Abstract
Objective: There are associations between some inflammatory and oxidative markers and obesity in adults, but whether prepubescent children of different weights also have such markers has not been studied. We investigated multiple inflammatory markers and levels of erythrocyte oxidant/antioxidant enzymes in prepubescent children of different weights.
Methods: Children aged 2–11 years were divided into three groups: 80 were underweight, 90 were obese but otherwise healthy, and 80 were healthy age- and sex-matched children of normal-weight. We analyzed inflammatory markers and the total oxidant status, total antioxidant status (TAS), and total thiol level were also determined, and the oxidative stress index was calculated as an indicator of the degree of oxidative stress.
Results: The obese group exhibited higher levels of fasting glucose, insulin, total cholesterol, triglycerides, the homeostatic model assessment of insulin resistance (HOMA-IR), and the homeostatic model assessment of β-cell function (HOMA-β), C-reactive protein (CRP), neutrophils, and neutrophil/lymphocyte ratio (NLR), as well as lower TAS and total thiol levels than the other two groups (all P < 0.001). Moreover, TAS and total thiols were negatively correlated with age in the obese group (r = −0.212, P = 0.001; r = −0.231, P < 0.001, respectively). CRP levels in plasma were positively correlated with the body mass index (BMI), insulin and glucose levels, HOMA-IR, HOMA-β, WBC and neutrophil counts, and the NLR, and were negatively correlated with TAS and total thiol levels in the overall studied population.
Discussion: The coexistence of increased obesity-related subclinical inflammation and decreased antioxidant capacity can be observed even in prepubescence, and may eventually increase the risk of long-term vascular damage.
Introduction
Obesity is becoming a global epidemic in both children and adults, and chronic multifactorial disorders result from the interaction between genetic, psychological, behavioral, environmental, socioeconomic, metabolic, and molecular factors.Citation1 Recent evidence suggest that obesity is characterized by increased oxidative stress and exacerbated inflammatory outcomes accompanying infiltration of immune cells in adipocytes.Citation2,Citation3 Additionally, obesity has been linked to a low-grade proinflammatory state, in which impairments in the oxidative stress and antioxidant mechanism could be involved.Citation4 Oxidative stress is generally described as an imbalance in net levels of reactive oxygen species (ROS) relative to the body's antioxidant capacity, resulting in the accumulation of oxidative products.Citation5 ROS occur under physiological conditions and in many diseases, and cause direct or indirect damage to different organs.Citation6 It has been reported that there are several possible mechanisms by which obesity causes oxidative stress. The peroxisomal and mitochondrial oxidation of fatty acids can occur in oxidation reactions.Citation7 Obesity increases mechanical load, which in turn increases myocardial metabolism and oxygen consumption. The over-consumption of oxygen, which generates free radicals (superoxide, hydroxyl radical, hydrogen peroxide) in the mitochondrial respiratory chain, is coupled with oxidative phosphorylation in mitochondria.Citation8
Recent studies demonstrated that excessive fat accumulation can cause cellular damage due to pressure from fat cells. This in turn leads to a high production of cytokines such as TNF-α, IL-1, and IL-6, which generates ROS in the tissues, increasing the lipid peroxidation rate. The formation of lipid hydroxyperoxides occurs in enzymatic or non-enzymatic reactions involving ROS which are responsible for toxic effects in the body via various tissue damages.Citation9 Also these increased cytokines are potent stimulators for the production of reactive oxygen and nitrogen by macrophages and monocytes. The elevated concentration of cytokines could be responsible for increased oxidative stress.Citation6
Oxidative stress occurs when the intracellular concentrations of free radicals increase over physiological values, which results in cell damage by lipid peroxidation, DNA and protein damage, enzyme oxidation, and stimulation of proinflammatory cytokine release.Citation10,Citation11 Oxidant status can be assessed by determining the total oxidant status (TOS). The adaptation of the cell to an increase in radical production consists of an increase in enzymatic antioxidant activity to prevent oxidative damage to cells. The antioxidant status of individuals depends on multiple factors. Enzymatic antioxidant (superoxide dismutase (SOD), catalase, glutathione peroxidase), the major non-enzymatic antioxidant (tripeptide glutathione), a subset of vitamins (α-tocopherol, ascorbic acid, carotenoids and flavonoids, zinc), and endogenous antioxidants (albumin, uric acid, and bilirubin) are the most widely used biomarkers of antioxidant status.Citation12 Another useful biomarker is the total antioxidant capacity which evaluates the integrated action of all antioxidants present in plasma.Citation13
Oxidative stress might be a major mechanism underlying obesity-related co-morbidities, including atherosclerosis, insulin resistance, type 2 diabetes, and cancer.Citation14 Such metabolic and inflammatory disturbances in children often remain until adulthood and contribute to the development of various health problems.Citation15
The enlargement of adipocytes by fat storage induces adipose tissue hypoxia and secretion of high levels of inflammatory cytokines and chemokines.Citation16 Increased secretion of these molecules by activated leukocytes, macrophages and other tissues has been demonstrated in obese patients.Citation17 The increased macrophage infiltration of adipose tissue may be an important component of the chronic inflammatory response in the development of insulin resistance.Citation18,Citation19 Additionally, ROS are also released by activated neutrophils and other leukocytes; indeed, WBC secretion of oxidative and inflammatory surrounding the lipid droplets of the atheromatic core is a crucial biochemical step linking atherosclerotic plaques to thrombotic luminal occlusion.Citation20
Many studies have shown a relationship between different oxidative stress and inflammatory markers in obese adults and older children/adolescents, but few studies have evaluated these together with oxidative/antioxidative status and inflammation markers in prepubescent children. Therefore, to investigate the potential relationships between multiple inflammatory and oxidative stress markers and the weight of children, we assessed white blood cell (WBC) count in serum, absolute neutrophil and lymphocyte counts, the neutrophil/lymphocyte ratio (NLR), C-reactive protein (CRP) levels, and oxidative/ antioxidative markers in three different groups (underweight, obese, and healthy children) aged 2–11 years. Our specific goal was to isolate effects according to age and gender, while eliminating the effects of puberty.
