ABSTRACT
Background: In Chinese folk medicine, Corni fructus (C. fructus) has traditionally been used to improve liver function, although the mechanism underlying its activity remains unclear. The aim of the present study was to evaluate the protective effects of wild C. fructus methanolic extract against acute alcoholic liver injury.
Methods: Alcohol was administered to mice for three consecutive days, either alone or in combination with C. fructus methanolic extract (50, 100, or 200 mg/kg body weight/d). Serum and liver tissue were collected from the animals and subjected to biochemical and histopathological analyses.
Results: C. fructus significantly alleviated alcohol-induced liver injury by reducing serum alanine aminotransferase, aspartate aminotransferase, and thiobarbituric acid reactive species, inhibiting hydroxyl radicals (•OH), and increasing total superoxide dismutase, glutathione peroxidase, and glutathione in the liver (P < 0.05). In addition, the C. fructus treatment inhibited the expression and activity of cytochrome P450 2E1 (P < 0.05).
Conclusions: C. fructus could be a promising natural substance for ameliorating acute alcohol-induced oxidative stress and hepatic injury.
GRAPHICAL ABSTRACT
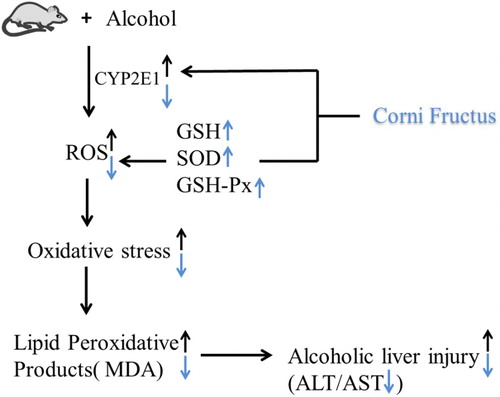
Introduction
Alcohol consumption is one of the major risk factors for liver damage. Alcoholic liver injury (ALI) is a major cause of morbidity and mortality in industrialized and developing countries, especially China [Citation1,Citation2]. ALI causes a series of changes, progressing from steatosis to hepatitis, fibrosis, cirrhosis, and finally hepatocellular carcinoma [Citation2–4]. Acute alcohol consumption can result in fatty liver disease, which is associated with the induction of the cytochrome P450 2E1 (CYP2E1) enzyme [Citation5]. Alcohol intake increases the expression and activity of this enzyme, which then accelerates the metabolism of excessive alcohol and results in the generation of reactive oxygen species (ROS) [Citation6]. Binge alcohol consumption (>60 g/d) can cause acute alcoholic liver injury (AALI) [Citation7]. Excessive ROS production caused by alcohol consumption can adversely affect proteins, lipids, and DNA [Citation8], leading to hepatocyte damage. Previous studies showed that ALI was associated with an increase in alcohol-induced CYP2E1 activity, which caused oxidative stress and lipid peroxidation in ALI models [Citation9,Citation10].
There are no efficacious therapeutic modalities to halt or reverse the pathogenesis and progression of ALI. Herbal medicines and associated active compounds have attracted increased attention as potential agents against ALI because of their multi-target actions, reduced toxicity, and few side effects [Citation1]. Corni fructus (C. fructus), obtained from the pulp of ripe fruit of Cornus officinalis Sieb. EtZucc, is one of the most common traditional Chinese medicines [Citation11]. The use of C. fructus as a treatment to invigorate the liver and kidney dates back to the Qin dynasty [Citation12]. Recent pharmacological studies have suggested that C. fructus has a wide range of biological activities, such as antioxidative, anti-inflammatory, immuno-modulatory, antidiabetic, and hypoglycemic [Citation13,Citation14]. Research has also suggested that it may be used to treat male infertility. A recent study reported that C. fructus prevented acetaminophen(APAP)-induced hepatotoxicity by inhibiting or alleviating oxidative stress [Citation15]. Thus, the antioxidant activity of C. fructus could play an important role in protecting against ALI.
