ABSTRACT
Tarim River basin is the largest endorheic river basin in China. Due to the extremely arid climate the water supply solely depends on water originating from the glacierised mountains with about 75% stemming from the transboundary Aksu River. The water demand is linked to anthropogenic (specifically agriculture) and natural ecosystems, both competing for water. Ongoing climate change significantly impacts the cryosphere. The mass balance of the glaciers in Aksu River basin was clearly negative since 1975. The discharge of the Aksu headwaters has been increasing over the last decades mainly due to the glacier contribution. The average glacier melt contribution to total runoff is 30–37% with an estimated glacier imbalance contribution of 8–16%. Modelling using future climate scenarios indicate a glacier area loss of at least 50% until 2100. River discharge will first increase concomitant with glacier shrinkage until about 2050, but likely decline thereafter. The irrigated area doubled in the Aksu region between the early 1990s and 2020, causing at least a doubling of water demand. The current water surplus is comparable to the glacial runoff. Hence, even if the water demand will not grow further in the future a significant water shortage can be expected with declining glacial runoff. However, with the further expansion of irrigated agriculture and related industries, the water demand is expected to even further increase. Both improved discharge projections and planning of efficient and sustainable water use are necessary for further socioeconomic development in the region along with the preservation of natural ecosystems.
Introduction
The availability of water is of high importance for the sustainable development of the arid Xinjiang Uyghur Autonomous Region in north-western China, where the mean annual precipitation is less than 100 mm/a and the potential evaporation can be up to about 3000 mm/a in the lowlands (Shen and Chen Citation2010). Hence, the settlements and cities, the agricultural land and natural ecosystems depend on and compete for the river water originating from glacierised mountains surrounding the endorheic Tarim basin (Thevs Citation2011; Rumbaur et al. Citation2015; Fang et al. Citation2018). The strong dependence on glacier melt water along with the anthropogenic development is making the Tarim basin one of the most vulnerable basins in the world (Immerzeel et al. Citation2020). The Tarim River is the main artery at the northern margin of the Taklamakan desert and terminates at Lake Taitema in the eastern Taklamakan. Important natural vegetation are the riparian Tugai forests, which are dominated by Populus euphratica. Tugai forests are not only a rich natural ecosystem but also regulate the climate of the oasis, prevent desert expansion, protect from sandstorms and are a source for firewood and timber (Thevs et al. Citation2009; Zeng et al. Citation2020). However, the area of the Tugai forests significantly decreased during the last decades specifically as a result of the huge increase in agricultural water demand (Li et al. Citation2013).
The main tributaries to the Tarim River are the Hotan River (originating mainly from the Western Kunlun Shan), the Yarkant River (Eastern Pamir, Northern Karakoram), and the Aksu River (Central and Eastern Tien Shan). About three quarters of Tarim’s water is provided by the Aksu, while the Hotan contributes water only during the meltwater and precipitation peak in summer (Chen et al. Citation2003). The Yarkant contributed only during two extreme flood events to the Tarim since 1985. We therefore concentrate our study on the Aksu basin (). Draining from Kyrgyzstan into Xinjiang, the Sary-Jaz (Kunmalike in Chinese) and Kokshaal (Toxkan in Chinese) rivers are by far the largest tributaries of Aksu River. The glacier meltwater contribution to runoff of Aksu River is highly important. Estimates vary between about 30% to 45% (Aizen and Aizen Citation1998; Sorg et al. Citation2012; Duethmann et al. Citation2015). Hence, the runoff of these rivers is significantly impacted by the characteristics and changes of the cryosphere, dominating large parts of the catchment, with the highest peak being Jengish Chokusu (7439 m a.s.l., Pik Pobeda in Russian; Tomur Feng in Chinese).
Figure 1. Map of the Aksu headwater catchments and irrigation systems along the Aksu River (top) and true-colour composite satellite imagery of the region (~2020, bottom).
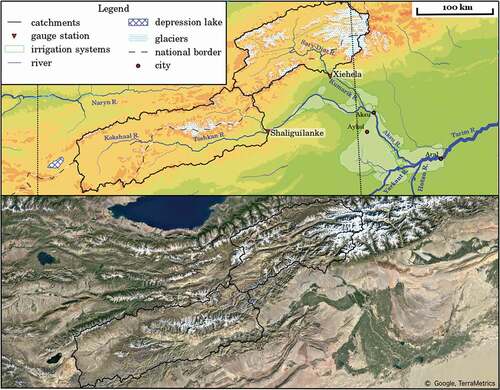
The mean annual air temperature has significantly increased in the Tien Shan during the last decades, like in many other regions of the Earth. In-situ precipitation measurements showed no significant trends in Tien Shan in Kyrgyzstan (Sorg et al. Citation2012). However, a transition from warm-dry to warm-wet conditions with both increasing temperatures and precipitation since the end of the 1980s were recorded for north-western China (Shi et al. Citation2007; Tao et al. Citation2021). Gridded climate data over the Aksu basin revealed a significant positive temperature trend for most areas, with higher rates and higher significance levels over the mountainous Kyrgyz part of the catchment for the analysed period 1961–2001. Precipitation showed significant positive trends in lower elevations, but no significant trend for most of the grid points over higher elevations (Krysanova et al. Citation2015).
