ABSTRACT
Diabetic nephropathy is the major cause of end-stage renal disease and effective and new therapeutic approaches are needed in diabetic nephropathy and chronic kidney diseases. Oxidative stress and inflammatory process are important factors contributing to kidney damage by increasing production of oxidants. KEAP1/Nrf2/ARE pathway regulates the transcription of many antioxidant genes and modulation of the pathway up regulates antioxidants. NFB controls the expression of genes involved in the inflammatory response. Natural substances have antioxidant and anti-inflammatory activities and have an impact on NFB and KEAP1/Nrf2/ARE pathways. The preclinical studies explored the effectiveness of whole herbs, plants or seeds and their active ingredients in established diabetic nephropathy. They ameliorate oxidative stress induced kidney damage, enhance antioxidant system, and decrease inflammatory process and fibrosis; most likely by activating KEAP1/Nrf2/ARE pathway and by deactivating NFB pathway. Whole natural products contain balanced antioxidants that might work synergistically to induce beneficial therapeutic outcome. In this context, more clinical studies involving whole plants or herbal products or mixtures of different herbs and plants and their active ingredients might change our strategies for the management of diabetic nephropathy. The natural products might be useful as preventive interventions and studies are required in this field.
Introduction
Diabetic nephropathy (DN) is the leading cause of chronic kidney disease (CKD) and end-stage renal disease (ESRD). It is a progressive and irreversible kidney disease that is characterized by initial hyperfiltration, albuminuria, expansion of mesangial matrix, interstitial fibrosis, thickening of basement membranes, and renal cell damage. DN affects 20–30% of the diabetic patients [Citation1].
Many pathways involved in DN have been postulated such as hyperglycemia, oxidative stress (OS), and activation of protein kinase C (PKC) [Citation2–4] (). The upregulation of the receptors for AGE (RAGE) has been implicated as a major mediator in the development and progression of DN. Renal fibrosis, characterized by the accumulation of extracellular matrix protein, results in CKD including DN. It was found that transforming growth factor (TGF-β1) signaling pathway plays a key role in mediating renal fibrosis. Therefore, agents that antagonize TGF-β signaling might be useful for kidney disease therapy.
Table 1. Oxidative stress and postulated pathways involved in diabetic nephropathy.
OS is characterized by increases in reactive oxygen species (ROS) and/or reactive nitrogen species (RNS). RNS and ROS include various agents that contribute to pathological processes in the renal systems. The antioxidant system includes the major ROS scavenging enzymes. Glutathione (GSH) and thioredoxin-2 system are the principal thiol antioxidant systems in mitochondria, which depend on the reducing power of NADPH/NADP+ [Citation5]. GSH is the major endogenous antioxidant produced by the cells [Citation6]. It has been shown that oxidative damage plays an important role in the pathogenesis of DN. At a high level, ROS have an important role in the generation of OS and several inflammatory cytokines that cause damage to protein, lipid, and nuclear DNA [Citation7–9].
In clinical practice, reduction of blood pressure, normalizing blood sugar, and controlling dyslipidemia are the main strategies to ameliorate albuminuria and to treat diabetic patients with DN [Citation2,Citation5]. However, these interventions do not prevent progression to ESRD. Furthermore, various etiologies and many mechanisms of pathogenesis of DN have been postulated, accordingly, multiple interventions have been tested in clinical settings, but they did not show promising outcome. Traditional herbs and spices are important sources of functional foods. They contain antioxidants and many of them showed considerable effects on biological activities and diseases process. Furthermore, many antioxidants, derived from dietary and medicinal plants, have been found to activate redox-sensitive transcription factors, and potentiate the cellular antioxidant or detoxification capability. This paper summarizes beneficial effects of common herbs and spices in DN.
The transcription factors
Nuclear factor-E2-related factor 2 (Nrf2), silent information regulator factor 2-related enzyme 1 (Sirt1), nuclear factor kappaB (NFκB), and antioxidant response element (ARE) are involved in the pathological progression of DN. Nrf2 plays a major role in the regulation of redox homeostasis and the cellular detoxification response. It modulates the gene expression of a number of enzymes to detoxify pro-oxidative stressors [Citation10]. The activation of Nrf2 results in the upregulation of several antioxidant enzymes and cytoprotective genes such as superoxide dismutase (SOD), catalase (CAT), glutathione peroxidase (GPx), and heme oxygenase-1(HO-1). Several Nrf2 activators have been proved effective at activating Nrf2 signaling through different mechanisms in both in vitro and in vivo models of diabetes [Citation11]. It was found that the activation of Nrf2–ARE pathway led to the quenching of ROS overproduction caused by advanced glycation end products (AGEs) [Citation12]. Nrf2 is the key to regulating GSH levels by upregulating enzymes involved in GSH synthesis. Nrf2 increases glutamate cysteine ligase catalyzes (GCL), the rate-limiting step in GSH synthesis [Citation13].
Under physiological conditions, Nrf2 locates in the cytoplasm and combines with its inhibitor Kelch-like ECH-associated protein-1 (KEAP1). During OS, Nrf2 is free from KEAP1 and binds to the genes encoding antioxidant enzymes in the nucleus to increase their expression [Citation14,Citation15]. It was found that Nrf2 has a protective effect on high glucose-induced oxidative damage in the cultured cells and on the diabetic complications in animal models [Citation16]. Hyperglycemia activates the Nrf2 pathway through the generation of ROS in the kidney tissue of human or animals with DN as well as tissue cultures () [Citation17–21].
Figure 1. Role of high blood sugar and transcriptase factors in OS in DM and DN and the effects of natural products. ⊕: activate or stimulate; ⊗: deactivate or inhibit; GSTs: glutathione-s-transferase; OS: oxidative stress; NO: nitric oxide; TNF-α: tumor necrosis factor; GCS: γ-glutamyl-cysteine synthetase; ROS: reactive oxygen species; PCK: protein kinase C; SOD: superoxide dismutase; RNS: reactive nitrogen species; AGEs: advanced glycation end products; NFκB: nuclear factor kappa B; NrF2: nuclear factor erythroid 2-related factor 2; HO1: heme oxygenase-1; NQO1: NAD(P)H quinone oxidoreductase 1; MCP-1: monocyte chemoattractant protein-1; IKK-β: inhibitor of nuclear factor kappa-B kinase subunit beta; IκBα: nuclear factor of kappa light polypeptide gene enhancer in B-cells inhibitor, alpha.
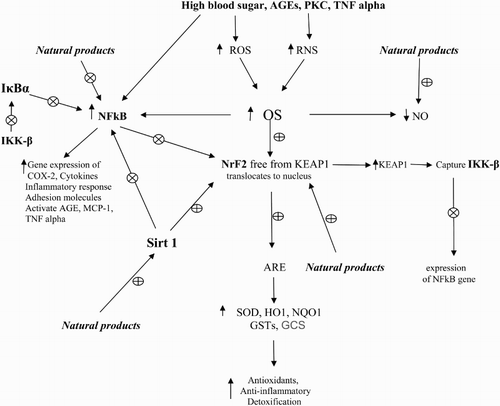
NFκB controls the expression of genes involved in chemokines, inflammatory response, growth factors, cytokines, and adhesion molecules. Increased activation of NFκB was observed in DN in animal and human studies [Citation17,Citation22]. In CKD and anti-GBM-glomerulonephritis, the increased activation of p65/NFκB molecule interacts with KEAP1 in the cytoplasm and prevents the release and translocation of Nrf2 in the nucleus, and in the nucleus, it prevents binding of Nrf2 to the ARE of its target genes [Citation23,Citation24].
