Abstract
Objectives: Reactive oxygen species (ROS), including superoxide (O2•−), play an important role in the biological effects of ionizing radiation. The human body has developed different antioxidant systems to defend against excessive levels of ROS. The aim of the present study is to investigate the redox status changes in the blood of radiologic technologists and compare these changes to control individuals.
Methods: We enrolled 60 medical workers: 20 occupationally exposed to ionizing radiation (all radiologic technologists), divided in three subgroups: conventional radiography (CR), computerized tomography (CT), and interventional radiography (IR) and 40 age- and gender-matched unexposed controls. Levels of O2•− and malondialdehyde (MDA) in blood were measured as an index of redox status, as were the activities of antioxidant enzymes superoxide dismutase (SOD) and catalase. Redox status was also assessed by measuring levels of reduced and oxidized glutathione (GSH, GSSG, respectively).
Results: Levels of O2•− and MDA, and SOD activity in the blood of IR and CT-exposed subjects were significantly higher than both the CR-exposed subjects and control individuals. However, there were no statistically significant differences in the levels of catalase, GSH and ratio of GSH/GSSG between exposed workers and control individuals.
Discussion: This study suggests that healthcare workers in CT and IR occupationally exposed to radiation have an elevated circulating redox status as compared to unexposed individuals.
Introduction
It has been shown that exposure of eukaryotic cells to ionizing radiation results in the immediate formation of reactive oxygen species (ROS) through the radiolysis of water, an indirect effect of radiation.Citation1,Citation2 This rapid increase in ROS induces oxidative stress damage to cellular biomolecules such as lipids, proteins, and DNA. Radiation-induced ROS include O2•−, hydrogen peroxide (H2O2) and hydroxyl radical (OH•).Citation3 Cells have several enzymatic antioxidants that detoxify ROS and protect the cell from oxidative damage such as superoxide dismutase (SOD), catalase, and glutathione peroxidase (GPx) as well as non-enzymatic antioxidants, such as glutathione (GSH). SOD catalyzes the dismutation of O2•− to H2O2,Citation4,Citation5 which is then decomposed into water and oxygen by catalase and/or GPx.Citation6,Citation7 GPx requires GSH for reducing hydroperoxides. In addition, GSH is well-accepted to be the primary intracellular small molecule reducing agent to protect cells against oxidative stress.Citation8,Citation9 In healthy subjects, a balance between ROS formation and antioxidant capacity exists. However, when this balance is disturbed as a result of disease process or long-term exposure to ionizing radiation, it can lead to oxidative stress.Citation10–Citation12 Chronic oxidative stress contributes to pathological effects including inflammation, fibrosis, and necrosis,Citation1 as well as DNA damage and cancer.Citation13–Citation15 Studies suggest that cumulative professional radiological exposure is associated with a non-negligible lifetime risk of cancer for the most exposed radiology staff.Citation16
Previous studies have suggested changes in the antioxidant status and lipid peroxidation in the blood of radiology workers.Citation17–Citation21 However, the results remain complicated and controversial. The specific ROS driving the oxidative stress in workers exposed to long-term low level of ionizing radiation is currently unknown and how these effects change with different occupational settings in radiography remains to be elucidated. In the present study, we assessed the circulating redox status of radiologic technologists exposed to long-term low level ionizing radiation in different occupational settings including: conventional radiography (CR), interventional radiography (IR), and computerized tomography (CT). More specifically, we measured SOD and catalase activity, the ratio of GSH to oxidized glutathione (GSSG), and levels of O2•− and lipid peroxidation products in blood from these individuals.