This study aimed to evaluate the protective effects of C. fructus methanolic extract against acute alcohol-induced liver injury and to explore the potential mechanisms underlying its effects.
Materials and methods
Preparation of C. fructus methanolic extract
C. fructus fruits were collected from Huayang, Shaanxi, in September 2014 and authenticated by Prof Xiaoying Zhang (Northwest A&F University, China) and Associate Prof Chen Chen (Shaanxi Sci-Tech University, China). The fruits of C. fructus (10 g) were crushed and mixed with 200 mL of methanol and boiled for 2 hours. The extract was then collected. Residue biomass was extracted with 100 mL of methanol. The two extracts were combined, lyophilized, and stored at 4°C until the analyses.
Total flavonoids and phenols of C. fructus methanolic extract
The total phenolic content of the extract was measured using the Folin–Ciocalteu method [Citation16]. Briefly, 0.5 mL of C. fructus extract, 0.5 mL of Folin–Ciocalteu reagent, and 1.5 mL of 20% Na2CO3 solution were mixed in a 10 mL test tube. The mixture was diluted with distilled water to 10 mL and incubated at 75°C for 10 minutes. The absorbance of the mixture was measured using a spectrophotometer at 760 nm. A standard curve for the total phenol content was prepared using gallic acid, and the total phenolic content was expressed as milligrams of gallic acid equivalents per gram of dried C. fructus extract.
The total flavonoid content was determined using the method described by Liu et al [Citation17]. Briefly, 0.5 mL of C. fructus extract, 0.3 mL of 5% Na2NO2, and 0.3 mL of 10% AlCl3-methanol solution were mixed in a 10 mL test tube. After 6 minutes, 2 mL of NaOH (1 M) solution were added. The mixture was then diluted with a 50% methanol solution to 10 mL and allowed to stand for 10 minutes at room temperature. The absorption value was measured using a spectrophotometer at 510 nm. A standard curve for total flavonoids was prepared using rutin, and the total flavonoid content was expressed as milligrams of rutin equivalents per gram of dried C. fructus extract.
2, 2-Diphenyl-1-picryhydrazyl radical scavenging assay
2, 2-Diphenyl-1-picryhydrazyl (DPPH) radical scavenging activity was measured as previously described [Citation18]. Briefly, 1 mL of different concentrations of C. fructus extract (10, 50, 100, 200, 400, 600, and 1000 µg/mL) was mixed with 1 mL of methanolic solution containing DPPH radicals. The mixture was shaken vigorously and left to stand for 30 minutes in the dark. The DPPH absorbance was then measured at 517 nm. All samples were analyzed in triplicates. N-acetyl-l-cysteine (NAC) was used as the positive control. The percentage scavenging effect was calculated as
where
was the absorbance of the DPPH radicals,
was the absorbance of C. fructus/NAC, and
was the absorbance of C. fructus/NAC without the DPPH radicals.
Animals and experimental design
In total, 36 adult female Kunming mice weighing 22 ± 2 g were purchased from the Experimental Animal Center of the Fourth Military Medical University (Xi’an, China). The animals were housed under normal environment conditions, with free access to pelleted food and drinking water, and they were maintained under 12-hour dark/light cycles in polypropylene cages. The experimental procedures were carried out in accordance with the university’s guidelines for the care and use of laboratory animals.
The mice were randomly divided into six groups of six mice each. Group 1 served as the normal control (NC) group. Group 2 was the model group and treated with 50% alcohol (10 mL/kg, 4 g/kg). Groups 3, 4, and 5 were the experimental groups and treated with alcohol (10 mL/kg) and low (50 mg/kg; LCF + alcohol), moderate (100 mg/kg; MCF + alcohol), and high (200 mg/kg; HCF + alcohol) doses of C. fructus. Group 6 served as the positive control and was treated with both alcohol (10 mL/kg) and 50 mg/kg of silybin (positive drug of hepatoprotective effect, SI + alcohol). The mice were administered the alcohol, followed by C. fructus (dissolved in distilled water) an hour later, twice a day by oral gavage for three consecutive days. The experimental animals were sacrificed after the final alcohol and/or C. fructus administration. The serum and liver tissue were stored until experiment.