Method
This cross-sectional study was carried out at the Bezmialem Vakif University Hospital General Pediatrics Clinic in Istanbul. Randomly selected pediatric patients who attended the outpatient clinic for routine examinations between October 2014 and April 2015 were recruited. The study population consisted of 250 prepubescent children aged 2–11 years; The three study groups included 80 healthy anorexic children (with a body mass index [BMI] <the 18.5th percentile for each age and sex), 90 obese but otherwise healthy children (with a BMI ≥the 95th percentile), and 80 healthy children of normal-weight (with a BMI in the 18.5th to 95th percentile). The inclusion criteria consisted of a dietary history with adequate nutrient intake (in quality and quantity). Underweight patients were able to obtain food, but had poor appetites. The exclusion criteria included the presence of endocrine disease (Cushing's syndrome or hypothyroidism), acute or chronic inflammatory disease, malabsorption syndromes such as celiac disease or cystic fibrosis, infections or systemic illnesses, and the use of prescription medications, vitamins, or mineral supplements for any reason. Each children underwent a detailed physical examination (including evaluation for systemic and endocrine diseases). To exclude gluten enteropathy, we examined total IgA, tissue transglutaminase antibody IgA (anti tTG-IgA) in both underweight and normal-weight children. We recruited four children who were diagnosed with celiac disease during the follow up. Consequently, children without any health problems except for anorexia were included in this study as underweight group. They had no dietary history of inadequate nutrient intake in means of quality or quantity. The children had the opportunity to obtain food, but they had only poor appetites. Subjects were classified as underweight, normal, or obese according to Cole's recently published BMI cut-offs for underweight/overweight/obese according to sex and age (for children aged 2–18 years).Citation21
After 12 hours of fasting, venous blood samples were collected between 8:00 a.m. and 9:00 a.m. The samples were separated by centrifugation (10 minutes at 3082 × g, 4°C) and stored at –80°C for subsequent use. The complete blood count, and levels of iron and total iron-binding capacity in serum, ferritin, thyroid stimulating hormone (TSH), T4, vitamin B12, folic acid, glucose, albumin, insulin, total cholesterol, triglycerides, and CRP were assessed in all subjects. Total cholesterol and triglycerides were measured using the homogeneous colorimetric enzyme technique (Roche Cobas 8000 modular analyzer; Roche Diagnostics, Mannheim, Germany). Glucose was measured using the glucose oxidase technique (Advia 1800; Siemens Healthcare Diagnostics, Tarrytown, NY, USA); insulin levels were analyzed using the direct chemiluminescence technique (Advia Centaur, Siemens). IR was estimated from plasma measurements after fasting using the homeostatic model assessment of insulin resistance (HOMA-IR, calculated as insulin [µU/l] × glucose [mmol/l]/22.5). The criterion for IR in prepubescent children was an HOMA-IR of >2.5. The homeostatic model assessment of β-cell function (HOMA-β, calculated as 20 × insulin [µU/l]/glucose [mmol/l] – 3.5) was estimated using the formulae developed by Wallace et al.Citation22
The number of leukocytes and leukocyte subtypes were computed with an autoanalyzer (Sysmex XT-2000i Kobe, Japan). The NLR was defined as neutrophil count/lymphocyte count. CRP levels in serum were measured with a particle-enhanced immunoturbidimetric assay using the Roche Cobas 8000 modular analyzer. The cut-off value for CRP was assigned as 5.0 mg/l. The maximum intra-assay and inter-assay coefficients of variation were 2.38% and 4%, respectively.
Total oxidant and antioxidant status and oxidative stress index (OSI)
The total antioxidant status (TAS) and TOS of serum were determined using an automated measurement method with an automated analyzer (Chromate Manager 4300, Palm City, USA), as described in a previous study.Citation23 In the measurement of TAS, the absorbance of the colored dianisidyl radicals was monitored to determine the rate of Fenton's reaction, which initiates free-radical reactions with the production of a hydroxyl radical. Then, the antioxidative effect of the sample against potent free-radical reactions was measured in terms of mmol equiv/l Trolox (Rel Assay Diagnostics, Gaziantep, Turkey).