IκBα (nuclear factor of kappa light polypeptide gene enhancer in B-cells inhibitor, alpha) is a cellular protein that inhibits the NFκB by masking the nuclear localization signals of NFκB proteins and keeping them sequestered in the cytoplasm and by inhibiting NFκB to bind to DNA. IKK-β, inhibitor of nuclear factor kappa-B kinase subunit beta, causes activation of NFκB and phosphorylates IκBα which is degraded by the ubiquitination pathway. Then NFκB is free and enters into the nucleus of the cell to activate various genes involved in inflammatory reactions.
Sirt1, a member of deacetylation enzymes, can deacetylate NFκB and Nrf2 [Citation25,Citation26]. Sirt1 can release Nrf2 by changing KEAP1 conformation, and then Nrf2 translocates into the nucleus and binds with ARE [Citation26,Citation27]. Sirt1 can inhibit the transcriptional activity of NFκB and reduce the levels of proinflammatory cytokines [Citation28,Citation29]. It was found that Sirt1 is downregulated in diabetic rats [Citation30]. Another study showed that AGEs/RAGE system downregulated Sirt1 expression and promoted TGF-β1 expression in male rat glomerular mesangial cells [Citation31].
Diabetes and oxidative stress
OS and inflammation are involved in the pathogenesis of DN. OS is an intercellular biochemical dysregulation of the redox status, whereas inflammation is the biological response to OS. Data from experimental and diabetic patients showed that increase of OS plays a major role in the initiation and progression of diabetic complications [Citation32,Citation33]. Furthermore, epidemiological studies have demonstrated an association between inflammation and OS markers with cardiovascular disease (CVD) and renal outcomes in CKD and ESRD [Citation34]. Compared with control subjects, antioxidative agents, such as SOD, GPx, CAT, and vitamin C, were decreased in patients with DN. There was a significant increase in serum malondialdehyde (MDA), AGEs, protein carbonyl, and 8-hydroxy-2′-deoxyguanosine in diabetes patients [Citation7]. Data showed that various factors and agents such as inflammatory cells, cytokines, and profibrotic growth factors including TGF-β, monocyte chemoattractant protein-1 (MCP-1), connective tissue growth factor (CTGF), tumor necrosis factor (TNF-α), interleukin (IL) IL-1, IL-6, IL-18, and cell adhesion molecules (CAMs) are involved in the pathogenesis of DN via increased vascular inflammation and fibrosis [Citation10].
Hyperglycemia causes high production of ROS [Citation8,Citation9]. It is known that free radicals cause oxidative damage to the kidney and it contributes to hypertension (HTN)-induced kidney and vascular injury and enhances fibrosis, cell proliferation, and matrix accumulation [Citation35,Citation36].
OS induces NFκB and activation of NFκB was observed in DN in animal and human studies [Citation37,Citation38]. NFκB mediates damages in extracellular matrix, glomerulosclerosis, and renal failure, and provides a molecular mechanism responsible for the inflammation and insulin resistance in diabetes mellitus (DM) [Citation39]. PKC activates NFκB and intensifies the inflammatory response [Citation40]. PKC-βII, isoform of PKC, induces the formation of production of TGF-β, CTGF, AGE, and vascular endothelial growth factor (VEGF) [Citation40,Citation41].
ROS are produced due to hyperglycemia, AGEs, PKC, free fatty acids, cytokines, and TGF-β that activate the NADPH/NADPH oxidase system in renal cells [Citation42,Citation43]. ROS are involved in the expression of mediators of inflammation, fibrosis, and cell death, and these are affected by the activation of NFκB, activator protein-1, and specificity protein-1 [Citation44,Citation45]. Another study showed that GSH level and GSH/GSSG ratio were decreased in diabetic rats [Citation46]. The increased production of ROS could compromise nitric oxide (NO) bioavailability and lead to the formation or activation of vasoconstrictive mediators that could affect tissue perfusion and glomerular filtration [Citation47]. In DM, NO availability is usually decreased, and it might contribute to the vasculopathy present in DN [Citation47]. Endothelial dysfunction has been commonly found in subjects with DN. It was found that blocking endothelial NO leads to an increase in microvascular disease in animals with CKD and arteriosclerosis [Citation48].
Therefore, OS, inflammation, Nrf2, Sirt1, and NFκB have a great role in the pathogenesis and prevention of DN. Factors upregulating Nrf2 and Sirt1 and downregulating NFκB and damping inflammation will contribute to new interventions for the management of DN. Natural products have antioxidant properties and many studies showed that they have important effects on the Nrf2, Sirt1, and NFκB.
Natural antioxidants in diabetic nephropathy
Many natural antioxidants, herbs, seeds, and plants’ extracts were tested in animals’ model of DN. Some of these natural products with their beneficial effects on DN and possible mechanism of action are summarized in .
Table 2. Effects of common plants and spices on kidney function in diabetic animals and human experiments with suggested mechanism of action.
Ginger (Zingiber officinale Roscoe)
Ginger shows an antioxidant activity, and more than 50 antioxidants were isolated from the rhizomes of ginger [Citation49]. Ginger also possessed antioxidants, anti-inflammatory, anticancer, anticlotting, antihyperglycemic, diuretics, and analgesic activities [Citation50–53]. It reduces blood glucose levels (BGL) in STZ-induced DM in rats [Citation54]. The combination of honey and ginger reduced SOD and CAT activities and lowered the MDA level and increased GSH level and GSH/GSSG ratio back to normal level in diabetic rats [Citation55]. Ginger increases insulin release and sensitivity, which involve interaction with the 5-HT3 receptor [Citation56].
Another study demonstrated that ethanolic ginger extract reduces BGL and restores the total carbohydrates, pyruvate, glycogen, and total protein in the kidney tissue of STZ-induced diabetic rats [Citation57]. Furthermore, ginger extract injected intraperitoneally decreased significantly serum glucose and urine protein, and it effectively attenuated the progression of structural nephropathy in diabetic rats [Citation58].
Compounds isolated from ginger (1-dehydro-6-gingerdione, 6-shogaol, and hexahydrocurcumin) increased NAD(P)H quinone oxidoreductase 1 (NQO1) activity and decreased induced nitric oxide synthase (iNOS) activity in LPS-stimulated macrophages [Citation59]. Zerumbone, one of the pungent constituents of Zingiber zerumbet (L) and Smith (Zingiberaceae family), decreased the expression of intercellular adhesion molecule-1, MCP-1, TGF-β1, and fibronectin in the DN [Citation60]. It improved histological architecture in the diabetic kidney. Furthermore, zerumbone suppresses NFκB activation induced by several proinflammatory stimuli by reducing the phosphorylation and degradation of IκBα by interfering with IKK activity [Citation61]. Zerumbone upregulates the expression of ARE-target genes and downregulates NFκB-targets by direct interaction with KEAP1 [Citation62].
Gingerol (100 mg/kg bw), the major pungent component in ginger, significantly decreased blood glucose in db/db type 2 diabetic mice and lowered lipids and fatty acid [Citation63]. Another study showed that gingerol has a protective effect on pancreatic β-cells and it restored the plasma insulin level [Citation64]. Ginger and its derivatives might decrease inflammatory processes in DN as well as increase antioxidants by affecting NFκB activation, expression of ARE-target genes and by interaction with KEAP1.
Turmeric
Curcumin is a famous spice used in cooking and traditional medicine. It is a polyphenol (diferuloylmethane) and it is the main active component of turmeric isolated from the plant Curcuma longa L. It was found that curcumin has many antidiabetic activities which was postulated to be due to the antioxidant property [Citation65,Citation66].