The glacier coverage in Aksu basin is approximately 3,500 km2, which is more than one-fifth of the glacier coverage of the whole Tien Shan (Pieczonka and Bolch Citation2015). In response to climate change, glaciers lost area and mass during the last decades with mass loss rates between 0.2 and 0.3 m water equivalent per year (w.e. a−1) and slightly decreased mass loss since 2000 (Sorg et al. Citation2012; Osmonov et al. Citation2013; Pieczonka et al. Citation2013; Farinotti et al. Citation2015; Pieczonka and Bolch Citation2015; Bolch et al. Citation2021). Analysis using AVHRR and MODIS satellite data from 1986 to 2012 showed that snow cover probability slightly decreased in lower altitudes up to 4000 m a.s.l., but there was an opposite trend at higher elevations. However, the trends were not significant (Peters et al. Citation2015).
The runoff of Aksu River has increased by about 30% since the start of the measurements at the end of the 1950s (Yu et al. Citation2011). This was mainly attributed to increased glacier melt due to atmospheric warming (Duethmann et al. Citation2015). Hence, a large part of the increased runoff can be attributed to increased glacier mass loss. However, with continuing mass loss the glacier melt water will decrease after a tipping point is reached (Huss and Hock Citation2018).
About 95% of the anthropogenic water demand in the Aksu-Tarim basin is due to irrigation of agricultural land (Fang et al. Citation2018). As the irrigated areas have significantly expanded both in the lower Aksu and the whole Tarim basin and more water dependent crops are grown, the water demand has strongly increased (Rumbaur et al. Citation2015; Huang et al. Citation2018; Wang et al. Citation2019). Consequently, runoff measured at Alar station (Tarim) downstream of the large irrigated areas (for the location see ) decreased as a result (Deng et al. Citation2013; Huang et al. Citation2018; Duethmann et al. Citation2021).
It is, hence, of high importance for a sustainable development of the Tarim region to carefully consider the potential future water supply from the glacierised mountains and plan the water consumption accordingly. The aims of this study are therefore:
To assess the past glacier changes in the Aksu headwater catchments,
Investigate the potential future glacier changes and the impact on runoff,
Model the future water availability form the Aksu headwater catchments
Assess the past changes of the water demand of the agricultural land and relate them to the water supply,
Provide recommendations for future land use along the Aksu and Tarim rivers and to improve the knowledge about the water supply.
To achieve these aims we both consider existing publications, summarise results of own previous work, mainly conducted within the project Sustainable Management Along the River Oasis of Tarim River (SuMaRiO, www.sumario.de, Rumbaur et al. Citation2015; Cyffka et al. Citation2021) and performed additional new analyses.
Methods and data
Water supply: past and future changes of glaciers and runoff
We used the glacier inventory generated by Pieczonka and Bolch (Citation2015) for the most important baseline information about glaciers, such as the general occurrence and area. The glacier inventory was generated based on Landsat TM data from about 2008. Most accurate information about the glacier changes can be obtained by in-situ mass balance measurements. However, these direct measurements are laborious, moreover most glaciers are located in remote areas and are therefore difficult to access. The closest glacier with long-term mass balance measurements is Urumchi Glacier No. 1 located in Eastern Tien Shan in Xinjiang. Few mass balance measurements are available for glaciers in the Ak-Shirak region (e.g. Glacier No. 345) and Terskey Alatau (Karabatkak Glacier) in Kyrgyzstan, but there are no glaciers with long-term direct measurements in the whole Aksu basin apart from very few ablation measurements at selected glaciers (e.g. Hagg et al. Citation2008; Juen et al. Citation2014). A suitable alternative to obtain information about glacier mass changes, especially for larger areas, is the geodetic method, i.e. to compare the surface elevation and volume changes of glaciers using multi-temporal digital elevation models (DEMs). We extracted the glacier mass changes data for periods 1975–2000 and 2000–2018 based on Pieczonka and Bolch (Citation2015) and Shean et al. (Citation2020). The former study used Hexagon KH-9 and the SRTM DEM while the latter study is mainly based on ASTER and Worldview DEMs.
The runoff for the Aksu headwater catchments was calculated based on two different glacio-hydrological models, WASA (Duethmann et al. Citation2016) and SWIM-G (Wortmann et al. Citation2019). WASA simulates hydrological processes, such as infiltration, evapotranspiration, runoff generation, snow and glacier melt, as well as long-term changes in glacier area and glacier surface elevation. The spatial discretization is primarily based on subbasins and elevation. For the calculation of the glacier geometry changes, all glaciers are considered individually and a finer spatial discretization is applied. In order to calculate changes in glacier area and surface elevation first local glacier mass balances are calculated and these are then redistributed according to an empirically derived function that accounts for ice flow. The glacio-hydrological model SWIM-G was specifically designed for large and data-scarce catchments, such as the Tarim tributaries (Wortmann et al. Citation2019). It disaggregates complex terrain into glacier response units, based on elevation, slopes and subbasins, that are used to simulate the ice flow of individual glaciers in an efficient manner. They seamlessly integrate with the underlying semi-distributed hydrological model. The important glacier processes are represented, including glacier melt, avalanching, sublimation and debris accumulation, and ice flow is explicitly simulated. The glacier dynamics of the module need to be initialised to reach an equilibrium with the driving data, which also allows for the calibration of the model components – high-elevation precipitation in particular (Wortmann et al. Citation2018) – to observed glacier extent and thickness (if available). Implemented and calibrated to glacier extent and/or thickness changes as well as measured runoff in the Tarim headwaters (e.g. Wang Citation2006; Yu et al. Citation2011), both models were used to simulate the past and future water balance, using three Representative Concentration Pathways (RCP) scenarios (2.6, 4.5, 8.5) and output of eight General Circulation Models (GCM) (Duethmann et al. Citation2016; Wortmann et al. Citation2021).
