ABSTRACT
Objectives: To investigate the effects of sodium ascorbate (SA) (5–3125 μM) and a combination of SA and Trolox (25 and 125 μM) on oxidative changes generated in red blood cells (RBCs) followed by up to 20 days refrigerated storage.
Methods: RBCs were isolated from CPD-preserved human blood. Percentage of hemolysis and extracellular activity of lactate dehydrogenase (LDH) were measured to assess the RBC membrane integrity. Lipid peroxidation (LPO), glutathione (GSH) and total antioxidant capacity (TAC) were quantified by thiobarbituric acid-reactive substances, Ellman’s reagent and 2,2′-azinobis-(3-ethylbenzothiazoline-6-sulfonate) -based assay, respectively.
Results: SA failed to reduce the storage-induced hemolysis and RBC membrane permeability. Addition of SA resulted in a concentration-independent LPO inhibition and increased TAC. A combination of SA/Trolox supplemented to the RBC medium significantly inhibited hemolysis, LDH leakage, LPO, GSH depletion and enhanced TAC.
Discussion: The effects of vitamin C action are closely concentration-dependent and may be modulated by a variety of compounds (e.g. Hb degradation products) released from RBCs during the prolonged storage, changing its properties from anti- to pro-oxidative. The two different class antioxidants (SA/Trolox) could possibly cooperate to be good potential RBC storage additives ensuring both antiradical and membrane stabilizing protection.
Introduction
Red blood cells (RBCs) for transfusion are routinely stored for up to 42 days [Citation1]. Such an extended RBC shelf-life is possible due to the application of the appropriate preparation methods, suitable storage preservative/additive solutions (e.g. CPD – citrate-phosphate-dextrose/SAGM or AS-5 – different combinations of saline-adenine-glucose-mannitol), polyvinyl chloride blood bags, and low temperature (4 ± 2°C) [Citation2]. During storage, the RBC functionality is progressively impaired resulting from the distorted metabolism, oxidative stress (OS) accumulation and membrane damage [Citation3] which can affect the recipient outcomes [Citation4,Citation5]. The storage lesion include a depletion of ATP, 2,3-diphosphoglycerate (2,3-DPG), glutathione (GSH) and NADH/NADPH, hemoglobin (Hb) oxidation, an exhaustion of the endogenous antioxidant defense system, leakage of Hb, lactate, lactate dehydrogenase (LDH) and potassium ions to the suspending medium (supernatant), membrane microvesiculation, abnormal morphology and decreased osmotic fragility [Citation1,Citation6]. A storage-dependent accumulation of the reactive oxygen species (ROS) in the RBC units results in irreversible oxidative damage to the red cell membrane lipids and oxidation of the membrane/cytoskeleton protein [Citation7]. Oral supplementation of blood donors with vitamins C, E, β-carotene can improve some of these RBC storage parameters [Citation8,Citation9].
OS reduction by application of the antioxidant-enriched nutrient mediums offers a possible way to diminish storage lesion. If successful, the development of a simple technology by virtue of adding readily available, cheap and biologically safe antioxidants would increase the shelf-life and improve the quality of stored RBCs. There is limited data on attempt to apply antioxidants as components of the RBC storage solutions. Previous efforts involving dehydroascorbate (DHA)/ascorbic acid (AA) [Citation10] or GSH [Citation11] had brought some encouraging results. Quite recent studies have shown the benefit of addition of AA [Citation12,Citation13], AA and N-acetylcysteine (NAC) [Citation14] or quercetin [Citation15] to the storage solutions but the effects such additives might have on the RBCs are not well understood. The ideal concentrations should be also established in which they shall be added to display the optimal effect. In human blood, vitamin C (vitC) protects biomembranes against peroxidative damage in the aqueous phase and maintains Hb in a reduced state [Citation16]. AA enhances the effects of vitamin E by reducing tocopheroxyl radicals [Citation17]. However, the in vitro AA-induced oxidative hemolysis [Citation18] and increased lipid peroxidation (LPO) [Citation19] in human RBCs has also been reported. Trolox, a water-soluble vitamin E analog, can penetrate membranes and protects mammalian cells against OS [Citation20,Citation21]. It can act synergistically with AA against the micelle lipid auto-oxidation [Citation22].