Curcuminoids have an antioxidant activity in several in vitro and in vivo models, such as preventing lipid peroxidation in a variety of cells [Citation67]. Curcumin scavenges superoxide anion (O2¯·), hydroxyl radical (OH), hydrogen peroxide (H2O2) singlet oxygen, NO, peroxynitrite, and peroxyl radical (ROO·) [Citation68,Citation69]. It can induce the expression of SOD, CAT, GPx, HO-1, GST, NQO1, and γ-glutamyl-cysteine ligase [Citation70,Citation71].
The renoprotective effect of curcumin has been evaluated in several experimental models including DN, CKD, ischemia and reperfusion, and nephrotoxicity induced by various compounds. The renoprotective effect of curcumin was due to the induction of Nrf2, inhibition of mitochondrial dysfunction, attenuation of the inflammatory response, preservation of antioxidant enzymes, and prevention of OS [Citation72]. Another study has suggested that curcumin treatment ameliorates DN via inhibition of inflammatory gene expression by reversing caveolin-1 Tyr14 phosphorylation that influenced Toll-like receptor 4 activation [Citation73].
Epithelial–mesenchymal transition plays a key role in DN. Recently, it was suggested that curcumin prevents epithelial–mesenchymal transition of podocytes, proteinuria, and kidney injury in DN by suppressing the phosphorylation of caveolin-1, and increasing stabilization of caveolin-1 and β-catenin [Citation74]. Curcumin protects DN by reducing AGE-induced OS and restoring AGE-induced mesangial cell apoptosis [Citation75]. In the treatment of DN in db/db mice, curcumin decreases albuminuria and attenuates glomerular sclerosis by inhibiting phosphorylation of STAT3 and degradation of IκB [Citation76].
In the clinical setting, it was found that curcumin at the dose of 500 mg/day markedly attenuates urinary micro-albumin excretion, reduces plasma MDA level with enhanced the Nrf2 system specifically regulated protein, NQO-1, together with other antioxidative enzymes in diabetic patients’ blood lymphocytes. After curcumin administration, there was an increased IκB, an inhibitory protein on inflammatory signaling in patient’s lymphocytes [Citation77]. A recent review of 14 randomized controlled studies showed that curcumin significantly lowers mesangial area, proteinuria, blood urea nitrogen (BUN), and serum creatinine (SCr) and protects the kidneys of rats or mice with diabetes [Citation78].
Curcumin activates Nrf2 and HO-1, and protects the NRK-52E cells from the high glucose-induced epithelial-to-mesenchymal transition of mature tubular epithelial cells. The expression levels of Nrf2 and HO-1 protein were elevated to a greater extent in the curcumin-pretreated NRK-52E cells [Citation79]. Furthermore, curcumin effectively attenuates OS, inflammation, and renal fibrosis by modulating Nrf2–KEAP1 pathway [Citation80]. It induced Nrf2 and prevents OS, glomerular hypertension, hyperfiltration, and the decrease in antioxidant enzymes in 5/6 nephrectomized rats [Citation81]. Furthermore, curcumin upregulates HO-1 in a rat smooth muscle cell line and it is a potent activator of Nrf2 [Citation82].
Coenzyme Q10
Coenzyme Q10 (ubiquinone) is an endogenous lipid and a natural antioxidant and scavenging free radicals. It is an oil-soluble vitamin-like substance that is a component of the electron transport chain. It is found in the membranes of many organelles, and it involves in aerobic cellular respiration as an electron carrier for generating energy. A meta-analysis of clinical studies concluded that coenzyme Q10 had the potential for lowering blood pressure in hypertensive patients [Citation83]. It was suggested that a deficiency in mitochondrial oxidized coenzyme Q10 may be a precipitating factor for DN [Citation84]. Coenzyme Q10 attenuates diabetes-induced decreases in antioxidant defense mechanisms and this effect was potentiated by IGF-1 antagonism [Citation85]. Coenzyme Q10 significantly inhibited leukocyte infiltration, glomerulosclerosis and decreased levels of MDA in serum and kidney content. Another study has found that coenzyme Q10 significantly increased serum levels of GSH, CAT, and SOD in the diabetic animals [Citation86]. Furthermore, coenzyme Q10 decreases leukocyte infiltration and glomerulosclerosis and it exerts beneficial effects on the lipid peroxidation and antioxidant enzymes activity in alloxan-induced type 1 diabetic rats [Citation86]. Data from another study demonstrated that coenzyme Q10 prevents altered mitochondrial function and morphology, glomerular hyperfiltration, and proteinuria in db/db mice [Citation87]. Coenzyme Q10 ameliorated glomerulosclerosis and improved tissue antioxidant enzymes in diabetic rats [Citation88].
It was shown that coenzyme Q10 induces Nrf2 nuclear translocation, augments the cellular antioxidant defense capacity and attenuates H2O2-induced in PC12 cells [Citation89]. Furthermore, solubilized coenzyme Q10 inhibited dimethylnitrosamine-induced liver fibrosis through suppression of TGF-β1 expression via Nrf2/ARE activation in mice [Citation90].
Green tea
Green tea (Camellia sinensis L.) has antioxidant and anti-inflammatory properties. It contains strong antioxidative flavonoids. Green tea polyphenols have a beneficial effect on pathological entities related to OS of renal tissues [Citation91]. It protects kidney against gentamycin, cyclosporine, and intravenous contrast [Citation92,Citation93]. In randomized clinical trial, it was found that green tea polyphenol reduces albuminuria in diabetic patients receiving the maximum recommended dose of renin–angiotensin inhibition [Citation94]. In this study, podocyte apoptosis and in vitro albumin permeability were higher in human podocytes exposed to plasma from diabetics compared to podocytes treated with plasma from normal individuals; therefore, it was suggested that reduction in podocyte apoptosis by activation of the WNT pathway may have contributed to the green tea effect.
Green tea treatment significantly reduced BGL, glycosylated protein (HbA1c), SCr, and BUN in diabetic rats. In addition, the green tee-treated group showed a significant higher creatinine clearance compared with the untreated diabetic rat and green tea also prevented glycogen accumulation in the renal tubules [Citation95]. Another study showed that green tea polyphenols increased the activity of SOD in the kidney rats that had been subjected to subtotal nephrectomy and injection of STZ [Citation96]. In mice, green tea extract increased Nrf2 and NQO1 mRNA, and lowered hepatic steatosis, MDA, lipid uptake and lipogenic gene expression, and NFκB-dependent inflammation [Citation97].
The green tea catechins have potent anti-inflammatory and antioxidant agents. They inhibit chelate transition metals, leukocyte chemotaxis, quench free radicals, and lipid peroxidation chain reaction [Citation98]. It has been demonstrated that high doses of catechins-rich OPLE for 4 weeks in STZ-induced diabetic rats attenuate proteinuria, hyperfiltration, glomerulosclerosis, and tubulointerstitial fibrosis and causes suppression of 8-hydroxy-2′-deoxyguanosine, lipid peroxides, and TGF-β1 [Citation99]. Another study showed that catechin has renoprotective properties comparable with renin–angiotensin inhibition, and co-administration with enalapril might be useful in reducing albumin excretion as well as improving endothelial function [Citation100].
Epigallocatechin-3-gallate is the most abundant and most active catechin polyphenol extracted from green tea. It could restore unilateral ureteral obstruction-induced renal dysfunction, and prevent unilateral ureteral obstruction-induced OS and inflammatory responses in the obstructed kidney. Furthermore, epigallocatechin-3-gallate could induce both NFκB and Nrf2 nuclear translocation in the unilateral ureteral obstruction kidney and promote HO-1 production [Citation101]. Epigallocatechin 3-O-gallate reversed an increase in the levels of BGL, BUN, SCr, and urine protein in STZ-induced DN in mice [Citation102]. Therefore, epigallocatechin 3-O-gallate might provide an effective protection against STZ-induced DN in mice by osteopontin suppression [Citation102].