Materials and methods
Subjects
Our investigation comprised 60 healthy medical workers: 20 occupationally exposed to ionizing radiation (all radiologic technologists, age = 39.4 ± 2.19 years), divided in three subgroups CR (n = 12), IR (n = 4), and CT (n = 4), and 40 age- and gender-matched unexposed control individuals (Table ). Lifetime radiation effective dose accumulated by occupation exposure over years was obtained from the official personal dose detector calculated from personal equivalent thermoluminescent dosimetry (TLD), following recommendations of the National Council of Radiation ProtectionCitation22 (expressed in millisieverts (mSV)) (Table ). All subjects are from Nebraska Medicine and were in good health, were not presently taking medications, and completed a detailed questionnaire concerning their habits, lifestyles and medical records (age, medications, smoking, drinking habits, X-ray exposure as a patient, exercise). The Leisure Score Index of the Godin-Leisure-Time Exercise Questionnaire (GLTEQ) was used to determine physical activity participation.Citation23 The GLTEQ contains three questions that assess the frequency of mild, moderate, and strenuous exercise for at least 15 minutes during a typical week. A total score is calculated in arbitrary units by weighting each frequency by an estimated intensity guided by metabolic equivalents using the equation (9 × strenuous) + (5 × moderate) + (3 × light). An additional question asked how often the participants engaged in any regular activity long enough to work up a sweat or make their heart beat rapidly in a 7-day period. Answer options were often, sometimes, or never/rarely. Persons with habits like smoking, tobacco chewing, or being pregnant were excluded from the study. Individuals with a history of tuberculosis, autoimmune disease such as rheumatoid arthritis and diabetes mellitus, all of which play a vital role in contributing to oxidative stress injury were also excluded from the study.
Table 1 Characteristics of the study subjects
Table 2 Lifetime effective radiation doses (mSv)
Blood sampling
Blood samples were collected from all subjects into EDTA tubes. Red blood cells were separated from plasma by centrifugation at 2500 × g at 4°C for 5 minutes and washed three times with PBS to remove the white buffy coat. The plasma and erythrocytes samples were then stored at −80°C until analyzed.
Measurement of O2•− generation
Immediately after sample collection, 100 μl of whole blood was incubated at 37°C with a O2•−-sensitive electron paramagnetic resonance (EPR) spin probe, 1-hydroxy-3-methoxycarbonyl-2,2,5,5-tetramethylpyrrolidine (CMH) for 60 minutes then frozen in liquid nitrogen. Superoxide levels were determined using a Bruker eScan EPR spectrometer and expressed as EPR amplitude arbitrary units (a.u.). The following EPR spectrometer settings were used: field sweep width, 100.0 G; microwave frequency, 9.75 kHz; microwave power, 1.10 mW; modulation amplitude, 5.94 G; conversion time, 10.24 ms; time constant, 40.96 ms.
Erythrocytes SOD, catalase activity, GSH assays, and plasma MDA levels
Activity of antioxidant enzymes in erythrocytes was measured using the OxiSelect Catalase Activity Assay Kit (Cell Biolabs, Inc., San Diego, CA, USA) for catalase, GSSG/GSH Quantification kit (DOJINDO, Inc. Rockville, MD, USA) for total, oxidized, and reduced GSH, and SOD Assay Kit-WST (DOJINDO, Inc) to measure intracellular SOD activity according to the manufacturers' guidelines. MDA levels were determined in plasma using the TBARS assay kit (Cayman Chemical, Inc., Ann Arbor, MI, USA).
Statistical analysis
All data are expressed as the mean ± SEM. Statistical analysis was done using GraphPad Prism version 5 for Windows (GraphPad Software, San Diego, CA, USA). Differences between three or more means were determined using one-way analysis of variance with Bonferroni post hoc tests. Correlations between variables were calculated by Pearson's correlation test. All statistical analyses were considered significant at P < 0.05.
Results
Demographic characteristics of the study subjects are shown in Table . Radiation-exposed and control individuals had comparable baseline characteristics, including age, sex, alcohol consumption, dietary supplements, and exercise. None of study subjects were smokers. The results of personal dosimetry of the three subgroups of exposed workers were analyzed and used to estimate mean doses. Mean lifetime effective radiation doses are presented in Table , from the least, second most and most radiation dose in CR-, IR-, and CT-exposed workers, respectively.