Serum aspartate aminotransferase and alanine aminotransferase measurements
Serum alanine aminotransferase (ALT) and aspartate aminotransferase (AST) activities were determined using commercial kits (Nanjing Jiancheng Bioengineering Institute, Nanjing, China), according to the manufacturer’s instructions.
Antioxidant and lipid peroxidation analyses
The liver tissue homogenate was prepared in ice-cold saline using an automatic homogenate machine and then centrifuged at 626g for 15 minutes. The hepatic levels of biochemical markers (total superoxide dismutase [T-SOD], glutathione peroxidase [GSH-Px], glutathione [GSH], hydroxyl radicals [•OH], and thiobarbituric acid reactive species [TBARS]), in the supernatant were measured using commercial detection kits (Nanjing Jiancheng Bioengineering Institute, Nanjing, China), according to the manufacturers’ instructions. The hydroxyl radical assay was based on the Fenton reaction, and the content of •OH hydroxyl radicals was determined by the color shade. The protein concentration of the tissue homogenates was measured using the Bradford protein assay, with bovine serum albumin as the standard (Tiangen Biotech, Beijing, China).
CYP2E1 protein expression and activity
A microsomal fraction was prepared according to the method of Jiang et al. [Citation19]. The protein concentration was determined using the Bradford protein assay, with bovine serum albumin as the standard (Tiangen Biotech, Beijing, China).
The microsome preparation was mixed with a loading buffer and boiled for 10 minutes. The sample mixture was run on 12% SDS–PAGE gel and transferred to a polyvinylidene fluoride membrane. Gel electrophoresis was conducted at 200 mA for 40 minutes. The membranes were blocked for 2 hours at room temperature with TBST containing 5% skimmed milk powder. Subsequently, the blocked membranes were incubated with rabbit polyclonal anti-CYP2E1 (1:800; Wuhan Boster Biological Technology, Wuhan, China) and mouse polyclonal anti-β-actin antibodies (1:800; Boster Biological Technology, Wuhan, China) overnight at 4°C. After incubation with the primary antibodies, the membranes were washed three times with TBST. HRP-conjugated goat anti-rabbit or goat anti-mice antibody (1:2000; Tianjin Sungene Biotech, Tianjin, China) was then added, followed by incubation for 1 hour at 37°C. After incubation, the membranes were washed three times with TBST. Signal detection was carried out using chemiluminescence detection reagents (Advansta, CA, U.S.A) and a Chemi Doc Imaging System (Tanon-3500, Shanghai, China).
The CYP2E1 activity of the liver microsome was determined by colorimetrically measuring the conversion (hydroxylation) of p-nitrophenol to 4-nitrocatechol, a reaction that is catalyzed specifically by CYP2E1. Briefly, the hepatic microsome was incubated in an incubation system for 30 minutes at 37°C. After terminating the reaction by adding 100 µL of cold 20% trichloroacetic acid, the samples were centrifuged to pellet the debris. The resulting supernatant was mixed with NaOH to produce a color reaction, and the absorbance was measured at 535 nm. A standard curve of 4-nitrocatechol was established to determine the quantity of 4-nitrocatechol in the CYP2E1-dependent oxidation reaction. The activity of CYP2E1 was expressed by generation of 4-nitrocatechol (nmol × min−1 × mg−1 protein).
Histological study
Liver samples were embedded in paraffin, sectioned at 5 µm thickness, and stained with hematoxylin and eosin for histopathological examination, as described previously [Citation20].
Statistical analysis
All the data were expressed as the mean ± standard deviation and analyzed using a one-way analysis of variance procedure in SPSS 19.0 (SPSS, Inc., Chicago, IL, U.S.A). A value of P < 0.05 was considered statistically significant.
Results
Total phenolic and total flavones of C. fructus extracts
The values of standard gallic acid and rutin showed an excellent linear relation, with y = 16.049x + 0.099 (R2 = 0.9923), and y = 3.9471x + 0.0082 (R2= 0.9996), respectively. The extract was rich in phenolic content (153.88 ± 1.26 mg/g) and total flavones (51.69 ± 0.66 mg/g).