Epigallocatechin-3-gallate activates Nrf2–ARE and peroxisome proliferator-activated receptor pathway, diminishes AKT/ERK/NFκB pathways to reduce OS and inflammation, and activates Sirt1 in mice with crescentic glomerulonephritis [Citation103]. Moreover, it upregulates Nrf2 signaling and ameliorates cisplatin-induced acute kidney injury and lupus nephritis in animal models [Citation104,Citation105].
Guava (Psidium guajava)
Guava (Psidium guajava) is one of the popular tropical fruits rich in vitamin C, fiber, and phenolic compounds. Psidium cattleianum Sabine (Myrtacea) is rich in vitamin C and phenolic compounds, including epicatechin and gallic acid as the main components. It was considered as a good source of natural antioxidants [Citation106]. Psidium guajava is an evergreen small tree or shrub whose origin is America. Its extract contains caffeic acid, myricetin, and quercetin, cinnamic, coumaric and ferulic acids [Citation107]. This fruit extract dose-dependently reserved GSH content, retained activity of CTA and GPx, and decreased ROS, IL-6, TNF-α, and IL-1β levels in the kidney and therefore, it can protect the kidney against diabetic progression via its antioxidative, anti-inflammatory, and anti-glycative effects [Citation107]. Extract of Psidium guajava possesses a significant free radical scavenging and antioxidant activities [Citation108]. Total triterpenoids from Psidium guajava leaves decrease the level of BGL of diabetic rats, increase the insulin sensitivity index, and protect renal lesions in diabetic rats [Citation109]. It also suppressed renal activity of aldose reductase. The levels of BUN and SCr were decreased and the renal structural damages were improved with the use of Psidium guajava leaves in STZ-induced diabetic rats [Citation109].
It was found that the flavonoid fraction of guava leaf extract inhibits LPS-induced NO and PGE2 production, TNF-α, IL-1β, IL-10, iNOS, COX-2, and LPS-induced NFκB transcriptional activity in diabetic mice [Citation107]. The flavonoid fraction of guava leaf extract suppresses the expression of inflammatory mediators that involves the inhibition of NFκB activation through the suppression of LPS-induced IκB-α degradation [Citation110].
Rosa laevigata Michx
Rosa laevigata Michx is a well-known medicinal plant, and it has been widely used in China for a long time. Previous studies revealed that the total flavonols fraction from the Rosa laevigata Michx has potent antioxidant, hypolipidemic, hepatoprotective, and antithrombotic activities through attenuating inflammation, suppression of apoptosis, and altering mitogen-activated protein kinase signaling pathways [Citation111]. Another study showed that Rosa laevigata Michx significantly ameliorates renal dysfunction in diabetic rats [Citation112]. The protection involves increasing the activity of SOD and total antioxidant capacity, decreasing the levels of MDA and ROS, and inhibiting the expression of NFκB p65 and MCP-1 at both the protein and mRNA levels with an increase in the expression of the IκBα protein [Citation112].
Total flavonoids from Rosa laevigata Michx fruit attenuated cell injury and decreased SCr and BUN levels in rats with ischemia/reperfusion injury [Citation113]. They also decreased the levels of MDA, SOD, GSH and GPx, and ROS, upregulated the levels of Nrf2, Sirt1, and HO-1, downregulated the levels of KEAP1 and NFκB p65, and decreased the mRNA levels of IL-6, IL-1β, and TNF-α. Furthermore, inhibiting Sirt1 attenuated the protecting effect of the total flavonoids on renal ischemia/reperfusion injury, suggesting that the effect of the extract depended on Sirt1. Therefore, the total flavonoids have nephroprotective effect against ischemia/reperfusion injury by affecting Sirt1/Nrf2/NFκB signaling pathway 116].
Garlic
Large data from human and animal studies have reported the health-promoting properties of garlic (Allium sativum L.), a widely used condiment and spice. Many studies have shown that fresh garlic has the antidiabetic properties. Raw garlic extract is active in reducing hyperglycemia and alleviating dyslipidemia and OS in both animal models and humans [Citation114–117].
In diabetic rats, garlic showed normalization or attenuation in plasma and kidney ACE-1 and angiotensinogen II, normalization of blood pressure, and renal clearance function [Citation114]. RAGE was significantly increased in renal and hepatic tissues of diabetic rats, which involved mesangial cells in glomeruli exhibiting signs of mesangial expansion, mesangial nodule formation, and glomerulosclerosis. Garlic significantly reduced RAGE throughout renal and hepatic regions [Citation118]. In diabetic rats, the use of the garlic extract (500 mg/kg body weight) caused a significant decrease in the expression of VEGF and extracellular signal-regulated kinase-1 compared to diabetic rats, and attenuating mesangial expansion and glomerulosclerosis [Citation119].
Garlic increased the serum antioxidant levels after 3 weeks of treatment in both diabetic and hypertensive rats. It decreased BGL and lowered systolic blood pressure [Citation116]. Another study showed that fresh garlic homogenate significantly attenuated STZ-induced DN by attenuation of BGL, insulin, total triacylglycerol, total cholesterol, and creatinine clearance in rats. Urinary excretions of albumin and N-acetyl-beta-d-glucosaminidase were reduced. In addition, a marked improvement of GSH content in the kidney homogenates was also observed. It enhanced urinary excretion of nitrites [Citation120].
Aged garlic extract is produced by storing sliced and macerated fresh garlic at room temperature for 20 months. Aged garlic extract contains many of the bioactive compounds found in fresh garlic including flavonoids and saponins, the water-soluble allyl amino acid derivatives, stable lipid-soluble allyl sulfides, and S-allylcysteine and S-allylmercaptocysteine [Citation121]. Aged garlic extract exhibits a dose-dependent ameliorative action on indicators of DM including serum insulin, total antioxidant level, and CAT activity in the serum, kidney, and liver in STZ-induced diabetic rats [Citation122]. In animal models, it was found that aged garlic extract lowers OS by increasing endogenous antioxidant enzymes, such as CAT and GPx, and lowering xanthine oxidase and NADPH oxidase [Citation123,Citation124]. Aged garlic extract reduces the formation of superoxide and lipid peroxidation [Citation125]. Aged garlic activated the Nrf2–ARE signaling pathway and induced the expression of antioxidant enzymes HO-1 and GCL modifier subunit [Citation126].
Allicin is a major biologically active component of garlic. A study showed that allicin treatment for 12 weeks ameliorated diabetes-induced morphological alterations of the kidney and decreased BGL, BUN, SCr, triglyceride, and 24 h urine albumin excretion in diabetic rats. It decreased the expression levels of collagen I, TGF-β1, and p-ERK1/2 [Citation127].
S-allylcysteine is a garlic-derived antioxidant. Data showed that S-allylcysteine (25 mg/kg) prevents the cisplatin-induced renal damage and attenuates cisplatin-induced decrease in Nrf2 levels and increase in PKCβ2, p47(phox) and gp91(phox) expression in renal cortex and OS, and decrease in the activity of CAT, GPx, and glutathione reductase (GSR) in the proximal and distal tubules [Citation128]. Recently, it was shown that S-allylcysteine activates Nrf2 factor in cerebral cortex [Citation129]. Diallyl trisulfide is the most powerful antioxidant among the sulfur-containing compounds in garlic oil. The study demonstrated that diallyl trisulfide protects against hyperglycemia-induced ROS-mediated apoptosis by upregulating the PI3K/Akt/Nrf2 pathway, which activates Nrf2 in cardiomyocytes exposed to high glucose [Citation130].
