ABSTRACT
The mental representations of the body depend on current perceptions, building on more reliable sensory inputs and decreasing the weight of less reliable afferences. While somatosensory manipulations have been repeatedly investigated, less is known about vision. We hypothesized that a decrease in visual input may result in an augmented relevance of somatosensation to mentally represent the body. 29 neurotypical participants performed mental rotation of hand images, while image visibility was manipulated: keeping the same background (grey), the contrast was decreased by 60% (Degraded Vision) with respect to Baseline. Results showed that Degraded Vision (1) slowed down the mental rotation of hand images typically sensitive to degrees of rotation (dorsum), and (2) established a rotation-dependent latency profile for the mental rotation of hand images that are not typically affected by rotation (little finger). Since the sensitivity to rotation indicates the recruitment of visual or somatosensory strategies to mentally represent the body, our findings indicate that in presence of degraded visual input, somatosensation had a heavier weight than vision in mental rotation. This suggests a relative shift from a pictorial representation of the body (body image) to a somatosensory one (body schema) as a function of the most reliable/available sensory input.
Introduction
Despite mostly unconsciously, during our daily activities, we make massif use of mental imagery, a cognitive ability thanks to which mental representations are activated and provide an almost-perceptual experience, even in absence of the proper sensory input (Munzert et al., Citation2009). Activities like parking a car, grabbing an object, planning our way to the theatre, would not be possible without such ability to mentally predict the outcome of our actions. In experimental settings, body-related mental imagery can be investigated through the mental rotation of body parts: participants are presented with the image of a body part (rotated in different orientations) and are required to identify whether the body part is left or right (Perruchoud et al., Citation2016). Typically, people perform the task by imagining to moving their own corresponding body part in the position shown by the image (Parsons, Citation1994). This is reflected in the profile of the response times (RTs) to perform mental rotation, which is proportional to the orientation of the image: progressively increasing RTs for images presented at 0° to 180° and vice versa up to 360° (Ionta et al., Citation2007).
However, the shape of RT profiles is sensitive not only to image orientation but also to the perspective from which the body part is shown. Mental rotation of body parts shown from common perspectives (e.g., a hand shown from the dorsum perspective) is heavily affected by the orientation of the hand image, resulting in steep slopes for the associated RT profiles (Ionta et al., Citation2007). Conversely, mental rotation of images representing body parts from uncommon perspectives (e.g., a hand from the little finger side) is less influenced by image orientation, leading to almost flat RTs profiles (Ionta & Blanke, Citation2009). These variations of the shape of RT profiles are also considered signs that people use different cognitive strategies to perform mental rotation. A large influence of image orientation (steeper slopes) on the RT profile suggests that participants use their own body as the reference frame and therefore a somatosensory simulation strategy (Parsons, Citation1987; Sirigu & Duhamel, Citation2001). A weak dependence of RTs on image's orientation (more flat RT profiles) would indicate that people mentally manipulate images in a 3D space and therefore use a visuo-spatial simulation strategy (Conson et al., Citation2013; Devlin & Wilson, Citation2010; Ionta et al., Citation2012).
Such a strategy-dependent difference of RT profile is particularly evident in clinical settings. For instance, in patients with spinal cord injury the RT profile for mental rotation of images representing the disconnected body parts is weakly affected by image's orientation (Ionta et al., Citation2016) and can become more orientation-dependent after physiotherapy (Scandola et al., Citation2019). In other words, as a result of somatosensory deprivation (spinal cord injury), patients use a visual strategy to perform mental rotation of images that otherwise would trigger the use of a somatosensory strategy. However, together with the somatosensory restoration due to physiotherapy, patients can shift back to a somatosensory strategy. This is evidence that (1) it is possible to shift between cognitive strategies (somatosensory-to-visual) for mental rotation as a function of contextual factors (e.g., decrease of sensory input), and (2) that this shift can be independent of the nature of the image.
In sum, mental rotation of body parts can be performed with different strategies depending on the most available/reliable sensory input. In opposition to the situation caused by spinal cord injury (loss of somatosensation and therefore greater reliance on visual strategies), we hypothesized that the contrary (decrease in the visual input and therefore greater reliance on somatosensory strategies) may result in a shift from a visual to a somatosensory strategy for the mental rotation of images that typically would trigger a visual strategy (e.g., little finger-perspective hands). More specifically, such a shift would be reflected in different shapes of RT profiles for the mental rotation of visually degraded images (steeper slopes – somatosensory strategy) with respect to normal ones (more flat slopes – visual strategy), specifically for images typically associated with a visual strategy (little finger-perspective).