We investigated the effects of sodium l-ascorbate (SA; BioXtra, ≥99.0%) (5–3125 μM) and the combination of SA and Trolox (25 and 125 μM, respectively) on the oxidative changes – a percentage of hemolysis, extracellular activity of LDH, MDA and GSH concentrations, and total antioxidant capacity (TAC) – generated in RBCs, followed by up to 20 days refrigerated storage.
Materials and methods
Preparation/storage of RBCs
Whole blood units from eight single donors, collected in CPD-anticoagulant preservative solution, were obtained from the Regional Center for Transfusion Medicine in Lodz (Poland). The study was approved by the local Ethics Committee (no KBBN-UŁ/I/4/2011), all individuals gave informed consent to have blood taken. Blood was centrifuged (2800g for 10 min), plasma was removed, and then a buffy coat was removed by aspiration and discarded. The RBC concentrate of 70% hematocrit (Ht) was prepared by addition of the autologous plasma to the remaining packed cells. The RBC concentrate was divided into nine aliquots (volume of 6 ml) of which two served as controls (containing saline), the other seven were stored in the presence of different antioxidant concentrations (SA, Trolox or the combination of both antioxidants). Polystyrene tubes with RBCs were stored at 4 ± 2°C for up to 20 days. Samples were collected at day 1, 10 and 20 after gentle mixing by inversion.
Hemolysis
The rate of hemolysis was calculated based on the measurement of Hb released from the cells, relatively to the total amount of Hb in the RBC suspension. Free Hb concentration was determined by cyanmethemoglobin method using Drabkin’s reagent. The percentage of hemolysis was calculated as described in [Citation23] using the following formula:A supernatant volume was calculated from Ht.
LDH activity
Extracellular LDH activity was measured by following a decrease in absorbance at a wavelength of λ = 340 nm (Spectrophotometer Ultraviolet/Visible (UV/Vis) Helios alpha Unicam, Cambridge, England) resulting from the NADH oxidation [Citation24].
LPO/GSH
LPO was quantified by measuring MDA concentration (expressed as the thiobarbituric acid-reactive substances; TBARS) [Citation25]. Briefly, equal volumes of the RBC suspension (Ht = 4%), 15% (m/v) trichloroacetic acid containing 0.25 M HCl, and 0.375% (m/v) TBA (thiobarbituric acid) containing 0.25 M HCl were mixed, incubated at 95°C for 10 min and cooled. The sample was centrifuged at 6000g for 20 min and absorbance was measured at 535 nm (Spectrophotometer UV/Vis Helios alpha Unicam). Glutathione content was measured spectrophotometrically with Ellman’s reagent. The MDA/GSH concentrations were calculated using the molar extinction coefficients (ε = 156,000/13,600 M−1 cm−1), respectively.
TAC assay
TAC was estimated by the method of Erel [Citation26]. Briefly, 200 µl of 0.4M acetate buffer, pH 5.8 was mixed with 5 µl of the RBC supernatant and the first absorbance was taken at 414 nm (sample blank, A0) (SPECTROstarNano, BMG LABTECH, Germany, Ortenberg). Alternatively, 205 µl of the acetate buffer was taken as a blank (to evaluate a spontaneous discoloration rate). Then, 20 µl of the 10 mM
stock solution was added to each well and the last absorbance at 414 nm (A) was taken at the end of the incubation period (5 min after the mixing). A–A0 was calculated; the reaction rate was calibrated with Trolox, and the assay results were expressed in mmol Trolox equivalent/l.
Statistical analysis
The results shown in figures are means ± standard deviation (SD) of eight independent experiments, each performed in triplicate/quadruplicate. The results were tested for normal distribution by means of the Kolmogorov–Smirnov test. The significance of differences between the mean values of various parameters was analyzed by one-way analysis of variance (ANOVA) followed by Bonferroni’s and Tukey’s post hoc tests. The significance of differences between the mean values of SA (25 µM), Trolox (125 µM) and the combination of SA/Trolox samples were analyzed by the non-parametric Wilcoxon test. The data were analyzed using statistical software StatSoft Inc. ‘Statistica’ v.10. A level p < .05 was accepted as statistically significant.