Black seed (Nigella sativa)
Black seed is composed of fixed and essential oil. Dihomo-γ-lionoleic acid presents in the fixed oil of seeds and it has a powerful antioxidant [Citation131]. It contains vitamin E, vitamin A, β-carotene, and thymoquinone. It was found that ethanol extract of Nigella sativa seeds to STZ-induced diabetic rats for 30 days significantly reduced the elevated levels of BGL, and improved levels of CAT, SOD, reduced GST and GPx in the liver and kidney [Citation132]. Results showed that vitamin C and Nigella sativa oil lowered the SCr, BUN, and antioxidant activity as compared to gentamicin control group values. When these were given as combination, they have synergistic nephroprotective effect [Citation133]. Pretreatment with black seed for 3 weeks prior to ischemia reperfusion injury improved renal function and reduced renal inflammation and oxidative injury [Citation134]. It was suggested that the antidiabetic properties of Nigella sativa seeds may be mediated by stimulated insulin release [Citation135]. In STZ-induced diabetic rats, black seed oils significantly induced the gene expression of insulin receptor and they upregulated the expression of IGF-1. Also they significantly reduced BGL, OS parameters, serum insulin/insulin receptor ratio, and TNF-α [Citation136].
Thymoquinone, an active quinone, that is the main component of black seed, has antioxidant, anti-inflammatory, and antidiabetic effects. A study showed that thymoquinone has markedly reduced renal damage induced by doxorubicin with the restoration of decrease in IL-10 levels, Nrf2 mRNA levels towards normal values [Citation137]. Thymoquinone is effective in protecting rats against N(omega)-nitro-l-arginine methyl esters-induced HTN and renal damage [Citation138].
Thymoquinone decreased MDA and 8-isoprostane levels in renal tissues and reduced levels of SCr and BUN concentration [Citation139]. It was found that the protective effect of thymoquinone in STZ-induced diabetic rats is mediated via inhibiting the phosphorylation of mitogen-activated protein kinases production of NO by iNOS enzyme [Citation140]. In a model of vancomycin- or gentamycin-induced nephrotoxicity in rats, thymoquinone caused reversal of the increase in BUN, SCr, and lipid peroxides [Citation141,Citation142].
Thymoquinone significantly abolished LPS-induced proinflammatory cytokines, such as IL-1β, TNF-α, COX-2, and prostaglandin E2 [Citation143]. Oral administration of thymoquinone (80 mg/kg) to diabetic rats for 45 days reversed the decreased activities of CAT, GPx, and glutathione-S-transferase (GST), and increased GSH and vitamins C and E [Citation144]. Nigella sativa oil and thymoquinone prevented diabetes-induced downregulation of mRNA expression of the podocyte-specific marker and the mRNA over expressions of collagen IV, TGF-β1, and VEGF in the diabetic kidney, and ameliorated albuminuria [Citation145]. It was suggested that the anti-inflammatory activities of black seed and thymoquinone may be mediated through the suppression of NFκB activation [Citation146].
Panax ginseng
The root of Panax ginseng has been widely used for the management of many diseases, including cancer, DM, and CVD for thousands of years [Citation147,Citation148]. Ginsenosides, main pharmacologically active constituents of ginseng and the secondary metabolites of the Panax species, have antioxidants and free radicals scavenging properties [Citation149]. It reduced the cisplatin-induced nephrotoxicity in cultured renal proximal tubular epithelial cells in a dose-dependent manner [Citation150]. North American ginseng has preventive effects on DN and it works through its antihyperglycemic and antioxidant activities [Citation151].
In type 1 insulin-dependent DN animal models induced by STZ, it was found that sun ginseng, heat-processed American ginseng, and 20(S)-ginsenoside Rg3 ameliorated hyperglycemia and renal damage [Citation152]. In type 2 insulin-independent DN animal models, ginsenoside Rg3 decreased hyperglycemia and proteinuria and augmented creatinine clearance [Citation111].
Panaxytriol, an active component of red ginseng extracts, is a potent ARE inducer. The upregulation of aldo–keto reductase enzymes, induced by chemically homogeneous panaxytriol, was partially dependent on PKC and PI3K kinases. Aldo–keto reductase enzymes play an important role in the transformation and detoxification of aldehydes and ketones generated during drug detoxification and xenobiotic metabolism. This cellular mechanism may account for panaxytriol’s neurotrophic, neuroprotective, and anticancer properties [Citation153]. It has been shown that ginseng induces NQO1, a phase 2 detoxification enzyme protect against carcinogenesis and OS, and the most potent inducing red ginseng extract has the highest panaxytriol content [Citation154].
Fenugreek (Trigonella foenum-graecum)
Fenugreek (Trigonella foenum-graecum) is used as a daily diet of the general population in many countries. It was found that extractions from fenugreek seed have antidiabetic and antioxidant effects. Fenugreek has a favorable function on peripheral glucose utilization and insulin secretagogue actions [Citation155]. Furthermore, another study showed that fenugreek ameliorates SCr in diabetic animals [Citation156]. In diabetic rats, treatment with fenugreek for 12 weeks reduces the high levels of glucose, BUN, SCr, and IL-6. It also reduced the levels of MDA and IL-6 in the kidney homogenate and increased the concentration of GSH and the activity of both SOD and CAT. These results suggest a therapeutic potential of fenugreek against DN by its antioxidative/anti-inflammatory properties [Citation156]. In STZ-induced DN in rats, fenugreek treatment significantly reduced BGL, improved renal functions, suppressed ECM accumulation, and it relieved OS with the restrained TGF-β1/CTGF signaling pathway [Citation157]. Cisplatin administration triggered inflammatory responses and apoptosis in rat livers and kidneys by increased expression of pro-inflammatory cytokine, TNF-α, and apoptotic marker p38 mitogen-activated protein kinases; results of overproduction of ROS. Fenugreek significantly attenuated the cisplatin-induced biochemical and histopathological alterations, inflammation, and apoptosis in rat kidneys. The results suggested that fenugreek has a powerful antioxidant effect [Citation158].
Acrylamide intoxication significantly increased serum levels of LDH, AST, ALT, APL, γ-GT, cholesterol, uric acid, BUN, SCr, 8-oxo-2′-deoxyguanosine, IL-1β, IL-6, and TNF-α. Fenugreek oil supplementation normalized the altered serum parameters, prevented lipid peroxidation, and enhanced the antioxidant biomarker concentrations and activities in the hepatic, renal, and brain tissues in a dose-dependent manner [Citation159].
Treatment with GII, a water-soluble compound purified from fenugreek seeds, for 15 days in the subdiabetic and moderately diabetic rabbits and for 30 days in the severely diabetic rabbits decreased the elevated TC, TG, LDL, VLDL and increased the decreased HDL, increased the decreased SOD and GPx. Therefore, the treatment with GII compound corrects the altered polyol pathway and antioxidant enzymes [Citation160].
Another study showed that Trigonella foenum-graecum seed aqueous extract confers protection against functional and morphologic injuries in the kidneys of diabetic rats by increasing activities of antioxidants and inhibiting the accumulation of oxidized DNA in the kidney [Citation161]. In addition, glycosides fenugreek seed extract showed anti-fibrotic efficacy through induction of Nrf2 in rats with bleomycin-induced pulmonary fibrosis [Citation162].
Rosmarinus officinalis
Rosmarinic acid is a polyphenolic phytochemical, found in Perilla, rosemary and mint; it is the main constituent of Rosmarinus officinalis. It has antioxidant and anti-inflammatory properties [Citation163]. Rosmarinic acid exerts an early renal protective role to DN by inhibiting CTGF [Citation164]. It significantly decreased focal glomerular necrosis, dilatation of Bowman’s capsule, degeneration of tubular epithelium, necrosis in tubular epithelium, and tubular dilatation [Citation165]. DM caused a significant decrease in the activity of SOD and CAT in rats. The treatment with rosmarinic acid (10 mg/kg) prevented the alteration in SOD and CAT activity and it reversed the decrease in ascorbic acid and non-protein-thiol levels in diabetic rats [Citation166].
