ABSTRACT
Objectives: Oxidative stress plays a key role in the pathogenesis of coronary artery disease. The aim of this study was to compare the effects of percutaneous transluminal coronary angioplasty (PTCA) and elective coronary angiography (EC) on erythrocytic antioxidant defense in elderly male patients.
Methods: Twenty-three stable angina pectoris (SAP) patients undergoing PTCA and 18 patients with ischemic symptoms scheduled to undergo diagnostic EC were included in the study. The concentrations of malondialdehyde (MDA) and reduced glutathione (GSH) and the activities of Zn,Cu-superoxide dismutase (SOD-1), catalase (CAT), and cytosolic glutathione peroxidase (GSH-Px) were examined in the erythrocytes before, immediately after and 2 weeks following PTCA or EC.
Results: The MDA concentrations were significantly higher and SOD-1, CAT, and GSH-Px activities were significantly lower in the PTCA group than in the EC group at baseline. Two weeks after treatment, the activities of the enzymes significantly increased in both groups, whereas the MDA concentrations decreased only in the PTCA patients.
Conclusions: The results confirm that an advanced state of atherosclerosis is related to greater levels of oxidative stress. The study indicates that both procedures may induce antioxidant defenses; however, PTCA exclusively induces a long-term reduction in lipid peroxidation.
Introduction
Coronary artery disease (CAD) is one of the leading causes of death worldwide [Citation1,Citation2]. According to data from the World Health Organization, of the 55.6 million global deaths in 2012, 7.4 million (13%) were due to CAD [Citation3]. Despite the significant decline in CAD mortality observed in industrialized countries during past decades, which reflects both the decrease in population risk factor levels and the development of more efficient therapies, CAD still remains the leading cause of mortality in the Western world [Citation1,Citation4]. For example, the death rate for CAD in Poland was halved between 1991 and 2005 because of both the reduction in risk factors and the existence of better medical treatment [Citation5]. Nevertheless, in 2012, CAD deaths accounted for approximately 24% of all deaths in Poland [Citation3]. Interestingly, the risk of death from CAD is age- and sex-dependent [Citation6–8]. In 2009, the CAD mortality rate in the Polish population aged 35–74 years was 3.5-fold greater in men than in women [Citation9].
CAD is a group of cardiovascular diseases that includes stable and unstable angina pectoris, nonfatal myocardial infarction, and sudden coronary death [Citation8]. Risk factors of CAD include increased blood pressure, high plasma cholesterol, high fasting plasma glucose, high body mass index, consumption of a suboptimal diet, physical inactivity, and tobacco smoking [Citation4]. Atherosclerosis, a multifactorial disorder of large- and medium-sized arteries, is the main underlying cause of CAD. During the formation of an atherosclerotic plaque, oxidized atherogenic lipoproteins, macrophages, lymphocytes, smooth muscle cells, cholesterol clefts, necrotic debris, and lipid-laden foam cells accumulate in the arterial wall, leading to increased arterial thickness and stiffness [Citation10–12]. The progression of atherosclerosis can narrow blood vessels and reduce blood flow, which causes oxygen and nutrient deficiency and malfunction of the local tissue [Citation10]. The patho-etiology of atherosclerosis is not completely understood. Multiple cellular processes, including endothelial cell dysfunction, inflammation, vascular smooth muscle cell proliferation, matrix alteration, and neovascularization, are suggested to participate in the progression of atherosclerosis [Citation11]. However, a growing body of evidence indicates that reactive oxygen species (ROS) and reactive nitrogen species (RNS) play a pivotal role in the process [Citation10–14]. Augmented vascular oxidative stress has been found to increase the risk of cardiovascular events in CAD patients [Citation15,Citation16]. Moreover, excessive ROS production has been linked to the impairment of endothelial functions [Citation14,Citation17]. Enzymes, such as NAD(P)H oxidase, uncoupled endothelial nitric oxide synthase, xanthine oxidase, and cyclooxygenase, are the most important sources of ROS and reactive nitrogen species in the vasculature [Citation10,Citation13,Citation18]. During the inflammatory stage of atherosclerosis, increased levels of ROS are produced by monocytes/macrophages, dysfunctional endothelial cells, and smooth muscle cells [Citation10,Citation14]. According to the oxidative theory of atherosclerosis, augmented oxidative stress results in the oxidation of low-density lipoproteins, triggering pathological responses in the vasculature and leading to atherogenesis [Citation12,Citation19,Citation20]. The precise mechanisms of these interactions have been only partly described.
CAD can also be defined by the procedures performed in the case of clinically confirmed atherosclerosis, such as coronary artery bypass surgery or percutaneous coronary intervention [Citation8]. Percutaneous transluminal coronary angioplasty (PTCA) is the most common procedure used for the mechanical revascularization of obstructed coronary arteries. PTCA is associated with the excessive vascular production of ROS, which leads to post-procedural pathological changes, including restenosis, thrombosis, and endothelial dysfunction in the treated artery [Citation18,Citation21]. As a result of the reintroduction of oxygen to the ischemic myocardium after the improvement of coronary artery blood flow, ROS-generated ischemia–reperfusion injury may occur [Citation22,Citation23]. The PTCA procedure itself is associated with short episodes of myocardial ischemia and increased oxidative stress in myocardial tissue [Citation22].
