi.org/10.1016/j.autcon.2019.01.023.
- Jiang, H., S. Geertman, and P. Witte. 2020. “A Socio-Technical Framework for Smart Urban Governance: Urban Technological Innovation and Urban Governance in the Realm of Smart Cities.” International Journal of E-Planning Research 9 (1): 1–19. https://doi.org/10.4018/IJEPR.2020010101.
- Johnson, A. G., and I. Samakovlis. 2019. “A Bibliometric Analysis of Knowledge Development in Smart Tourism Research.” Journal of Hospitality & Tourism Technology 10 (4): 600–623. https://doi.org/10.1108/JHTT-07-2018-0065.
- Jones, E. 2018. “The early development of female sexuality.” In Female Sexuality, 133–145. Routledge. https://www.taylorfrancis.com/chapters/edit/10.4324/9780429474675-10/early-development-female-sexuality-ernest-jones.
- Joshi, S., S. Saxena, T. Godbole. 2016. “Developing Smart Cities: An Integrated Framework.” Procedia Computer Science 93:902–909. https://doi.org/10.1016/j.procs.2016.07.258.
- Kaluarachchi, Y. 2021. “Potential Advantages in Combining Smart and Green Infrastructure Over Silo Approaches for Future Cities.” Frontiers of Engineering Management 8 (1): 98–108. https://doi.org/10.1007/s42524-020-0136-y.
- Kaluarachchi, Y. 2022. “Implementing Data-Driven Smart City Applications for Future Cities.” Smart Cities 5 (2): 455–474. Accessed July 13th, 2022. https://doi.org/10.3390/smartcities5020025.
- Keshavarzi, G., Y. Yildirim, and M. Arefi. 2021. “Does Scale Matter? An Overview of the “Smart cities” Literature.” Sustainable Cities and Society 74:103151. https://doi.org/10.1016/j.scs.2021.103151.
- Kim, S., and J. Lee. 2019. “Citizen Participation, Process, and Transparency in Local Government: An Exploratory Study.” Policy Studies Journal 47 (4): 1026–1047. https://doi.org/10.1111/psj.12236.
- Kitchin, R. 2014. “The Real-Time City? Big Data and Smart Urbanism.” Geo Journal 79 (1): 1–14. https://doi.org/10.1007/s10708-013-9516-8.
- Kitchin, R., and G. McArdle. 2016. “What Makes Big Data, Big Data? Exploring the Ontological Characteristics of 26 Datasets.” Big Data & Society 3 (1): 2053951716631130. https://doi.org/10.1177/2053951716631130.
- Komninos, N. 2015. The Age of Intelligent Cities: Smart Environments and Innovation-For-All Strategies, 1–298. 1st ed. London, UK: Routledge.
- Krugman, P. 1991. “The Move Toward Free Trade Zones.” Economic Review 76 (6): 5.
- Latif, G., J. M. Alghazo, R. Maheswar, P. Jayarajan, and A. Sampathkumar. 2020. “Impact of IoT-Based Smart Cities on Human Daily Life.” In Integration of WSN and IoT for Smart Cities, 103–14.
- Latif, S. A., F. B. X. Wen, C. Iwendi, F. W. Li-Li, S. M. Mohsin, Z. Han, and S. S. Band. 2022. “AI-Empowered, Blockchain and SDN Integrated Security Architecture for IoT Network of Cyber Physical Systems.” Computer Communications 181:274–283. https://doi.org/10.1016/j.comcom.2021.09.029.
- Le Duc, C. 2022. “The Invested City.” In The Climate City, 363–375. New York, NY, USA: Wiley. https://onlinelibrary.wiley.com/doi/abs/10.1002/9781119746294.ch22.
- Lee, J. H., M. Hancock, and M. C. Gand Hu. 2013. “Towards an Effective Framework for Building Smart Cities: Lessons from Seoul and San Francisco.” Technological Forecasting & Social Change 80 (2): 286–306. https://doi.org/10.1016/j.techfore.2012.09.020.
- Mahapatra, C., A. K. Moharana, and V. C. M. Leung. 2017. “Energy Management in Smart Cities Based on Internet of Things: Peak Demand Reduction and Energy Savings.” Sensors 17 (12): 2812. https://doi.org/10.3390/s17122812.