DPPH scavenging activity
The color of the DPPH decreased following the addition of the antioxidants, and DPPH absorbance gradually declined with time. The IC50 values of the C. fructus extract and NAC were 90.98 ± 0.35 µg/mL and 85.86 µg/mL, respectively. The DPPH free radical scavenging activity of the C. fructus extract was similar to that of NAC ().
Effect of C. fructus on serum AST and ALT activities
Compared with the NC group, the serum ALT and AST levels were significantly elevated (2.7- and 1.5-fold, respectively) in the alcohol group (). The levels of serum ALT and AST were markedly decreased in LCF + alcohol, MCF + alcohol, and HCF + alcohol group.
Figure 2. Effects of C. fructus on serum ALT and AST activities.
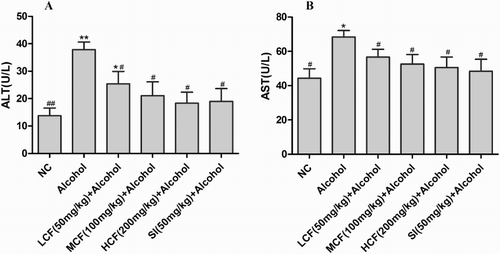
Effect of C. fructus on hepatic lipid peroxidation
In the alcohol group, the production of lipid peroxidation was significantly increased compared to the NC group. However, lipid peroxidation was inhibited in the LCF + alcohol, MCF + alcohol, and HCF + alcohol group, as shown by a decrease in TBARS ().
Figure 3. Effect of C. fructus on TBARS.
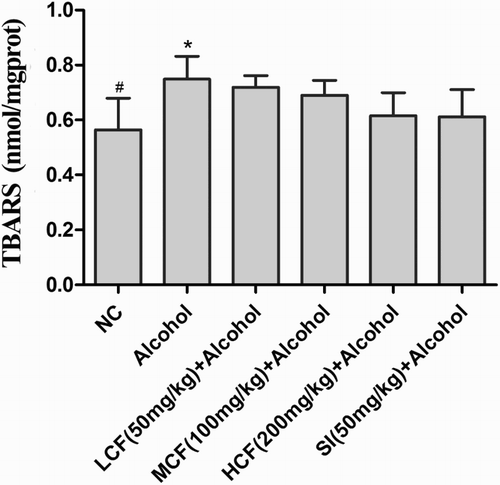
Effect of C. fructus on levels of •OH hydroxyl radicals
The inhibition of •OH hydroxyl radicals was evidently decreased in the alcohol group compared to the NC group (). With an increase dose of C. fructus treated, the inhibition of •OH hydroxyl radicals was significantly increased (HCF + alcohol group) compared to the alcohol group.
Figure 4. Effect of C. fructus on the inhibition of free hydroxyl radicals.
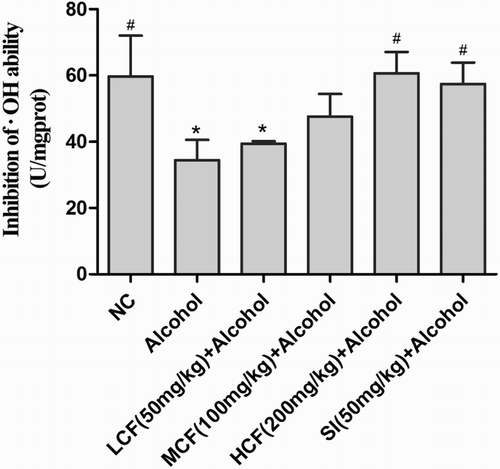
Effect of C. fructus on antioxidative defense
The activities of SOD and GSH-Px and the level of GSH were significantly reduced in the alcohol group (P < 0.05). In contrast, the activities of SOD and GSH-Px and levels of GSH were increased in the C. fructus-treated groups ().