Water demand
We identified irrigated land and its changes based on Landsat TM and OLI satellite data. Four scenes were needed (path/row 146/31, 146/32, 147/31, 147/32) to cover the major parts of the irrigated land of this basin. The goal was to obtain a full coverage with imagery having the least cloud cover and a similar acquisition time in summer from the period around 1990 and from the most recent time. We finally selected scenes from 13/07/1992, 20/08/1994 and 30/09/1994, as well as 26/09/2019 and 27/09/2020.
Atmospherically corrected (Level 2) versions of these scenes were ordered and downloaded from the USGS Earth Explorer website. These scenes were then mosaicked with Path/Row 146/031 and 146/032 (acquired 30/09/1994 and 27/09/2020) being the primary source as about three quarters of the irrigated land was covered by those.
A supervised classification was performed for each mosaic with 20 initial classes, which were then merged into 10 final classes.
The information for the water demand for each crop is based on Wang et al. (Citation2019) who estimated the crop water requirement for the different crops and subcatchments of the Tarim basin (amongst the Aksu basin) for the period 1990–2015. The percentage of the different crops are based on the statistical year books (Huang et al. Citation2018) and Wang et al. (Citation2019). We assumed that the percentage of crops in 2019/2020 was similar to 2015 as more recent data was unavailable.
Results and discussion
Observed past and projected future glacier changes
Glaciers of whole Aksu basin showed a total mass loss of 0.56 ± 0.09 Gt (specific mass balance: −0.18 ± 0.03 m w.e. a−1) between 2000 and 2018 according to the data of Shean et al. (Citation2020). Glaciers in the Sary-Jaz subcatchment, which cover almost 80% of the glacierised area, experienced clearly higher mass loss than the glaciers of the Kokshaal subcatchment, both in absolute values (0.52 ± 0.08 Gt) and the specific mass balance (−0.21 ± 0.04 m w.e. a−1). This is in agreement with the finding that glaciers in the Kokshaal-Too mountain range, which constitutes the major part of the Kokshaal catchment, showed clearly lower shrinkage than glaciers in most of the other mountain ranges of the Aksu catchment (Pieczonka and Bolch Citation2015). In the period 1975–2000, glaciers had probably a higher mass loss of 0.92 ± 0.86 Gt (specific mass balance −0.33 ± 0.31 m w.e. a−1) although the uncertainty is large. Pieczonka et al. (Citation2013) found also slightly decreased mass loss in the region for the period 1999–2009 (0.23 ± 0.19 m w.e. a−1) in comparison to 1976–1999 (0.42 ± 0.23 m w.e. a−1) of the glaciers south of Tomur Peak/Jengish Chokusu, directly adjacent to our study region. No mass balance data is available for the Kokshaal catchment before 2000. The slightly decreased mass loss in the Aksu catchment is in contrast to the entire Tien Shan and most other regions of High Mountain Asia, where the glacier mass loss is clearly higher in the decade(s) after 2000 than before (Bolch et al. Citation2020; Bolch et al., Citation2019b; Farinotti et al. Citation2015; King et al. Citation2019; Zhou et al. Citation2018). However, studies agree that the mass loss in the Central Tien Shan is lower than in the other parts of the Central Asian’s largest mountain range (Sorg et al. Citation2012; Farinotti et al. Citation2015).
All model scenarios project a clear glacier recession in both subcatchments until the end of this century. According to the WASA model and the worst scenario (RCP-8.5), an almost entire deglaciation of the Kokshaal catchment is possible and also the highly glacierised Sary-Jaz catchment could lose about 80% of the area relative to the 1971–2000 reference period (Wortmann et al. Citation2021). Even with the most positive scenario (RCP-2.6) the glaciers would lose at least 40% of their area. SWIM-G simulates consistently lower area losses until 2100, which can mainly be attributed to the fact that glacier flow and debris accumulation, that is abundant in our study region, are explicitly considered. While the former compensates mass loss in the ablation region due to increased flow from the accumulation region to a certain degree, the latter is considered to reduce surface melt. However, this model does not consider the increased melt of debris-covered glaciers due to ice cliffs, supra- and proglacial lakes (e.g. Juen et al. Citation2014; Ragettli et al. Citation2016; King et al. Citation2019). Farinotti et al. (Citation2015) could also simulate the obtained geodetic results for the Central Tien Shan regions well without considering debris cover, which could be attributed to the fact that the reducing and increasing melt factors of debris-covered glaciers could balance each other.
These results match well the projections of Rounce et al. (Citation2020) for Central Tien Shan, who simulate a mass loss between 40% and 80% depending on the RPC scenario. Similar deglaciation is projected by Kraaijenbrink et al. (Citation2017) for Western Tien Shan, where the Central Tien Shan is a part of.