- Maldonado Silveira Alonso Munhoz, P. A., F. da Costa Dias, C. Kowal Chinelli, A. L. Azevedo Guedes, J. A. Neves dos Santos, W. da Silveira e Silva, and C. A. Pereira Soares. 2020. “Smart Mobility: The Main Drivers for Increasing the Intelligence of Urban Mobility.” Sustainability 12 (24): 10675. https://doi.org/10.3390/su122410675.
- Martina, C., J. Evansa, A. Karvonenb, P. Krassimira, D. Yangd, and T. Linjordete. 2019. “Smart-Sustainability: A New Urban Fix?” Sustainability: Science, Practice, & Policy 45:640–648. https://doi.org/10.1016/j.scs.2018.11.028.
- Martin, P., and C. A. Rogers. 1995. “Industrial Location and Public Infrastructure.” Journal of International Economics 39 (3–4): 335–351. https://doi.org/10.1016/0022-1996(95)01376-6.
- Mawkhlieng, D., and S. Debbarma. 2018. “Conditions of Urban Slums and Its Quality of Life in India: A Regional Analysis.” Journal of Regional Development and Planning 7 (1): 71–84.
- McNeill, M., S. Noyek, E. Engeda, and N. Fayed. 2021. “Assessing the Engagement of Children and Families in Selecting Patient-Reported Outcomes (PROs) and Developing Their Measures: A Systematic Review.” Quality of Life Research 30 (4): 983–995. https://doi.org/10.1007/s11136-020-02690-4.
- Miller, D. 2024. “Nonprofits Should Adopt a User-Centric Change Model to Scale Corporate Environmental Action Faster.” Journal of Nonprofit Innovation 4 (1): 5.
- Moglia, M., A. Podkalicka, and J. McGregor. 2018. “An Agent-Based Model of Residential Energy Efficiency Adoption.” Journal of Artificial Societies and Social Simulation 21 (3). https://doi.org/10.18564/jasss.3729.
- Mohamed, M., M. ALSurf, and S. AL-Kesmi. 2022. “Green Sustainable Urban Systems: The Case of Jeddah.” In Proceedings of the International Research & Innovation Forum, Athens, Greece, April 27–29.
- Moraes, C. R. D., and P. R. Cunha. 2022. “Enterprise Servitization: Practical Guidelines for Culture Transformation Management.” Sustainability 15 (1): 705. https://doi.org/10.3390/su15010705.
- Najran Municipality. 2019. Preparing the Local and Detailed Plan for Najran City. Najran, Saudi Arabia: Najran Municipality.
- Nam, T., and T. A. Pardo. 2011. “Smart City As Urban Innovation: Focusing on Management, Policy, and Context.” In Proceedings of the 5th international conference on theory and practice of electronic governance, September, 185–194. https://doi.org/10.1145/2072069.2072100.
- Narayan, D. 2002. “Bonds and Bridges: Social Capital and Poverty.” In Social Capital and Economic Development: Well-Being in Developing Countries, 58–81. Northampton, MA: Edward Elgar. https://www.elgaronline.com/edcollbook/9781840646993.xml#page=74.
- Nasiri, F., F. Mafakheri, D. Adebanjo, and F. Haghighat. 2016. “Modeling and Analysis of Renewable Heat Integration into Non-Domestic Buildings-The Case of Biomass Boilers: A Whole Life Asset-Supply Chain Management Approach.” Biomass and Bioenergy 95:244–256. https://doi.org/10.1016/j.biombioe.2016.10.018.
- Nunnally, J. 1978. Psychometric Theory. Vol. 2. New York: McGraw-Hill.
- Ochoa, J. J., Y. Tan, Q. K. Qian, L. Shen, and E. L. Moreno. 2018. “Learning from Best Practices in Sustainable Urbanization.” Habitat International 78:83–95. https://doi.org/10.1016/j.habitatint.2018.05.013.
- Offiong, O. J., and R. Dibie. 2014. “Environmental Policy and Issues in Nigeria.” In Comparative Perspectives on Environmental Policies and Issues, 268–300. Routledge. https://www.taylorfrancis.com/chapters/edit/10.4324/9780203067819-12/environmental-policy-issues-nigeria-offiong-offiong-robert-dibie.
