ABSTRACT
Objectives: Catechins are important components of human diet and have received special attention due to their antioxidant capacity. The purpose of this paper was to study the antioxidant action of (+)-catechin (CTQ) in the presence of vitamin B2 (riboflavin) as light-absorbing agent. Furthermore, two model compounds, catechol (CTC) and resorcinol (RSC), were selected in order to elucidate the reactive target of the CTQ molecule. The influence of pH-medium was investigated.
Methods: Stationary photolysis, polarographic detection of dissolved oxygen, reactive oxygen species (ROS)-scavengers, time-resolved near-IR phosphorescence detection, stationary, and time-resolved fluorescence and laser flash photolysis techniques were employed.
Results: CTQ interacts with riboflavin under visible-light photoirradiation as well as with different ROS which are generated in this mechanism. Radical-scavenging activity increases with increasing of pH-medium.
Discussion: pH-effect of the medium on radical-scavenging activity comes from the increased electron-donating ability of CTQ upon deprotonation. These results are very interesting due to the fact that the pH of the food products displays important variations.
The -scavenging ability of CTQ, would be equal to the additive contribution of each reactive center, CTC, and RSC, present at the molecule of CTQ. However, CTQ would have a moderate ability to removal of
-species at pH 7.
Introduction
A matter of great concern in the food industry world, not only for manufacturers but also for consumers, is the preservation of food products. It is quite important to maintain both their good quality as well as their fine organoleptic properties. One of the most relevant causes of spoilage and loss of their good characteristics is due to oxidation of some of their components, mainly lipids. In these processes, reactive oxygen species (ROS) plays a key role.Citation1,Citation2 Light exposure is known to be one of the agents that can initiate oxidation of compounds like proteins, which result in a ‘burnt feather’ off-flavor of the product.Citation3 Most food components are colorless and do not absorb light but the oxidation processes are started by the participation of dyes or pigments (photosensitizers) as essential links. A light absorber widely and commonly present in foods like dairy products, meat, eggs, and vegetables is vitamin B2 or riboflavin (Rf). The presence of Rf in foods is important for the assurance of good nutrition, but has the drawback to enhancing the photooxidation processes by the production of different ROS. Particularly, the dairy products are quite susceptible to suffer Rf-sensitized degradation, due to their high content of this vitamin.
Industry has applied many strategies in order to avoid this drawback. In the last decades, the use of natural reducing agents – commonly referred to as antioxidants – is one of the most popular ones. Nowadays, polyphenols are the most abundant antioxidants present in foods. Among them, flavonoids can be mentioned. These are secondary plant metabolites with antioxidant activity and are potentially protective compounds. They can lower the risk of many diseases like cardiovascular problems and many cancers.Citation4 Flavonoids are the most abundant polyphenols in our diets. They can be divided into several classes according to the degree of oxidation of the oxygen heterocycle. One of the six classes of flavonoids are catechins, a group of compounds which now are receiving more interest not only because of their good antioxidant activity but also for their wide distribution in fruits, vegetables, legumes, and many derivatives as red wine, tea and chocolate.Citation5–7 They act as effective antioxidants because of their free radical-scavenging and metal-chelating capacity.Citation8,Citation9 In order to play their specific role, most of the interaction ROS-antioxidant must occur by physical inactivation. In this way, ROS can be removed leaving the antioxidant available to continue with its protective action. On the other hand, it was reported that the radical-scavenging capacity of the catechins is pH-dependent and it increases with increasing pH of the medium.Citation10 This is particularly interesting due to the fact that the pH of different human body fluids varies from pH 1 in the stomach to pH 8.6 in the pancreas.Citation11,Citation12 Furthermore, the pH of food products that may contain catechins as antioxidants also exhibits significant variations.Citation9
There is a great interest in studying if catechins are able to maintain their antioxidant action in the presence of light-absorbing agents as Rf because they can be found in the same microenvironment, mainly in products like flavored milk-based beverages. For this reason, the aim of this study was to mimic a picture where a photosensitizer and a potentially uncolored antioxidant are simultaneously present in a given food product.
In the present work, we investigated the capacity of deactivation by (+)-catechin (CTQ) of Rf-electronically excited states and the CTQ antioxidant ability against ROS photogenerated from these states. Furthermore, two model compounds catechol (CTC) and resorcinol (RSC) were selected, in order to elucidate the reactive target of the CTQ molecule. The dye Rose Bengal (RB) was used as auxiliary photosensitizer. Besides, the influence of the pH-medium was studied upon ROS – scavenging capacity by CTQ, CTC, and RSC. From here on, these substrates will be generically denoted as dCTQ.