To this aim, we assessed the impact of lowering the visual input on the RT profile of mental rotation of hands. Twenty-nine healthy participants performed mental rotation of hand images shown from the dorsum or the little finger-perspectives, in two within-subject conditions of visibility: Degraded Vision, Baseline. Considering that in normal visual conditions (Baseline) the mental rotation of hands shown from the little finger-perspective is weakly affected by image orientation (visual strategy), we predicted that Degraded Vision would trigger the use of a somatosensory strategy (larger impact of image orientation on RT profiles, steeper slopes). This should determine that the RT profile for mental rotation of little finger images would be more influenced by the image orientation in Degraded Vision than Baseline.
Methods and results
Participants
An a-priori power analysis performed with the Statistica software (StatSoft Inc., Tulsa, USA) on previous unpublished pilot data recorded with a similar experimental protocol (not included in the sample of the present study) estimated that, with respect to normal visual input, the effect of degraded visual input should be statistically significant (effect size = 0.64; alpha = 0.05; power goal = 0.9) with a minimum of 28 participants/datasets. On this basis, after signing a written informed consent prior to the experiment, 29 participants (17 females) aged 18–31 years (M = 24.9 years, SD = 2.9) have been enrolled, one of which was excluded due to mental rotation performance at chance level (accuracy 52%), resulting in 28 definitive datasets. While the influence of gender on mental rotation is controversial (Guizzo et al., Citation2019; Sanchis-Segura et al., Citation2018), the impact of hand dominance has been well-documented (e.g., Ionta & Blanke, Citation2009). Therefore, both female and male participants were included in the study, but all of them were right-handed (Edinburgh Handedness Inventory; Oldfield, Citation1971). The local Ethics Committee approved the experiment, which was performed in accordance with the Declaration of Helsinki 2013.
Stimuli
The stimuli consisted of naturalistic pictures of one hand shown either from the dorsum of the little finger-perspective (). All pictures were black/white images and were oriented in one of four clockwise orientations from the fingers pointing upwards (0°, 90°, 180°, 270°). According to the two visual conditions, two classes of stimuli were prepared. In both conditions, the background of the image was the same (grey), but in Degraded Vision the contrast between the hand and the background was decreased by 60% with respect to the control vision condition (Baseline), when the images were presented with a normal contrast (). To avoid task habituation and/or automatic responses, the images varied in terms of laterality. Left-lateralized hands were mirror-reversed images of the right-lateralized ones. The two adopted perspectives showed the hand either from a top perspective (dorsum) or a lateral perspective (little finger) (). The overall configuration and visual features (gender, age, ethnicity, shape, size, etc.) of the two perspectives were the same. All images covered a visual angle comprised between 11° and 13° at a distance of 60cm.
Figure 1. Experimental stimuli. In the Baseline condition the hand images were presented with normal visibility with respect to the background (left panel). In the Degraded Vision condition, the visibility of the hand images with respect to the background was lowered by 60% (right panel). The background was the same in Degraded Vision and Baseline. Both Degraded and Baseline images varied in terms of Perspective (D = dorsum, LF = little finger) and were presented in one out of four orientations (0°, 90°, 180°, 270°). Beyond visibility, the overall configuration of Degraded and Baseline images was the same. Also hand laterality varied across images, but for illustrational purposes only left hands are represented in the figure.
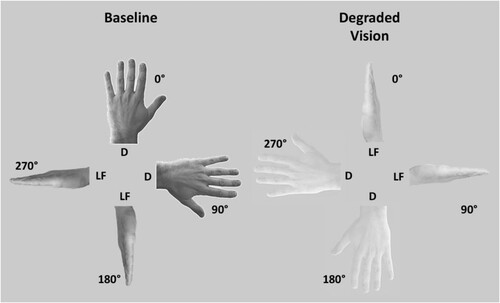
Procedure
Participants sat in front of a computer screen positioned at 60cm from their eyes, with the hands on their laps and hidden under the table (Ionta et al., Citation2010). A microphone was positioned in front of the participant. The presentation of images was controlled by the E-Prime software (Psychology Software Tools Inc., Pittsburgh USA). Before the experimental session, all participants practiced the task with a set of images different from the experimental ones (different orientations). During both the training and the actual experiment, participants were presented with one image at a time preceded by a fixation cross (1000ms). Then, the image appeared on the screen and remained visible until a response was given. For each image participants were asked to indicate whether the image represented a left or a right hand. To this aim, participants provided verbal responses (“left” or “right”) for each image, as quickly and accurately as possible. RT was defined as the time between the onset of the image and the participant’s verbal response. RT was recorded automatically with a microphone. Participants’ responses (left, right) were manually encoded. RTs and accuracy were analyzed offline. The experiment comprised one block, including both dorsum and little finger images presented in both the Degraded Vision and Baseline vision conditions. The order of vision conditions was counterbalanced across participants. Each block contained 96 images in total, varying in terms of visibility (Degraded, Baseline), laterality (left, right), perspective (dorsum, little finger), and orientation (0°, 90°, 180°, 270°), totalling 32 images, each randomly presented 3 times in the block.