In the measurement of TOS, the intensity of the colored complex produced by the reaction of ferric ions, which were oxidized from a ferrous iono-dianisidine complex due to the presence of oxidants, with xylenol orange in an acidic medium was used to determine the total amount of oxidant molecules in the sample. The calibration of the assay was performed with hydrogen peroxide. TOS values are expressed in terms of micromolar hydrogen peroxide equivalents per liter (μmol H2O2 equiv/l) (Rel Assay Diagnostics, Gaziantep, Turkey).Citation24
The OSI is the ratio of the TOS level to the TAS level. For the resulting micromolar unit, TAS was converted into millimoles per liter, and the OSI value was calculated according to the following formula: OSI (arbitrary unit) = TOS/TAS × 100.
The measurement of total free sulfhydryl groups (–SH) in serum samples was assayed according to Ellman's method, as modified by Hu and Chou.Citation25 Briefly, 1 ml of a buffer containing 0.1 M Tris, 10 mM EDTA (pH 8.2), and 50 µl serum was added to cuvettes, followed by 50 µl 10 mM DTNB in methanol. Blanks were run for each sample as a test, but without the DTNB. Following incubation for 15 minutes at room temperature, the sample absorbance was read at 412 nm on a Cecil 3000 spectrophotometer (Cecil Instruments, Cambridge, UK). Sample and reagent blanks made up the substrate. The concentration of sulfhydryl groups was calculated using reduced glutathione as the free sulfhydryl group standard, and the results are expressed as mmol/l. The CV to measure the serum –SH level was 3.9%.
Statistical analysis
Analyses were conducted using the IBM SPSS for Windows software package (ver. 20.0; IBM Corp., Armonk, NY, USA). The results are presented as means ± SD, with categorical variables presented as frequencies and percentages. The comparison of the groups was performed using a one-way analysis of variance (ANOVA) for parametric tests with Tukey's HSD post hoc test applied for multiple comparisons of the oxidant/antioxidant/inflammatory markers between the BMI groups. Obese children with IR were compared to obese children without IR using a t-test. A Pearson's correlation was used to determine the relationships between variables. A P-value of <0.05 was taken to indicate statistical significance.
Information concerning the aim of the study was provided to the children's parents at the time of enrollment; written informed consent was also obtained. Ethical approval was granted by the Bezmialem Vakif University Local Research Ethics Committee.
Results
Of the 250 children, 121 were male and 129 were female. The anthropometric and metabolic characteristics of the groups are summarized in Tables –. The age and sex distribution did not differ among the three groups (P = 0.840 and 0.993, respectively). The underweight group had a significantly lower mean weight, weight z-score, mean height, height z-score, BMI, BMI z-score, and BMI percentage (all P < 0.001) than the control and obese groups.
Table 1 Comparison of demographic and anthropometric characteristics in underweight, normal-weight and obese children
Table 2 Comparison of laboratory characteristics of subjects according to BMI stage group
Table 3 Comparison of proinflammatory indicators of subjects according to BMI stage group
There were no significant group differences in the levels of hemoglobin, hematocrit (hct), albumin, vitamin B12, or ferritin (P = 0.790, 0.055, 0.880, 0.060, 0.606, respectively) (Table ). In addition, there were no significant group differences in WBC and lymphocyte counts, TOS levels, or OSI (P = 0.055, 0.187, 0.289, 0.075, respectively) (Table ).
No significant differences were found between the underweight and normal-weight children in terms of fasting glucose, insulin, total cholesterol, triglycerides, HOMA-IR, HOMA-β, neutrophil counts, NLR, CRP, TAS, or total thiol level (P = 0.940, 0.427, 0.900, 0.740, 0.999, 0.524, 0.125, 0.540, 0.605, 0.933, 0.529, 0.778, respectively).
The obese group had higher fasting glucose, insulin, total cholesterol, triglyceride, HOMA-IR, HOMA-β, CRP, neutrophil count, and NLR but lower TAS and thiol levels than the other two groups (all P < 0.001). Moreover, TAS and total thiol level were negatively correlated with age in the obese group (r = −0.212, P = 0.001; r = −0.231, P < 0.001, respectively). However, WBC counts, neutrophils, NLR, and CRP levels all showed significant and positive correlations with BMI (r = 0.176, P = 0.007; r = 0.176, P < 0.001; r = 0.205, P = 0.002; r = 0.471, P < 0.001) (Fig. ). TAS and total thiol levels were negatively correlated with BMI (r = −0.191, P = 0.004; r = −0.231, P < 0.001) (Fig. ). In addition, analysis showed a significant negative correlation between TAS and HOMA-IR, HOMA-β, CRP, WBC counts, neutrophils, NLR, and OSI, and a significantly positive correlation between TAS and total thiol levels. The Pearson's correlation for multiple comparisons of the oxidant/antioxidant/inflammatory markers between the BMI groups are summarized in Tables and
Figure 1 Scatter plot figures for Pearson's correlation of WBC, WBC subtypes, and NLR with BMI in overall population. WBC: White blood cell, BMI: Body mass index, NLR: Neutrophil–lymphocyte ratio, blood cell is expressed as ×103/µl.