Scheme 1 represents a simplified and self-defined reaction sequence of the photosensitizing processes that may be taking place for dCTQ in the presence of photosensitizer (S).
Scheme 1. Possible pathways involved in the photosensitized oxidation of CTQ, CTC, and RSC. S represents the photosensitizer and dCTQ denotes generically to the substrates CTQ, CTC, and RSC.
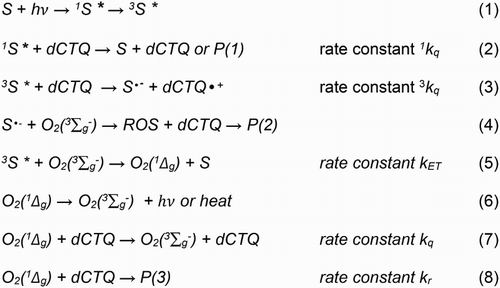
On the basis of this scheme, a systematic kinetic study was carried out in order to evaluate and characterize the nature and mechanism involved, and get some light on the possibility of these processes to take place and evaluate the efficiency of the antioxidant action of CTQ to preserve food quality during manufacture and storage.
Experimental
Selected compounds
To study the behavior of catechins as model antioxidant compound CTQ, the simplest compound of flavonol family and the most abundant in fruits, was used. In order to relate reactivity with structure two compounds, CTC and RSC were also tested. The chemical structure of these substrates is presented in Scheme 2. As photosensitizer not only Rf was used but also experiments using RB were set up. It is a common artificial dye employed in many food products.Citation13,Citation14
Chemicals
CTQ, Rf, RB, sodium azide (NaN3), catalase from bovine liver (CAT), superoxide dismutase from bovine erythrocytes (SOD), and D-Mannitol were purchased from Sigma Chem. Co (USA). Deuterated water 99.9% (D2O) and furfuryl alcohol (FFA) were provided by Sigma-Aldrich (USA). CTC and RSC, from Fluka and Merck, respectively; NaH2PO4 and KOH, from Ciccarelli.
Bidistilled water was obtained from Laboratorios de la Unidad Académica de Río Gallegos, UNPA.
Sample preparation
The buffer solutions were prepared with NaH2PO4 10−2 M and adjusted to pH 7 or pH 9 using KOH 0.1 M. For stationary-photolysis experiments, each of them was used to prepare Rf solutions with an absorbance of 0.5 at 445 nm. Similarly, RB solutions were prepared with absorbance of 0.5 at 556 nm. In both cases, the selected wavelength was the maximum absorbance of the visible-band of each photosensitizer. Meanwhile, for time-resolved experiments, the photosensitizer solutions were prepared with absorbance of 0.3 at the excitation wavelength of the laser pulse (532 nm for RB and 355 nm for Rf). All measurements were carried out with freshly prepared solutions.
Instrumentation and methods
Stationary photolysis
Stationary aerobic photolysis of aqueous solutions containing the photosensitizer (Rf or RB) plus the substrates (dCTQ) was carried out employing a home-made photolyzer. Light from 150 W quartz-halogen lamp was focused on the reaction vessel containing an Orion 081010 oxygen electrode with a PCMCIA interphase Orion Sensor Link PCM 800. An appropriate cut-off filter was used in order to ensure that the light was absorbed only by the photosensitizer. Solutions were continuously stirred. Experiments were performed in buffer solutions at pH 7 and pH 9.
Relative rates of oxygen consumption by dCTQ, in the presence of the photosensitizer (Rf or RB), were determined by evaluation of the initial slopes of graphs oxygen uptake vs. irradiation time.
In order to elucidate the nature of ROS involved in the dCTQ-Rf system, under visible-light irradiation, specific inhibitors of ROS were used. NaN3, SOD, CAT and D-Mannitol were utilized as scavengers of ,
, H2O2 and HO•, respectively.