A better understanding of the mechanisms that lead to ROS generation during and after PTCA and the pathophysiological consequences of this process are crucial to improving the success rate of the procedure. Thus, the aim of the present study was to evaluate the changes in selected parameters of oxidative stress in the erythrocytes of male patients with stable angina pectoris (SAP) scheduled to undergo PTCA and male patients with ischemic symptoms scheduled to undergo diagnostic elective coronary angiography (EC).
Materials and methods
Participants
Twenty-three male SAP patients scheduled to undergo PTCA and 18 male patients with ischemic symptoms scheduled to undergo diagnostic EC (control group) were included in the study. All patients received standard physical examinations and routine clinical laboratory tests. Criteria for the inclusion and exclusion of patients are presented in . All subjects provided informed consent to participate in the research. The study was approved by the Local Ethics Committee at Collegium Medicum, Nicolaus Copernicus University (Bydgoszcz, Poland).
Table 1. Inclusion and exclusion criteria for participation in the study.
PTCA and EC procedures
PTCA and EC were performed according to standard techniques via the femoral route. Before both procedures, all patients received dual antiplatelet therapy. During the procedures, serial coronary angiograms of the left (in at least five projections) and right (in at least two projections) coronary arteries were obtained after the intracoronary administration of non-ionic contrast agents, namely, iomeprol (Iomeron 400, Bracco ALTANA Pharma GmbH, Konstanz, Germany), iopromide (Ultravist 370, Schering, Berlin, Germany), or ioversol (Optiray 300, Tyco Healthcare GmbH, Germany). Heparin (100 U/kg) was given before the introduction of the guide wire. In the case of PTCA, after the diagnostic evaluation of coronary arteries, percutaneous coronary interventions with or without stenting were performed. After the procedure, the patients were treated with aspirin indefinitely. The use of ticlopidine therapy depended on the stenting procedure.
Experimental protocols
Blood specimens were collected from every patient three times, including before, immediately after, and 14 days after the PTCA/EC procedure. The samples were obtained from the cubital vein in heparinized tubes (6 ml) after patients had fasted overnight to obtain erythrocytes and plasma. All samples were centrifuged (2500 g for 10 minutes). After plasma separation, the hemolysate was prepared by three-fold freezing and thawing the washed erythrocytes, which were suspended in bidistilled water.
Biochemical analysis
The hemolysate was used to determine the parameters of oxidative stress. The malondialdehyde (MDA) and reduced glutathione (GSH) concentrations, as well as the activities of Zn,Cu-superoxide dismutase (SOD-1; EC 1.15.1.1), catalase (CAT; EC 1.11.1.6), and cytosolic glutathione peroxidase (GSH-Px; EC 1.11.1.9), were assayed by the methods described by Placer et al. [Citation24], Beutler [Citation25], Paoletti and Mocali [Citation26], Beers and Sizer [Citation27], and Paglia and Valentine [Citation28], respectively. The MDA level was read at 532 nm as a concentration of thiobarbituric acid reactive substances. The GSH level was read at 412 nm as the concentration of a yellow product of 5,5′-dithiobis(2-nitrobenzoic acid) reduction by the sulfhydryl group of thiol-containing compounds. SOD-1 activity was determined at 37°C by recording the increase in absorbance at 480 nm, following the auto-oxidation of adrenaline, which was inhibited by SOD-1. One unit (U) of SOD-1 activity was defined as the amount of enzyme that inhibited the maximal rate of adrenaline auto-oxidation by 50%. CAT activity was recorded at 25°C by recording H2O2 decomposition at 240 nm. One Bergmeyer unit of this activity was defined as the amount of enzyme that decomposed 1 g of H2O2 per minute.The activity of GSH-Px was determined at 25°C by measuring the decrease in absorbance at 340 nm, following the oxidation of NADPH in the presence of tert-butyl hydroperoxide as a substrate, GSH, yeast glutathione reductase, and NaN3 as a CAT inhibitor. One unit (U) of GSH-Px activity was defined as the amount of the enzyme that oxidized 1 μmol of NADPH/min. The hemoglobin concentration in the hemolysate was read at 540 nm after conversion into the cyanmethemoglobin form using a commercial reagent (Biomed, Lublin, Poland).
Statistical analysis
All results are expressed as the means ± S.E.M. Statistical analysis included Student’s t-test for the comparison of the PTCA and EC groups and a paired t-test for the comparison of the same group before and after the procedures. The hypothesis of normal distribution was verified using the Shapiro–Wilk test. In turn, the homogeneity of variances was assessed by Levene’s test. Pearson’s correlation coefficient was used to quantify the relationship between the parameters measured. The level of significance was set at p < 0.05.