Figure 5. Effect of C. fructus on GSH-Px (A), T-SOD (B), and GSH (C).
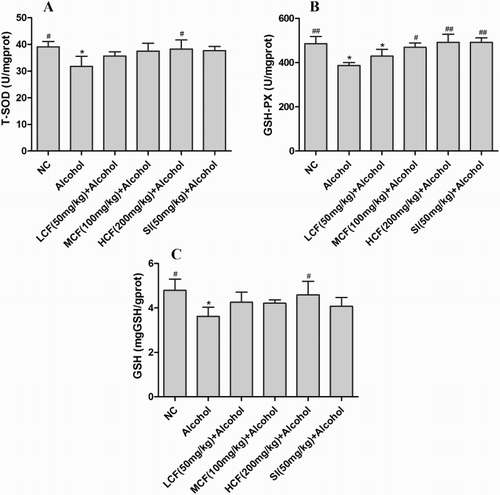
Effect of C. fructus on CYP2E1 protein expression and activity
The protein expression and activity of CYP2E1 were noticeably increased in the alcohol group (P < 0.01). In contrast, the protein expression (61.61–80.71%) and activity (33.93–45.94%) of CYP2E1 were inhibited in the C. fructus-treated groups ().
Figure 6. Effect of C. Fructus on CYP2E1 activity (A) and protein expression (B).
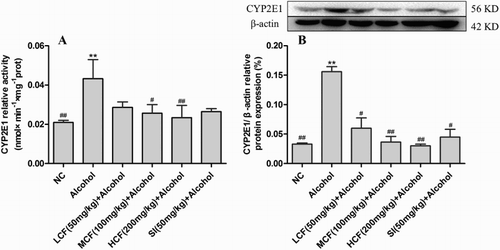
Effect of C. fructus on alcohol-induced hepatic histopathological changes
As shown in the photomicrograph in the figure, the architecture of the liver tissue obtained from the NC group and HCF + alcohol group appeared normal. In contrast, the architecture of the liver tissue in the alcohol group had an abnormal appearance: The spaces between the hepatic sinusoids appeared to be increased. The liver tissue also contained tiny cavities, and the liver cells showed hepatic steatosis, suggesting that the liver cells were severely damaged. However, the alcohol-induced abnormalities in the architecture of the liver tissue were clearly reversed in accordance with an increase in the dose of C. fructus. Thus, the spaces between the hepatic sinusoids were decreased, and the structure of the liver cells was intact (MCF + alcohol and HCF + alcohol group) ().
Figure 7. Effect of C. fructus on alcohol-induced histopathological changes in the livers of mice (original magnification 400×).
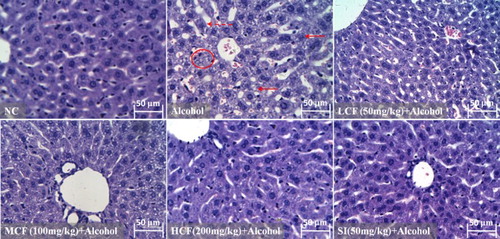
Discussion and conclusions
AST and ALT are semiotic markers of hepatocyte damage and reflect the severity of liver injury. The present study demonstrated that C. fructus (50, 100, 200 mg/kg body weight) provided significant protection against AALI by suppressing alcohol-induced increases in serum AST and ALT levels (). The histopathological examination suggested that C. fructus reversed alcohol-induced abnormalities in liver morphology, pointing to the potential of C. fructus in AALI interventions.
Numerous studies have demonstrated the association of alcohol-induced hepatotoxicity with oxidative stress and lipid peroxidation [Citation21,Citation22]. Lipid peroxidation is a primary marker of oxidative injury, resulting in structural and/or functional membrane damage, and TBARS is an important product of lipid peroxidation, which is formed when ROS attack polyunsaturated fatty acids [Citation23]. The present study demonstrated that alcohol administration significantly increased the TBARS content (0.75 nmol/mg protein) in mice but that it decreased to nearly normal levels (0.62 nmol/mg prot) following the treatment containing the C. fructus extract ().