- Oke, A. E., D. O. Aghimien, O. I. Akinradewo, and C. O. Aigbavboa. 2020. “Improving Resilience of Cities Through Smart City Drivers.” Construction Economics and Building 20 (2): 45–64. https://doi.org/10.5130/AJCEB.v20i2.6647.
- Olsthoorn, D., F. Haghighat, and P. A. Mirzaei. 2016. “Integration of Storage and Renewable Energy into District Heating Systems: A Review of Modeling and Optimization.” Solar Energy 136:49–64. https://doi.org/10.1016/j.solener.2016.06.054.
- Paroutis, S., M. Bennett, and L. Heracleous. 2013. “A Strategic View on Smart City Technology: The Case of IBM Smarter Cities During a Recession.” Technological Forecasting and Social Change 89:262–272. https://doi.org/10.1016/j.techfore.2013.08.041.
- Paulussen, S., A. Heinonen, D. Domingo, and T. Quandt. 2007. “Doing it Together: Citizen Participation in the Professional News-Making Process.” OBSERVATORIO 1 (3): 131–154. https://doi.org/10.15847/obsOBS132007148.
- Pellicer, S., G. Santa, A. L. Bleda, R. Maestre, A. J. Jara, and A. G. Skarmeta. 2013. “A Global Perspective of Smart Cities: A Survey.” In 2013 Seventh International Conference on Innovative Mobile and Internet Services in Ubiquitous Computing, Taichung, Taiwan, July, 439–444. IEEE. https://doi.org/10.1109/IMIS.2013.79.
- Pelling, M., and D. Manuel-Navarrete. 2011. “From Resilience to Transformation: The Adaptive Cycle in Two Mexican Urban Centers.” Ecology and Society 16 (2). https://doi.org/10.5751/ES-04038-160211.
- Pereira, G. V., M. A. Macadar, E. M. Luciano, and M. G. Testa. 2017. “Delivering Public Value Through Open Government Data Initiatives in a Smart City Context.” Information Systems Frontiers 19 (2): 213–229. https://doi.org/10.1007/s10796-016-9673-7.
- Petesch, P., C. Smulovitz, and M. Walton. 2005. “Evaluating Empowerment: A Framework with Cases from Latin America.” In Measuring Empowerment: Cross-Disciplinary Perspectives, edited by D. Narayan-Parker, 39–68. Washington, DC, USA: World Bank Press.
- Petrovic´, N., P. Milic´, and B. Prlincˇevic´. 2022. “Using Open Government Data for Economic Development.” The European Journal of Applied Economics 19 (2): 129–141. https://doi.org/10.5937/EJAE19-39004.
- Pineo, H. 2022. “Towards Healthy Urbanism: Inclusive, Equitable and Sustainable (THRIVES)–An Urban Design and Planning Framework from Theory to Praxis.” Cities & Health 6 (5): 974–992. https://doi.org/10.1080/23748834.2020.1769527.
- Porru, S., F. Edoardo Misso, F. Eros Pani, and C. Repetto. 2020. “Smart Mobility and Public Transport: Opportunities and Challenges in Rural and Urban Areas.” Journal of Traffic and Transportation Engineering (English Edition) 7 (1): 88–97, ISSN 2095-7564, https://doi.org/10.1016/j.jtte.2019.10.002.
- Pratama, A., and S. A. Imawan. 2019. “A Scale for Measuring Perceived Bureaucratic Readiness for Smart Cities in Indonesia.” Public Administration and Policy: An Asia-Pacific Journal 22 (1): 25–39. https://doi.org/10.1108/PAP-01-2019-0001.
- Priano, F., and C. F. Guerra. 2014. “A Framework for Measuring Smart Cities.”In Proceedings of the 15th Annual International Conference on Digital Government Research, Aguascalientes, Mexico, 18–21 June 2014, 44–54.
- Puron-Cid, G. 2015. “Smart Cities, Smart Governments, and Smart Citizens: A Brief Introduction.” International Journal of E-Planning Research 4 (2): 1–4.
- Rahman, A., V. Srikumar, and A. D. Smith. 2018. “Predicting Electricity Consumption for Commercial and Residential Buildings Using Deep Recurrent Neural Networks.” Applied Energy 212:372–385. https://doi.org/10.1016/j.apenergy.2017.12.051.