Interaction of dCTQ with
For the purpose of investigating the stability of dCTQ in the presence of the species singlet oxygen (), the interactions between dCTQ and
were quantified through the reactive rate constants (kr) and the overall rate constant (kt) of deactivation of
. In these experiments, the synthetic photosensitizer RB was used as an exclusive
-generator.Citation12,Citation13
kr values were determined by a comparative method,Citation15 for which knowledge of the reactive rate constant (krR) for the -mediated photooxidation of a reference compound R is required. Assuming that the reaction of
with the substrates (dCTQ and R) is the only way of oxygen consumption, the ratio of the slopes of the first-order plot for oxygen consumption by dCTQ and R, under identical conditions, is equal to the ratio kr/krR. FFA was used as reference compound with a krR value of 1.2 × 108 M−1 s−1, in water (this value was determined in our laboratory). It is known that,Citation16 this krR value is pH-independent in the range 2–12. Conversions lower than 10% were employed in order to avoid possible interference from photoproducts.
kt, which takes into account the physical and reactive contribution to the overall process of -deactivation by dCTQ (kt = kq
+ kr, processes (7) and (8), Scheme 1), was determined by time-resolved phosphorescence detection of
at 1270 nm (TRPD).Citation17 Briefly, a Nd:YAG laser (Spectron Laser System, SL400) was used as excitation source. The output at 532 nm was employed to excite the photosensitizer RB. Experiments were made in D2O as solvent instead of H2O, in order to enhance the
-lifetime, as already discussed elsewhere.Citation18 The kt values were graphically obtained through Stern–Volmer treatment (Eq. (1))
(1) where τo and τ represent the
phosphorescence lifetime in the absence and in the presence of different dCTQ concentrations, respectively.
Interaction of dCTQ with electronically excited states of Rf
With the purpose of investigating the behavior of dCTQ in a medium containing Rf, under visible-light irradiation, the interaction between dCTQ and the electronically excited singlet (1Rf*) and triplet (3Rf*) states, was studied. The interaction dCTQ-1Rf* was estimated by measuring the intensity of stationary fluorescence of Rf at different concentrations of dCTQ, with a 5301-PC Shimadzu Spectrofluorometer. The excitation and emission wavelengths were 445 and 518 nm, respectively. All the measurements were made at 25 ± 1°C and quartz cells of 1.0 cm path-length were employed. Stern–Volmer treatment was applied in order to determine the values of (process (2), Scheme 1).
(2) where
is the fluorescence lifetime of Rf in the absence of dCTQ. For calculating the
values from the slopes of the Stern–Volmer graphs, a value of 4.9 ns was used for
.Citation19
On the other hand, the interaction dCTQ-3Rf* was studied by Laser Flash Photolysis experiments. The details of the equipment and methodology have been previously described.Citation18,Citation20 The lifetime of 3Rf* was evaluated in the absence and in the presence of different dCTQ concentrations. As before, a Stern–Volmer treatment was used and the magnitude of the interaction dCTQ-3Rf* was determined (, process (3), Scheme 1).
Results and discussion
Stationary photolysis
The photolysis of the system CTQ/Rf/air at λ > 360 nm, a zone where Rf is the only light-absorbing agent, produces major perturbations in the absorption spectrum of the mixture ( and ), with the appearance of new bands in 300–600 nm zone. This result indicates that both Rf and CTQ undergo chemical transformation under irradiation with visible-light and photoproducts are generated. Furthermore, as can be observed in , the changes in the spectra are more significant at pH 9 than at pH 7. Although similar results were found for CTC and RSC, the comparison of the spectral changes observed implies that each substrate produced different photoproducts .
Figure 1. Spectral changes of 0.4 mM CTQ + 0.02 mM Rf vs. 0.02 mM Rf upon visible-light photoirradiation, in buffer pH 7 (main figure) and in buffer pH 9 (insert). Numbers on the spectra represent irradiation time, in seconds.
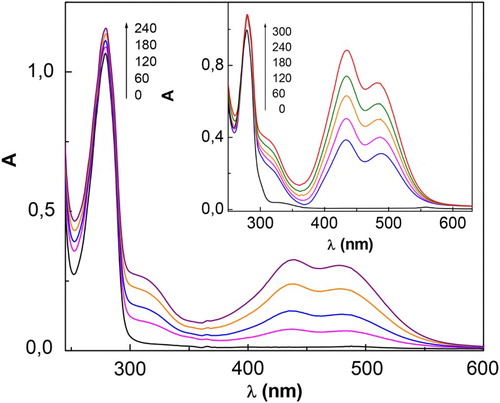
Figure 2. Spectral changes of 0.7 mM RSC + 0.02 mM Rf vs. 0.02 mM Rf, upon visible-light photoirradiation. Insert: spectral changes for 0.4 mM CTC + 0.02 mM Rf vs. 0.02 mM Rf, upon visible-light photoirradiation. Solvent: buffer pH 7. Numbers on the spectra represent irradiation time, in seconds.