Binge alcohol consumption can result in overproduction of ROS, such as OH−, superoxide anion radicals, and hydrogen peroxide, all of which strongly contribute to ALI [Citation7]. Previous research reported that oxidative stress was induced by elevated levels of ROS and that this affected antioxidant defense by SOD, GSH-Px, and GSH [Citation24]. In the current study, C. fructus enhanced antioxidant defense in vivo by enhancing the activities of SOD (12–20%) and GSH-Px (11–27%) and the level of GSH in the alcohol-treated groups (). Previous studies showed that flavonoids, phenols, and polysaccharides of C. fructus possessed strong free radical scavenging activity in terms of DPPH reduction (59.2, 81.2, and 89.9%, respectively) [Citation17,Citation25,Citation26], similar to the findings of the present study (80.12%). In the current study, both the in vitro and in vivo data demonstrated that C. fructus possessed strong antioxidant effects and radical scavenging ability.
CYP2E1 is an important metabolic enzyme, which catalyzes the oxidation of exogenous and endogenous compounds, and it plays a significant role in the metabolism of alcohol by the liver. Thus, it is particularly relevant to the development of ALI caused by the generation of alcohol-induced ROS [Citation27]. Previous studies showed that the administration of CYP2E1 inhibitors ameliorated acute alcohol-induced oxidative stress and lipid accumulation in CYP2E1 knockout mice [Citation5,Citation28]. A similar study showed that a wide range of natural products and their active substances possessed strong CYP2E1 inhibitory abilities [Citation10]. The results of the present study revealed that C. fructus significantly inhibited CYP2E1 expression and activity. Previous studies suggested that C. fructus contained many active components, such as flavones (quercetin and kaempferol), organic acids, phenolic acids (gallic acid and tannic acid), iridoid glycosides, saponins, and other chemical components [Citation29,Citation30]. In the present study, the methanolic extract of C. fructus is rich in phenols (153.88 ± 1.26 mg/g) and flavones (51.69 ± 0.66 mg/g). A previous study reported that the one of major polyphenol, gallic acid significantly decreased the expression of CYP2E1 in rat liver tissue in carbon tetrachloride-induced chronic liver injury [Citation31]. The flavonoid kaempferol was also reported to inhibit CYP2E1 expression and protect against alcohol-induced liver injury in mice [Citation10]. The results of the current study suggested that down-regulation of CYP2E1 (61.61–80.71% of protein expression and 33.93–45.94% of activity, ) was a potential mechanism underlying the activity of C. fructus in reducing alcohol-induced oxidative stress. The results also indicated that flavonoids and phenolic substances may play an important role in inhibiting CYP2E1 expression and activity.
In conclusion, C. fructus significantly ameliorated AALI by enhancing antioxidative defenses. C. fructus could be an effective therapeutic agent for ALI by inhibiting the activity and expression of CYP2E1 and inhibiting oxidative stress.
Acknowledgement
Contributors Zhihui Jiang designed and performed the study, wrote the manuscript, Jian Wang performed study, analyzed data, Meng Wang and Huiting Xue analyzed data, Hai Jiang, Yinku Liang and Alberto C. Dias designed study and organized discussion; Marslin Gregory proof read the manuscript; Chen Chen and Xiaoying Zhang designed the study, organized discussion, and revised the manuscript.
Ethics approval The protocol of this study was approved by the institutional review board for care and use laboratory animals of Shaanxi Sci-Tech University.
Disclosure statement
No potential conflict of interest was reported by the authors.
Notes on contributors
Zhihui Jiang is studying in Agriculture & Forestry University (PhD student), China. Her research area is pharmacology of traditional Chinese medicine.
Jian Wang graduated from Shaanxi Sci-Tech University (postgraduate) in Pharmacology, China. His research area is pharmacology of traditional Chinese medicine.
Huiting Xue is studying in Xinjiang Agriculture University (master student). Her research area is ethnopharmacology.
Dr Meng Wang graduated from Northwest Agriculture & Forestry University (PhD), China. Her research area is ethnopharmacology.