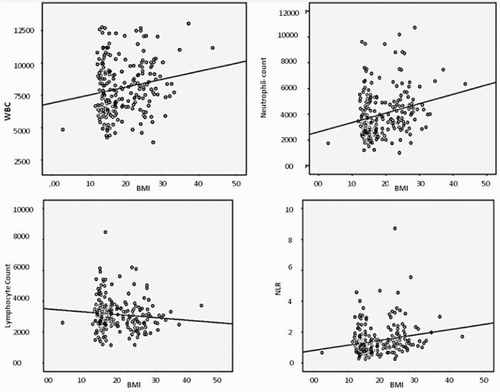
Figure 2 Pearson's correlation of TAS, TOS, OSI and Total thiol with BMI. TAS: Total antioxidant status (mmol equiv/l Trolox), TOS: Total oxidant status (μmol H2O2 equiv/l), OSI: Oxidative stress index (arbitrary units), Total thiol (mmol/l).
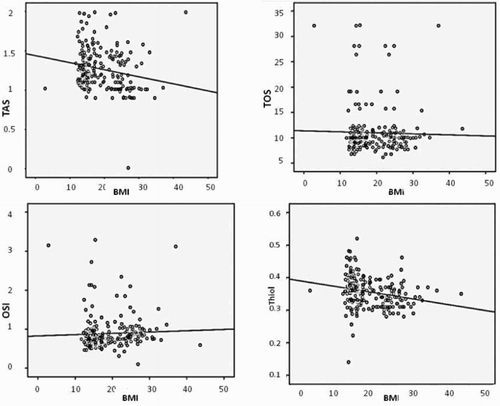
Table 4 The results of Pearson's correlation between proinflammatory markers and other anthropometric and metabolic parameters
Table 5 The results of Pearson's correlation between oxidant/antioxidant status and other anthropometric and metabolic parameters
Discussion
In the present study, we evaluated the pattern of oxidative stress and the different inflammatory markers in three different weight groups of prepubescent children. Although associations between these parameters and obesity have been found to be greater for older children and adolescents,Citation26,Citation27 it was unknown whether this relationship existed from an early age. We observed significantly higher levels of fasting glucose, insulin, total cholesterol, triglycerides, HOMA-IR, HOMA-β, CRP, neutrophil count, and NLR in obese prepubescent children compared to underweight and normal-weight children. However, antioxidant status and total thiol level were markedly lower in obese children compared to the other groups, and these parameters are negative correlated with age in obese group. Correlation analysis revealed a significant negative correlation between TAS and BMI, insulin, HOMA-IR, and HOMA-β. These observations indicated that at an early age, obese prepubescent children had concurrently elevated inflammatory and decreased antioxidant status compared to underweight/normal-weight and gender matched controls.
Previously many studies have evaluated antioxidant status in obese subjects and diverse results have been found. Kobayasi et al.Citation28 demonstrated that the production of superoxide anions and Cu/Zn SOD activity increased in obese mice. Sfar et al.Citation29 showed that the activity of antioxidant enzymes was markedly higher in obese children than in normal-weight ones. Similarly, Amaya-Villalva et al.Citation30 found that in adults, obese individuals had a higher antioxidant capacity, and antioxidant status was correlated with obesity-related indicators. However, other studies have determined that low antioxidant status and reduced antioxidant capacity are common in obese children and adolescents compared with normal children similar to our study results. For example, Beydoun et al.,Citation31 reported that obese U.S. adolescents with metabolic syndrome had lower serum carotenoid concentrations compared with their counterparts without MetS in recent national survey. Karamouzis et al.Citation32 recently demonstrated that the loss of the normal homeostatic balance between the oxidant–antioxidant state leads to enhanced oxidative stress combined with a reduced antioxidant capacity in obese prepubescent and adolescent girls. Another study, OlusiCitation33 found that erythrocyte Cu/Zn SOD activity was significantly lower in obese subjects than in controls. Similarly, Molnár et al.Citation34 showed that the TAS in plasma and α-tocopherol levels were reduced in obese children with metabolic syndrome. Interestingly, Brown et al.Citation35 showed that there were no significant differences in TAS and a reduction in glutathione among normal-weight, overweight, and obese adults. These many diverse results can be explained by the age properties of study populations. We suggest that the long duration of oxidative stress in adults resulted in elevation of antioxidants for compensation. In studies with adolescents and prepubescent studies the duration of obesity may be not enough for the increase of antioxidants.
An inadequacy of antioxidant defenses probably depends on different factors: obese individuals may have a lower intake of antioxidant- and phytochemical-rich food, such as fruits and vegetables.Citation36 Recent studies suggest that despite excessive dietary consumption, obese individuals have high rates of micronutrient deficiencies. In these studies it was demonstrated that deficiency in minerals and vitamins can also contribute to impairment of antioxidant defenses.Citation37 Also low levels of carotenoids, vitamin C and E, despite increased BMI, have been demonstrated in obese children. Furthermore, low concentrations of zinc, vitamins A and E in children who were overweight and obese were associated with lipids, inflammation and insulin resistance.Citation38 Otherwise, the consumption of antioxidants is normal, but obese individuals may have an increased utilization of these molecules, and inadequate physical activity may account for a decreased antioxidant state.Citation39
In the recent studies, the role of specific nutrients has been investigated, and suggested that obese children should be urged to consume products containing greater levels of antioxidants. Codoñer-Franch et al. demonstrated that obese children on a hypocaloric diet supplemented with 100% mandarin juice, a product naturally rich in antioxidant such as vitamin C, carotenoids, and flavonoids, displayed a decrease in parameters of oxidative stress and an increase in antioxidant defense.Citation40 Murer et al.,Citation41 conducted to examine the effect of antioxidant supplementation on biomarkers of oxidative stress and inflammation in obese adolescents, and they found that combined antioxidant supplementation for 4 months significantly improve antioxidant status. Another study showed that fruit and vegetable intake was associated with lower concentrations of systemic oxidative stress and inflammation in early adolescents.Citation42
Our study showed that TAS and the total thiol level was reduced in prepubescent obese children, suggesting that the balance between the oxidant and antioxidant system is disrupted due to a reduced TAS. Thiols are organic compounds that contain a sulphydryl group. Thiols constitute the greatest portion of total antioxidants and play a significant role in defense against ROS.Citation43 In addition, they play a significant role in detoxification, signal transduction, apoptosis, and various other functions at the molecular level. Decreased levels of thiols have been noted in various medical disorders including chronic renal failure and cardiovascular disorders.Citation44 In a similar study, Yokota et al.Citation45 demonstrated that total thiol levels in serum were decreased in patients with metabolic syndrome compared to healthy subjects.