Rosmarinic acid has antioxidant and anti-inflammatory activity [Citation163,Citation167,Citation168]. It prevented ischemia/reperfusion injury in the kidneys by decreasing OS [Citation165]. Furthermore, rosmarinic acid was found to reduce NFκB, and increase glutathione transferase, anti Bcl-2 activity, and scavenger of peroxynitrite [Citation169,Citation170]. It conserves the glomerular number in diabetic rats and ameliorates glomerulosclerosis [Citation171]. Furthermore, it was found that rosemary extract can inhibit the increased serum MDA in treated diabetic rats [Citation172]. Other studies showed that rosmarinic acid improves activity of kidney antioxidants, such as SOD, GPx, and CAT, in gentamicin-induced nephrotoxicity and ameliorated DN in rats [Citation173].
Carnosic acid, a phenolic diterpene isolated from Rosmarinus officinalis, exerts anti-inflammatory, antioxidant, and anticarcinogenic activities. It was found to cross the blood–brain barrier to support neuronal growth, and upregulates the production of neural protection factors in an Nrf2-dependent manner [Citation174,Citation175]. Furthermore, it was found that carnosic acid activated Nrf2 through modulation of PI3K/Akt pathway resulting in increased levels of antioxidant enzymes [Citation176]. Carnosic acid prevented methylglyoxal-dependent neurotoxicity by activating the PI3K/Akt/Nrf2 signaling pathway and the antioxidant enzymes modulated by Nrf2 transcription factor [Citation177].
Grape seed
Whole grape-based diet increased cardiac GSH and GSR activity in hypertensive rats [Citation178]. Grape-derived phytochemicals, resveratrol and pterostilbene, were both protective against azoxymethane-induced colon carcinoma by inhibiting NFκB activation and subsequent iNOS and COX-2 expression, concomitant with increased ARE-responsive HO-1 and GSR [Citation179].
Grape seed proanthocyanindin extract, a polyphenol compound derived from grape seeds, has antioxidant, anti-inflammatory, and antitumor activities, and it mediates resistance to free radicals and has protection from CVD [Citation180,Citation181]. Its antioxidant activity is 50 times higher than that of vitamin E and 20 times higher than that of vitamin C. It has been shown that this extract could downregulate OS proteins and increase renal antioxidant enzyme activity and ameliorate DN [Citation182,Citation183]. Another study showed that it can decrease proteinuria, attenuating the progression of nephropathy in diabetic rats that are correlated with suppression on AGEs/RAGE axis, and downregulating expression of CTGF [Citation184]. Furthermore, it was found that proanthocyanidins protect the kidney of diabetic rats by increasing the renal antioxidative ability [Citation185].
A recent study showed that proanthocyanidins decreases fasting BGL, serum insulin, HbA1c, and systolic blood pressure, improves renal function parameters, reduces the expression of tissue inhibitor of metalloproteinase1 and increases the activity of matrix metalloproteinase in diabetic rats. It also increased the activity of antioxidant enzymes and reduced the levels of CRP and the expression of TNF-α, MCP-1, and ICAM-1 in the kidney [Citation186]. It was found that proanthocyanindin significantly decreases lipid peroxidation and augments the activities of antioxidant enzymes in the kidney of DN rats and also reduces albuminuria. It was suggested that these results were due to the reduction of the OS and increase in renal antioxidant enzyme activity [Citation182]. In rat mesangial cells treated with high-dose glucosamine, the administration of grape seed procyanidin B2 significantly increased the viability of mesangial cells, inhibited apoptosis, and suppressed OS. It activated the protein expression of PPARγ co-activator-1α, SIRT1, and AMP-activated protein kinase in mesangial cells. It was suggested that grape seed procyanidin B2 ameliorates mitochondrial dysfunction and inhibits apoptosis due to the activation of the AMPK-SIRT1-PGC-1α axis [Citation187].
Grape seed proanthocyanindin causes a significant improvement in renal OS markers in the kidneys of cadmium-treated rats, decreases the amount of iNOS, NFκB, TNF-α, caspase-3, and Bax and increases the levels of Bcl-2 protein expression. Furthermore, it normalizes the renal expression of Nrf2/Keap1 and its downstream regulatory proteins. These results suggested that proanthocyanindin ameliorates renal dysfunction and OS through the activation of the Nrf2 pathway in cadimum-intoxicated rats [Citation188].
Ginkgo biloba
Ginkgo biloba has a number of benefits including scavenging ROS [Citation189]. Extract of Ginkgo biloba could inhibit AGEs production and downregulate RAGE expressions by reducing OS, and improve the renal tissue structure and renal functions of DN rats [Citation190]. Furthermore, Ginkgo biloba extract has a protective property against glomerulosclerosis of mesangial cells in DN [Citation191]. Data showed that Ginkgo biloba decreased BGL, SCr, BUN, urine protein, and the intensity of the OS in DN rats. It reduced AGE, collagen IV, laminin, TGF-β1 mRNA, CTGF, mesangium hyperplasia, and thickness of GBM [Citation192]. A systemic review of randomized controlled trials conducted on adults with early DN showed that Ginkgo biloba extract decreased the urinary albumin excretion, BGL, SCr, and BUN [Citation193].
Endothelial progenitor cells are precursor cells that can differentiate into vascular endothelial to form new blood vessels. These cells could be damaged by OS in DM [Citation194]. It was found that Ginkgo biloba can improve SOD activity and reduce the rate of apoptosis of endothelial progenitor cells within the peripheral blood of diabetic patients [Citation195].
In mouse C2C12 myoblasts, Ginkgo biloba extract shows that cytoprotective effects from OS induced by alcohol depend on the transcriptional upregulation of HO-1 via the major mitogen-activated protein kinases/Nrf2 pathway. Furthermore, Ginkgo biloba extract produced an increase in Nrf2 and upregulation of HO-1 [Citation196]. It could inhibit cytokine-induced endothelial adhesiveness by inducing HO-1 expression via the activation of p38 and Nrf-2 pathways. Ginkgo biloba extract might exert its anti-atherogenesis and vascular protective effects by inducing vascular HO-1 expression [Citation197]. It could reduce leukocyte adherence to injured arteries, and enhance HO-1 expression in circulating monocytes and in blood vessels.
Flaxseed (Linum usitatissimum)
Flaxseed (Linum usitatissimum) contains plenty of omega-3 fatty acid. Therefore, flaxseed oil may prevent many diseases such as CVD, HTN, cancer, skin diseases, renal failure, rheumatoid arthritis, and multiple sclerosis [Citation198]. Data showed that it has favorable effects on renal injury [Citation199]. Flaxseed also prevents heavy metals-induced nephrotoxicity and OS damage [Citation200]. It was found that flaxseed can reduce proteinuria and glomerular and tubulointerstitial lesions in obese SHR/N-cp rats [Citation201]. It was found that flaxseed may have beneficial effects on DN by reducing the levels of the OS and increasing the antioxidant defense systems [Citation202]. Flaxseed and Pumpkin seed mixture supplemented in diet may be helpful to prevent DM and its complications in diabetic rats [Citation203].