Data analysis
Hand images presented with the fingers pointing upright were classified as “No” rotation (NO), while images with the fingers pointing downwards were classified as “Upside Down” rotations (UP/DN). Since mental rotation is faster for hand images oriented towards the participant’s midsagittal plane (Funk & Brugger, Citation2008; Ionta et al., Citation2013; Parsons, Citation1994), for both the dorsum and little finger-perspectives, right hands presented at 90° and left hands presented at 270° (clockwise from the upright) were defined as “Lateral” rotations (LAT), while right hands presented at 270° and left hands presented at 90° were defined as “Medial” rotations (MED). This resulted in classifying each mental rotation trial in one out four rotations (NO, MED, UP/DN, LAT).
Trials with incorrect responses were excluded from the analysis (7.5% of the trials) and only RTs of the remaining correct responses (92.5%) were analyzed (Zeugin et al., Citation2017) with a 3-way repeated measures ANOVA with Vision (Degraded, Baseline), Perspective (dorsum, little finger), and Rotation (NO, MED, UP/DN, LAT) as main factors. Absolute accuracy values (before the selection of only correct responses) were also entered in a 3-way ANOVA with the factors Vision, Perspective, and Rotation. Images’ laterality varied only to avoid habituation and its influence on mental rotation has been anyways repeatedly documented (e.g., Ionta et al., Citation2007, Citation2016). Therefore, the ANOVAs did not include laterality as a factor. The confidence interval for both ANOVAs was set at 95% (p = 0.05). The partial eta-squared (η2p) was used to establish the effect size of all significant main effects and interactions, with the confidence interval set at 90%, and the lower (CIlow) and upper (CIhigh) limits of such confidence interval calculated for each effect size. The significance of the post-hoc comparisons was corrected for multiple comparisons with the Tukey HSD test (p < 0.05). All statistical analyses were performed with the STATISTICA software (StatSoft Inc., Tulsa, USA).
Results
The ANOVA showed the significant main effect of Vision [F(1,26) = 15.1; p < 0.05; η2p = 0.37; CIlow = 0.12; CIhigh = 0.54] and interaction between Vision, Perspective, and Rotation [F(3,78) = 9.9; p < 0.05; η2p = 0.28; CIlow = 0.12; CIhigh = 0.38]. The main effect of Vision showed that participants were slower during Degraded Vision (2104.9ms) than Baseline (1701.8ms). The post-hoc comparisons of the interaction between Vision, Perspective, and Rotation showed that Degraded Vision has a different effect on the mental rotation of dorsum versus little finger images. Mental rotation of dorsum images was slower with respect to Baseline, but maintained the same rotation-dependent RT profile. Mental rotation of little finger images also was generally slower during Degraded Vision than Baseline but, importantly, it assumed a RT profile more strongly dependent on the degrees of rotation. For dorsum images, the difference between rotations was significant in both Degraded Vision and Baseline (Degraded Vision: NO = 1228.6ms, MED = 1492.3ms, UP/DN = 2072ms, LAT = 1567.8ms, all p < 0.012; Baseline: NO = 1683ms, MED = 2009.6ms, UP/DN = 2266.1ms, LAT = 1927.9, all p < 0.017). For little finger images the difference between rotations was significant only in Degraded Vision (Degraded Vision: NO = 2004.5ms, MED = 2289.9ms, UP/DN2568.1ms, LAT = 2090.2ms, all p < 0.004; Baseline: NO = 1743.6ms, MED = 1873,1ms, UP/DN = 1905.7ms, LAT1731.8ms, all p > 0.38) (). The absence of statistical significance for the corresponding 3-way interaction for the accuracy data (p = 0.54) excluded the possibility of a speed-accuracy trade off ().
Figure 2. Degraded Vision affects response time. Box and whiskers representations of the obtained data show that, with respect to Baseline, Degraded Vision slowed down mental rotation of both dorsum and little finger images, and additionally modified the shape of the response time profile for little finger images.
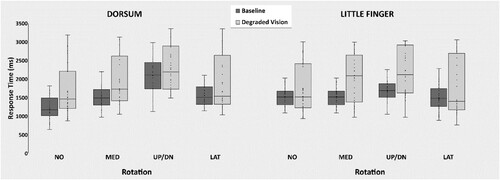
Figure 3. Vision-independent Accuracy. The lacking statistical significance of the 3-way interaction between Vision, Perspective, and Rotation, indicated the absence of a speed/accuracy trade off. Participants equally performed the mental rotation task in both vision conditions and types of hand images. Error bars represent standard errors.