Obesity is considered a state of chronic low-grade inflammation, and several studies in obese adults and older children have linked inflammation and oxidative stress.Citation46 Inflammation of adipose tissue causes the major events of the immune response, such as the early participation of neutrophils, the following procurement of diverse lymphocyte types, and the final procurement of both macrophage and mast cell polarization.Citation47 The adipose tissue macrophages plays a key role in systemic IR, glucose tolerance, and the development of metabolic syndromes and type 2 diabetes.Citation48 Wu et al.Citation49 showed that plasma CRP levels are positively related to anthropometric variables, such as body weight and BMI, insulin levels, and the IR index, and negatively related to high-density lipoprotein cholesterol (HDL-C) in Taiwanese children. The study including the youngest population about obesity and inflammation was done by Skinner et al.Citation50 They demonstrated that an increased risk of an abnormal neutrophil count and the CRP level are positively associated with higher weight in children, and that this relationship begins as early as the age of 3. Our study showed similarities to the recent literature, in that the obese group had a higher CRP, neutrophil count, and NLR. In addition, we found that plasma CRP levels, neutrophil counts, and NLR were positively correlated with BMI, insulin and glucose levels, HOMA-IR, and HOMA-β, and that these parameters were positively correlated with each other. Our results suggest that healthy young subjects with more body fat or a higher BMI have higher concentrations of inflammatory markers than their leaner peers, supporting the idea that obesity should be considered a state of chronic low-grade inflammation. Also Ryder et al.Citation51 demonstrated a positive correlation between leukocytes and the lymphocyte count and BMI and HOMA-IR in non-diabetic, obese adults without clinical and biochemical changes. Their results suggest that obese individuals without a metabolic syndrome could represent an initial stage of inflammation during obesity. Even in the absence of IR, inflammatory markers were increased. Ferrari et al.Citation52 demonstrated that the adiposity of European adolescents was sufficient to cause chronic inflammation, and the mean CRP values were significantly higher in overweight/obese adolescents than in thin/normal-weight adolescents. The causes of obesity-induced chronic inflammation have focused on monocyte/macrophage infiltration and activation in the adipose tissue.Citation53 Previous studies in adults have shown that CRP has now emerged as one of the most powerful predictors of inflammatory and lipid markers for the evidence of cardiovascular events in adults.Citation54 There are currently no guidelines associating the CRP level and cardiovascular risk in children, but adults with a CRP >3 mg/l are considered to have a 1.5- to 2-fold increased risk of cardiovascular disease.Citation55 In our study, obese children had markedly elevated mean CRP values (4.11 ± 3.10 mg/l) compared to underweight/normal-weight children. These results suggest that the obese children in our study population had a high risk of future cardiovascular disease according to the adult cut-off point. However, there were no significant differences in the CRP level between underweight and normal-weight children (P = 0.933).
Childhood obesity as a consequence of high fat deposition has been demonstrated to be related to high levels of oxidative stress and redox imbalance. Warolin et al.Citation56 showed that oxidative stress was positively associated with the percentage of body fat and truncal fat in youth (8–17-years-old). In literature there are a few studies performed in obese children related to biomarkers of inflammation and oxidative stress. Oliver et al.Citation57 demonstrated that obese children had elevated biomarkers of inflammation (elevated total WBC counts and neutrophils) and oxidative stress, independent of gender (n = 113, 12.9 ± 0.3 years). Similarly in another study, Giannini et al.Citation58 demonstrated that the oxidative status were higher in pre-pubertal lean and obese subjects compared with controls whereas no differences were documented between lean and obese children. Although the general consensus is that obesity increases oxidative stress even during childhood, we did not find significant differences among the different weight groups. This could be explained by the fact that our study population consisted of prepubescent children with a mean age of 7.0–7.4 years.
Our work had some limitations. The study sample size was small for the proper investigation of the subgroups of obesity such as IR, and also the relationship between variables, thus providing a lack of statistical significance in terms of the TOS and OSI values. A prospective study with a large sample size may provide more reliable data in the future.
In conclusion, we found that obese children have higher inflammatory markers and lower TAS values than underweight and normal-weight children even in prepubescence, which indicates a risk of future cardiovascular events. But we could not demonstrate an increased burden of inflammation or decreased antioxidant status in underweight children. Further studies to determine the cause-and-effect relationship between inflammation and oxidation in children are warranted.