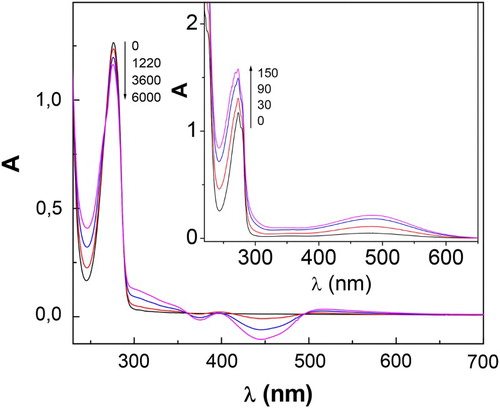
In parallel with dCTQ consumption, oxygen uptake has been observed at both pHs assayed. The experiments of oxygen consumption were also dependent on the pH of the environment (). It is known that in ROS-mediated reactions, the phenolate groups are more reactive than the non-ionized species.Citation16,Citation21 The reactivity of these three substrates is related to the acidity dissociation constants (pKa),Citation22–25 for CTQ are 8.64, 9.41, 11.26, and 13.26; for CTC are 9.25 and 13.0; for RSC are 9.32 and 9.81. So, the fact that the reactivity of dCTQ is higher in alkaline solutions than that observed at neutral pH is due to the fact that for all substrates a large percentage of molecules are ionized at pH 9.
Figure 3. Profiles of oxygen consumption, upon Rf-sensitized photoirradiation (a) CTC, (b) CTQ, and (c) RSC, in buffer pH 7 (main figure) and pH 9 (insert). [dCTQ] = 0.4 mM.
![Figure 3. Profiles of oxygen consumption, upon Rf-sensitized photoirradiation (a) CTC, (b) CTQ, and (c) RSC, in buffer pH 7 (main figure) and pH 9 (insert). [dCTQ] = 0.4 mM.](/cms/asset/9520f804-ec3a-4003-a3db-33f446cd0c52/yrer_a_1240287_f0003_c.jpg)
So far, results could be indicative that the interactions dCTQ-Rf include the participation of electronically excited states of Rf and/or ROS photogenerated from these states.
The rates of oxygen consumption by dCTQ in the presence of Rf or by adding RB instead, either at pH 7 or at pH 9, were used to get some insight into the mechanism of degradation. Results were expressed as relative oxygen consumption rates (vO2rel), an arbitrary value = 1 was given to the highest of them from each photosensitizer and pH triad. If the oxidation of these substrates took place through the same mechanism with Rf and RB, the values of v-O2 rel for both dyes would display a parallelism between all the substrates studied, but not found in this case. Those highest rates were the ones for RCS in presence of Rf, but when the photosensitizer was RB they were the ones for CTQ (). The results indicate that different sources of oxygen consumption must be operating for Rf and RB. So, the differences among these relative rates in the presence of Rf compared with the ones in presence of RB reveal the structure effect and indicate that different pathways might be taken place. In aqueous solution, upon visible-light irradiation both photosensitizers generate the species O2 with good quantum yieldsCitation12,Citation13; however, Rf can also generate other ROS and radical species.Citation19,Citation26–28
Table 1 Relative rates of oxygen consumption by CTQ, CTC, and RSC, sensitized by RB (A556 nm = 0.6) and 0.02 mM Rf. Solvents: buffer pH 7 and buffer pH 9.
Participation of ROS in the Rf-photosensitized oxidation of dCTQ
In order to investigate the nature of the ROS involved in the photodegradation of dCTQ in the presence of Rf, assays using different ROS-scavengers were performed. The initial slopes of oxygen uptake vs. irradiation time in the absence and in the presence of the scavengers were compared, as can be seen in , inset. Similar experiments have been formerly employed to confirm/discard the participation of ,
, H2O2 and HO•, respectively in a given oxidative process.Citation19,Citation29–33
Figure 4. Stern–Volmer plot for the quenching of O2 -phosphorescence emission by dCTQ: (a) CTQ, (b) CTC, and (c) RSC, upon RB-sensitized photoirradiation, in D2O pD 7. Inset: profiles of oxygen consumption, in buffer pH 7, upon Rf-sensitized photoirradiation (a) 0.02 mM Rf + 0.4 mM CTQ; (b) 0.02 mM Rf + 0.4 mM CTQ + 1 mg/100 ml SOD; (c) 0.02 mM Rf + 0.4 mM CTQ + 10 mM NaN3; (d) 0.02 mM Rf + 0.4 mM CTQ + 1 mg/100 ml CAT; (e) 0.02 mM Rf + 0.4 mM CTQ + 1.0 mM D-Mannitol (1.0 mM).