Results
The clinical characteristics of the patients are presented in . The MDA levels were significantly higher in the SAP patients than in the control group at baseline (0.261 ± 0.007 and 0.224 ± 0.004 μmol/g Hb, respectively; p < 0.001) ((a)), and the activities of SOD-1 (2405 ± 51 and 2559 ± 39 U/g Hb, respectively; p < 0.05) ((a)), CAT (21.7 ± 0.54 and 23.3 ± 0.36 U/g Hb, respectively; p < 0.05) ((b)), and GSH-Px (12.98 ± 0.73 and 15.08 ± 0.40 U/g Hb, respectively; p < 0.05) ((c)) were significantly lower in the SAP patients than in the control group at baseline. There were no significant differences in the GSH concentrations (2.34 ± 0.06 and 2.53 ± 0.05 µmol/l, respectively) ((b)) between the groups.
Figure 1. Effect of PTCA on the (a) MDA and (b) reduced GSH concentrations in the erythrocytes of male patients with SAP compared with the effect of diagnostic EC on the erythrocytes of male subjects (control group). Data are presented as the means ± S.E.M. *p < 0.001, before the procedure versus 14 days after the procedure, #p < 0.001, SAP patients versus control subjects.
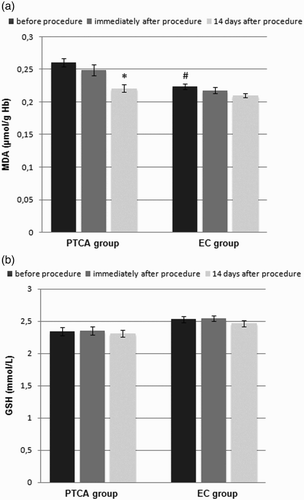
Figure 2. Effect of PTCA on the activities of antioxidant enzymes in the erythrocytes of male patients with SAP compared with the effect of diagnostic EC on the erythrocytes of male subjects (control group): (a) Cu–Zn SOD-1, (b) CAT, (c) cellular GSH-Px. Data are presented as the means ± S.E.M. *p < 0.05, before the procedure versus 14 days after the procedure, #p < 0.05, SAP patients versus control subjects.
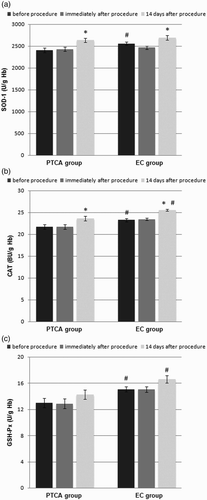
Table 2. Clinical characteristics of the patients included in the study.
Immediately after the PTCA and EC procedures, the MDA concentrations (0.249 ± 0.008 and 0.218 ± 0.004, respectively) ((a)), GSH concentrations (2.35 ± 0.06 and 2.55 ± 0.04, respectively) ((b)), and activities of SOD-1 (2429 ± 50 and 2465 ± 41, respectively) ((a)), CAT (21.7 ± 0.54 and 23.5 ± 0.28, respectively) ((b)), and GSH-Px (12.88 ± 0.72 and 15.06 ± 0.42, respectively) ((c)) in the SAP patients and control subjects did not change significantly.
Fourteen days after the PTCA procedure, the concentrations of MDA significantly decreased (0.221 ± 0.006; p < 0.001) ((a)) and the activities of SOD-1 (2635 ± 49; p < 0.05) ((a)) and CAT (23.6 ± 0.55; p < 0.05) ((b)) significantly increased. In the case of EC, 14 days after the procedure, the concentrations of MDA (0.210 ± 0.004) ((a)) remained unchanged, whereas the activities of SOD-1 (2694 ± 53; p < 0.05) ((a)) and CAT (25.6 ± 0.22; p < 0.05) ((b)) significantly increased. Neither the PTCA nor the EC procedure affected the GSH (2.31 ± 0.05 and 2.46 ± 0.05, respectively) ((b)) concentrations or the GSH-Px (14.27 ± 0.73 and 16.60 ± 0.54, respectively) ((c)) activities.
When data from all subjects were pooled, the following correlations were found between the parameters. The MDA concentration was negatively correlated with SOD-1 activity (r = −0.193; p < 0.05), CAT activity (r = −0.192, p < 0.05), and GSH-Px activity (r = −0.474, p < 0.000001). Significant positive correlations were noted between GSH concentration and CAT activity (r = 0.393, p < 0.00001), SOD-1 activity and CAT activity (r = 0.425, p < 0.00001), SOD-1 activity and GSH-Px activity (r = 0.184, p < 0.05), and CAT activity and GSH-Px activity (r = 0.292, p < 0.001).