Results
Effects of the antioxidants on hemolysis and LDH leakage
A time-dependent increases of the degree of hemolysis and extracellular LDH activity in stored RBCs were noticed (approximately 4-fold at day 20; p < .001). As shown in (a) and (a), SA supplemented to the RBCs, did not protect against progressive hemolysis and the LDH leakage. High SA concentrations even increased hemolysis rate and LDH leakage. Significantly lower hemolysis and extracellular LDH were achieved in RBCs stored with a combination of SA (25 µM) and Trolox (125 µM); by above 20% (p < .05), when compared to saline controls ((b) and (b)). The same trend was seen with supplementation of Trolox alone.
Figure 1. The effects of the RBC storage in the presence of vitC (a) or vitC and Trolox (b) on the degree of hemolysis. The results shown are means ± SD of eight independent experiments (n = 8), where n means number of donors; *p < .05 in the presence of antioxidant (antioxidants) at any given day of storage versus in the absence of antioxidants (control) at the appropriate day of storage. The significance of differences between the mean values of various parameters were analyzed by one-way analysis of variance (ANOVA) with Bonferroni correction (Bonferroni post hoc test) (a). The differences between the groups (the mean values of SA (25 μM), Trolox (125 μM) and the combination of SA/Trolox samples) were analyzed by the non-parametric Wilcoxon test (b).
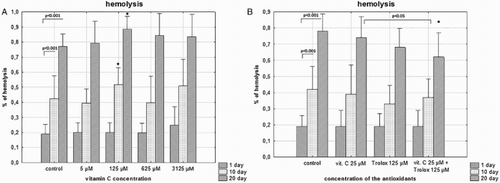
Figure 2. The effects of the RBC storage in the presence of vitC (a) or vitC and Trolox (b) on the extracellular LDH activity. The results shown are means ± SD of eight independent experiments (n = 8), where n means number of donors; *p < .05 in the presence of antioxidant (antioxidants) at any given day of storage versus in the absence of antioxidants (control) at the appropriate day of storage. The significance of differences between the mean values of various parameters were analyzed by one-way analysis of variance (ANOVA) with Bonferroni post hoc test (a). The differences between the groups (the mean values of SA (25 μM), Trolox (125 μM) and the combination of SA/Trolox samples) were analyzed by the non-parametric Wilcoxon test (b).
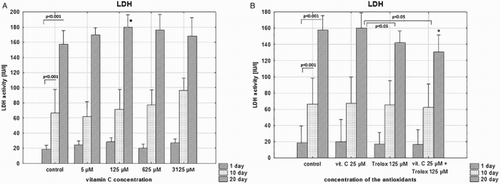
The concentrations of the antioxidants had earlier been experimentally selected. The combinations of higher antioxidant concentrations (125 µM SA/125 µM Trolox; 625 µM SA/625 µM Trolox) provided worse RBC protection in terms of hemolysis, LDH leakage and OS markers described in section below, when compared to 25 µM SA/125 µM Trolox (data not shown).
Effects of the antioxidants on storage-induced oxidative changes
Supplementation of the RBC storage solution with SA, at the concentration of 125 µM, resulted in significant inhibition of storage-induced TBARS generation; by nearly 20% versus control (p < .05), at day 20 ((a)). Similar protective effect was seen in RBCs stored in the presence of 25 µM SA/125 µM Trolox ((b)).
Figure 3. The effects of the RBC storage in the presence of vitC (a) or vitC and Trolox (b) on the TBARS concentration. The results shown are means ± SD of eight independent experiments (n = 8) where n means number of donors; *p < .05 in the presence of antioxidant (antioxidants) at any given day of storage versus in the absence of antioxidants (control) at the appropriate day of storage. The significance of differences between the mean values of various parameters were analyzed by one-way analysis of variance (ANOVA) with Bonferroni post hoc test (a). The differences between the groups (the mean values of SA (25 μM), Trolox (125 μM) and the combination of SA/Trolox samples) were analyzed by the non-parametric Wilcoxon test (b).