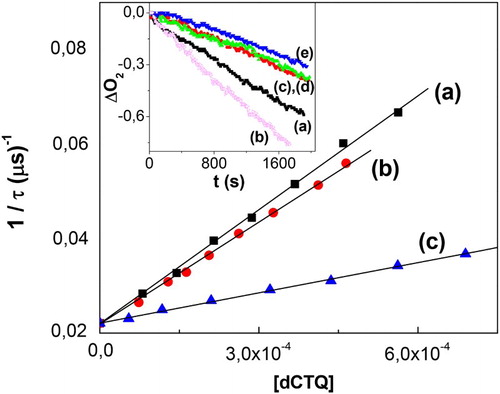
NaN3 was used as –scavenger. This salt is a physical quencher of
, as shown in the following reaction
NaN3 reacts with a rate constant of 3 × 108 M−1 s−1 in aqueous solution.Citation34 The rate of oxygen uptake by dCTQ was decreased in the presence of 10 mM NaN3 at both pH assayed, as can see in , inset for CTQ as typical example. Results strongly suggest the involvement of
in the Rf-photosensitized degradation of dCTQ.
On the other hand, the enzyme SOD was used as scavenger of . This enzyme eliminates the species
, through the next reaction
In the presence of 1 µg/ml SOD, the rate of oxygen consumption by dCTQ was increased regarding the rate in the absence of the scavenger, at pH 7 (, inset). However, at pH 9 the enzyme caused a delay in the oxygen uptake by CTQ and RSC, whereas the run in the presence of CTC was not affected. The increase and the delay observed, confirm the involvement of in the photooxidation process of dCTQ, sensitized by Rf. The former could be a consequence of the different stoichiometry of the involved processes, as has been reported for other phenolic compounds.Citation26
The enzyme CAT was employed as scavenger of H2O2. CAT decomposes H2O2 through the following reaction
Results showed that the rate of oxygen uptake by dCTQ was affected in the presence of 1 μg/ml CAT, indicating that H2O2 is involved in the Rf-sensitized degradation of dCTQ.
The carbohydrate D-Mannitol was used in order to evaluate the eventual presence of HO• in the dCTQ-photooxidation. The participation of this species was only detected in the case of CTQ, at both pH assayed. D-Mannitol reacts with the species HO• with a rate constant kq = 1.9 × 109 M−1 s−1, by the following reaction.Citation35
Results of the experiments with ROS-scavengers demonstrate that diverse ROS are implicating in the photooxidation of dCTQ, in the presence of Rf. Furthermore, results show that the pH of the environment has significant influence over these processes, suggesting that different mechanisms could be happening.
Interaction of dCTQ with
Due to the fact that the experiments with NaN3 confirmed the participation of in the photodegradation of dCTQ, in the presence of the vitamin Rf, the magnitude of the interaction
was evaluated. With this purpose the values of kr and kt were determined ( and ). Results show an important effect of medium pH on kr for all the substrates studied. As it was mentioned above, taking into account the pKa values, at pH 9, a great percentage of molecules of dCTQ would have the –OH group ionized. The high dependence of kr on the degree of ionization of –OH group has been reported.Citation28 In these cases, it is postulated the formation of an encounter complex substrate-
with partial charge-transfer character, a formation that is favored by the higher electron-donor ability of the ionized species. The deactivation of this complex could lead to physical quenching or produce the oxidation of the substrate by complete electron transfer.Citation36,Citation37
Table 2 Rate constants for the reactive (kr) and overall (kt) quenching of singlet oxygen by CTQ, CTC, and RSC; kr/kt ratios; rate constants for the quenching of electronically excited singlet state of Rf (1Kg); rate constants for the quenching of electronically excited triplet state of Rf (1Kg) × 2. Solvents: buffer pH 7 and buffer pH 9. kt values were determined in buffer pD 7.
In , an interesting structural effect on kr and kt can be observed. The values of these rate constants for CTQ are approximately equal to the additive contribution of each reactive center, CTC and RSC, at both pH assayed. This result is quite novel due to the fact that the photooxidative behavior of most systems cannot be interpreted on the basis of an additive effect of each particular substituent or reactive center. Consequently, in this type of study, it is necessary to investigate the behavior of each specific system. In general, the photodegradative conduct of a given system cannot be extrapolated in a general way to understand the behavior of other similar systems.Citation16,Citation20
In , the kr
/kt ratio at pH 7 for three substrates studied are also displayed. This ratio is a simple and useful approach, which can be interpreted as the fraction of the overall interaction that leads to effective chemical deactivation. These values show that CTQ has a component of
-chemical transformation higher than CTC and RSC. Nevertheless, it could be concluded, given the small value of kr
/kt for CTQ, this substrate would have a moderate ability to remove the
-species in an environment at pH 7.