Flax seed ,walnuts, and fish oil contain omega 3. Omega-3 fatty acids, such as α-linolenic acid, eicosapentaenoic acid, and docosahexaenoic acid, are polyunsaturated fatty acids that have anti-inflammatory and antioxidant activity. Omega-3 has antithrombotic, antihyperlipidemic, antiatherogenic, and anti-inflammatory actions [Citation204,Citation205]. Omega-3 can slow disease progression in human and experimental DN. It was suggested that omega-3 can ameliorate glomerular lesions by the inhibition of thromboxan A2 and B, prostaglandin E, and prostaglandin I, activating protein-1, MCP-1, and mitogen-activated protein kinase/extracellular signal-regulated kinase signaling [Citation205,Citation206]. The omega-3 polyunsaturated fatty acids docosahexaenoic acid and/or eicosapentaenoic acid protect rodents against ischemic acute renal failure, IgA- or cyclosporine A-induced nephrotoxicity, and STZ-induced type 1 and type 2 DN [Citation207,Citation208]. It was found that TAK-085, a concentrated formulation of omega-3 polyunsaturated fatty acids, can attenuate albuminuria and renal dysfunction in type 2 diabetic db/db mice [Citation209]. The omega-3 polyunsaturated fatty acids protect against DN by inhibiting inflammation [Citation210]. During tarcolimus use, it was suggested that renoprotective effects of long-chain omega-3 fatty acids derived from fish oil may be attributed to decreased production of inflammatory mediators, decreasing the generation and expression of proinflammatory cytokines, and a block of angiotensin II signaling in the kidney [Citation211]. Another study demonstrated that omega-3 fatty acids lowered several components of OS and markers of inflammatory and fibrotic response and they attenuated tubulointerstitial fibrosis and inflammation in the remnant kidney [Citation212].
Cinnamon (Cinnamomum zeylanicum l)
Cinnamon is a well-known spice and it is the second most important spice used in the USA and European markets. It has antimicrobial, antioxidant, antiulcerogenic, antidiabetic, and analgesic effects [Citation213,Citation214]. Cinnamon is beneficial for the health of patients with type 2 diabetes [Citation215].
The oils and extracts from Cinnamomum zeylanicum L. possess a powerful antioxidant activity due to the presence of phenolic and polyphenolic substances [Citation216]. Cinnamomum zeylanicum demonstrated numerous beneficial effects both in vitro and in vivo as a potential therapeutic agent for DM, and it also showed beneficial effects against diabetic neuropathy and DN [Citation217]. It reduces BSL. Furthermore, studies showed that polyphenols from cinnamon have in vitro and in vivo insulin-enhancing biological activity [Citation218]. The volatile oil from cinnamon contains more than 98% cinnamaldehyde and that it showed a dose-dependent significant protection against alloxan-induced renal damage [Citation219]. Cinnamon can inhibit AGE, and its procyanidin-B2 – fraction ameliorates the diabetes-mediated renal malfunction in rats by reducing urinary albumin and creatinine [Citation220].
Trans-cinnamic aldehyde (cinnamaldehyde), the key flavor compound in cinnamon essential, was found to be an inducer of Nrf2 transcriptional activity. Cinnamaldehyde treatment upregulates cellular protein levels of Nrf2, GSH levels, and HO-1 and gamma-glutamyl-cysteine synthetase. Cinnamaldehyde pretreatment strongly upregulates cellular GHS levels and protected HCT116 cells against H2O2-induced genotoxicity and arsenic-induced oxidative insult in cultured human epithelial colon cells [Citation221]. The volatile oil from cinnamon contains more than 98% cinnamaldehyde and that shows a dose-dependent, significant protection against alloxan-induced renal damage [Citation219]. In vascular endothelial cells treated with TNF-α, cinnamaldehyde pretreatment prevented NFκB activation by inhibiting IκBα, resulting in reduced expression of VCAM-1 and ICAM-1 [Citation222]. Cinnamaldehyde exerts its anti-inflammatory effects by blocking the degradation of the inhibitory protein IκBα, and by the induction of Nrf2-related genes, including HO-1. In addition, cinnamaldehyde upregulates Nrf2, increases ARE-luciferase activity, and upregulates thioredoxin reductase-1, another Nrf2-related gene.
Astragalus membranaceus
Astragalus membranaceus is a flowering plant in the family Fabacceae which is commonly used in traditional Chinese medicine. It is used to strengthen immune system and help wound healing.
It has anti-inflammatory and antioxidant activity [Citation223,Citation224]. A meta-analysis comprising 25 studies showed that Astragalus had a therapeutic effect in DN patients, such as decreasing BUN, SCr, and urine protein and improving creatinine clearance and serum albumin level [Citation225]. A review of 13 reports focusing on animal models showed that the Astragalus membranaceus are effective in reducing BGL and albuminuria, in reversing the glomerular hyperfiltration state, and in ameliorating the pathological changes of early DN in rat models [Citation226].
Radix astragali, the dried root of perennial herbs Astragalus membranaceus, was reported to upregulate c-met (a receptor tyrosine kinase) expression in human kidney fibroblasts to delay the progression of DN [Citation227]. Two major isoflavonoids in radix astragali, calycosin and calycosin-7-O-beta-d-glucoside, could inhibit high glucose-induced mesangial cell early proliferation and AGEs-mediated cell apoptosis [Citation228]. Astragaloside IV, active constituent in radix astragali, inhibits human tubular epithelial cells apoptosis and reduces TGF-β1 expression probably by the inhibition of p38 MAPK pathway activation [Citation229].
The mechanisms of Astragalus membranaceus action in DN are based on the reduction in the mRNA level of NFκB, and an increase in inhibitory NFκB protein mRNA expression in the renal cortex [Citation230,Citation231]. Astragalus may protect diabetic rats’ kidney by downregulation of Tie-2 (a receptor tyrosine kinase of the Tie family), a receptor for angiopoietin 1 [Citation232]. Furthermore, it was suggested that the modulation of TGF-β/Smad signaling could be a potential mechanism to prevent DN in KK-Ay mice [Citation233].
Vitamin C and E
Vitamin C plays a major role in the antioxidant defense system and in the apoptosis. The decrease in vitamin C was observed in patients with DN [Citation234]. In diabetes, it was demonstrated that vitamin C exclusion from tubular epithelial cells, through the competition of glucose and dehydroascorbate for a common transport mechanism, will deprive the cells of antioxidant ability and could lead to ROS accumulation [Citation235]. Another study showed that in diabetic rats the administration of vitamin C can protect podocyte by increasing antioxidative capacity and ameliorating the renal OS [Citation236,Citation237]. In addition, vitamin C decreased lipid peroxidation and augmented the activities of antioxidant enzymes, SOD, CAT, and GPx and reduced albuminuria, and GBM thickness in the kidneys of diabetic rats [Citation238]. Vitamin C decreased BUN, SCr, and urinary albumin excretion rate as well as increased creatinine clearance rate in DN rats. Furthermore, the expressions of collagen type IV were significantly downregulated in treatment groups. It was found that vitamin C protects renal lesions in DN by inhibiting expression of type IV collagen [Citation239]. It has been shown that vitamin C increases HO-1 protein expression in a concentration- and time-dependent manner via Nrf2 activation in RAW 264.7 cells [Citation240].
In a double-blind study, 4 weeks’ treatment with vitamin C and E in pharmacological doses lowers albuminuria in type 2 diabetic patients with micro/macroalbuminuria [Citation241]. A combination of metformin and vitamin C significantly reduced HbA1c levels in diabetic patients as compared to metformin alone [Citation242]. Another study showed that administering vitamin C with antidiabetic drugs enhanced antioxidant defenses and reduced oxidative damage [Citation243].