Discussion
In Poland, the risk of death from CAD for men aged 60–69 is almost 3-fold greater than that for women of the same age [Citation3]. Therefore, an investigation of the changes in oxidative stress markers in male patients undergoing PTCA or EC was performed in the present study. According to the results of previous studies, cardiovascular risk factors may exert a gender-dependent impact [Citation29]. Among other differences, gender-related differences in oxidative stress status were found to exist in elderly CAD patients [Citation30,Citation31]. Oxidative stress was observed to be greater in postmenopausal women than in elderly men [Citation30,Citation31], whereas the opposite results were obtained in a comparison of premenopausal women and young men [Citation32].
It was demonstrated in the present study that pro- and antioxidant balance is more disturbed in male SAP patients than in elderly men with a high risk of ischemic heart disease but without obstructive CAD: the MDA concentrations were higher and the activities of SOD-1, CAT, and GSH-Px were lower. However, the GSH concentrations were comparable in both groups. The elevated MDA levels reflect cell membrane damage due to lipid peroxidation, one of the most important pathological processes evoked by ROS [Citation33]. A high concentration of lipid peroxides causes the production of peroxyl radicals that may inactivate the vasodilator nitric oxide (NO) through the formation of lipid peroxynitrites, leading to the impairment of endothelium-dependent vasodilator function [Citation14,Citation34,Citation35]. The increase in oxidative stress may be at least partly associated with the weakened antioxidant enzymatic defense. SODs, CATs, and GSH-Pxs account for the first line of cellular defense against ROS [Citation36]. SODs catalyze the dismutation of the superoxide radical to form oxygen and hydrogen peroxide, whereas CAT catalyzes the decomposition of hydrogen peroxide to yield oxygen and water. GSH-Pxs are able to decompose both hydrogen peroxide and lipid hydroperoxides to form water or corresponding alcohols via GSH. The observed decreases in the activities of SOD-1, CAT, and GSH-Px may be a result of oxidative modifications of enzymatic proteins, although deficiencies in micronutrients such as zinc and selenium in elderly men should also be taken into consideration [Citation37].
Increased oxidative stress may impair endothelial and cardiomyocyte functions, leading to structural vascular and cardiac abnormalities. Many studies have demonstrated that the pro- and antioxidant balance in CAD patients is disturbed toward oxidation reactions, pointing to the important role of oxidative stress in atherogenesis [Citation11,Citation38–42]. In the present study, a more advanced state of atherosclerosis was associated with a greater pro- and antioxidant imbalance. Thus, the level of oxidative stress may be used as an independent predictor of cardiovascular events, in agreement with the observations of other authors [Citation15,Citation40,Citation41,Citation43–47]. Blankenberg et al. [Citation43] found an inverse association between erythrocytic GSH-Px and the risk of cardiovascular events. In the Gupta et al. study [Citation40], antioxidant defense was increased during the initial stages of CAD, but it was significantly decreased with increasing disease severity. Additionally, Patel et al. [Citation47] described a positive association between oxidative burden and mortality of patients with CAD. Correspondingly, Kotur-Stevuljevic et al. [Citation41] observed significantly lower SOD-1 activities in patients with advanced CAD (stenosis greater than 50%) compared with both healthy people and patients with stenosis of less than 50%. However, Lubrano et al. [Citation46] found a slight enhancement of extracellular SOD activity in relation to the severity of CAD, suggesting the presence of a mild compensatory mechanism.
The weakened antioxidant system that was observed in the SAP patients supports the potential use of antioxidants in CAD treatment. Many authors recommended antioxidant treatment in patients with CAD [Citation12,Citation22,Citation39,Citation41,Citation48,Citation49], although some of them suggested the fitting of the therapies to a patient’s characteristics, including gender and age [Citation39]. Undoubtedly, the complexity of ROS effects on vascular endothelium should be taken into consideration. ROS are molecules potentially deleterious to cells, causing the oxidation of lipids, proteins, and DNA. However, ROS are involved in normal signaling pathways that are essential for cardiovascular physiology [Citation35,Citation36] and may have a protective role in atherosclerosis [Citation50]. Furthermore, ROS are also mediators in redox-sensitive pathways that are activated during atherogenesis [Citation13,Citation36,Citation48]. This may explain the discrepancies that exist between different studies testing the impact of exogenous antioxidant treatment on atherosclerosis development. Many in vitro and animal model experiments demonstrated an anti-atherosclerotic effect of various antioxidants, while the majority of human clinical trials and meta-analyses demonstrated no beneficial influence of antioxidant vitamin supplementation on cardiovascular events [Citation10,Citation12,Citation20,Citation42]. Certainly, further studies are required to establish novel antioxidant delivery strategies to treat patients with CAD [Citation10,Citation51].