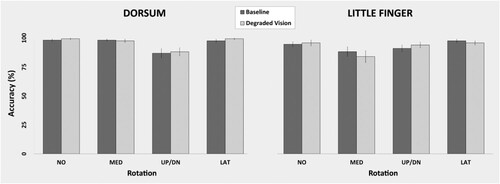
Additional Vision-unrelated effects on response time comprised the significant main effect of Perspective [F(1,26) = 47.4; p < 0.001; η2p = 0.65; CIlow = 0.42; CIhigh = 0.74], the main effect of Rotation [F(3,78) = 36.5; p < 0.001; η2p = 0.58; CIlow = 0.44; CIhigh = 0.65], and the 2-way interaction between Perspective and Rotation [F(3,78) = 5.7; p < 0.001; η2p = 0.17; CIlow = 0.04; CIhigh = 0.27]. The main effect of Perspective showed slower responses for little finger (2025.8ms) than dorsum images (1780.9ms). The main effect of Rotation showed that mental rotation of UP/DN images (2202.9ms) was the slowest, NO rotation was the fastest (1664.9ms), and MED (1916.2ms) and LAT rotations (1829.4ms) were in between NO and UP/DN. The interaction between Perspective and Rotation showed that, for the dorsum images, all the rotation-related differences were significant (NO = 1455.8ms, MED = 1750.9ms, UP/DN = 2169ms, LAT = 1747.8ms, all p < 0.001). Conversely, for the little finger images, only the difference between UP/DN and LAT images was significant (p < 0.001) (NO = 1874.1ms, MED = 2081.5ms, UP/DN 2236.9ms, LAT = 1910.1ms). Finally, the analysis of accuracy data (before removing the incorrect trials) showed the significant main effect of Rotation [F(3,78) = 7.6; p < 0.001; η2p = 0.23; CIlow = 0.08; CIhigh = 0.33] and interaction between Perspective and Rotation [F(3,78) = 4.9; p < 0.003; η2p = 0.15; CIlow = 0.03; CIhigh = 0.25]. The main effect of Perspective showed that participants were more accurate with LAT images (97.5%) with respect to MED (92.6%) and UP/DN images (90.7%, all p < 0.02). The Perspective by Rotation interaction indicated that the only significant difference between the two perspective was for MED images (Degraded = 87.4%, Baseline = 97.8%, p < 0.003). In general, these Vision-unrelated effects confirmed well-documented evidence from previous studies and will not be further discussed.
Discussion
Even if unconsciously, every day we repeatedly use motor imagery to mentally simulate the possible outcomes of our actions, fine-tune motor planning, and increase the chances of success. One experimental approach to investigate the characteristics of these motor simulations is mental rotation of body parts. The temporal profiles of mental rotation inform us about the type of strategy used (Conson et al., Citation2013; Devlin & Wilson, Citation2010) and depend on the quality of the sensory input (Scandola et al., Citation2019). The present study suggests that a decrease in the visual input facilitates a shift from a visuo-spatial to a somatosensory strategy for mental rotation of hands and, therefore, for the mental representation of the body. This stronger reliance on somatosensory aspects is line with the functional changes following visual impairments, including an augmented relative weigh of touch over vision (Lederman & Klatzky, Citation2009).
Vision or somatosensation?
We found that the Decreased Vision condition differentially affected the mental rotation of dorsum – versus little finger-perspective images. With respect to the relative Baseline, in Decreased Vision both dorsum and little finger images were mentally rotated more slowly. However, while for the dorsum images the shape of the RT-profiles was Rotation-dependent in both Baseline and Degraded Vision (suggesting the use of a somatosensory strategy), for the little finger images degrading the visibility of the images was associated with a change from Rotation-independent RT-profiles in Baseline (typically considered a sign of using a visuo-spatial strategy) to Rotation-dependent RT-profiles in Degraded Vision (suggesting the adoption of a somatosensory strategy). Such a differential influence of Rotation as a function of visibility and hand perspective supports the existence of selective and visibility-dependent cognitive strategies for the mental representation and manipulation of the body. The change to a Rotation-dependent RT profile of mental rotation of Degraded little finger images supports the possibility of a shift between these cognitive strategies. Typically, mental rotation of little finger-perspective hand images is weakly affected by image orientation (Ionta et al., Citation2007; Parsons, Citation1994; Petit et al., Citation2003). This is in line with the results of the present study that mental rotation of little finger images at Baseline was not affected by Rotation. Notably, in Degraded Vision the mental rotation of the same little finger-perspective hand images was more pronouncedly influenced by Rotation. Since between Degraded Vision and Baseline, the little finger images differed only in terms of visibility, while all the other visual aspects were the same (posture, gender, age, ethnicity, size, shape, etc.), we propose that the larger dependency on Rotation for the mental rotation of little finger images is associated to the Degraded Vision condition. This means that a decrease in the visibility of a hand image may trigger a shift between concurrent cognitive strategies used to mentally represent it. The specificity of the impact of visibility (little finger but not dorsum images) indicates a fine recalibration of mental body representations as a function of the available visual information, and is in line with seminal observations that mental rotation is sensitive to rotation also in blind people (Marmor & Zaback, Citation1976). Previous data showed that a large influence of Rotation posits the activation of a somatosensory strategy for mental rotation, while a small impact of Rotation supports the use of a visuo-spatial strategy (Conson et al., Citation2013; Devlin & Wilson, Citation2010). Our results show that mental rotation of little finger images was more sensitive to Rotation in the Decreased