Radiation-exposed workers showed a significant increase in O2•− compared with the control individuals (Fig. B) with marked differences among the occupational setting subgroups, as shown in the representative EPR spectrum (Fig. A) and summary data (Fig. C). A significant difference was found between IR and CT versus control individuals and CR-exposed workers (P < 0.05), while no significant differences were found between CR versus control individuals and IR versus CT (P > 0.05).
Figure 1 Representative EPR spectroscopy spectrum obtained from blood collected from control, CR-, IR-, and CT-exposed workers, and incubated with the O2•−-sensitive spin probe, CMH (A). The EPR spectrum amplitude is directly proportional to the levels of O2•− in the sample. Summary data showing EPR spectrum amplitude in control individuals and all radiation-exposed workers (B) and the specific radiation-exposed subgroups (CR, IR, and CT) (C). a.u. = arbitrary unit. Data represent the mean ± SEM. *P < 0.05 versus control and CR.
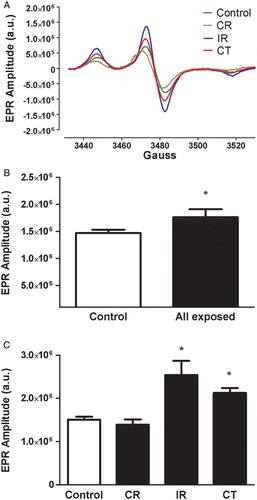
Plasma MDA levels were significantly higher in all exposed workers when compared with control individuals (Fig. A, P < 0.05). Taking a closer examination of the occupational setting subgroups, we observed a significant difference between the control individuals and the IR and CT subgroups (Fig. B, P < 0.05). IR-exposed workers also displayed significantly elevated levels of MDA compared to CR-exposed workers (P < 0.05). However, there was no significant difference between the IR and CT subgroups or the control individuals and CR-exposed workers (P > 0.05).
Figure 2 Plasma MDA levels in control individuals as well as in all radiation-exposed workers (A) and in the different occupational subgroups (CR, IR, and CT) (B). Data represent the mean ± SEM. *P < 0.05 versus controls; ψP < 0.05 versus CR.
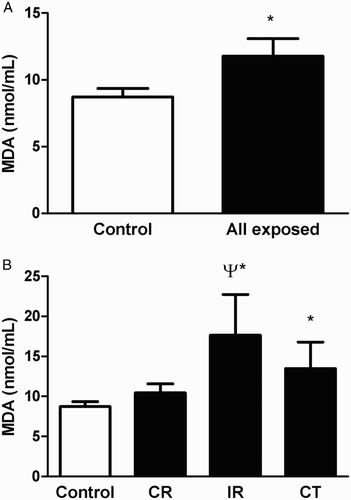
Owing to elevated levels of O2•− and MDA, it would be expected to observe a difference in antioxidant levels in the exposed versus control individuals. Enzymatic and non-enzymatic pathways exist in human red blood cells to scavenge and detoxify ROS, such as SOD/catalase and GSH.Citation24 As shown in Fig. A, SOD activity increased significantly in all exposed workers as compared to control individuals (P < 0.05), and particularly in IR and CT subgroups versus control individuals and CR-exposed workers (P < 0.05, Fig. B). Similar to changes in O2•−, there were no significant differences between CR and control individuals (P > 0.05, Fig. B). However, as shown in Fig. A and B, there were no significant differences in catalase activity or the GSH/GSSG ratio in all exposed workers and control individuals.
Figure 3 SOD activity in erythrocytes isolated from all radiation-exposed workers and control individuals (A), and in the different occupational subgroups (CR, IR, and CT) (B). The levels expressed are mean values ± SEM. *P < 0.05 versus control & CR; ψP < 0.05 versus IR.