During PTCA, blood flow is restored in infarct-related arteries, which reintroduces oxygen to the ischemic myocardium. After the restoration of blood flow, vascular cells produce more ROS, which results in ROS-generated reperfusion injury [Citation23,Citation36,Citation45]. Ischemia/reperfusion injury is a major clinical problem due to the initiation of myocardial damage [Citation52], which is why oxidative stress during and after PTCA or EC has been intensively investigated in numerous studies [Citation22,Citation23,Citation44,Citation45,Citation53]. In the present study, the parameters of oxidative stress in the blood remained unchanged immediately after the PTCA and EC procedures. Similarly, Kaminski et al. [Citation45] did not find any changes in oxidative stress parameters in blood from the coronary sinus immediately after the reopening of the infarct-related artery in acute myocardial infarction (AMI) patients treated with PTCA. In contrast, Iuliano et al. [Citation53] observed increased levels of F2-isoprostanes, markers of lipid peroxidation, in the coronary sinus immediately after balloon deflation during PTCA but not in blood from the coronary sinus of patients undergoing diagnostic EC. However, it should be taken into consideration that the group of EC patients in the Iuliano et al. study [Citation53] consisted of only four patients. Yardim-Akaydm et al. [Citation23] demonstrated that oxidation state parameters were increased in the blood of CAD patients 4 hours after PTCA. Interestingly, Ciçek et al. [Citation22] detected increased MDA concentrations in venous blood 10 minutes after the first balloon inflation during PTCA, but the MDA levels in arterial blood samples remained unchanged. The limitation of the present study was the assessment of biochemical parameters in blood samples obtained from the cubital vein rather than the coronary sinus. This discrepancy may explain why no significant changes were observed in the oxidative state immediately after PTCA or EC.
Fourteen days after PTCA and EC, the activities of SOD-1 and CAT significantly increased, whereas the GSH-Px activities and GSH concentrations did not change in the present study. Interestingly, the MDA levels significantly decreased 14 days after the procedure only in the PTCA group. The decreased MDA levels indicated the improvement of the pro- and antioxidant balance in the SAP patients, probably due to the reduction in the number of ischemic events. These observations are consistent with the results of other studies [Citation38,Citation44,Citation54,Citation55]. Bashar and Akhtan [Citation38] demonstrated that the regular treatment of AMI patients led to a significant decrease in oxidative stress parameters. Kochiadakis et al. [Citation54] also observed changes in plasma total peroxides after PTCA with stenting in CAD patients. Similarly, the levels of 8-hydroxydeoxyguanosine, which is a marker of oxidative DNA damage, sharply decreased 3 days after PTCA in AMI patients in the Himmetoglu et al. study [Citation44]. Moreover, vitamin C infusion 2–6 hours before PTCA resulted in significantly lower serum values of 8-hydroxydeoxyguanosine after the procedure [Citation55].
It was demonstrated in the present study that the activities of the antioxidant enzymes increased significantly 14 days after both PTCA and EC. These results may indicate that diagnostic EC is sufficient to augment oxidative stress, leading to a compensatory enhancement of enzymatic defense. Goldberg et al. [Citation56] found that uncomplicated EC induced an inflammatory response in SAP patients. In contrast, Almagor et al. [Citation57] demonstrated no changes in inflammatory markers in patients undergoing EC. Increased inflammatory parameters in SAP patients after PTCA were observed in several other studies [Citation58,Citation59]. Several mechanisms may explain the augmented oxidative stress and inflammatory response after diagnostic EC, including local soft tissue injury caused by the puncture of the femoral artery, arterial wall injury during catheterization, or a systemic response to the contrast agent used.
Conclusions
In this study, it was found that a more advanced state of atherosclerosis was related to a greater pro- and antioxidant imbalance. This finding suggests that the level of oxidative stress may be a useful independent predictor of cardiovascular events. In addition, it was demonstrated that EC and PTCA induce a long-term increase in antioxidant enzymatic defense, suggesting that both diagnostic and interventional procedures cause an increase in ROS generation and a compensatory enhancement of antioxidant enzyme activities. Thus, the contribution of EC should be taken into consideration when interpreting the significance of changes in oxidative stress markers observed after PTCA. However, it was shown that PTCA exclusively reduced the level of lipid peroxidation in the SAP patients. This finding could be at least partly explained by a reduction in the number of ischemic events after the restoration of blood flow in the infarct-related arteries. In summary, the present study confirmed the involvement of oxidative stress in the atherosclerosis process. Undoubtedly, further studies are necessary to understand the molecular mechanisms linking ROS, atherogenesis, and CAD and to design antioxidant therapies that support the treatment of cardiovascular diseases.
Disclosure statement
No potential conflict of interest was reported by the authors.
Notes on contributors
Karolina Szewczyk-Golec is an assistant professor in the Chair of Medical Biology of the Ludwik Rydygier Collegium Medicum of Nicolaus Copernicus University, Bydgoszcz, Poland. She is a biochemist. Her main studies are focused on oxidative stress and melatonin.
Paweł Grzelakowsk is a cardiologist in the Clinic of Cardiology and Cardiac Surgery of 10, Military Training Hospital, Bydgoszcz, Poland. They both graduated from Ludwik Rydygier Collegium Medicum of Nicolaus Copernicus University (PhD), Bydgoszcz, Poland. Their research area is the role of oxidative stress in cardiovascular disease processes.