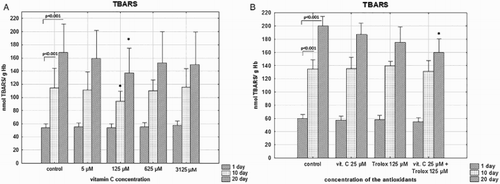
Reduction of the cellular GSH level occurs due to its leakage to the medium and oxidation to GSSG. We did not notice any effect of SA supplementation (5–3125 µM) on GSH depletion (data not shown). RBCs stored in the presence of SA/Trolox maintained higher GSH level, at day 20, compared to these stored for the same time period without the antioxidants ().
Figure 4. The effects of the RBC storage in the presence of vitC and Trolox on the GSH depletion. The results shown are means ± SD of eight independent experiments (n = 8), where n means number of donors; **p < .01 in the presence of antioxidants at day 20 versus in the absence of antioxidants (control) at the 20 day of storage. The differences between the groups (the mean values of SA (25 μM), Trolox (125 μM) and the combination of SA/Trolox samples) were analyzed by the non-parametric Wilcoxon test.
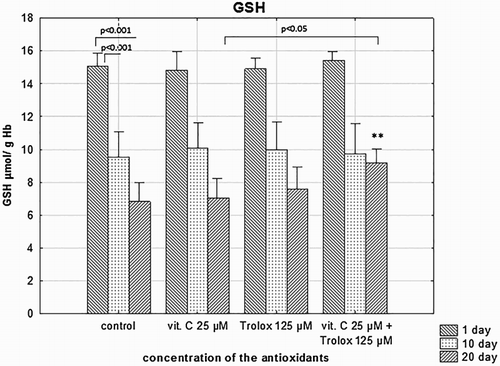
A TAC assay measured the electron- or hydrogen-donating (scavenging) abilities of the sum of antioxidants in the RBC suspending medium. There was a storage-time-dependent decrease of the RBC medium antioxidant capacity due to depletion of endogenous antioxidants, whereas SA had the ability to restore/increase the TAC in a concentration-dependent manner. The high amounts of SA (3 125 µM) kept the TAC elevated in RBC medium up to day 20 ((a)). Addition of Trolox or a combination of SA/Trolox significantly increased TAC of the RBC supernatant at day 1 (p < .05 and p < .001) but after the prolonged storage this effect was not relevant ((b)).
Figure 5. The effects of the RBC storage in the presence of vitC (a) or vitC and Trolox (b) on TAC in the supernatant. The results shown are means ± SD of eight independent experiments (n = 8), where n means number of donors; *p < .05, **p < .01, ***p < .001 in the presence of antioxidant (antioxidants) at any given day of storage versus in the absence of antioxidants (control) at the appropriate day of storage. The significance of differences between the mean values of various parameters were analyzed by one-way analysis of variance (ANOVA) with Tukey’s post hoc test (a). The differences between the groups (the mean values of SA (25 μM), Trolox (125 μM) and the combination of SA/Trolox samples) were analyzed by the non-parametric Wilcoxon test (b).
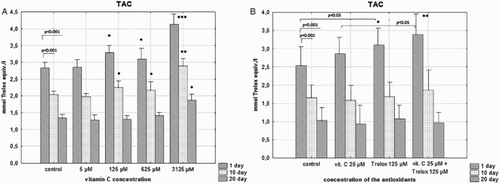
Discussion
RBCs are constantly exposed to the ROS produced by Hb, the oxygen carrier protein that undergoes auto-oxidation to generate the superoxide anion () [Citation27]. Depletion of the intracellular antioxidant defence system increases the susceptibility of stored RBCs to OS. The primary hypothesis of the present study assumed that supplementation of the RBC units with SA or SA/Trolox could restore the extracellular antioxidant capacity which would allow RBCs to better respond to OS. However, the addition of SA alone failed to reduce, at higher concentrations (≥125 µM) it even increased, the storage-induced hemolysis and permeability of the RBC membrane, although a concentration-dependent increase of TAC was achieved. One possible explanation is that SA, at higher concentrations (125–3125 µM), could have promoted radical generation, especially in the medium containing a non-transferrin-bound iron (NTBI). VitC is a potent antioxidant but in the presence of transition metals it can also exhibit the pro-oxidant activity. The non-protein-bound, desferrioxamine-chelatable iron is released from Hb in a number of conditions in which the erythrocyte is subjected to OS [Citation28]. During the RBC refrigerated storage, hem and NTBI are accumulated in the supernatant and can serve as the substrates in Fenton/Haber-Weiss pro-oxidant reactions to produce hydroxyl radical [Citation29,Citation30]. Oxidation of Hb, increased with storage time, produces a number of hem degradation products (i.e. hemin, hematin) and the precipitated globin chains which can induce membrane damage. Hemin has been