Interaction of dCTQ with 1Rf* and 3Rf*
The eventual interaction of dCTQ with 1Rf* was investigated through measurements of steady state-fluorescence. The presence of dCTQ produced a decrease in the intensity of the emission of 1Rf*. From these data an estimate of the magnitude of the interaction dCTQ-1Rf* was performed (, ). Nevertheless, this interaction may be disregarded due to the short lifetime of 1Rf* (ca. 5 ns).Citation38,Citation39 Therefore these species cannot be intercepted by dCTQ at concentration range employed in our experiments (ca. 0.4 mM). Thereby, it can be assumed that the population of 3Rf* is almost not affected by any deactivation effect on 1Rf*, under our experimental conditions.
Meanwhile, in anaerobic conditions Rf photodegradation proceeds mainly through 3Rf*, the rate of this process can be estimated by a decrease in its characteristic absorption bands.Citation40 The irradiation of Ar-saturated aqueous solutions of Rf (0.06 mM) showed that the absorption of their visible-bands decreases in the absence of CTQ (not shown). However, in the presence of CTQ (0.4 mM), the absorption of these bands were increased, due to the appearance of photoproducts in the same spectral region of the visible-bands of Rf. Although the interaction CTQ-3Rf* could not be assessed from this experiment, this result clearly demonstrates that CTQ interacts with 3Rf*, upon anaerobic-photoirradiation conditions.
In order to quantify the magnitude of the interaction dCTQ-3Rf* Laser Flash Photolysis experiments were performed. Results showed that the lifetime of 3Rf* decrease in the presence of CTQ; the same occurred in the presence of CTC or RSC. values were determined, as described, from Stern–Volmer plots ().
Conclusions
The results herein presented show that CTQ interacts with the vitamin Rf under visible-light photoirradiation as well as with different ROS, which are generated in this mechanism. CTQ is a good protector against the oxidative action of ROS, like and
. As claimed before, these ROS are generated in food products by visible-light irradiation on compounds like Rf or colorants as RB that are part of their manufacture recipes. This is quite remarkable for dairy products or milk-based beverages which have a high content of vitamin B2 (Rf). The interaction of CTQ with Rf would result, indirectly, in a protective effect against the proteins present in food, which undergo oxidation in the presence of the vitamin Rf with the consequent appearance of off-flavors.
Furthermore, results show a significant influence of pH on the mechanism and the antioxidant capacity of CTQ. Radical-scavenging activity increases with increasing pH of the medium and this effect comes from the increased electron-donating ability of CTQ upon deprotonation. These results could be very interesting due to the fact that the pH of the food products, in which CTQ could act as antioxidant, displays important variations.
On the other hand, the -scavenging ability of CTQ, would be equal to the additive contribution of each reactive center, CTC and RSC, present at the molecule of CTQ.
Disclosure statement
No potential conflict of interest was reported by the authors.
ORCiD
M. G. Barua http://orcid.org/0000-0002-7341-4162
J. P. Escalada http://orcid.org/0000-0002-9822-9844
M. Bregliani http://orcid.org/0000-0002-0354-9424
A. Pajares http://orcid.org/0000-0003-4772-9184
References
- Rawls H, Van Santen P. A possible role for singlet oxygen in the initiation of fatty acid autoxidation. J Am Oil Chem Soc. 1970;47:121–125. doi: 10.1007/BF02640400
- Lee J, Koo N, Min DB. Reactive oxygen species, aging, and antioxidative nutraceuticals. Compr Rev Food Sci Food Saf. 2004;3:21–33. doi: 10.1111/j.1541-4337.2004.tb00058.x
- Miquel Becker EC, Cardoso DR, Skibsted LH. Deactivation of riboflavin triplet-excited state by phenolic antioxidants: mechanism behind protective effects in photooxidation of milk-based beverages. Eur Food Res Technol. 2005;221:382–386. doi: 10.1007/s00217-005-1184-6