Vision than Baseline condition. This suggests that Decreased Vision triggered the use of a somatosensory strategy for the mental rotation of a type of images that typically is mentally rotated with a visuo-spatial strategy. Conversely, possibly because it facilitates the use of a somatosensory-based strategy, Decreased Vision did not impact the already Rotation-dependent shape of RT profiles for dorsum images (beyond an overall generalized slowdown). It is reasonable that this lack of influence is due to the fact that, in typical conditions, dorsum images are already mentally processed using somatosensation as the principal frame of reference (Rotation-dependent RT profile at Baseline), and therefore the somatosensory-tending effect of Degraded Vision sums up on an already present somatosensory-based body-related mental processing. In other words, steeper RTs slopes (larger impact of Rotation) suggest the use of a somatosensory strategy, while more flat RTs distributions (smaller influence of Rotation) suggest the use of visuo-spatial strategy. In this framework, it is possible to note that, specifically for little finger-perspective images, the RT profile for visually degraded images was steeper than that of visually normal images (). In particular, while at Baseline there was no RT difference between different Rotations (flat RT profile = visuo-spatial strategy), Degraded Vision was associated with slower RT for MED and UP/DN Rotation with respect to NO and LAT rotations (steep RT profile = somatosensory strategy). Such a difference was not present for dorsum-perspective images, possibly because mental processing of this hand perspective is already based on somatosensory strategies. It is worth noting that, however, Degraded Vision did not unspecifically affect mental rotation of dorsum images, since responses were slower than Baseline for all rotations except UP/DN. This finding supports that the slower mental rotation of dorsum images in Decreased Vision was not due to the mere visibility differences with respect to Baseline.
The impact of vision on body representation
Our interpretation of the vision-to-somatosensation shift is linked to the difference between the psychological constructs of body schema and body image (Gallagher, Citation1986). The traditional definition body schema refers to the online representation of the somatosensory aspects of the body (Head, Citation1920). In particular, the body schema represents the mental reference frame used to plan, monitor, and control body configurations and movements (Berlucchi & Aglioti, Citation1997) and representing the current body state based on somatosensory and motor information (Ghez et al., Citation1995; Sainburg et al., Citation1993). Conversely, the classic definition of body image focuses the pictorial aspects of the body (Schilder, Citation1935), relies on previous visual experience (Adame et al., Citation1989, Citation1991), and concerns the appearance of one’s own body from the outside (Gardner & Moncrieff, Citation1988). In normal conditions, body schema and body image are coherently integrated, but some clinical conditions (e.g., sensory deprivation) can dramatically affect their mutual exchange (Gallagher & Cole, Citation1995). However, a decrease in the sensory input, such as somatosensory loss, affects the relationship between body schema and body image, inducing a shift from a (body centred, first-person) somatosensory strategy to a (object-centred, third-person) visuo-spatial one (Scandola et al., Citation2019). At a similar logic, in the present study the inverse shift from body image to body schema for mental processing of little finger images could be due to insufficient visual information in Degraded Vision, which in turn could render the body image unreliable and promote the use of body schema. Indeed, the rotation-dependent increase of RTs for mental rotation of Degraded little finger images, suggests that this process shares at least some properties with manual actions (Zapparoli et al., Citation2014). The idea of the body image-to-schema shift is in line with previous evidence showing that blindness can trigger the use of alternative strategies for mental rotation (Ungar et al., Citation1995) and is associated with aberrant patterns of brain activity during mental rotation (Roder et al., Citation1997).
The use of the body schema as a more reliable frame of reference in case of low vision matches the idea of functional changes following or accompanying vision loss or deterioration. There is indeed a large body of evidence showing that decreased vision is associated with increased somatosensory sensitivity (e.g., Norman & Bartholomew, Citation2011) and that visually impaired people put in place alternative strategies in order to compensate the lack of visual input, including a stronger reliance on somatosensory information (Wan et al., Citation2010). Such perceptual modifications might drive or support the cognitive (Rovira et al., Citation2011) and behavioural (Ballesteros et al., Citation1997; Wijntjes et al., Citation2008) adaptations necessary to achieve goals at accuracy levels similar to typical visual conditions (Vinter et al., Citation2012). Our interpretation that a decrease in visual input can determine a shift from body image to body schema, further reflected in the stronger reliance on somatosensory information, is also supported by behavioural evidence that the relationship between vision and touch affects higher order cognitive processing (Ionta et al., Citation2013), as well as by brain imaging data showing that visual deficiencies are associated with somatosensory-dependent neuroplastic changes in brain areas typically implied in visual processing (Burton et al., Citation2002; Lacey & Sathian, Citation2014; Merabet et al., Citation2007).