shown to intercalate into the membrane and greatly disturb the bilayer structure leading to RBC hemolysis [Citation31]. We established that SA did not protect RBCs from storage-induced hemolysis and increased membrane permeability, neither in very low nor very high concentrations. Moreover, a statistically significant hemolysis/permeability raise was seen at 125 µM SA. Based on these findings, it can be suggested that radical generation based on NTBI might initiate higher levels of hemolysis/membrane permeability in high SA concentrations. Although, SA at concentrations of 625 and 3125 µM did not show significant differences to the control, the same tendency to promote hemolysis was noted which imply that such NTBI-radical formation might occur as well. The preliminary experiments conducted in the presence of varying concentrations of desferrioxamine mesylate, a chelator of Fe3+, support this hypothesis. When free iron was sponged up by the chelator, which could prevent the NTBI-radical formation through the Fenton/Haber-Weiss reactions, SA at 625 and 3125 µM protected RBCs from storage-induced hemolysis; however, under the same conditions no such effect was seen at the concentration of 125 µM (data not shown).
In the previous study conducted by Raval et al. [Citation12], no pro-oxidative effect was observed for vitC concentrations at the range of 2.93–8.78 mM. Nonetheless, our results do not remain fairly inconsistent with these findings. Raval et al. reported that treatment of the AS-5-preserved RBCs with AA improved the RBC membrane fragility and decreased hemolysis, over 56 days of the cold storage. Only AA concentrations much higher than in our study (8.78 mM) demonstrated these both beneficial effects on storage days 21 and 42. RBCs treated with 2.93 or 5.86 mM AA did not exhibit significantly lower hemolysis at any of the time points. It has to be noted that the level of OS has not been measured, except Hb oxidation that remained identical between the AA-treated and control units [Citation12]. Studies conducted by Pallotta et al. [Citation14] showed that AA/NAC (0.23/0.5 mM, respectively) supplemented to CPD/SAGM-preserved RBC units contributed to reduction of the OS-related hemolysis due to depression of glucose uptake and promotion of the glutathione homeostasis. However, addition of 0.5 mM AA did not affect the hemolysis rate. Some discrepancies between our findings and others could result from different preparation methods/storage conditions such as storage solutions and/or contamination with white blood cells. As shown earlier [Citation32], despite the fact that qualitative alterations remain largely the same, the noticeable quantitative changes exist between RBCs stored under our experimental storage conditions and the conventionally stored RBC units. The Hb breakdown strongly depends on the amounts of generated through the activated neutrophils/macrophages [Citation2]. This fraction was possibly much smaller in the leukoreduced RBC units used in experiments described in [Citation12,Citation14] than in our experimental system. Consequently, this could result in the increased NTBI-radical formation in the presence of high SA concentrations.
The RBC treatment with 125 µM SA inhibited LPO, with no better protection while using the higher SA doses which may suggest that the relatively small SA concentrations are sufficient to reduce OS ((a)). The same inhibition of LPO has been accomplished by RBC supplementation with SA/Trolox. This could be reasonable, given that the intracellular level of antioxidants may be important for the RBC protection. RBCs have a high capacity to take up DHA and to reduce it to ascorbate, with a half-maximal uptake/conversion of DHA to SA at 400 μM DHA, achieving a maximal intracellular ascorbate concentration of 1.5 mM [Citation33]. As a result, the TAC of the supernatant of RBCs stored with addition of SA or SA/Trolox, at day 20, may not reflect the real antioxidant protective potential.