Past and future runoff and glacier melt contribution
Previous results indicate that the increased runoff of Aksu River is mainly related to the temperature increase and the associated increase in glacier melt in the highly glacierised Sary-Jaz catchment, while both temperature and precipitation increase were equally important in the less glacierised Kokshaal catchment (Duethmann et al. Citation2015). The same study finds a glacier melt contribution (as defined as firn and ice melt without the contribution of snow melt or rain from the glacier area) of 9–24% for the Kokshaal and 35–48% for the Sary-Jaz catchment. Zhao et al. (Citation2013) estimated also based on glacio-hydrological modelling 23% and about 44% glacier melt contribution for the same rivers. Pritchard (Citation2019) estimates even a contribution of about 50%. The glacier imbalance contribution (the additional glacier contribution due to the ongoing mass loss) was estimated to be between 4–21% and 21–40%, respectively, based on modelling by Duethmann et al. (Citation2015) and about 12–15% for whole Aksu by Pritchard (Citation2019). Pieczonka and Bolch (Citation2015) estimate the glacier imbalance contribution to be about 20% for Sary-Jaz, based on comparison of the geodetic mass balance estimates and available runoff data for the period before 2000. The comparison of the geodetic mass balance estimates by Shean et al. (Citation2020) and available runoff data reveals a lower imbalance contribution of about 10% for Sary-Jaz and only 2% for Kokshaal. The lower relative contribution after 2000 can be explained by the decreased mass loss and the overall increased runoff. We used the most reasonable ranges estimate for the total glacier contribution and the glacier imbalance contribution for this study ().
Table 1. Glacier contribution to total runoff for the Sary-Jaz River (measured at Xiehela station) and Kokshaal River (Shaliguilanke station); data sources glacier melt: Duethmann et al. (Citation2015), Pieczonka and Bolch (Citation2015), Zhao et al. (Citation2013), data source runoff: Yu et al. (Citation2011). The glacier contribution was calculated for the priod after 2000. No runoff data was available for the period after 2008. We consider the period 2000-2008 to be also representative for the period until 2020
Modelling results using different GCMs project both increases in air temperature and precipitation for the Aksu headwater regions. While a clear temperature increase is projected for all models and scenarios, the precipitation increase is less strong and some lower ranges project even precipitation decrease (Duethmann et al. Citation2021). As a result, all scenarios project an overall increase in runoff of Aksu River until 2040 in both Sary-Jaz and Kokshaal catchments (). Most scenarios project a runoff decline in the Kokshaal catchment with the strongest decline shown in the WASA model and the RCP 8.5 scenario. This can mainly be attributed to the decreased glacier melt. Results are contrasting for Sary-Jaz river; while the WASA model projects a runoff decline for all RCP scenarios, an increase in runoff is projected by the SWIM-G model under RCP-8.5 until the period 2071–2100 (). Hagg et al. (Citation2018) projects a runoff decline only after an area reduction of more than 43% for a subcatchment including Northern and Southern Inylchek glaciers, the largest glaciers in the whole Aksu River basin. The most likely scenario for the entire Aksu River is a runoff increase until around 2050 and a decline thereafter. This tendency is also in line with the projections by Huss and Hock (Citation2018) and Rounce et al. (Citation2020) although none of these studies model the Aksu River separately.
Figure 2. Projected future changes in annual mean discharge of Sary-Jaz and Kokshaal catchments simulated by SWIM-G and WASA relative to the baseline period 1971–2000 for three different RPC scenarios (A); Projected seasonality changes in runoff for Aksu River (B), data source: Wortmann et al. (Citation2021)

Figure 3. Landsat false colour mosaics (SWIR-NIR-RED) of the irrigated area around Aksu of about 1994 and 2020 (above) and classified land cover/land use of the year 2020 (below).
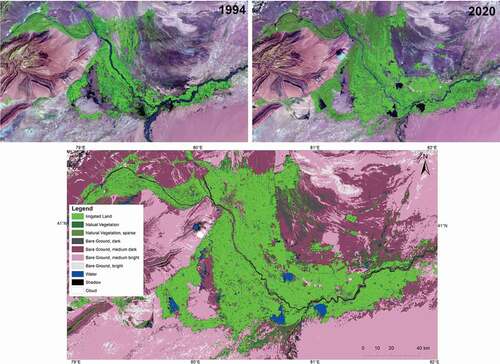
Even in the unlikely runoff scenario with only little or no overall runoff decline until the end of this century, the seasonality will be strongly affected: All model results agree that there will be a runoff increase in spring but a runoff decline in the summer months (), when the water demand for irrigation is very high. Similar modelling results were obtained for the adjacent Kaidu catchment (Fang et al. Citation2017) and other glacierised river catchments in other regions (e.g. Hagg et al. Citation2006; Huss Citation2011). It needs, however, to be noted that none of these studies considered the existence of subsurface ice under permafrost conditions (e.g. stored in rock glaciers), which can make a significant portion of the ice in the study region (Bolch et al. Citation2019a).
Changes in irrigated area and water demand
Our results reveal a doubling of the irrigated area from about 4100 km2 to about 8200 km2 from 1994 to 2020 (). The irrigated area has expanded in almost all directions along the middle and lower reaches along Aksu River but with lower expansion along the Kokshaal (here called: Toxkan) River. A clear extension occurred also along the Tarim River downstream of the confluence of Aksu, Hotan and Yarkant rivers (). Analysis of statistical year books revealed also a clear increase in cultivated area in the Aksu catchment between 1989 and 2011 with the strongest increase between 2004 and 2009 (Huang et al. Citation2018). Changes of irrigated land for the whole Tarim River basin were even larger with an increase from 13,300 km2 in 1990 to more than 33,500 km2 in 2015 according to data from the Data Center for Resources and Environmental Sciences, Chinese Academy of Sciences (Wang et al. Citation2019). Our analysis revealed that the total water demand in our investigated area more than doubled between 1994 and 2020 (from about 2.54 to 5.75 km3 a−1). This can be mainly attributed to the increase in irrigated areas but also to the slight increase in water demand per area ().