- Raosoft Sample Size Calculator. 2023). Available online: Accessed May 7, 2023. https://www.raosoft.com/samplesize.html.
- Ringle, C. M. 2005. “SmartPLS 2.0 (M3).” http://www.smartpls.de.
- Ringle, C. M., S. Wende, and J. M. Becker. 2015. “SmartPLS 3.” Bönningstedt: SmartPLS. http://www.smartpls.
- Saeedi, K., A. Visvizi, D. Alahmadi, and A. Barbour. 2023. “Smart Cities and Households’ Recyclable Waste Management: The Case of Jeddah.” Sustainability 15 (8): 6776. https://doi.org/10.3390/su15086776.
- Sánchez-Corcuera, R., A. Nuñez-Marcos, J. Sesma-Solance, A. Bilbao-Jayo, R. Mulero, U. Zulaika, G. Azkune, and A. Almeida. 2019. “Smart Cities Survey: Technologies, Application Domains and Challenges for the Cities of the Future.” International Journal of Distributive Sensing Networks 15 (6): 1550147719853984. https://doi.org/10.1177/1550147719853984.
- Sarstedt, M., and J. H. Cheah. 2019. “Partial Least Squares Structural Equation Modeling Using SmartPLS: A Software Review.” Journal of Marketing Analytics 7 (3): 196–202. https://doi.org/10.1057/s41270-019-00058-3.
- Schaffers, H., N. Komninos, M. Pallot, B. Trousse, M. Nilsson, and A. Oliveira. 2011. Smart Cities and the Future Internet: Towards Cooperation Frameworks for Open Innovation, 431–446. Berlin Heidelberg: Springer.
- Schewenius, M., T. McPhearson, and T. Elmqvist. 2014. “Opportunities for Increasing Resilience and Sustainability of Urbansocial–Ecological Systems: Insights from the URBES and the Cities and Biodiversity Outlook Projects.” AMBIO: A Journal of the Human Environment 43 (4): 434–444. https://doi.org/10.1007/s13280-014-0505-z.
- Schraven, D., S. Joss, and M. De Jong. 2021. “Past, Present, Future: Engagement with Sustainable Urban Development Through 35 City Labels in the Scientific Literature 1990–2019.” Journal of Cleaner Production 292:125924. https://doi.org/10.1016/j.jclepro.2021.125924.
- Sharifi, A., Z. Allam, S. Bibri, E. Khavarian-Garmsir, and A. R. 2024. “Smart Cities and Sustainable Development Goals (SDGs): A Systematic Literature Review of Co-Benefits and Trade-Offs.” Cities 146:104659, ISSN 0264-2751, https://doi.org/10.1016/j.cities.2023.104659.
- Sharifi, A., S. Kawakubo, and A. Milovidova. 2020. “Urban Sustainability Assessment Tools: Toward Integrating Smart City Indicators.” In Urban Systems Design, 345–372. Elsevier. https://doi.org/10.1016/B978-0-12-816055-8.00011-7.
- Sharma, J., M. Tyagi, A. Bhardwaj, and R. S. Walia. 2023. “Factors Assessment for Encumbering the Implementation of Sustainability Based Lean Six Sigma Practices in Food Supply Chain.” Evergreen 10 (1): 379–388. https://doi.org/10.5109/6781097.
- Sheridan, J. C., S. Lyndall, and O. Clara. 2010. Analysis without Anguish: SPSS Version 17.0 for Windows, 67–71. Australia: John Wiley & Australia, Publishers Ltd.
- Shiraishi, N., and N. Yabe. 2014. “MEMS Project in Saudi Arabia: Global Solution Business Initiative.” Fujitsu Scientific & Technical Journal 50:9–15.
- Simonofski, A., E. S. Asensio, and Y. Wautelet. 2019. “Chapter 4 - Citizen Participation in the Design of Smart Cities: Methods and Management Framework.” In Smart Cities: Issues and Challenges, edited by A. Visvizi and M. D. Lytras, 47–62, ISBN 9780128166390, Elsevier. https://doi.org/10.1016/B978-0-12-816639-0.00004-1.
- Spiliotopoulou, M., and M. Roseland. 2020. “Urban Sustainability: From Theory Influences to Practical Agendas.” Sustainability 12 (18): 7245. https://doi.org/10.3390/su12187245.