The major RBC protection was provided by supplementation of the storage solution with Trolox. However, our results show that there is a little advantage of the simultaneous application of SA and Trolox over Trolox alone which can suggest the cooperative action of the antioxidants. Similar to α-tocopherol, Trolox is primarily a chain-breaking antioxidant. Its antioxidant activity is related to the structural characteristics and the ability to interact with/penetrate the lipid bilayers [Citation34]. Due to the hydrophobic nature α-tocopherol distributes totally in the membranes restricting the mobility of their components. Trolox lacks the long fatty acid chain but has the same chroman ring structure, reduction activity and membrane stabilizing potential. Trolox can donate hydrogen from the hydroxyl group to the peroxyl radical converting it into a lipid hydroperoxide and a Trolox phenoxyl radical thus terminating the chain reaction [Citation34,Citation35]. Similar as in other in vitro studies [Citation36], Trolox could also reduce ferryl Hb back to ferric Hb, thereby providing a way for the decay of this highly oxidant species. Treatment of RBCs with SA/Trolox seems to decrease hemolysis and maintain higher GSH levels after 20 days, yet the protective effect of the antioxidants at day 10 is hardly visible (especially the GSH levels at day 10 are comparable to all other data points). Since SA and Trolox are water-soluble antioxidants, their partition and location in the membrane surface are pH dependent. In RBC units pH decreases with storage time due to lactate accumulation. Alteration of pH can change SA and/or Trolox location in the membrane making them more effective as protectors (through interactions/associations with the membrane and/or as a radical scavenger) which could explain protective effects seen at day 20 and little effect of the additive for shorter storage times.
Summarizing supplementation of the storage solution with SA did not ensure expected RBC protection against OS and membrane damage. The impact vitC might have on RBCs is firmly concentration-dependent and may be modulated by a variety of compounds released from red cells during the prolonged storage; e.g. Hb degradation products, cytokines, proteases and/or active lipids can change its properties from anti- to pro-oxidative. Given that individual RBC units considerably vary regarding the levels of bioactive substances in the supernatant and thus how well they are maintained during storage [Citation30] vitC application as a potential additive should be subjected to a strict control. Our results have advanced our understanding of the effects of two common antioxidants on storage lesion but the potential mechanisms of protection remain mostly unknown. It seems that radical-mediated reactions may be responsible for the effects of vitC at lower concentrations, whereas at high concentrations at which the improved membrane fragility/decreased hemolysis were reported [Citation12] some other mechanisms (physical interactions with the membrane and/or osmotic regulation) might also be involved. The two different class antioxidants (SA/Trolox) could possibly cooperate to be good potential RBC storage additives ensuring both antiradical and membrane stabilizing protection.
Ethics approval
This study was approved by the local Ethics Committee (no KBBN-UŁ/I/4/2011).
Disclosure statement
No potential conflict of interest was reported by the authors.
Notes on contributors
Kamila Czubak received her M.Sc. in medical biotechnology in 2014 from the University of Lodz (Poland), Faculty of Biology and Environmental Protection with Prof. H.M. Zbikowska for her work on the role of antioxidants on the oxidative changes in stored red blood cells for transfusion. Currently, she is a third-year student of the full-time biochemical-biophysical doctoral study at the same Faculty of the University of Lodz. Her research interests include the qualitative and quantitative evaluation of proteins, lipids and variety of active mediators that accumulate during routine storage of blood compounds, mechanisms of red blood cell storage-dependent adverse effects seen in transfused patients.
Adam Antosik received his Ph.D. in 2015 from the University of Lodz (Poland) with Prof. H.M. Zbikowska for his work on the role of antioxidants in the maintenance of the structure and properties of red blood cells for transfusion. His research interests include the mechanisms of erythrocyte aging.
Natalia Cichon received her M.Sc. in medical biotechnology in 2013 from the University of Lodz (Poland) with Prof. H.M. Zbikowska for her work on the impact of Trolox and vitamin C on oxidative changes in stored red blood cells for transfusion. Currently, she is a Ph.D. student at Department of General Biochemistry University of Lodz. Her research interests include the effect of electromagnetic field on oxidative stress and neuroplasticity of post-stroke patients.