Disclaimer statements
Contributors All authors contributed equally.
Funding None.
Conflict of interest The authors have indicated that they have no potential conflicts of interest to disclose.
Ethical approval Ethical approval was granted by the Bezmialem Vakif University Local Research Ethics Committee.
ORCID
Aysel Vehapoglu http://orcid.org/0000-0002-4000-6330
References
- Vos MB, Welsh J. Childhood obesity: update on predisposing factors and prevention strategies. Curr Gastroenterol Rep 2010;12(4):280–7. doi: 10.1007/s11894-010-0116-1
- Revelo XS, Luck H, Winer S, Winer DA. Morphological and inflammatory changes in visceral adipose tissue during obesity. Endocr Pathol 2014;25(1):93–101. doi: 10.1007/s12022-013-9288-1
- McNelis JC, Olefsky JM. Macrophages, immunity, and metabolic disease. Immunity 2014;41(1):36–48. doi: 10.1016/j.immuni.2014.05.010
- Calabrò P, Limongelli G, Pacileo G, Di Salvo G, Golino P, Calabrò R. The role of adiposity as a determinant of an inflammatory milieu. J Cardiovasc Med (Hagerstown) 2008;9(5):450–60. doi: 10.2459/JCM.0b013e3282eee9a8
- Poljsak B, Šuput D, Milisav I. Achieving the balance between ROS and antioxidants: when to use the synthetic antioxidants. Oxid Med Cell Longev 2013;2013:956792. doi: 10.1155/2013/956792
- Fernández-Sánchez A, Madrigal-Santillán E, Bautista M, Esquivel-Soto J, Morales-González A, Esquivel-Chirino C, et al.,. Inflammation, oxidative stress, and obesity. Int J Mol Sci 2011;12(5):3117–32. doi: 10.3390/ijms12053117
- Jung UJ, Choi MS. Obesity and its metabolic complications: the role of adipokines and the relationship between obesity, inflammation, insulin resistance, dyslipidemia and nonalcoholic fatty liver disease. Int J Mol Sci 2014;15(4):6184–223. doi: 10.3390/ijms15046184
- Li X, Fang P, Mai J, Choi ET, Wang H, Yang XF. Targeting mitochondrial reactive oxygen species as novel therapy for inflammatory diseases and cancers. J Hematol Oncol 2013;6:19. doi: 10.1186/1756-8722-6-19
- Ramos-Nino ME. The role of chronic inflammation in obesity-associated cancers. ISRN Oncol 2013;2013:697521.
- Codoñer-Franch P, Valls-Bellés V, Arilla-Codoñer A, Alonso-Iglesias E. Oxidant mechanisms in childhood obesity: the link between inflammation and oxidative stress. Transl Res 2011;158(6):369–84. doi: 10.1016/j.trsl.2011.08.004
- Crujeiras AB, Díaz-Lagares A, Carreira MC, Amil M, Casanueva FF. Oxidative stress associated to dysfunctional adipose tissue: a potential link between obesity, type 2 diabetes mellitus and breast cancer. Free Radic Res 2013;47(4):243–56. doi: 10.3109/10715762.2013.772604
- Rizzo AM, Berselli P, Zava S, Montorfano G, Negroni M, Corsetto P, et al. Endogenous antioxidants and radical scavengers. Adv Exp Med Biol 2010;698:52–67. doi: 10.1007/978-1-4419-7347-4_5
- Pinchuk I, Shoval H, Dotan Y, Lichtenberg D. Evaluation of antioxidants: scope, limitations and relevance of assays. Chem Phys Lipids 2012;165(6):638–47. doi: 10.1016/j.chemphyslip.2012.05.003
- Marseglia L, Manti S, D'Angelo G, Nicotera A, Parisi E, Di Rosa G, et al. Oxidative stress in obesity: a critical component in human diseases. Int J Mol Sci 2014;16(1):378–400. doi: 10.3390/ijms16010378
- Llewellyn A, Simmonds M, Owen CG, Woolacott N. Childhood obesity as a predictor of morbidity in adulthood: a systematic review and meta-analysis. Obes Rev 2015. doi:10.1111/obr.12316.
- O'Rourke RW, White AE, Metcalf MD, Olivas AS, Mitra P, Larison WG, et al. Hypoxia-induced inflammatory cytokine secretion in human adipose tissue stromovascular cells. Diabetologia 2011;54(6):1480–90. doi: 10.1007/s00125-011-2103-y
- Romeo GR, Lee J, Shoelson SE. Metabolic syndrome, insulin resistance, and roles of inflammation – mechanisms and therapeutic targets. Arterioscler Thromb Vasc Biol 2012;32(8):1771–6. doi: 10.1161/ATVBAHA.111.241869
- Fuentes E, Fuentes F, Vilahur G, Badimon L, Palomo I. Mechanisms of chronic state of inflammation as mediators that link obese adipose tissue and metabolic syndrome. Mediators Inflamm 2013;2013:136584. doi: 10.1155/2013/136584
- Samuel VT, Shulman GI. Mechanisms for insulin resistance: common threads and missing links. Cell 2012;148(5):852–71. doi: 10.1016/j.cell.2012.02.017
- Hansson GK, Libby P, Tabas I. Inflammation and plaque vulnerability. J Intern Med 2015;278(5):483–93. doi:10.1111/joim.12406.