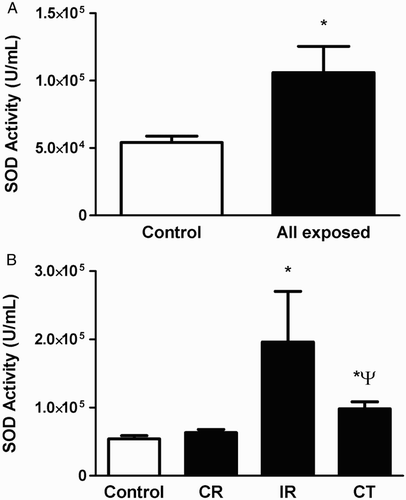
Figure 4 Catalase activity (A) and the GSH/GSSB ratio (B) in erythrocytes isolated from all radiation-exposed workers and control individuals. The levels expressed are mean values ± SEM. P > 0.05.
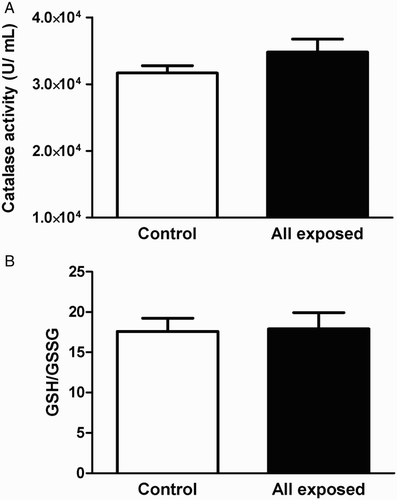
Levels of O2•− as well as changes in the antioxidant levels of SOD, catalase, and GSH/GSSG ratio were positively correlated with the lifetime effective radiation dose as well the years of occupational radiation exposure. However, MDA levels were not correlated with the low-dose range or years of occupational radiation exposure.
Discussion
In our study, radiation-exposed workers had an average annual effective dose of radiation of 2.03 mSv, which is below the maximal allowable limits set by the International Commission of Radiation Protection (ICRP).Citation25 Low doses of radiation (i.e. less than 50 mSv) to which radiation-exposed workers are subject is generally not high enough to cause deterministic (i.e. direct) damage such as skin erythema/burns. However, an increased incidence of certain types of neoplasms, including leukemia and multiple myeloma or solid cancers, has been reported.Citation26 Although the mechanism of these phenomena is complex, the production of ROS triggered by ionizing radiation appears to play a central role. ROS may damage cell structures and, most importantly, DNACitation27–Citation29 as well as result in pathological health effects including inflammation, fibrosis, necrosis,Citation1 and cancer.Citation13–Citation15 Chronic exposure to low doses of radiation may lead to systemic oxidative stress, as suggested by the increased level of MDA and circulating O2•− in high-dose radiologic procedures (i.e. IR and CT) as compared to low-dose radiologic procedures, such as CR. Oxidative stress occurs as a consequence of an alteration in the equilibrium between the production and removal of ROS.Citation30 ROS include non-organic molecules, such as O2•−, H2O2, and OH•, as well as organic molecules, such as alkoxyl and peroxyl radicals. ROS are continuously generated during oxidative metabolism. In order to avoid damage caused by ROS, such as lipid peroxidation, protein modification, and DNA strand breaks, mechanisms exist which remove ROS or prevent the generation of ROS.Citation31,Citation32 In our current study, we examined the circulating redox status in radiologic technologists in different occupational settings (i.e. CR, CT, and IR) by measuring O2•− levels in blood using the gold standard for detecting O2•−, that is, EPR spectroscopy.Citation33 Our data reveal that in IR- and CT-exposed workers, long-term exposure to low level ionizing radiation is associated with an altered redox balance mirrored by an increase in O2•−.