Halina M. Zbikowska received her Ph.D. in 1996 from the University of Lodz (Poland). After postdoctoral research at the University of Lodz with Prof. B. Wachowicz, in 2000 she joined the group of S. Soukharev at Jerome Holland Laboratory of American Red Cross (Rockville, Maryland) as a research fellow II. She then moved to Clearant Incorporation at Gaithersburg (MD) and joined the group of M. Mc Phee as a scientist. In 2002 she moved back to the University of Lodz and re-joined the group of Prof. B. Wachowicz. In 2008, after being awarded the Doctor of Science degree, she was promoted to associate professor. Her research interests include the role of oxidative stress in hemostasis, oxidative stress in red cell ageing and in stored blood for transfusion, radiation-induced oxidative stress and the mechanisms of protection by plant antioxidants.
Additional information
Funding
References
- Karkouti K. From the Journal archives: the red blood cell storage lesion: past, present, and future. Can J Anaesth. 2014;61:583–586. doi: 10.1007/s12630-013-0106-5
- Hess JR. Red cell storage. J Proteomics. 2010;73:368–373. doi: 10.1016/j.jprot.2009.11.005
- Orlov D, Karkouti K. The pathophysiology and consequences of red blood cell storage. Anaesthesia. 2015;70: Suppl 1:29–37, e9–e12. doi: 10.1111/anae.12891
- Hod EA, Zhang N, Sokol SA, et al. Transfusion of red blood cells after prolonged storage produces harmful effects that are mediated by iron and inflammation. Blood. 2010;115:4284–4292. doi: 10.1182/blood-2009-10-245001
- Pavenski K, Saidenberg E, Lavoie M, et al. Red blood cell storage lesions and related transfusion issues: a Canadian Blood Services research and development symposium. Transfus Med Rev. 2012;26:68–84. doi: 10.1016/j.tmrv.2011.07.003
- Hess JR. Red cell changes during storage. Transfus Apher Sci. 2010;43:51–59. doi: 10.1016/j.transci.2010.05.009
- Kriebardis AG. With or without you: a tale about oxygen removal from stored, packed erythrocytes. Blood Transfus. 2014;12:449–451.
- Knight JA, Blaylock RC, Searles DA. The effect of vitamins C and E on lipid peroxidation in stored erythrocytes. Ann Clin Lab Sci. 1993;23:51–56.
- Racek J, Herynkova R, Holecek V, et al. Influence of antioxidants on the quality of stored blood. Vox Sang. 1997;72:16–19. doi: 10.1046/j.1423-0410.1997.00016.x
- Dawson RB, Hershey RT, Myers CS, et al. Blood preservation XLIV. 2,3-DPG maintenance by dehydroascorbate better than D-ascorbic acid. Transfusion. 1980;20:321–323. doi: 10.1046/j.1537-2995.1980.20380214899.x
- Dumaswala UJ, Wilson MJ, Wu YL, et al. Glutathione loading prevents free radical injury in red blood cells after storage. Free Radic Res. 2000;33:517–529. doi: 10.1080/10715760000301061
- Raval JS, Fontes J, Banerjee U, et al. Ascorbic acid improves membrane fragility and decreases haemolysis during red blood cell storage. Transfus Med. 2013;23:87–93. doi: 10.1111/tme.12013
- Stowell SR, Smith NH, Zimring JC, et al. Addition of ascorbic acid solution to stored murine red blood cells increases posttransfusion recovery and decreases microparticles and alloimmunization. Transfusion. 2013;53:2248–2257.
- Pallotta V, Gevi F, D’Alessandro A, et al. Storing red blood cells with vitamin C and N-acetylcysteine prevents oxidative stress-related lesions: a metabolomics overview. Blood Transfus. 2014;12:376–387.
- Zbikowska HM, Antosik A, Szejk M, et al. A moderate protective effect of quercetin against gamma-irradiation- and storage-induced oxidative damage in red blood cells for transfusion. Int J Radiat Biol. 2014;90:1201–1210. doi: 10.3109/09553002.2013.877173
- Frei B, England L, Ames BN. Ascorbate is an outstanding antioxidant in human blood plasma. Proc Natl Acad Sci USA. 1989;86:6377–6381. doi: 10.1073/pnas.86.16.6377
- Chan AC. Partners in defense, vitamin E and vitamin C. Can J Physiol Pharmacol. 1993;71:725–731. doi: 10.1139/y93-109
- Ibrahim IH, Sallam SM, Omar H, et al. Oxidative hemolysis of erythrocytes induced by various vitamins. Int J Biomed Sci. 2006;2:295–298.