Further considerations
It might be argued that the dorsum and little finger images have different intrinsic visual characteristics (e.g., shape or shading), that the impact of Degraded Vision on these characteristics is different between the two images (e.g., one image losing more relevant information than the other), and that these divergencies may explain the dissimilar mental rotation of the two images. However, we highlight that, with respect to both the shape and shading, both the dorsum and little finger image were asymmetrical, and that Degraded Vision kept the original shape and shading ratio in both images (brighter and darker parts remained distinct also in Degraded Vision). Noteworthily, even assuming that different weight of eventual changes in shape and/or shading could explain the difference between Baseline and Degraded Vision, this would not explain why between Degraded Vision and Baseline there were no RT differences at NO and LAT rotations of little finger-perspective images. If shape and/or shading would drive the performance in mental rotation, then some Degraded/Baseline differences should be significant at all Rotations, which is not the case. On this basis and considering the overall shape of RTs, we propose that the differences are due to a change in the cognitive strategy as a function of the sensory input.
It might be also argued that using different types of images, possibly not associated with somatosensory strategies such as inanimate objects, might affect the findings of the present study. We agree that additional manipulations may better specify or strengthen our findings, but we also feel that this should be addressed by series of future experiments, based on and following up the present one, which would constitute a first step into the investigation of the effects of varying visual input on mental rotation. Considering that even inanimate objects can be mentally rotated using a somatosensory strategy (Wexler et al., Citation1998; Wohlschläger & Wohlschläger, Citation1998), future studies should implement images of inanimate objects, but controlling their sensorimotor affordances (graspability, common use, manipulability, etc.), and possibly comparing more and less graspable, usable, manipulable objects. Future studies should not be limited to the investigation of inanimate objects, rather exploring the effects of degraded vision on a panel of possible variations, including the comparisons between corporal and non-corporal images [e.g., real vs. reproduced hands (wood)], partial and full bodily images (only hands vs. hands attached to the body), known and unknown objects, human vs. animal body parts, etc.
Conclusions
In the present study, degrading the visual input seemed to induce a vision-to-somatosensation shift in the frame of reference for mentally representing and manipulating specific parts of the body (little finger) typically associated with visuo-spatial reasoning. This indicates a change with respect to the Rotation-independent visuo-spatial strategy based on the body image, which typically would be expected for little finger-perspective images. Based on the present results it is possible to conclude that the visibility of a bodily image can affect the characteristics of its mental representation, eventually facilitating a shift from a pictorial to a somatosensory representation as a function of the available sensory input.
The impact of the present study is twofold. First, we provide a direct and within-subject comparison of the behavioural effects of modified visibility on the mental representation of the body. Second, such dependence on visibility is associated with a stronger reliance on the somatosensory or the visuo-spatial aspects of the mental representation of the body. Altogether this is evidence of the existence of differential visibility-dependent mechanisms previously only hypothesized, which in turn is particularly important in clinical settings where the degeneration/restoration of visual abilities might be associated with or facilitated by changes in the body representation. This might have important outcomes for understanding the nature of mental body representations in populations affected by visual deficits and for training and rehabilitation.
Disclosure statement
No potential conflict of interest was reported by the author(s).
Additional information
Funding
References
- Adame, D. D., Johnson, T. C., & Cole, S. P. (1989). Physical fitness, body image, and locus of control in college freshman men and women. Perceptual and Motor Skills, 68(2), 400–402. https://doi.org/10.2466/pms.1989.68.2.400
- Adame, D. D., Radell, S. A., Johnson, T. C., & Cole, S. P. (1991). Physical fitness, body image, and locus of control in college women dancers and nondancers. Perceptual and Motor Skills, 72(1), 91–95. https://doi.org/10.2466/pms.1991.72.1.91
- Ballesteros, S., Manga, D., & Reales, J. M. (1997). Haptic discrimination of bilateral symmetry in 2-dimensional and 3-dimensional unfamiliar displays. Perception & Psychophysics, 59(1), 37–50. https://doi.org/10.3758/BF03206846
- Berlucchi, G., & Aglioti, S. (1997). The body in the brain: Neural bases of corporeal awareness. Trends in Neurosciences, 20(12), 560–564. https://doi.org/10.1016/S0166-2236(97)01136-3
- Burton, H., Snyder, A. Z., Conturo, T. E., Akbudak, E., Ollinger, J. M., & Raichle, M. E. (2002). Adaptive changes in early and late blind: A fMRI study of Braille Reading. Journal of Neurophysiology, 87(1), 589–607. https://doi.org/10.1152/jn.00285.2001
- Conson, M., Mazzarella, E., & Trojano, L. (2013). Developmental changes of the biomechanical effect in motor imagery. Experimental Brain Research, 226(3), 441–449. https://doi.org/10.1007/s00221-013-3456-x
- Devlin, A. L., & Wilson, P. H. (2010). Adult age differences in the ability to mentally transform object and body stimuli. Aging, Neuropsychology, and Cognition, 17(6), 709–729. https://doi.org/10.1080/13825585.2010.510554
- Funk, M., & Brugger, P. (2008). Mental rotation of congenitally absent hands. Journal of the International Neuropsychological Society, 14(1), 81–89.