MDA, a product of lipid peroxidation which is the oxidation of polyunsaturated fatty acids in membranes induced by free radicals, is a biomarker of oxidative damage. Many studies have examined the potential link between lipid peroxidation and radiation.Citation34–Citation36 In the present study, we found MDA levels to be significantly higher in the IR- and CT-exposed workers compared to control individuals and CR-exposed workers. These data are consistent with previous reports indicating MDA is elevated in subjects exposed to ionizing radiation. For example, Malekirad et al.Citation19 found that MDA levels are increased in the blood of hospital workers, especially radiology staff, due to chronic exposure to low-dose ionizing radiation. Further, Arterbery et al.Citation37 and Clemens et al.Citation38 observed that after whole-body radiation, lipid peroxidation is increased in animals and humans. Our present study extends these and other observations by reporting an increase in MDA levels in specific subgroups of radiologic technologists, particularly in those technologists working in the IR and CT occupational setting. Cell membrane damage induced by lipid peroxidation is the molecular basis for disturbance of signal transduction, gene expression, and regulation of cell functions involved in apoptosis, adaptation, and/or genomic instability which are closely correlated to carcinogenesis.Citation39,Citation40 The carcinogenic risk induced by low doses of ionizing radiation is controversial. The linear-non-threshold (LNT) model which implies proportionality between dose and cancer risk was introduced as a concept to facilitate radiation protection.Citation41 But the use of this model led to the claim that even the smallest dose may initiate carcinogenesis –for instance, from diagnostic X-ray sources.Citation42,Citation43 Interestingly, our results demonstrate the presence of a threshold dose at 17 mSv (CR) for radiation health effect as evidenced by no changes in the levels of O2•− or MDA between the CR and control individuals. Moreover, it showed that the response increases above the threshold dose and the lack of linearity of the response was observed above 31 mSv (IR) as evidenced by no significant differences in the levels of O2•− and MDA between the IR and CT subgroups. This observation is incompatible with the LNT model and should be interpreted with caution.
In addition to measuring levels of ROS (i.e. O2•−) and lipid peroxidation (i.e. MDA), we also examined the activity of endogenous antioxidants enzymes (e.g. SOD, catalase) in radiology technologists. Of particular interest, we report an increase in the activity of SOD, a primary antioxidant in red blood cells that catalyzes the dismutation of O2•− to H2O2, in radiation-exposed IR and CT workers compared to control individuals and CR-exposed workers. Our study demonstrates a physiological adaptive response, that is, an enhanced antioxidant defense (increased level in SOD activity) as a compensatory protective mechanism triggered by low-dose exposure and elevated levels of O2•−. However, elevated levels of SOD may increase the rate of H2O2 production that can contribute to oxidative damage of cell membrane as evidenced by an increase in MDA. Although these data are in accordance with others,Citation17 it should be noted that other previous studies have reported decreased SOD activity in workers occupationally exposed to low-dose radiation.Citation18,Citation21 The discrepancy between our data and others can potentially be explained by taking a closer look at the specific subgroups of radiation-exposed workers examined in the various studies. For example, in our study we enrolled IR-, CT-, and CR-exposed workers, while other studies have examined different groups of individuals occupationally exposed to low-dose radiation, such as interventional cardiologists.Citation21
Considering the increase in O2•− and SOD activity we observed in radiologic technologists in the IR and CT occupational settings, it is tempting to speculate that levels of H2O2 would also be elevated as H2O2 is the product of SOD-catalyzed dismutation of O2•−. Although we did not directly measure H2O2 levels in the present study, we did measure catalase activity and GSH. Intracellular catalase provides protection against elevated levels of H2O2 by directly scavenging H2O2 to oxygen and water.Citation44 Glutathione is considered to be an important factor in cellular defense against oxidative stress induced by radiation and drug resistanceCitation45 by acting as an intracellular reductant and by serving as a cofactor for the family of GPX which scavenge peroxides, including H2O2.Citation8 In this study, the ratio of reduced to oxidized glutathione (GSH/GSSG) was unchanged between all radiation-exposed workers and control individuals. These data are supported by previous studies.Citation17,Citation20 Catalase activity was also unchanged in radiation-exposed workers compared to control individuals. Interestingly, conflicting results have been reported concerning the effects of radiation on catalase activity with increased,Citation46 decreased,Citation18 and unchangedCitation20,Citation21 activities being reported. Similar to the discrepancy in the literature regarding changes in SOD activity, these differences in catalase activity may be dependent on the specific group of radiation-exposed workers examined in the various studies. A second explanation for these conflicting results is that the doses of radiation workers are exposed to is likely different depending on radiological procedures being performed between different institutions, which can differ substantially in terms of the equipment and protocols used, and the duration of radiation exposure.