Associate Prof. Hai Jiang has been appointed as associate professor at Shaanxi Sci-Tech University, China. His research area is natural product research.
Associate Prof. Yinku Liang has been appointed as associate professor at Shaanxi Sci-Tech University, China. His research area is natural product research.
Prof. Alberto C. Dias has been appointed as professor at University of Minho, Portugal. His research area is natural product research.
Associate Prof. Marslin Gregory has been appointed as associate professor at Shaanxi Sci-Tech University, China. He graduated from University of Minho, Portugal. His research area is natural product research.
Associate Prof. Chen Chen has been appointed as associate professor at Shaanxi Sci-Tech University, China. His research area is natural product research.
Prof. Dr Xiaoying Zhang has been appointed as professor at Northwest Agricultural & Forestry University, China since 2008. He graduated from Charité – Universitätsmedizin Berlin (postgraduate and PhD) in Pharmacology.
Additional information
Funding
References
- Ding R-B, Tian K, Huang L-L, et al. Herbal medicines for the prevention of alcoholic liver disease: a review. J Ethnopharmacol. 2012;144(3):457–465. doi: 10.1016/j.jep.2012.09.044
- Mathurin P, Bataller R. Trends in the management and burden of alcoholic liver disease. J Hepatol. 2015;62(1):S38–S46. doi: 10.1016/j.jhep.2015.03.006
- Altamirano J, Bataller R. Alcoholic liver disease: pathogenesis and new targets for therapy. Nat Rev Gastro Hepat. 2011;8(9):491–501. doi: 10.1038/nrgastro.2011.134
- Cao Y-W, Jiang Y, Zhang D-Y, et al. Protective effects of Penthorum Chinese Pursh against chronic ethanol-induced liver injury in mice. J Ethnopharmacol. 2015;161:92–98. doi: 10.1016/j.jep.2014.12.013
- Chen Y-Y, Zhang C-L, Zhao X-L, et al. Inhibition of cytochrome P450 2E1 by chlormethiazole attenuated acute ethanol-induced fatty liver. Chem Biol Interact. 2014;222:18–26. doi: 10.1016/j.cbi.2014.08.009
- Jin M, Ande A, Kumar A, et al. Regulation of cytochrome P450 2E1 expression by ethanol: role of oxidative stress-mediated pkc/jnk/sp1 pathway. Cell Death Disease. 2013;4(3):e554. doi: 10.1038/cddis.2013.78
- O’Shea RS, Dasarathy S, McCullough AJ. Alcoholic liver disease. Hepatology. 2010;51(1):307–328. doi: 10.1002/hep.23258
- Wang ZG, Su B, Fan S, et al. Protective effect of oligomeric proanthocyanidins against alcohol-induced liver steatosis and injury in mice. Biochem Bioph Res Co. 2015;458:757–762. doi: 10.1016/j.bbrc.2015.01.153
- Stice CP, Liu C, Aizawa K, et al. Dietary tomato powder inhibits alcohol-induced hepatic injury by suppressing cytochrome p450 2E1 induction in rodent models. Arch Biochem Biophys. 2015;572:81–88. doi: 10.1016/j.abb.2015.01.004
- Wang M, Sun J, Jiang Z, et al. Hepatoprotective effect of kaempferol against alcoholic liver injury in mice. Am J Chin Med. 2015;43(2):241–254. doi: 10.1142/S0192415X15500160
- Zhang LT, Yuan ZF, Du YF, et al. Recent development and prospect of Cornus officinalis. Chinese Tradit Herb Drugs. 2004;35:952–958.
- Yang JF, Lu FP, Gao WY, et al. Research and development of chemical compositions and pharmacology of Cornus officinalis. Prog Mod Biomed. 2006;6(12):127–130.
- Zhou JH, Li CS, Li DD. Progress in research of the active chemical components of Cornus officinalis. Chinese J New Drugs. 2001;10:809–812.
- Yu M, Wang XX. Progress in pharmacological study of cornel. Mil Med J Southeast China. 2010;12:240–243.