Table 2. Changes in irrigated land and annual water demand of the irrigated area. 1Average data calculated based on data from Wang et al. (Citation2019), p. 2 See 3 Estimated based on the average total runoff and the irrigation water demand
Cotton is the main crop cultivated in the Aksu basin accounting for approximately 50% of the total agricultural land. The share of winter wheat dropped from more than 25% to about 10% and the share of rice continuously declined to about 5% between 1989 and 2011, while fruit crops increased to about 20% in 2011 (Huang et al. Citation2018). Hence, the agricultural land is dominated by water demanding crops like cotton and fruit trees. The share of fruit trees strongly increased although they have the highest water demand amongst the crops grown in the Aksu basin, explaining the higher water demand per unit.
Similar trends are also observed for the whole Tarim basin. The irrigation water requirements more than doubled since 1990 and especially increased since 2004 concomitant with the general increase of the cultivated land and the increased growth of water demanding fruits (Wang et al. Citation2019). Runoff measured at Alar station of Tarim River downstream of the large irrigated areas (for the location see ) decreased as a result of the increased water demand (Deng et al. Citation2013; Huang et al. Citation2018; Duethmann et al. Citation2021).
The strong increase in water demand for irrigation along with only a slight runoff increase led to a halving in water surplus for other uses, i.e. water available for households, industry or to feed the middle and lower Tarim River. This water surplus is in the range of the total glacier runoff (both the balance and imbalance runoff, ). Hence specifically downstream of Alar both the natural ecosystems and the anthropogenic land use will suffer from potential future water shortages.
Calculation with the hydrological model MIKE SHE for the Tarim river oasis Yingibazar located in the middle reach of Tarim River showed that the strong increases of the agricultural land in the past (2004–2012, ) had a great influence on the ecosystem and the impact of future land use will further increase (Keilholz et al. Citation2015). In the near future (2050), the water demand can probably be satisfied by higher river discharge as a consequence of the increased icemelt. However, in the more distant future (2100) the glacier mass will be reduced, the river discharge will decrease and the water availability will decline strongly if not counterbalanced by increases in precipitation (Keilholz et al. Citation2015). This lack of water will produce water stress and increase the desertification of the area ().
Figure 4. Changes in the vitality zones in future scenarios caused by land-use changes along the river oasis Yingibazar and land-use changes combined with climate change; source: Keilholz et al. (Citation2015)
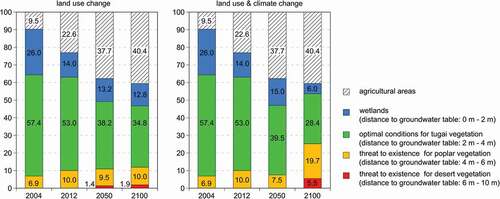
A further important point to consider is the expected change in seasonality of runoff. Specifically, in the summer months when water demand is very high a significant decline of glacier melt water can be expected. Hence, it is evident that glacier runoff is essential for the water supply in this arid region and that water shortages can be expected in the future with declining glacier runoff. Even today the glacial runoff is crucial as glaciers provide a reliable water source while runoff from rain shows high annual and interannual variability (Duethmann et al. Citation2016; Bolch Citation2017). The drastic increase in agricultural water demand has already led to water shortages and ecological degradation in the lower parts of Tarim River and the Tugai forests and other important riparian ecosystems are at risk (Thevs et al. Citation2009; Shen et al. Citation2013; Rumbaur et al. Citation2015).
Though most scenarios project a runoff increase of Hotan and Yarkant until 2100 due to the projected precipitation increase but also the large glacier cover and the fact that Eastern Pamir and Western Kunlun exhibited balanced budgets during the last decades (Wortmann et al. Citation2021; Bolch et al., Citation2019b; Holzer et al. Citation2015; Shean et al. Citation2020), it remains very uncertain if and to what extent they can counterbalance the decline in runoff of Aksu River. Their overall contribution to Tarim River has been only about 25% in the past and increased water demand is also expected along these rivers.
Conclusions and recommendations
Glaciers have continuously lost area and mass in the headwaters of Aksu River in Central Tien Shan and are projected to further decline. It can be expected that at least 50% of the glacier area will be lost until the end of this century but greater deglaciation is more likely. The further glacier decline will lead to an initial increase of runoff of Aksu River but the runoff will very likely decline after a tipping point will be reached between 2040 and 2060. The irrigation water demand more than doubled since the end of the last century and the current water surplus is similar to the glacial runoff. Hence, even if the water demand will not grow further in the future, a significant water shortage can be expected in the long term with declining glacier runoff leading to even stronger competition for water both amongst the anthropogenic needs and the anthropogenic and natural water demand. A reduction in water consumption is needed in order to sustain the current or growing irrigation agricultural land and to restore and preserve natural ecosystems. Moreover, as the uncertainties of the future projections are large, the collection of the data and the related modelling need to be further improved. This is specifically true for the precipitation in high altitudes and their future changes. Therefore, we recommend the following measures:
Integrated studies at selected benchmark catchments with intensive climatological, hydrological (esp. discharge measurements) and glaciological (in-situ mass balance complemented by remote-sensing derived geodetic mass balance) measurements.
Geophysical investigations to detect subsurface ice.
Improved high-resolution climate modelling to better understand the climate system in high-mountain areas and reduce uncertainties of future climate change projections.
Establishing a remote sensing-based snow and glacier monitoring for the entire Tarim basin.
Promoting agricultural production technologies that save water and control salinity and the planting of crops that have low water requirements.