- Cole TJ, Lobstein T. Extended international (IOTF) body mass index cut-offs for thinness, overweight and obesity. Pediatric Obes 2012;7:284–94. doi: 10.1111/j.2047-6310.2012.00064.x
- Wallace TM, Levy JC, Matthews DR. Use and abuse of HOMA modeling. Diabetes Care 2004;27(6):1487–95. doi: 10.2337/diacare.27.6.1487
- Erel O. A novel automated method to measure total antioxidant response against potent free radical reactions. Clin Biochem 2004;37:112–9. doi: 10.1016/j.clinbiochem.2003.10.014
- Erel O. A new automated colorimetric method for measuring total oxidant status. Clin Biochem 2005;38:1103–11. doi: 10.1016/j.clinbiochem.2005.08.008
- Hu TM, Chou TC. The kinetics of thiol-mediated decomposition of S-nitrosothiols. AAPS J 2006;8(3):E485–92. doi: 10.1208/aapsj080357
- Nagel G, Rapp K, Wabitsch M, Büchele G, Kroke A, Zöllner I, et al. Prevalence and cluster of cardiometabolic biomarkers in overweight and obese schoolchildren: results from a large survey in southwest Germany. Clin Chem 2008;54(2):317–25. doi: 10.1373/clinchem.2007.094821
- Lande MB, Pearson TA, Vermilion RP, Auinger P, Fernandez ID. Elevated blood pressure, race/ethnicity, and C-reactive protein levels in children and adolescents. Pediatrics 2008;122(6):1252–7. doi: 10.1542/peds.2007-3162
- Kobayasi R, Akamine EH, Davel AP, Rodrigues MA, Carvalho CR, Rossoni LV. Oxidative stress and inflammatory mediators contribute to endothelial dysfunction in high-fat diet-induced obesity in mice. J Hypertens 2010;28(10):2111–9. doi: 10.1097/HJH.0b013e32833ca68c
- Sfar S, Boussoffara R, Sfar MT, Kerkeni A. Antioxidant enzymes activities in obese Tunisian children. Nutr J 2013;12:18. doi: 10.1186/1475-2891-12-18
- Amaya-Villalva MF, Gonzalez-Aguilar G, Rouzaud-Sandez O, Gorinstein S, Astiazaran-García H, Robles-Sanchez M. Obesity-related indicators and their relationship with serum antioxidant activity levels in Mexican adults. Nutr Hosp 2015;31:1989–95.
- Beydoun MA, Canas JA, Beydoun HA, Chen X, Shroff MR, Zonderman AB. Serum antioxidant concentrations and metabolic syndrome are associated among U.S. adolescents in recent national surveys. J Nutr 2012;142(9):1693–704. doi: 10.3945/jn.112.160416
- Karamouzis I, Pervanidou P, Berardelli R, Iliadis S, Papassotiriou I, Karamouzis M, et al. Enhanced oxidative stress and platelet activation combined with reduced antioxidant capacity in obese prepubertal and adolescent girls with full or partial metabolic syndrome. Horm Metab Res 2011;43(9):607–13. doi: 10.1055/s-0031-1284355
- Olusi SO. Obesity is an independent risk factor for plasma lipid peroxidation and depletion of erythrocyte cytoprotectic enzymes in humans. Int J Obes Relat Metab Disord 2002;26(9):1159–64. doi: 10.1038/sj.ijo.0802066
- Molnár D, Decsi T, Koletzko B. Reduced antioxidant status in obese children with multimetabolic syndrome. Int J Obes Relat Metab Disord 2004;28(10):1197–202. doi: 10.1038/sj.ijo.0802719
- Brown LA, Kerr CJ, Whiting P, Finer N, McEneny J, Ashton T. Oxidant stress in healthy normal-weight, overweight, and obese individuals. Obesity (Silver Spring) 2009;17(3):460–6. doi: 10.1038/oby.2008.590
- Rendo-Urteaga T, Puchau B, Chueca M, Oyarzabal M, Azcona-Sanjulián MC, Martínez JA, Marti A. Total antioxidant capacity and oxidative stress after a 10-week dietary intervention program in obese children. Eur J Pediatr 2014;173(5):609–16. doi: 10.1007/s00431-013-2229-7
- Via M. The malnutrition of obesity: micronutrient deficiencies that promote diabetes. ISRN Endocrinol 2012;2012:103472. doi: 10.5402/2012/103472
- García OP, Ronquillo D, del Carmen Caamaño M, Martínez G, Camacho M, López V, et al. Zinc, iron and vitamins A, C and e are associated with obesity, inflammation, lipid profile and insulin resistance in Mexican school-aged children. Nutrients 2013;5(12):5012–30. doi: 10.3390/nu5125012
- Savini I, Catani MV, Evangelista D, Gasperi V, Avigliano L. Obesity-associated oxidative stress: strategies finalized to improve redox state. Int J Mol Sci 2013;14(5):10497–538. doi: 10.3390/ijms140510497
- Codoñer-Franch P, López-Jaén AB, De La Mano-Hernández A, Sentandreu E, Simó-Jordá R, Valls-Bellés V. Oxidative markers in children with severe obesity following low-calorie diets supplemented with mandarin juice. Acta Paediatr 2010;99(12):1841–6. doi: 10.1111/j.1651-2227.2010.01903.x