Tomasz Ługowski is a cardiologist in the Clinic of Cardiology and Cardiac Surgery of 10, Military Training Hospital, Bydgoszcz, Poland. They both graduated from Ludwik Rydygier Collegium Medicum of Nicolaus Copernicus University (PhD), Bydgoszcz, Poland. Their research area is the role of oxidative stress in cardiovascular disease processes.
Józef Kędziora is a retired professor of biochemistry and physiology. He was the head of Department of Biochemistry in the Ludwik Rydygier Collegium Medicum of Nicolaus Copernicus University, Bydgoszcz, Poland. His main research focus was the role of oxidative stress in a variety of human physiological and pathological conditions, including aging, Down’s syndrome, cardiovascular diseases, and diabetes mellitus.
ORCID
Karolina Szewczyk-Golec http://orcid.org/0000-0001-8123-3882
References
- Mozaffarian D, Benjamin EJ, Go AS, et al. Heart disease and stroke statistics – 2015 update: a report from the American Heart Association. Circulation. 2015;131:e29–322. doi: 10.1161/CIR.0000000000000152
- World Health Organization. Global status report on noncommunicable diseases 2014. Geneva: WHO; 2014. (WHO publication, ISBN 978 92 4 156485 4).
- Global Health Estimates 2014 Summary tables: DALYs by cause, age and sex, 2000-2012 [Internet]. Geneva: World Health Organization. [cited 2016 Apr 1]. Available from: http://www.who.int/healthinfo/global_burden_disease/en/
- Huffman MD, Lloyd-Jones DM, Ning H, et al. Quantifying options for reducing coronary heart disease mortality by 2020. Circulation. 2013;127:2477–2484. doi: 10.1161/CIRCULATIONAHA.112.000769
- Bandosz P, O’Flaherty M, Drygas W, et al. Decline in mortality from coronary heart disease in Poland after socioeconomic transformation: modelling study. Brit Med J. 2012;344:d8136. doi: 10.1136/bmj.d8136
- Claassen M, Sybrandy KC, Appelman YE, et al. Gender gap in acute coronary heart disease: myth or reality?. World J Cardiol. 2012;4:36–47. doi: 10.4330/wjc.v4.i2.36
- Lehto HR, Lehto S, Havulinna AS, et al. Sex differences in short- and long-term case-fatality of myocardial infarction. Eur J Epidemiol. 2011;26:851–861. doi: 10.1007/s10654-011-9601-6
- Wong ND. Epidemiological studies of CHD and the evolution of preventive cardiology. Nat Rev Cardiol. 2014;11:276–289. doi: 10.1038/nrcardio.2014.26
- Go AS, Mozaffarian D, Roger VL, et al. Heart disease and stroke statistics – 2013 update: a report from the American Heart Association. Circulation. 2013;127:e6–e245. doi: 10.1161/CIR.0b013e31828124ad
- He F, Zuo L. Redox roles of reactive oxygen species in cardiovascular diseases. Int J Mol Sci. 2015;16:27770–27780. doi: 10.3390/ijms161126059
- Perrotta I, Aquila S. The role of oxidative stress and authophagy in atherosclerosis. Oxid Med Cell Longev. 2015;2015:130315. doi: 10.1155/2015/130315
- Salvayre R, Negre-Salvayre A, Camaré C. Oxidative theory of atherosclerosis and antioxidants. Biochimie. 2016;125:281–296. doi: 10.1016/j.biochi.2015.12.014
- Eelen G, de Zeeuw P, Simons M, et al. Endothelial cell metabolism in normal and diseased vasculature. Circ Res. 2015;116:1231–1244. doi: 10.1161/CIRCRESAHA.116.302855
- Victor VM, Rocha M, Solá E, et al. Oxidative stress, endothelial dysfunction and atherosclerosis. Curr Pharm Des. 2009;15:2988–3002. doi: 10.2174/138161209789058093
- Heitzer T, Schlinzig T, Krohn K, et al. Endothelial dysfunction, oxidative stress, and risk of cardiovascular events in patients with coronary artery disease. Circulation. 2001;104:2673–2678. doi: 10.1161/hc4601.099485
- Vassalle C, Bianchi S, Bianchi F, et al. Oxidative stress as a predictor of cardiovascular events in coronary artery disease patients. Clin Chem Lab Med. 2012;50:1463–1468. doi: 10.1515/cclm-2011-0919
- Guzik TJ, West NE, Black E, et al. Vascular superoxide production by NAD(P)H oxidase: association with endothelial dysfunction and clinical risk factors. Circ Res. 2000;86:e85–90. doi: 10.1161/01.RES.86.9.e85
- Juni RP, Duckers HJ, Vanhoutte PM, et al. Oxidative stress and pathological changes after coronary artery interventions. J A Coll Cardiol. 2013;61:1471–1481. doi: 10.1016/j.jacc.2012.11.068
- Peluso I, Morabito G, Urban L, et al. Oxidative stress in atherosclerosis development: the central role of LDL and oxidative burst. Endocr Metab Immune Disord Drug Targets. 2012;12:351–360. doi: 10.2174/187153012803832602
- Stocker R, Keaney JF Jr. New insights on oxidative stress in the artery wall. J Thromb Haemost. 2005;3:1825–1834. doi: 10.1111/j.1538-7836.2005.01370.x
- Neuzil J, Rayner BS, Lowe HC, et al. Oxidative stress in myocardial ischaemia reperfusion injury: a renewed focus on a long-standing area of heart research. Redox Rep. 2005;10:187–197. doi: 10.1179/135100005X57391
- Ciçek D, Tamer L, Pekdemir H, et al. Coronary angioplasty induced oxidative stress and its relation with metoprolol use and plasma homocysteine levels. Anadolu Kardiyol Derg. 2006;6:308–313.