Significant increase of the price for agricultural water and compensation for income losses by targeted subsidization of water-efficient production (i.e. drip irrigation).
Shifting towards labor-intensive high-value commodities (e.g. vegetables) and therefore reducing the agricultural area.
Re-establishment of a continuous flow of water in the Tarim River down to its terminal lake Taitema to maintain and restore the Tugai forests along the lower reaches of the Tarim River.
Acknowledgments
The study is based on results of the project Sustainable Management of River Oases along the Tarim River (SuMARiO) supported by the German Ministry of Science and Education (Bunderministerium für Bildung und Forschung, BMBF, Code 01 LL0918 A-K).
Disclosure statement
No potential conflict of interest was reported by the author(s).
Additional information
Funding
References
- Aizen VB, Aizen EM. 1998. Estimation of glacial runoff to the Tarim river, central Tien Shan. IAHS Publ. 248:191–198.
- Bolch T. 2017. Asian glaciers are a reliable water source. Nature. 545(7653):161–162. doi:https://doi.org/10.1038/545161a.
- Bolch T, Rohrbach N, Kutuzov S, Robson BA, Osmonov A. 2019a. Occurrence, evolution and ice content of ice-debris complexes in the Ak-Shiirak, Central Tien Shan revealed by geophysical and remotely-sensed investigations. Earth Surf Process Landforms. 44(1):129–143. doi:https://doi.org/10.1002/esp.4487.
- Bolch T, Shea JM, Liu S, Azam FM, Gao Y, Gruber S, Immerzeel WW, Kulkarni A, Li H, Tahir AA, et al. 2019b. Status and change of the cryosphere in the extended Hindu Kush Himalaya region. In: Wester P, Mishra A, Mukherji A, Ab S, editors. The Hindu Kush Himalaya assessment: mountains, climate change, sustainability and people. Cham: Springer International Publishing; p. 209–255.
- Bolch T, Bhattacharya A, King O. 2020. Die Gletscher Hochasiens im Klimawandel. In: Lozán JL, S-W Breckle, Graßl H, Paul F, editors. Warnsignal Klima: Hochgebirge im Wandel. Verlag Wissenschaftliche Auswertungen, Hamburg; p. 23–27.
- Bolch T, Pieczonka T, Peters J, Mukherjee K, Goerlich F, Shangguan D, Liu S, Kurban A, Osmonov A, Moldobekov B, et al. 2021. Characteristics and changes of the cryosphere in the Aksu-Tarim basin (Central Tien Shan). In: Cyffka B, Disse M, Betz F, editors. Sustainable management of river oases along the Tarim river/China (SuMaRiO). Stuttgart (Germany): Schweizerbart; p. 41–52.
- Chen, YN, Li, W, Chen Y, and Zhang, H. 2003. Water resources and ecological problems in Tarim River Basin, Xinjiang, China. In: Wilderer, P. A., Zhu, J., and Schwarzenbeck, N. (eds), Water in China. Water and Environmental Management Series. London: IWA Publishing. 3–12.
- Cyffka B, Disse M, Beetz F. Eds. 2021. Sustainable management of river oasis along the Tarim River/China (SuMaRiO). Stuttgart: Schweizerbart.
- Deng X, Yang Z, Long A. 2013. Ecological operation in the Tarim River basin based on rational allocation of water resources. J Glaciol Geocryol. 35:1600–1609.
- Duethmann D, Huang S, Wortmann M, Krysanova V, Vorogushyn S. 2021. Hydrology of the upper Tarim River. In: Cyffka B, Disse M, Betz F, editors. Sustainable management of river oases along the Tarim river/China (SuMaRiO). Stuttgart (Germany): Schweizerbart; p. 53–64.
- Duethmann D, Bolch T, Farinotti D, Kriegel D, Vorogushyn S, Merz B, Pieczonka T, Jiang T, Su B, Güntner A. 2015. Attribution of streamflow trends in snow- and glacier melt dominated catchments of the Tarim River, Central Asia. Water Resour Res. 51(6):4727–4750. doi:https://doi.org/10.1002/2014WR016716.
- Duethmann D, Menz C, Tong J, Vorogushyn S. 2016. Projections for headwater catchments of the Tarim River reveal glacier retreat and decreasing surface water availability but uncertainties are large. Environ Res Lett. 11(5):54024. doi:https://doi.org/10.1088/1748-9326/11/5/054024.
- Fang G, Chen Y, Li Z. 2018. Variation in agricultural water demand and its attributions in the arid Tarim River Basin. J Agricult Sci. 156(3):301–311. doi:https://doi.org/10.1017/S0021859618000357.
- Fang G, Yang J, Chen Y, Li Z, Maeyer PD. 2017. Impact of GCM structure uncertainty on hydrological processes in an arid area of China. Hydrol Res. 49(3):893–907. doi:https://doi.org/10.2166/nh.2017.227.
- Farinotti D, Longuevergne L, Moholdt G, Duethmann D, Mölg T, Bolch T, Vorogushyn S, Guntner A. 2015. Substantial glacier mass loss in the Tien Shan over the past 50 years. Nature Geosci. 8(9):716–722. doi:https://doi.org/10.1038/ngeo2513.
- Hagg W, Braun L, Weber M, Becht M. 2006. Runoff modelling in glacierized Central Asian catchments for present-day and future climate. Nord Hydrol. 37(2):93–105. doi:https://doi.org/10.2166/nh.2006.0008.