In the present study, we controlled for various confounding factors that could influence the levels of circulating redox status by comparing matched study groups for age, alcohol consumption, and exercise. None of the study subjects were smokers. As shown in Table , there were no significant interaction between those confounding factors and the study groups, indicating that any changes in the levels of circulating redox status due to these confounding factors are occurring in both study groups. Our results indicate that exposure to radiation even within limits recommended by the ICRP appears to result in an altered redox balance evidenced by an increase in O2•− and lipid peroxidation. The increase in these oxidants is accompanied by an increase in SOD activity. These observations were particularly evident in radiologic technologists working with high-dose radiologic procedures such as CT and IR as compared to low-dose radiologic procedures, such as CR and control individuals. From these results, it is apparent that age, exercise, and alcohol consumption are probably not contributing to the significant increase in the levels of these circulating redox status. From these comparisons and the statistically significant differences, we believe that the changes in the circulating redox status observed are caused, in large part, by long-term exposure to low-level ionizing radiation and not by differences due to sampling. Although this pilot study provides insight and brings awareness to radiologic technologists in CT and IR for improving occupational protection, it must be noted that a limitation of the current study is the small sample size and the potential negligence of the radiation-exposed workers in wearing their TLDs, which may represent a source of significant underestimation of calculated radiation exposure and risk. In fact, we speculate that the lack of statistical significance in the lifetime effective dose of radiation received by CR versus IR and CT workers (Table ) is, at least in part, due to these limitations. Additional studies with increased numbers of CR-, IR-, and CT-exposed workers in which wearing of their TLDs can be controlled and the effective dose of radiation can be more accurately determined are warranted.
Disclaimer statements
Contributors All authors contributed equally.
Funding None.
Conflicts of interest Authors declare no conflicts of interest.
Ethics approval The present study was reviewed and approved by the Institutional Review Board of University of Nebraska Medical Center (Protocol No. 222-14-EP), and written informed consent was obtained from each participant.
Acknowledgements
This work is supported in part by the Charles R. O'Malley Charitable Lead Trust. EPR Spectroscopy data were collected in the University of Nebraska's EPR Spectroscopy Core, which is supported, in part, by a grant from the National Institute of General Medical Sciences of the National Institutes of Health (P30GM103335) awarded to the University of Nebraska's Redox Biology Center. The research team would like to thank the clinical research centre staff and workers at Nebraska Medicine.
References
- Spitz DR, Azzam EI, Li JJ, Gius D. Metabolic oxidation/reduction reactions and cellular responses to ionizing radiation: a unifying concept in stress response biology. Cancer Metastasis Rev 2004;23(3–4):311–22.
- Uma Devi P, Nagarathnam A, Satish Rao BS. Introduction to radiation biology. New Delhi: Churchill Livingstone; 2000.
- Riley PA. Free radicals in biology: oxidative stress and the effects of ionizing radiation. Int J Radiat Biol 1994;65(1):27–33.
- McCord JM, Fridovich I. Superoxide dismutase: an enzymic function for erythrocuprein (hemocuprein). J Biol Chem 1969;244(22):6049–55.
- Oberley LW. Anticancer therapy by overexpression of superoxide dismutase. Antioxid Redox Signal 2001;3(3):461–72.
- Aebi H. Catalase in vitro. Methods Enzymol 1984;105:121–6.
- Kono Y, Fridovich I. Superoxide radical inhibits catalase. J Biol Chem 1982;257(10):5751–4.
- Shimizu T, Iwanaga M, Yasunaga A, Urata Y, Goto S, Shibata S, et al. Protective role of glutathione synthesis on radiation-induced DNA damage in rabbit brain. Cell Mol Neurobiol 1998;18(3):299–310.