- Yardim-Akaydin S, Kesimer M, Imren E, et al. Urate oxidation during percutaneous transluminal coronary angioplasty and thrombolysis in patients with coronary artery disease. Clin Chim Acta. 2005;362:131–137. doi: 10.1016/j.cccn.2005.06.002
- Placer ZA, Cushman LL, Johnson BC. Estimation of product of lipid peroxidation (malonyl dialdehyde) in biochemical systems. Anal Biochem. 1966;16:359–364. doi: 10.1016/0003-2697(66)90167-9
- Beutler E. Red cell metabolism. In: Beutler E, editor. Red cell metabolism. A manual of biochemical methods. New York (NY): Grune-Stratton; 1971. p. 103–105.
- Paoletti F, Mocali A. Determination of superoxide dismutase activity by purely chemical system based on NAD(P)H oxidation. Methods Enzymol. 1990;186:209–220. doi: 10.1016/0076-6879(90)86110-H
- Beers RF Jr, Sizer IW. A spectrophotometric method for measuring the breakdown of hydrogen peroxide by catalase. J Biol Chem. 1952;195:133–140.
- Paglia DE, Valentine WN. Studies on the quantitative and qualitative characterization of erythrocyte glutathione peroxidase. J Lab Clin Med. 1967;70:158–169.
- Anand SS, Islam S, Rosengren A, et al. Risk factors for myocardial infarction in women and men: insights from the INTERHEART study. Eur Heart J. 2008;29:932–940. doi: 10.1093/eurheartj/ehn018
- Vassalle C, Maffei S, Boni C, et al. Gender-related differences in oxidative stress levels among elderly patients with coronary artery disease. Fertil Steril. 2008;89:608–613. doi: 10.1016/j.fertnstert.2007.03.052
- Vassalle C, Sciarrino R, Bianchi S, et al. Sex-related differences in association of oxidative stress status with coronary artery disease. Fertil Steril. 2012;97:414–419. doi: 10.1016/j.fertnstert.2011.11.045
- Ide T, Tsutsui H, Ohashi N, et al. Greater oxidative stress in healthy young men compared with premenopausal women. Arterioscler Thromb Vasc Biol. 2002;22:438–442. doi: 10.1161/hq0302.104515
- Gil L, Siems W, Mazurek B, et al. Age-associated analysis of oxidative stress parameters in human plasma and erythrocytes. Free Radic Res. 2006;40:495–505. doi: 10.1080/10715760600592962
- Forgione MA, Weiss N, Heydrick S, et al. Cellular glutathione peroxidase deficiency and endothelial dysfunction. Am J Physiol Heart Circ Physiol. 2002;282:H1255–1261. doi: 10.1152/ajpheart.00598.2001
- Weidinger A, Kozlov AV. Biological Activities of Reactive Oxygen and Nitrogen Species: Oxidative Stress versus Signal Transduction. Biomolecules. 2015;5:472–484. doi: 10.3390/biom5020472
- Brown DI, Griendling KK. Regulation of signal transduction by reactive oxygen species in the cardiovascular system. Circ Res. 2015;116:531–549. doi: 10.1161/CIRCRESAHA.116.303584
- Kedziora-Kornatowska K, Szewczyk-Golec K, Czuczejko J, et al. Effect of melatonin on the oxidative stress in erythrocytes of healthy young and elderly subjects. J Pineal Res. 2007;42:153–158. doi: 10.1111/j.1600-079X.2006.00394.x
- Bashar T, Akhter N. Study on oxidative stress and antioxidant level in patients of acute myocardial infarction before and after regular treatment. Bangladesh Med Res Counc Bull. 2014;40:79–84. doi: 10.3329/bmrcb.v40i2.25226
- Cavalca V, Veglia F, Squellerio I, et al. Glutathione, vitamin E and oxidative stress in coronary artery disease: relevance of age and gender. Eur J Clin Invest. 2009;39:267–272. doi: 10.1111/j.1365-2362.2009.02094.x
- Gupta S, Sodhi S, Mahajan V. Correlation of antioxidants with lipid peroxidation and lipid profile in patients suffering from coronary artery disease. Expert Opin Ther Targets. 2009;13:889–894. doi: 10.1517/14728220903099668
- Kotur-Stevuljevic J, Memon L, Stefanovic A, et al. Correlation of oxidative stress parameters and inflammatory markers in coronary artery disease patients. Clin Biochem. 2007;40:181–187. doi: 10.1016/j.clinbiochem.2006.09.007