- Hagg W, Mayer C, Lambrecht A, Helm A. 2008. Sub-debris melt rates on southern inylchek glacier, central tian shan. Geogr Ann. 90A(1):55–63. doi:https://doi.org/10.1111/j.1468-0459.2008.00333.x.
- Hagg W, Mayr E, Mannig B, Reyers M, Schubert D, Pinto J, Peters J, Pieczonka T, Juen M, Bolch T, et al. 2018. Future climate change and its impact on runoff generation from the debris-covered Inylchek Glaciers. Central Tian Shan, Kyrgyzstan Water. 10(11):1513. doi:https://doi.org/10.3390/w10111513
- Holzer N, Vijay S, Yao T, Xu B, Buchroithner M, Bolch T. 2015. Four decades of glacier variations at Muztagh Ata (eastern Pamir): a multi-sensor study including Hexagon KH-9 and Pléiades data. Cryosphere. 9(6):2071–2088. doi:https://doi.org/10.5194/tc-9-2071-2015.
- Huang S, Wortmann M, Duethmann D, Menz C, Shi F, Zhao C, Su B, Krysanova V. 2018. Adaptation strategies of agriculture and water management to climate change in the upper Tarim river basin, NW China. Agric Water Manage. 203:207–224. doi:https://doi.org/10.1016/j.agwat.2018.03.004.
- Huss M. 2011. Present and future contribution of glacier storage change to runoff from macroscale drainage basins in Europe. Water Resour Res. 47(7):W07511. doi:https://doi.org/10.1029/2010WR010299.
- Huss M, Hock R. 2018. Global-scale hydrological response to future glacier mass loss. Nature Clim Change. 8(2):135–140. doi:https://doi.org/10.1038/s41558-017-0049-x.
- Immerzeel WW, Lutz AF, Andrade M, Bahl A, Biemans H, Bolch T, Hyde S, Brumby S, Davies BJ, Elmore AC, et al. 2020. Importance and vulnerability of the world’s water towers. Nature. 577(7790):364–369. doi:https://doi.org/10.1038/s41586-019-1822-y
- Juen M, Mayer C, Lambrecht A, Han H, Liu S. 2014. Impact of varying debris cover thickness on ablation: a case study for Koxkar Glacier in the Tien Shan. The Cryosphere. 8(2):377–386. doi:https://doi.org/10.5194/tc-8-377-2014.
- Keilholz P, Disse M, Halik Ü. 2015. Effects of Land Use and Climate Change on Groundwater and Ecosystems at the Middle Reaches of the Tarim River Using the MIKE SHE Integrated Hydrological Model. Water. 7(6):2073–4441. doi:https://doi.org/10.3390/w7063040.
- King O, Bhattacharya A, Bhambri R, Bolch T. 2019. Glacial lakes exacerbate Himalayan glacier mass loss. Sci Rep. 9(1):18145. doi:https://doi.org/10.1038/s41598-019-53733-x.
- Kraaijenbrink PDA, Bierkens MFP, Lutz AF, Immerzeel WW. 2017. Impact of a global temperature rise of 1.5 degrees Celsius on Asia’s glaciers. Nature. 549(7671):257–260. doi:https://doi.org/10.1038/nature23878.
- Krysanova V, Wortmann M, Bolch T, Merz B, Duethmann D, Huang S, Jiang T, Su B, Vorogushyn S, Kundzewicz Z. 2015. Analysis of current trends in climate parameters, water discharge, glaciers and land cover in the Aksu-Tarim River basin /Central Asia). Hydrol Sci J. 60(4):566–590. doi:https://doi.org/10.1080/02626667.2014.925559.
- Li W, Zhou H, Fu A, Chen Y. 2013. Ecological response and hydrological mechanism of desert riparian forest in inland river, northwest of China. Ecohydrology. 6(6):949–955. doi:https://doi.org/10.1002/eco.1385.
- Osmonov A, Bolch T, Xi C, Wei J, Kurban A. 2013. Glaciers characteristics and changes in the Sary-Jaz river basin (central Tien Shan) 1990-2010. Remote Sens Let. 4(8):725–734. doi:https://doi.org/10.1080/2150704X.2013.789146.
- Peters J, Bolch T, Gafurov A, Prechtel N. 2015. Snow cover distribution in the Aksu Catchment (Central Tien Shan) 1986–2013 based on AVHRR and MODIS data. IEEE J Sel Top Appl Earth Observations Remote Sensing. 8(11):5361–5375. doi:https://doi.org/10.1109/JSTARS.2015.2477108.
- Pieczonka T, Bolch T. 2015. Region-wide glacier mass budgets and area changes for the Central Tien Shan between ~1975 and 1999 using Hexagon KH-9 imagery. Global Planet Change. 128:1–13. doi:https://doi.org/10.1016/j.gloplacha.2014.11.014.
- Pieczonka T, Bolch T, Wei J, Liu S. 2013. Heterogeneous mass loss of glaciers in the Aksu-Tarim Catchment (Central Tien Shan) revealed by 1976 KH-9 Hexagon and 2009 SPOT-5 stereo imagery. Remote Sens Environ. 130:233–244. doi:https://doi.org/10.1016/j.rse.2012.11.020.
- Pritchard HD. 2019. Asia’s shrinking glaciers protect large populations from drought stress. Nature. 569(7758):649–654. doi:https://doi.org/10.1038/s41586-019-1240-1.