- Bier H, Hoffmann T, Eickelmann P, Hafner D. Chemosensitivity of head and neck squamous carcinoma cell lines is not primarily correlated with glutathione level but is modified by glutathione depletion. J Cancer Res Clin Oncol 1996;122(11):653–8.
- Chakraborty S, Singh OP, Dasgupta A, Mandal N, Das HN. Correlation between lipid peroxidation-induced TBARS level and disease severity in obsessive–compulsive disorder. Prog Neuro-Psychopharmacol Biol Psychiatry 2009;33(2):363–6.
- Hagelstrom AH, Goria NB, Larripa IB. Chromosomal damage in workers occupationally exposed to chronic low level ionizing radiation. Toxicol Lett 1995;76(2):113–7.
- de Zwart LL, Meerman JH, Commandeur JN, Vermeulen NP. Biomarkers of free radical damage applications in experimental animals and in humans. Free Radic Biol Med 1999;26(1–2):202–26.
- Zakeri F, Hirobe T. A cytogenetic approach to the effects of low levels of ionizing radiations on occupationally exposed individuals. Eur J Radiol 2010;73(1):191–5.
- Jacob P, Rühm W, Walsh L, Blettner M, Hammer G, Zeeb H. Is cancer risk of radiation workers larger than expected? Occupat Environ Med 2009;66(12):789–96.
- Zielinski JM, Garner MJ, Band PR, Krewski D, Shilnikova NS, Jiang H, et al. Health outcomes of low-dose ionizing radiation exposure among medical workers: a cohort study of the Canadian national dose registry of radiation workers. Int J Occup Med Environ Health 2009;22(2):149–56.
- Venneri L, Rossi F, Botto N, Andreassi MG, Salcone N, Emad A, et al. Cancer risk from professional exposure in staff working in cardiac catheterization laboratory: insights from the National Research Council's Biological Effects of Ionizing Radiation VII Report. Am Heart J 2009;157(1):118–24.
- Djurovic B, Spasic-Jokic V, Djurovic B. Influence of occupational exposure to low-dose ionizing radiation on the plasma activity of superoxide dismutase and glutathione level. Vojnosanit Pregl 2008;65(8):613–8.
- Klucinski P, Wojcik A, Grabowska-Bochenek R, Gminski J, Mazur B, Hrycek A, et al. Erythrocyte antioxidant parameters in workers occupationally exposed to low levels of ionizing radiation. Ann Agric Environ Med 2008;15(1):9–12.
- Malekirad AA, Ranjbar A, Rahzani K, Pilehvarian AA, Rezaie A, Zamani MJ, et al. Oxidative stress in radiology staff. Environ Toxicol Pharmacol 2005;20(1):215–8.
- Akköse A, Ömer B, Yiğitbaşı A. DNA damage and glutathione content in radiology technicians. Clin Chim Acta 2003;336(1–2):13–18.
- Russo GL, Tedesco I, Russo M, Cioppa A, Andreassi MG, Picano E. Cellular adaptive response to chronic radiation exposure in interventional cardiologists. Eur Heart J 2012;33(3):408–14.
- NCRP Report No. 122. Use of Personal Monitors to Estimate Effective Dose Equivalent and Effective Dose to Workers for External Exposure to Low-LET Radiation The National Council on Radiation Protection and Measurements (NCRP). 1995; Report No. 122.
- Jacobs DR, Ainsworth BE, Hartman TJ, Leon AS. A simultaneous evaluation of 10 commonly used physical activity questionnaires. Med Sci Sports Exer 1993;25(1):81–91.
- Cimen MY. Free radical metabolism in human erythrocytes. Clin Chim Acta 2008;390(1–2):1–11.
- ICRP. The 2007 recommendations of the International Commission on Radiological Protection. ICRP publication 103. Ann ICRP 2007;37(2–4):1–332.
- Linet MS, Kim KP, Miller DL, Kleinerman RA, Simon SL, Berrington de Gonzalez A. Historical review of occupational exposures and cancer risks in medical radiation workers. Radiat Res 2010;174(6):793–808.