- Lee NH, Seo CS, Lee HY, et al. Hepatoprotective and antioxidative activities of Cornus officinalis against acetaminophen-induced hepatotoxicity in mice. Evid-Based Compl Alt. 2012;2012:804924.
- Cao YP, Dai HZ, Cao W, et al. Determination of total phenols in Zizyphus jujuba Mill by Folin-Ciocalteu colorimetry. J Anhui Agri Sci. 2008;36:1299–1302.
- Liu HY, Qiu NX, Yao RQ, et al. Correlation analysis between antioxidant capacity and total phenolic content of 86 Chinese edible herbal extracts. J Northwest A&F Univ (Nat Sci Ed). 2009;37:173–180.
- Wang H, Gao XD, Zhou GC, et al. In vitro and in vivo antioxidant activity of aqueous extract from Choerospondias axillaris fruit. Food Chem. 2008;106(3):888–895. doi: 10.1016/j.foodchem.2007.05.068
- Jiang Z, Chen C, Wang J, et al. Purple potato (Solanum tuberosum L.) anthocyanins attenuate alcohol-induced hepatic injury by enhancing antioxidant defense. J Nat Med. 2016;70(1):45–53. doi: 10.1007/s11418-015-0935-3
- Jiang ZH, Wang J, Li XP, et al. Echinacoside and Cistanchetubulosa (Schenk) R. Wight ameliorate bisphenol A-induced testicular and sperm damage in rats through gonad axis regulated steroidogenic enzymes. J Ethnopharmacol. 2016;193:321–328 doi: 10.1016/j.jep.2016.07.033
- Arteel GE. Oxidants and antioxidants in alcohol-induced liver disease. Gastroenterology. 2003;124(3):778–790. doi: 10.1053/gast.2003.50087
- Yan SL, Yang HT, Lee HL, et al. Protective effects of maslinic acid against alcohol-induced acute liver injury in mice. Food Chem Toxicol. 2014;74:149–155. doi: 10.1016/j.fct.2014.09.018
- Yoshida Y, Umeno A, Shichiri M. Lipid peroxidation biomarkers for evaluating oxidative stress and assessing antioxidant capacity in vivo. J Clin Biochem Nutr. 2013;52(1):9–16. doi: 10.3164/jcbn.12-112
- Zhao J, Chen H, Li Y. Protective effect of bicyclol on acute alcohol-induced liver injury in mice. Eur J Pharmacol. 2008;586(1–3):322–331. doi: 10.1016/j.ejphar.2008.02.059
- Zhang YP, You YR, Dai ZY. Study on scavenging radicals capability and antioxidant activity of polysaccharides from Cornus officinalis Seib.et Zucc. J Chinese Inst Food Sci Technol. 2008;8:18–22.
- Qiao S, Li C, Wang LF, et al. Analysis on content of polysaccharides of Fructus Corni. J LiaoNing Univ TCM. 2010;12:238.
- Lu Y, Cederbaum AI. CYP2E1 and oxidative liver injury by alcohol. Free Radical Bio Med. 2008;44(5):723–738. doi: 10.1016/j.freeradbiomed.2007.11.004
- Lu Y, Zhuge J, Wang X, et al. Cytochrome P450 2E1 contributes to ethanol-induced fatty liver in mice. Hepatology. 2008;47(5):1483–1494. doi: 10.1002/hep.22222
- Kim DK, Kwak JH. A furan derivative from Cornus officinalis. Arch Pharm Res. 1998;21:787–789. doi: 10.1007/BF02976779
- An YA, Hwang JY, Lee JS, et al. Cornus officinalis methanol extract upregulates melanogenesis in melan-a cells. Toxicol Res. 2015;31(2):165–172. doi: 10.5487/TR.2015.31.2.165
- Tung YT, Wu JH, Huang CC, et al. Protective effect of Acacia confusa bark extract and its active compound gallic acid against carbon tetrachloride-induced chronic liver injury in rats. Food Chem Toxicol. 2009;47(6):1385–1392. doi: 10.1016/j.fct.2009.03.021