- Higdon JV, Frei B. Tea catechins and polyphenols: health effects, metabolism, and antioxidant functions. Crit Rev Food Sci. 2003;43(1);89–143. doi: 10.1080/10408690390826464
- Medina I, Gallardo JM, Gonzalez MJ, et al. Effect of molecular structure of phenolic families as hydroxycinnamic acids and catechins on their antioxidant effectiveness in minced fish muscle. J Agric Food Chem. 2007;55:3889–3895. doi: 10.1021/jf063498i
- Van de Putte B, Hollman PCH. Catechin contents of foods commonly consumed in the Netherlands. 1. Fruits, vegetables, staple foods, and processed foods. J Agric Food Chem. 2000;48:1746–1751. doi: 10.1021/jf000025h
- Van de Putte B, Hollman PCH. Catechin contents of foods commonly consumed in the Netherlands. 2. Tea, wine, fruit juices, and chocolate milk. J Agric Food Chem. 2000;48:1752–1757. doi: 10.1021/jf000026+
- Shahidi F, Wanasundata PK. Phenolic antioxidants. Crit Rev Food Sci Nutr. 1992;32:67–103. doi: 10.1080/10408399209527581
- Kondo K, Kurihara M, Miyata N, et al. Mechanistic studies of catechins as antioxidants against radical oxidation. Arch Biochem Biophys. 1999;362:79–86. doi: 10.1006/abbi.1998.1015
- Muzolf-Panek M, Szymusiak H, Gliszczyńska-Swigło A, et al. pH-Dependent radical scavenging capacity of green tea catechins. J Agric Food Chem. 2008;56:56(3),816–823. doi: 10.1021/jf0712189
- Haeberlin B, Friend DR. Anatomy and physiology of the gastrointestinal tract: implications for colonic drug delivery. In: Friend DR, editor. Oral colon specific drug delivery. Boca Raton: CRC Press; 2000. p. 1–44.
- Rubinstein A. Gastrointestinal anatomy, physiology and permeation pathways. In: Touitou E, Barry BW, editors. Enhancement in drug delivery. Boca Raton: CRC Press; 2007. p. 3–37.
- Amat-Guerri F, López-González MMC, Martínez-Utrilla R, et al. Singlet oxygen photogeneration by ionized and un-ionized derivatives of Rose Bengal and eosin Y in diluted solutions. J Photochem Photobiol A Chem. 1990;53:199–210. doi: 10.1016/1010-6030(90)87124-T
- Neckers DC. Rose Bengal. J Photochem Photobiol A Chem. 1989;47:1–29. doi: 10.1016/1010-6030(89)85002-6
- Foote CS, Ching TY. Chemistry of singlet oxygen XXI. Kinetics of bilirubin photooxigenation. J Am Chem Soc. 1975;97:6209–6214. doi: 10.1021/ja00854a045
- Wilkinson F, Helman WP, Ross A. Rate constants for the decay of the lowest electronically excited singlet state of molecular oxygen in solution. An expanded and revised compilation. J Phys Chem Ref Data. 1995;24:663–1021. doi: 10.1063/1.555965
- Miskoski S, Bertolotti S, Argüello G, et al. On the -mediated photooxidative behaviour of tripeptide glycyltyrosyl-alanine in alkaline medium: a kinetic study. Amino Acids. 1993;4:101–110. doi: 10.1007/BF00805805
- Nonell SL, Moncayo L, Trull F, et al. Solvent influence on the kinetics of the photodynamic degradation of Trolox, a water-soluble model compound for vitamin E. J Photochem Photobiol B: Biol. 1995;29:157–162. doi: 10.1016/1011-1344(95)07137-Q
- Reynoso E, Spesia MB, García NA, et al. Riboflavin-sensitized photooxidation of ceftriaxone and cefotaxime. Kinetic study and effect on Staphylococcus aureus. J Photochem Photobiol B. 2015;142:35–42. doi: 10.1016/j.jphotobiol.2014.11.004
- Criado S, Allevi C, García NA. Visible-light-promoted degradation of the antioxidants propyl gallate and t-butylhydroquinone. Mechanistic aspects. Redox Rep. 2012;17(3):131–138. doi: 10.1179/12Z.00000000018
- Criado S, Soltermann AT, García NA. Influence of the nuclear and extranuclear substitution of the singlet molecular oxygen mediated photooxidation of tyrosine derivates. A kinetic study. Amino Acids. 1995;8:367–377. doi: 10.1007/BF00806554
- Kennedy JA, Munro MHG, Powell HKJ, et al. The protonation reactions of catechin, epicatechin and related compounds. Aust J Chem. 1984;37(4):885–892. doi: 10.1071/CH9840885
- Schweigert N, Hunziker R, Escher BI, et al. The acute toxicity of (chloro-) catechol and (chloro-) catechol/copper combinations in Escherichia coli corresponds to the membrane toxicity of these compounds. Environ Toxicol Chem. 2001;20:239–247.