- Andreassi MG, Foffa I, Manfredi S, Botto N, Cioppa A, Picano E. Genetic polymorphisms in XRCC1, OGG1, APE1 and XRCC3 DNA repair genes, ionizing radiation exposure and chromosomal DNA damage in interventional cardiologists. Mutat Res 2009;666(1–2):57–63.
- Andreassi MG, Cioppa A, Manfredi S, Palmieri C, Botto N, Picano E. Acute chromosomal DNA damage in human lymphocytes after radiation exposure in invasive cardiovascular procedures. Eur Heart J 2007;28(18):2195–99.
- Andreassi MG, Cioppa A, Botto N, Joksic G, Manfredi S, Federici C, et al. Somatic DNA damage in interventional cardiologists: a case-control study. FASEB J 2005;19(8):998–9.
- Fang YZ, Yang S, Wu G. Free radicals, antioxidants, and nutrition. Nutrition 2002;18(10):872–9.
- Sies H. Oxidative stress: from basic research to clinical application. Am J Med 1991;91(3C):S31–8.
- Halliwell B, Gutteridge JMC. Free radicals in biology and medicine. New York: Oxford University Press; 1999.
- Berg K, Ericsson M, Lindgren M, Gustafsson H. A high precision method for quantitative measurements of reactive oxygen species in frozen biopsies. PLoS ONE 2014;9(3):e90964.
- Sinha M, Das DK, Datta S, Ghosh S, Dey S. Amelioration of ionizing radiation induced lipid peroxidation in mouse liver by Moringa oleifera Lam. leaf extract. Indian J Exp Biol 2012;50(3):209–15.
- Deger Y, Dede S, Belge A, Mert N, Kahraman T, Alkan M. Effects of X-ray radiation on lipid peroxidation and antioxidant systems in rabbits treated with antioxidant compounds. Biol Trace Elem Res 2003;94(2):149–56.
- Kang SK, Rabbani ZN, Folz RJ, Golson ML, Huang H, Yu D, et al. Overexpression of extracellular superoxide dismutase protects mice from radiation-induced lung injury. Int J Radiat Oncol Biol Phys 2003;57(4):1056–66.
- Arterbery VE, Pryor WA, Jiang L, Sehnert SS, Foster WM, Abrams RA, et al. Breath ethane generation during clinical total body irradiation as a marker of oxygen-free-radical-mediated lipid peroxidation: a case study. Free Radic Biol Med 1994;17(6):569–76.
- Clemens MR, Ladner C, Schmidt H, Ehninger G, Einsele H, Buhler E, et al. Decreased essential antioxidants and increased lipid hydroperoxides following high-dose radiochemotherapy. Free Radic Res Commun 1989;7(3–6):227–32.
- Trosko JE, Inoue T. Oxidative stress, signal transduction, and intercellular communication in radiation carcinogenesis. Stem Cells 1997;15(Suppl. 2):59–67.
- Loft S, Poulsen HE. Cancer risk and oxidative DNA damage in man. J Mol Med (Berl) 1996;74(6):297–312.
- Kathren RL. Pathway to a paradigm: the linear non threshold dose-response model in historical context. The American Academy of Health Physics 1995 Radiology Centennial Hartman Oration. Health Phys 1996;70(5):621–35.
- Berrington de González A, Darby S. Risk of cancer from diagnostic X-rays: estimates for the UK and 14 other countries. Lancet 2004;363(9406):345–51.
- Brenner DJ, Hall EJ. Computed tomography – an increasing source of radiation exposure. N Engl J Med 2007;357(22):2277–84.
- Weitberg AB, Corvese D. Oxygen radicals potentiate the genetic toxicity of tobacco-specific nitrosamines. Clin Genet 1993;43(2):88–91.
- Deneke SM, Fanburg BL. Regulation of cellular glutathione. Am J Physiol 1989;257(4 Pt 1):L163–73.
- Puthran SS, Sudha K, Rao GM, Shetty BV. Oxidative stress and low dose ionizing radiation. Indian J Physiol Pharmacol 2009;53(2):181–4.