- Gallagher, S. (1986). Body image and body schema: A conceptual clarification. Journal of Mind and Behavior, 7, 541–554.
- Gallagher, S., & Cole, J. (1995). Body image and body schema in a deafferented subject. Journal of Mind and Behaviour, 16, 369–390.
- Gardner, R. M., & Moncrieff, C. (1988). Body image distortion in anorexics as a non-sensory phenomenon: A signal detection approach. Journal of Clinical Psychology, 44(2), 101–107. https://doi.org/10.1002/1097-4679(198803)44:2<101::AID-JCLP2270440203>3.0.CO;2-U
- Ghez, C., Gordon, J., & Ghilardi, M. F. (1995). Impairments of reaching movements in patients without proprioception. II. Effects of visual information on accuracy. Journal of Neurophysiology, 73(1), 361–372. http://www.ncbi.nlm.nih.gov/pubmed/7714578
- Guizzo, F., Moe, A., Cadinu, M., & Bertolli, C. (2019). The role of implicit gender spatial stereotyping in mental rotation performance. Acta Psychologica, 194, 63–68. https://doi.org/10.1016/j.actpsy.2019.01.013
- Head, H. (1920). Studies in neurology II. Oxford University Press.
- Ionta, S., & Blanke, O. (2009). Differential influence of hands posture on mental rotation of hands and feet in left and right handers. Experimental Brain Research, 195(2), 207–217. https://doi.org/10.1007/s00221-009-1770-0
- Ionta, S., Fourkas, A. D., & Aglioti, S. M. (2010). Egocentric and object-based transformations in the laterality judgement of human and animal faces and of non-corporeal objects. Behavioural Brain Research, 207(2), 452–457. https://doi.org/10.1016/j.bbr.2009.10.037
- Ionta, S., Fourkas, A. D., Fiorio, M., & Aglioti, S. M. (2007). The influence of hands posture on mental rotation of hands and feet. Experimental Brain Research, 183(1), 1–7. https://doi.org/10.1007/s00221-007-1020-2
- Ionta, S., Perruchoud, D., Draganski, B., & Blanke, O. (2012). Body context and posture affect mental imagery of hands. PLoS One, 7(3), e34382. https://doi.org/10.1371/journal.pone.0034382
- Ionta, S., Sforza, A., Funato, M., & Blanke, O. (2013). Anatomically plausible illusory posture affects mental rotation of body parts. Cognitive, Affective, & Behavioral Neuroscience, 13(1), 197–209. https://doi.org/10.3758/s13415-012-0120-z
- Ionta, S., Villiger, M., Jutzeler, C. R., Freund, P., Curt, A., & Gassert, R. (2016). Spinal cord injury affects the interplay between visual and sensorimotor representations of the body. Scientific Reports, 6(1), 20144. https://doi.org/10.1038/srep20144
- Lacey, S., & Sathian, K. (2014). Visuo-haptic multisensory object recognition, categorization, and representation. Frontiers in Psychology, 5, 730. https://doi.org/10.3389/fpsyg.2014.00730
- Lederman, S. J., & Klatzky, R. L. (2009). Haptic perception: A tutorial. Attention, Perception & Psychophysics, 71(7), 1439–1459. https://doi.org/10.3758/APP.71.7.1439
- Marmor, G. S., & Zaback, L. A. (1976). Mental rotation by the blind: Does mental rotation depend on visual imagery? Journal of Experimental Psychology: Human Perception and Performance, 2(4), 515–521. https://doi.org/10.1037/0096-1523.2.4.515
- Merabet, L. B., Swisher, J. D., McMains, S. A., Halko, M. A., Amedi, A., Pascual-Leone, A., & Somers, D. C. (2007). Combined activation and deactivation of visual cortex during tactile sensory processing. Journal of Neurophysiology, 97(2), 1633–1641. https://doi.org/10.1152/jn.00806.2006
- Munzert, J., Lorey, B., & Zentgraf, K. (2009). Cognitive motor processes: The role of motor imagery in the study of motor representations. Brain Research Reviews, 60(2), 306–326. https://doi.org/10.1016/j.brainresrev.2008.12.024
- Norman, J. F., & Bartholomew, A. N. (2011). Blindness enhances tactile acuity and haptic 3-D shape discrimination. Attention, Perception, & Psychophysics, 73(7), 2323–2331. https://doi.org/10.3758/s13414-011-0160-4
- Oldfield, R. C. (1971). The assessment and analysis of handedness: The Edinburgh inventory. Neuropsychologia, 9(1), 97–113. https://doi.org/10.1016/0028-3932(71)90067-4
- Parsons, L. M. (1987). Imagined spatial transformations of one's hands and feet. Cognitive Psychology, 19(2), 178–241. https://doi.org/10.1016/0010-0285(87)90011-9