- Serjeant EP, Dempsey B, International Union of Pure and Applied Chemistry, Commission on Equilibrium Data, International Union of Pure and Applied Chemistry, Commission on Electrochemical Data. Ionisation constants of organic acids in aqueous solution. IUPAC Chemical Data Series 23. Oxford: Pergamon Press; 1979. p. 162.
- Lide DR. CRC Handbook of chemistry and physics: dissociation constants of organic acids and bases: resorcinol. 76th ed. Boca Raton, FL: CRC Press; 1995. p. 8–49.
- Montaña MP, Massad WA, Criado S, et al. Stability of flavonoids in the presence of riboflavin-photogenerated reactive oxygen species. A kinetic and mechanistic study of quercetin, morin and rutin. Photochem Photobiol. 2010;86:827–834. doi: 10.1111/j.1751-1097.2010.00754.x
- Escalada JP, Pajares A, Gianotti J, et al. Photosensitized degradation in water of the phenolic pesticides bromoxynil and dichlorophen in the presence of riboflavin, as a model of their natural photodecomposition in the environment. J Hazard Mater. 2011;186:466–472. doi: 10.1016/j.jhazmat.2010.11.026
- Haggi E, Blasich N, Gutiérrez L, et al. On the generation and quenching of reactive-oxygen-species by aqueous vitamin B2 and serotonin under visible-light irradiation. J Photochem Photobiol B. 2012;113:22–28. doi: 10.1016/j.jphotobiol.2012.04.010
- García NA. Singlet molecular oxygen mediated photodegradation of aquatic phenolic pollutants. A kinetic and mechanistic overview. J Photochem Photobiol B. 1994;22:185–196. doi: 10.1016/1011-1344(93)06932-S
- Criado S, García NA. A comparative kinetic and mechanistic study between tetrahydozoline and naphazoline toward photogenerated reactive oxygen species. Photochem Photobiol. 2010;86:23–30. doi: 10.1111/j.1751-1097.2009.00616.x
- Escalada JP, Pajares A, Gianotti J, et al. Dye-sensitized photodegradation of the fungicide carbendazim and related benzimidazoles. Chemosphere. 2006;65:237–244. doi: 10.1016/j.chemosphere.2006.02.057
- Silva E, Herrera L, Edwards AM, et al. Enhancement of riboflavin-mediated photo-oxidation of glucose 6-phosphate dehydrogenase by uronic acid. Photochem Photobiol. 2005;81:206–211. doi: 10.1562/2004-07-14-RA-233.1
- Silva E, Edwards AM, Pacheco D. Visible light-induced photooxidation of glucose sensitized by riboflavin. J Nutr Biochem. 1999;10:181–185. doi: 10.1016/S0955-2863(98)00093-X
- Wilkinson F, Brummer JG. Rate constants for the decay and reactions of the lowest electronically excited singlet state of molecular oxygen in solution. J Phys Chem Ref Data. 1981;10:809–999. doi: 10.1063/1.555655
- Zang PG, Misra HP. Superoxide radicals generated during the autooxidation of 1-methyl-4-phenyl-2,3-dihydropyridinium perchlorate. J Biol Chem. 1992;267:17547–17552.
- Gorman AA, Gould IR, Hamblett I, et al. Reversible exciplex formation between singlet oxygen and vitamin E. Solvent and temperature effects. J Am Chem Soc. 1984;106:6956–6959. doi: 10.1021/ja00335a014
- Gorman AA, Gould IR, Hamblett I. Time-resolved study of solvent and temperature dependence of singlet oxygen (1Δg) reactivity toward enol ethers: reactivity parameters typical of rapid reversible exciplex formation. J Am Chem Soc. 1982;104:7098–7104. doi: 10.1021/ja00389a036
- Criado S, García NA. Vitamin B2-sensitised photooxidation of the ophthalmic drugs timolol and pindolol. Kinetics and mechanism. Redox Rep. 2004;9(5):291–297. doi: 10.1179/135100004225006047
- Reynoso E, Nesci A, Allegretti P, et al. Kinetic and mechanistic aspects of sensitized photodegradation of β-lactam antibiotics: microbiological implications. Redox Rep. 2012;17(6):275–283. doi: 10.1179/1351000212Y.0000000032
- Fritz BJ, Matsui K, Kasai S, et al. Triplet lifetime of some flavins. Photochem Photobiol. 1987;45:539–541. doi: 10.1111/j.1751-1097.1987.tb05415.x