- Murer SB, Aeberli I, Braegger CP, Gittermann M, Hersberger M, Leonard SW, et al. Antioxidant supplements reduced oxidative stress and stabilized liver function tests but did not reduce inflammation in a randomized controlled trial in obese children and adolescents. J Nutr 2014;144(2):193–201. doi: 10.3945/jn.113.185561
- Holt EM, Steffen LM, Moran A, Basu S, Steinberger J, Ross JA, et al. Fruit and vegetable consumption and its relation to markers of inflammation and oxidative stress in adolescents. J Am Diet Assoc 2009;109(3):414–21. doi: 10.1016/j.jada.2008.11.036
- Kemp M, Go YM, Jones DP. Nonequilibrium thermodynamics of thiol/disulfide redox systems: a perspective on redox systems biology. Free Radic Biol Med 2008;44(6):921–37. doi: 10.1016/j.freeradbiomed.2007.11.008
- Balcerczyk A, Grzelak A, Janaszewska A, Jakubowski W, Koziol S, Marszalek M, et al. Thiols as major determinants of the total antioxidant capacity. Biofactors 2003;17(1–4):75–82. doi: 10.1002/biof.5520170108
- Yokota T, Kinugawa S, Yamato M, Hirabayashi K, Suga T, Takada S, et al. Systemic oxidative stress is associated with lower aerobic capacity and impaired skeletal muscle energy metabolism in patients with metabolic syndrome. Diabetes Care 2013;36(5):1341–6. doi: 10.2337/dc12-1161
- Bondia-Pons I, Ryan L, Martinez JA. Oxidative stress and inflammation interactions in human obesity. J Physiol Biochem 2012;68(4):701–11. doi: 10.1007/s13105-012-0154-2
- Chatzigeorgiou A, Karalis KP, Bornstein SR, Chavakis T. Lymphocytes in obesity-related adipose tissue inflammation. Diabetologia 2012;55(10):2583–92. doi: 10.1007/s00125-012-2607-0
- Osborn O, Olefsky JM. The cellular and signaling networks linking the immune system and metabolism in disease. Nat Med 2012;18(3):363–74. doi: 10.1038/nm.2627
- Wu DM, Chu NF, Shen MH, Wang SC. Obesity, plasma high sensitivity C-reactive protein levels and insulin resistance status among school children in Taiwan. Clin Biochem 2006;39(8):810–5. doi: 10.1016/j.clinbiochem.2006.05.007
- Skinner AC, Steiner MJ, Henderson FW, Perrin EM. Multiple markers of inflammation and weight status: cross-sectional analyses throughout childhood. Pediatrics 2010;125(4):e801–9. doi: 10.1542/peds.2009-2182
- Ryder E, Diez-Ewald M, Mosquera J, Fernández E, Pedreañez A, Vargas R, et al. Association of obesity with leukocyte count in obese individuals without metabolic syndrome. Diabetes Metab Syndr 2014;8(4):197–204. doi: 10.1016/j.dsx.2014.09.002
- Ferrari M, Cuenca-García M, Valtueña J, Moreno LA, Censi L. HELENA Study Group. Inflammation profile in overweight/obese adolescents in Europe: an analysis in relation to iron status. Eur J Clin Nutr 2015;69(2):247–55. doi: 10.1038/ejcn.2014.154
- Lolmède K, Duffaut C, Zakaroff-Girard A, Bouloumié A. Immune cells in adipose tissue: key players in metabolic disorders. Diabetes Metab 2011;37(4):283–90. doi: 10.1016/j.diabet.2011.03.002
- Szmitko PE, Verma S. C-reactive protein and the metabolic syndrome: useful addition to the cardiovascular risk profile? J Cardiometab Syndr 2006;1(1):66–9. doi: 10.1111/j.0197-3118.2006.05340.x
- Salazar J, Martínez MS, Chávez M, Toledo A, Añez R, Torres Y, et al. C-reactive protein: clinical and epidemiological perspectives. Cardiol Res Pract 2014;2014:605810. doi:10.1155/2014/605810.
- Warolin J, Coenen KR, Kantor JL, Whitaker LE, Wang L, Acra SA, et al. The relationship of oxidative stress, adiposity and metabolic risk factors in healthy Black and White American youth. Pediatr Obes 2014;9(1):43–52. doi: 10.1111/j.2047-6310.2012.00135.x
- Oliver SR, Rosa JS, Milne GL, Pontello AM, Borntrager HL, Heydari S, et al. Increased oxidative stress and altered substrate metabolism in obese children. Int J Pediatr Obes 2010;5(5):436–44. doi: 10.3109/17477160903545163
- Giannini C, de Giorgis T, Scarinci A, Cataldo I, Marcovecchio ML, Chiarelli F, et al. Increased carotid intima-media thickness in pre-pubertal children with constitutional leanness and severe obesity: the speculative role of insulin sensitivity, oxidant status, and chronic inflammation. Eur J Endocrinol 2009;161(1):73–80. doi: 10.1530/EJE-09-0042