- Parsons, L. M. (1994). Temporal and kinematic properties of motor behavior reflected in mentally simulated action. Journal of Experimental Psychology: Human Perception and Performance, 20(4), 709–730. https://doi.org/10.1037/0096-1523.20.4.709
- Perruchoud, D., Michels, L., Piccirelli, M., Gassert, R., & Ionta, S. (2016). Differential neural encoding of sensorimotor and visual body representations. Scientific Reports, 6(1), 37259. https://doi.org/10.1038/srep37259
- Petit, L. S., Pegna, A. J., Mayer, E., & Hauert, C. A. (2003). Representation of anatomical constraints in motor imagery: Mental rotation of a body segment. Brain and Cognition, 51(1), 95–101. https://doi.org/10.1016/S0278-2626(02)00526-2
- Roder, B., Rosler, F., & Hennighausen, E. (1997). Different cortical activation patterns in blind and sighted humans during encoding and transformation of haptic images. Psychophysiology, 34(3), 292–307. https://doi.org/10.1111/j.1469-8986.1997.tb02400.x
- Rovira, K., Deschamps, L., & Baena-Gomez, D. (2011). Mental rotation in blind and sighted adolescents: The effects of haptic strategies. European Review of Applied Psychology, 61(3), 153–160. https://doi.org/10.1016/j.erap.2011.05.001
- Sainburg, R. L., Poizner, H., & Ghez, C. (1993). Loss of proprioception produces deficits in interjoint coordination. Journal of Neurophysiology, 70(5), 2136–2147. https://doi.org/10.1152/jn.1993.70.5.2136
- Sanchis-Segura, C., Aguirre, N., Cruz-Gomez, A. J., Solozano, N., & Forn, C. (2018). Do gender-related stereotypes affect spatial performance? Exploring when, How and to whom using a chronometric Two-choice mental rotation task. Frontiers in Psychology, 9, 1261. https://doi.org/10.3389/fpsyg.2018.01261
- Scandola, M., Dodoni, L., Lazzeri, G., Arcangeli, C. A., Avesani, R., Moro, V., & Ionta, S. (2019). Neurocognitive benefits of physiotherapy for spinal cord injury. Journal of Neurotrauma, 36(12), 2028–2035. https://doi.org/10.1089/neu.2018.6123
- Schilder, P. (1935). The image and appearance of the human body. International Universities Press.
- Sirigu, A., & Duhamel, J. R. (2001). Motor and visual imagery as two complementary but neurally dissociable mental processes. Journal of Cognitive Neuroscience, 13(7), 910–919. https://doi.org/10.1162/089892901753165827
- Ungar, S., Blades, M., & Spencer, C. (1995). Mental rotation of a tactile layout by young visually impaired children. Perception, 24(8), 891–900. https://doi.org/10.1068/p240891
- Vinter, A., Fernandes, V., Orlandi, O., & Morgan, P. (2012). Exploratory procedures of tactile images in visually impaired and blindfolded sighted children: How they relate to their consequent performance in drawing. Research in Developmental Disabilities, 33(6), 1819–1831. https://doi.org/10.1016/j.ridd.2012.05.001
- Wan, C. Y., Wood, A. G., Reutens, D. C., & Wilson, S. J. (2010). Congenital blindness leads to enhanced vibrotactile perception. Neuropsychologia, 48(2), 631–635. https://doi.org/10.1016/j.neuropsychologia.2009.10.001
- Wexler, M., Kosslyn, S. M., & Berthoz, A. (1998). Motor processes in mental rotation. Cognition, 68(1), 77–94. https://doi.org/10.1016/S0010-0277(98)00032-8
- Wijntjes, M. W., Van Lienen, T., Verstijnen, I. M., & Kappers, A. M. (2008). Look what I have felt: Unidentified haptic line drawings are identified after sketching. Acta Psychologica, 128(2), 255–263. https://doi.org/10.1016/j.actpsy.2008.01.006
- Wohlschläger, A., & Wohlschläger, A. (1998). Mental and manual rotation. Journal of Experimental Psychology: Human Perception and Performance, 24(2), 397–412. https://doi.org/10.1037/0096-1523.24.2.397
- Zapparoli, L., Invernizzi, P., Gandola, M., Berlingeri, M., De Santis, A., Zerbi, A., Banfi, G., & Paulesu, E. (2014). Like the back of the (right) hand? A new fMRI look on the hand laterality task. Experimental Brain Research, 232(12), 3873–3895. https://doi.org/10.1007/s00221-014-4065-z
- Zeugin, D., Arfa, N., Notter, M., Murray, M. M., & Ionta, S. (2017). Implicit self-other discrimination affects the interplay between multisensory affordances of mental representations of faces. Behavioural Brain Research, 333, 282–285. https://doi.org/10.1016/j.bbr.2017.06.044