- Knight JA, Voorhees RP, Martin L, et al. Lipid peroxidation in stored red cells. Transfusion. 1992;32:354–357. doi: 10.1046/j.1537-2995.1992.32492263451.x
- Wu TW, Hashimoto N, Wu J, et al. The cytoprotective effect of Trolox demonstrated with three types of human cells. Biochem Cell Biol. 1990;68:1189–1194. doi: 10.1139/o90-176
- Guo C, He Z, Wen L, et al. Cytoprotective effect of trolox against oxidative damage and apoptosis in the NRK-52e cells induced by melamine. Cell Biol Int. 2012;36:183–188. doi: 10.1042/CBI20110036
- Barclay LR, Bailey AM, Kong D. The antioxidant activity of alpha-tocopherol-bovine serum albumin complex in micellar and liposome autoxidations. J Biol Chem. 1985;260:15809–15814.
- Janatpour K, Denning L, Nelson K, et al. Comparison of X-ray vs. gamma irradiation of CPDA-1 red cells. Vox Sang. 2005;89:215–219. doi: 10.1111/j.1423-0410.2005.00699.x
- Wroblewski F, Ladue JS. Lactic dehydrogenase activity in blood. Proc Soc Exp Biol Med. 1955;90:210–213. doi: 10.3181/00379727-90-21985
- Stocks J, Dormandy TL. The autoxidation of human red cell lipids induced by hydrogen peroxide. Br J Haematol. 1971;20:95–111. doi: 10.1111/j.1365-2141.1971.tb00790.x
- Erel O. A novel automated direct measurement method for total antioxidant capacity using a new generation, more stable ABTS radical cation. Clin Biochem. 2004;37:277–285. doi: 10.1016/j.clinbiochem.2003.11.015
- Cimen MY. Free radical metabolism in human erythrocytes. Clin Chim Acta. 2008;390:1–11. doi: 10.1016/j.cca.2007.12.025
- Comporti M, Signorini C, Buonocore G, et al. Iron release, oxidative stress and erythrocyte ageing. Free Radic Biol Med. 2002;32:568–576. doi: 10.1016/S0891-5849(02)00759-1
- Collard KJ, White DL. On the source of the non-transferrin-bound iron which accumulates in packed red blood cell units during storage. Blood Transfus. 2014;12:527–532.
- Spitalnik SL, Francis RO. Oxidative damage and iron effects following RBC transfusion. ISBT Sci Ser. 2015;10: Suppl:1, 219–224. doi: 10.1111/voxs.12145
- Wang F, Wang T, Lai J, et al. Vitamin E inhibits hemolysis induced by hemin as a membrane stabilizer. Biochem Pharmacol. 2006;71:799–805. doi: 10.1016/j.bcp.2005.12.002
- Antosik A, Czubak K, Gajek A, et al. Influence of pre-storage irradiation on the oxidative stress markers, membrane integrity, size and shape of the cold stored red blood cells. Transfus Med Hemother. 2015;42:140–148. doi: 10.1159/000371596
- Mendiratta S, Qu ZC, May JM. Erythrocyte ascorbate recycling: antioxidant effects in blood. Free Radic Biol Med. 1998;24:789–797. doi: 10.1016/S0891-5849(97)00351-1
- Lucio M, Nunes C, Gaspar D, et al. Antioxidant activity of vitamin E and Trolox: understanding of the factors that govern lipid peroxidation studies in vitro. Food Biophysics. 2009;4:312–320. doi: 10.1007/s11483-009-9129-4
- Rezk BM, Haenen GR, Van Der Vijgh WJ, et al. The extraordinary antioxidant activity of vitamin E phosphate. Biochim Biophys Acta. 2004;1683:16–21. doi: 10.1016/j.bbalip.2004.03.005
- Lu N, Chen W, Peng YY. Effects of glutathione, Trolox and desferrioxamine on hemoglobin-induced protein oxidative damage: anti-oxidant or pro-oxidant? Eur J Pharmacol. 2011;659:95–101. doi: 10.1016/j.ejphar.2011.03.009