- Stepniak, M., and P. Rosik. 2018. “The Role of Transport and Population Components in Change in Accessibility: The Influence of the Distance Decay Parameter.” Networks and Spatial Economics 18 (2): 291–312. https://doi.org/10.1007/s11067-017-9376-8.
- Streukens, S., and S. Leroi-Werelds. 2016. “Bootstrapping and PLS-SEM: A Step-By-Step Guide to 26, Get More Out of Your Bootstrap Results.” European Management Journal 34 (6): 618–632. https://doi.org/10.1016/j.emj.2016.06.003.
- Tabachnick, B. G., and L. S. Fidell. 2007. Using Multivariate Statistics, 46–49. 5th ed. Boston: Pearson Education Inc.
- Tabuchi, T. 1998. “Urban Agglomeration and Dispersion: A Synthesis of Alonso and Krugman.” Journal of Urban Economics 44 (3): 333–351. https://doi.org/10.1006/juec.1997.2074.
- Talari, S., M. Shafie-Khah, P. Siano, V. Loia, A. Tommasetti, and J. P. S. Catalão. 2017. “A Review of Smart Cities Based on the Internet of Things Concept.” Energies 10 (4): 421. https://doi.org/10.3390/en10040421.
- Tenenhaus, M., V. E. Vinzi, Y. M. Chatelin, and C. Lauro. 2005. “PLS Path Modeling.” Computational Statistics & Data Analysis 48 (1): 159e205. https://doi.org/10.1016/j.csda.2004.03.005.
- Thornbush, M. J., and O. Golubchikov. 2019. Sustainable Urbanism in Digital Transitions: From Low Carbon to Smart Sustainable Cities. Springer Publıshers.
- UN-Habitat. 2019. “Saudi Cities Report. Available online: Accessed June 15, 2023. https://unhabitat.org/saudi-cities-report-2019.
- van Zoonen, L. 2016. “Privacy Concerns in Smart Cities.” Government Information Quarterly 33 (3): 472–480. https://doi.org/10.1016/j.giq.2016.06.004.
- Wlodarczak, P. 2017. “Smart Cities – Enabling Technologies for Future Living.” In City Networks. Springer Optimization and Its Applications, edited by A. Karakitsiou, A. Migdalas, S. Rassia, and P. Pardalos, Vol. 128. Cham: Springer https://doi.org/10.1007/978-3-319-65338-9_1.
- World Bank Group. 2017. “Sovereign Catastrophe Risk Pools: World Bank Technical Contribution to the G20.” World Bank. Retrieved from https://maint.elibrary.worldbank.org/.
- Xie, J., H. Tang, T. Huang, F. R. Yu, R. Xie, J. Liu, and Y. Liu. 2019. “A Survey of Blockchain Technology Applied to Smart Cities: Research Issues and Challenges.” IEEE Communications Surveys & Tutorials 21 (3): 2794–2830. https://doi.org/10.1109/COMST.2019.2899617.
- Yigitcanlar, T., K. Desouza, L. Butler, and F. Roozkhosh. 2020. “Contributions and Risks of Artificial Intelligence (AI) in Building Smarter Cities.” Energies 13 (6): 1473. https://doi.org/10.3390/en13061473.
- Zakka, S. D., A. S. Permana, H. C. Siong, A. N. Baba, and O. P. Agboola. 2017. “Implications of Present Land Use Plan on Urban Growth and Environmental Sustainability in a Sub-Saharan Africa City.” International Journal of Built Environment and Sustainability (IJBES) 4 (2): 105–112. https://doi.org/10.11113/ijbes.v4.n2.181. ISNN 1551-1369.
- Zeng, X., Y. Yu, S. Yang, Y. Lv, and M. N. I. Sarker. 2022. “Urban Resilience for Urban Sustainability: Concepts, Dimensions, and Perspectives.” Sustainability 14 (5): 2481. https://doi.org/10.3390/su14052481.
- Zubairu, M. 2020. “Assessment of the Implementation of the National Urban Policy, 2012, and Formulation of Sub-National Urban Policy and Smart City Strategy by Niger State Government, Nigeria.” In Developing National Urban Policies, edited by D. Kundu, R. Sietchiping, and M. Kinyanjui, 255–279. Singapore: Springer Nature.