- Ragettli S, Bolch T, Pellicciotti F. 2016. Heterogeneous glacier thinning patterns over the last 40 years in Langtang Himal, Nepal. Cryosphere. 10(5):2075–2097. doi:https://doi.org/10.5194/tc-10-2075-2016.
- Rounce DR, Hock R, Shean DE. 2020. Glacier mass change in High Mountain Asia through 2100 using the open-source python glacier evolution model (PyGEM). Front Earth Sci. 7:331. doi:https://doi.org/10.3389/feart.2019.00331.
- Rumbaur C, Thevs N, Disse M, Ahlheim M, Brieden A, Cyffka B, Duethmann D, Feike T, Frör O, Gärtner P, et al. 2015. Sustainable management of river oases along the Tarim River (SuMaRiO) in Northwest China under conditions of climate change. Earth Syst Dynam. 6(1):83–107. doi:https://doi.org/10.5194/esd-6-83-2015
- Shean DE, Bhushan S, Montesano P, Rounce DR, Arendt A, Osmanoglu B. 2020. A systematic, regional assessment of High Mountain Asia glacier mass balance. Frontiers Earth Sci. 7:363. doi:https://doi.org/10.3389/feart.2019.00363.
- Shen Y, Chen Y. 2010. Global perspective on hydrology, water balance, and water resources management in arid basins. Hydrol Proc. 24:129–135. doi: https://doi.org/10.1002/hyp.7428
- Shen Y, Li S, Chen Y, Qi Y, Zhang S. 2013. Estimation of regional irrigation water requirement and water supply risk in the arid region of Northwestern China 1989–2010. Agric Water Manage. 128:55–64. doi:https://doi.org/10.1016/j.agwat.2013.06.014.
- Shi Y, Shen Y, Kang E, Li D, Ding Y, Zhang G, Hu R. 2007. Recent and future climate change in Northwest China. Clim Change. 80(3):379–393. doi:https://doi.org/10.1007/s10584-006-9121-7.
- Sorg A, Bolch T, Stoffel M, Solomina O, Beniston M. 2012. Climate change impacts on glaciers and runoff in Tien Shan (Central Asia). Nature Clim Change. 2(10):725–731. doi:https://doi.org/10.1038/nclimate1592.
- Tao H, Su B, Wen S, Qin J, Tong J. 2021. Climate change and its impact on Xinjiang. In: Cyffka B, Disse M, Betz F, editors. Sustainable Management of River Oases along the Tarim River/China (SuMaRiO). Stuttgart (Germany): Schweizerbart; p. 33–40.
- Thevs N. 2011. Water scarcity and allocation in the Tarim Basin: decision structures and adaptations on the local level. J Current Chinese Affairs. 40(3):113–137. doi:https://doi.org/10.1177/186810261104000305.
- Thevs N, Zerbe S, Schnittler M, Abdusalih N, Succow M. 2009. Structure, reproduction and flood-induced dynamics of Tugai forests at the Tarim River in Xinjiang, NW China. Forestry. 81(1):45–57. doi:https://doi.org/10.1093/forestry/cpm043.
- Wang F, Chen Y, Li Z, Fang G, Li Y, Xia Z. 2019. Assessment of the irrigation water requirement and water supply risk in the Tarim River Basin. Northwest China Sustainability. 11(18): 2071–1050. doi:https://doi.org/10.3390/su11184941
- Wang Y. 2006. Local records of the Akesu river basin. Beijing: Fangzi Publisher.
- Wortmann M, Bolch T, Menz C, Tong J, Krysanova V. 2018. Comparison and correction of high-mountain precipitation data based on glacio-hydrological modelling in the Tarim River headwaters (High Asia). J Hydromet. 19(5):777–801. doi:https://doi.org/10.1175/JHM-D-17-0106.1.
- Wortmann M, Bolch T, Su B, Krysanova V. 2019. An efficient representation of glacier dynamics in a semi-distributed hydrological model to bridge glacier and river catchment scales. J Hydrol. 573:136–152. doi:https://doi.org/10.1016/j.jhydrol.2019.03.006.
- Wortmann M, Duethmann D, Menz C, Bolch T, Huang SC, Tong J, Kundzewicz ZW, Krysanova V. 2021. Climate change impacts on glaciers and water resources in the headwaters of the Tarim River, NW China/Kyrgyzstan. Clim Change. http://hdl.handle.net/10023/23115
- Yu P, Xu H, Liu S, An H, Zhang Q, Gong J. 2011. The nonlinear characteristics of annual runoff change in Aksu River. J Nat Resour. 26(8):1412–1422.
- Zeng Y, Zhao C, Kundzewicz ZW, Lv G. 2020. Distribution pattern of Tugai forests species diversity and their relationship to environmental factors in an arid area of China. PLoS ONE. 15(5):e0232907. doi:https://doi.org/10.1371/journal.pone.0232907.
- Zhao Q, Ye B, Ding Y, Zhang S, Yi S, Wang J, Shangguan D, Zhao C, Han H. 2013. Coupling a glacier melt model to the Variable Infiltration Capacity (VIC) model for hydrological modeling in north-western China. Environ Earth Sci. 68(1):87–101. doi:https://doi.org/10.1007/s12665-012-1718-8.
- Zhou Y, Li Z, Li J, Zhao R, Ding X. 2018. Glacier mass balance in the Qinghai–Tibet Plateau and its surroundings from the mid-1970s to 2000 based on Hexagon KH-9 and SRTM DEMs. Remote Sens Environ. 210:96–112. doi:https://doi.org/10.1016/j.rse.2018.03.020.