- Sharma A, Yuen D, Huet O, et al. Lack of glutathione peroxidase-1 facilitates a pro-inflammatory and activated vascular endothelium. Vascul Pharmacol. 2015;79:32-42. doi: 10.1016/j.vph.2015.11.001
- Blankenberg S, Rupprecht HJ, Bickel C, et al. Glutathione peroxidase 1 activity and cardiovascular events in patients with coronary artery disease. N Engl J Med. 2003;349:1605–1613. doi: 10.1056/NEJMoa030535
- Himmetoglu S, Dincer Y, Bozcali E, et al. Oxidative DNA damage and antioxidant defense after reperfusion in acute myocardial infarction. J Investig Med. 2009;57:595–599. doi: 10.2310/JIM.0b013e31819d87c1
- Kaminski K, Bonda T, Wojtkowska I, et al. Oxidative stress and antioxidative defense parameters early after reperfusion therapy for acute myocarddial infarction. Acute Card Care. 2008;10:121–126. doi: 10.1080/17482940701744334
- Lubrano V, Di Cecco P, Zucchelli GC. Role of superoxide dismutase in vascular inflammation and in coronary artery disease. Clin Exp Med. 2006;6:84–88. doi: 10.1007/s10238-006-0100-0
- Patel RS, Ghasemzadeh N, Eapen DJ, et al. A novel biomarker of oxidative stress is associated with risk of death in patients with coronary artery disease. Circulation. 2015;133:361–369. doi: 10.1161/CIRCULATIONAHA.115.019790
- Lam YT. Critical roles of reactive oxygen species in age-related impairment in ischemia-induced neovascularization by regulating stem and progenitor cell function. Oxid Med Cell Longev. 2015;2015:7095901.
- Lubrano V, Balzan S. Enzymatic antioxidant system in vascular inflammation and coronary artery disease. World J Exp Med. 2015;5:218–224. doi: 10.5493/wjem.v5.i4.218
- Gray SP, Di Marco E, Kennedy K, et al. Reactive oxygen species can provide atheroprotection via NOX4-dependent inhibition of inflammation and vascular remodeling. Arterioscler Thromb Vasc Biol. 2016;36:295-307. doi: 10.1161/ATVBAHA.115.307012
- Wu J, Hecker JG, Chiamvimonvat N. Antioxidant enzyme gene transfer for ischemic diseases. Adv Drug Deliv Rev. 2009;61:351–363. doi: 10.1016/j.addr.2009.01.005
- Rodrigo R, Libuy M, Feliú F, et al. Molecular basis of cardioprotective effect of antioxidant vitamins in myocardial infarction. Biomed Res Int. 2013;2013:437613. doi: 10.1155/2013/437613
- Iuliano L, Praticò D, Greco C, et al. Angioplasty increases coronary sinus F2-isoprostane formation: evidence for in vivo oxidative stress during PTCA. J Am Coll Cardiol. 2001;37:76–80. doi: 10.1016/S0735-1097(00)01040-8
- Kochiadakis GE, Arfanakis DA, Marketou ME, et al. Oxidative stress changes after stent implantation: a randomized comparative study of sirolimus-eluting and bare metal stents. Int J Cardiol. 2010;142:33–37. doi: 10.1016/j.ijcard.2008.12.105
- Wang ZJ, Hu WK, Liu YY, et al. The effect of intravenous vitamin C infusion on periprocedural myocardial injury for patients undergoing elective percutaneous coronary intervention. Can J Cardiol. 2014;30:96–101. doi: 10.1016/j.cjca.2013.08.018
- Goldberg A, Zinder O, Zdorovyak A, et al. Diagnostic coronary angiography induces a systemic inflammatory response in patients with stable angina. Am Heart J. 2003;146:819–823. doi: 10.1016/S0002-8703(03)00407-1
- Almagor M, Keren A, Banai S. Increased C-reactive protein level after coronary stent implantation in patients with stable coronary artery disease. Am Heart J. 2003;145:248–253. doi: 10.1067/mhj.2003.16
- Gach O, Legrand V, Biessaux Y, et al. Long-term prognostic significance of high-sensitivity C-reactive protein before and after coronary angioplasty in patients with stable angina pectoris. Am J Cardiol. 2007;99:31–35. doi: 10.1016/j.amjcard.2006.07.059
- Saleh N, Svane B, Jensen J, et al. Stent implantation, but not pathogen burden, is associated with plasma C-reactive protein and interleukin-6 levels after percutaneous coronary intervention in patients with stable angina pectoris. Am Heart J. 2005;149:876–882. doi: 10.1016/j.ahj.2004.07.039