Studies showed that vitamin E and tocotrienol ameliorate SCr in diabetic animals [Citation244,Citation245]. In a clinical setting, oral vitamin E normalized elevated baseline creatinine clearance in type 1 diabetic patients without much effect on glycemic control in an 8-month randomized double-blind placebo-controlled trial [Citation246]. Vitamin E reduced HbA1C levels in type 2 diabetes [Citation247]. It was shown that vitamin E and C combination improved renal function and reduced albuminuria in patients with type 2 diabetes [Citation241]. In 19 diabetic patients, the treatment with vitamin E decreased microalbuminuria, and it showed nephroprotective effect that was mediated by the antioxidant action and the decrease of thromboxane A2 production [Citation248]. Tocotrienol, a member of the vitamin E family, reduced proximal tubular injury and renal LPO, increased GSH level and CAT activity and improved the index of NO2−/NO3– generation [Citation249]. However, vitamin E did not show a beneficial effect on diabetic complication including albuminuria [Citation250].
At the early stage of nitrosomethylbenzylamine-induced esophageal cancer, α-tocopherol could block the initiation of carcinogenesis through suppressing the activation of NFκB signaling pathway [Citation251]. This might be a case in DN though no study was conducted to show such effect.
Zinc
Zinc is an essential trace metal that has important physiological functions and it acts as a cofactor for many enzymes and proteins. Furthermore, it is an essential component of numerous proteins involved in the defense system against the OS. It was known that patients with DM often have zinc deficiency because of the increased urinary excretion and the restricted certain food intake [Citation252].
Meta-analyses showed that zinc supplementation has a favorable effect on glycemic control in diabetic patients [Citation253]. Furthermore, zinc supplementation in diabetic mice and rats significantly reduce diabetes-induced renal damage [Citation254]. In STZ-induced diabetic rats, zinc supplementation can attenuate diabetes-induced renal pathological changes likely through prevention of hyperglycemia-induced CTGF expression by zinc-induced cardiac metallothionein in renal tubular cells [Citation255]. It was found also that supplementation of zinc improved the effectiveness of hypoglycemic agents and may be beneficial in decreasing BSL, triglyceride, urinary albumin excretion, and inflammation in type 2 diabetic nephropathy patients [Citation256]. A recent study showed that zinc has a protective effect against diabetic damage through stimulation of metallothionein synthesis, a potent antioxidant, and regulation of the OS [Citation255].
Zinc upregulates Nrf2 function via activating Akt-mediated inhibition of Fyn function, Nrf2 nuclear exporter. Treatment of diabetic mice with TPEN, zinc chelator, decreased renal zinc level and Nrf2 expression and transcription, with an exacerbation of renal oxidative damage, inflammation and fibrosis. These results suggest that zinc is essential for Nrf2 expression and transcription function [Citation257]. Zinc has antioxidant properties that may be mediated by the upregulation of Nrf2 [Citation258]. Zinc treatment of diabetic mice for 3 months significantly upregulated the expression and function of Nrf2 and the expression of metallothionein [Citation259].
Oats
Oats are a source of several natural antioxidants [Citation260]. Oat downregulates the TGF-β1 and receptors for AGE expression at mRNA levels and it can suppress DN in rats and may slow down renal fibrosis by the disruption of the detrimental receptors for AGE-NFκB axis [Citation261]. After 12 weeks of diabetes in rats, oat treatment reduced BGL, HbA1c, and OS markers, and normalized NFκB activation and TNF-α expression. Furthermore, it reduced VEGF in the diabetic retina by 43% (P < 0.001). Oat β-d-glucan had a strong hypoglycemic effect in streptozotocin-nicotinamide-induced mice [Citation262]. Meta-analysis of four articles dealing with 350 type 2 DM patients showed that the administrated oat β-d-glucan from 2.5 to 3.5 g/day for 3–8 weeks caused significantly lowered concentrations in BSL and HbA1c [Citation263]. Avenanthramides, unique group of alkaloids in oat that contain a phenolic group, could significantly increase HO-1 expression in both a dose- and time-dependent manner which was mediated by Nrf2 translocation in HK-2 cells [Citation264]. They have antioxidant and anti-inflammatory activities.
Lycopus lucidus Turcz
Lycopus lucidus Turcz has been widely used as a traditional oriental medicine and it contains antioxidant phenol. It was shown that Lycopus can inhibit renal fibrosis by blocking TGF-β signaling pathway and it has a protective effect on renal damage of STZ-induced DN in rats [Citation265]. In primary cultured human umbilical vein endothelial cells, it was found that high glucose induces H2O2 production and ROS formation; however, pretreatment with aqueous extract of the leaves of L. lucidus Turcz inhibited the high glucose-induced ROS formation. In addition, the aqueous extract suppressed the translocation and promoter transcriptional activity of NFκB that was increased by high glucose [Citation266]. Furthermore, administration of lycopene decreased the levels of MDA content and expression of CTGF, increased protein kinase (Akt/PKB) phosphorylation and SOD activity in diabetic renal tissues in rats. It was proposed that lycopene prevents development of DN and ameliorates renal function through improving OS and regulating p-Akt and CTGF [Citation267].
Lycopus suppressed rhTGF-β1-induced Smad2 and ERK1/2 activation, downregulated the expression of TGF-βRI, TGF-βRII, Smad4 and Smad7 in SV40 MES13 cells [Citation268]. In STZ-induced DN rat’s model, Lycopus inhibited Smad2 phosphorylation, reduced mRNA level of TGF-β1, ameliorated expansion of the mesangial area and decreased SCr, BUN and reduced the SOD activity. Therefore, it was suggested that by blocking TGF-β signaling pathway, Lycopus is a novel inhibitor of renal fibrosis [Citation268].
Milk thistle
Milk thistle has been known as a remedy for a variety of disorders. Silybum marianum, the Latin term for the plant and its seeds, contains flavonolignans and silymarin is a dry mixture of these compounds. Silymarin has antioxidant and anti-inflammatory effects. It reduces urinary excretion of albumin, TNF-α, and MDA in patients with DN [Citation269]. Silybin, the active constituent of silymarin, was tested on cultures of mouse podocytes and in the OVE26 mouse, a model of type 1 DM and DN [Citation270]. The results showed a protective effect of silybin against high glucose-induced podocyte injury. Another study showed that silymarin lowers levels of BSL, HbA1c, urine volume, SCr, serum uric acid, and urine albumin, and the histopathological studies strongly supported the protective effect of silymarin [Citation271]. In diabetic rats, silibinin decreased levels of HbA1c, serum triglyceride, cholesterol, BGL, and the apoptosis ratio of pancreatic β-cells. Interestingly, silibinin reversed STZ-induced downregulation of Sirt-1 expression. Silibinin may reverse hyperglycemia and repair damaged pancreatic β-cells by promoting Sirt-1 expression [Citation272].
In a randomized, triple-blinded, placebo-controlled clinical trial, 40 type 2 diabetes patients were treated with silymarin supplementation which increased SOD, GPx activity and total antioxidant capacity, reduced CRP levels, and decreased MDA concentration [Citation273]. In another randomized, double-blind, placebo-controlled, 2-arm parallel trial, 60 patients with type 2 diabetes with macroalbuminuria despite treatment with the maximum dose of a renin–angiotensin system inhibitor were randomly assigned to two equal groups to receive three 140-mg tablets of silymarin or three tablets of placebo daily for 3 months. The results showed that the urinary albumin–creatinine ratio, urinary levels of TNF-α, and urinary and serum levels of MDA decreased significantly in the silymarin compared with the placebo group [Citation269]. It increases CAT, albumin, and hemoglobin in ESRD [Citation274]. Silibinin decreased the NADPH oxidase, iNOS and NFκB over expression by arsenic and upregulated the Nrf2 expression in the renal tissue [Citation275]. Furthermore, it caused a decrease in lipid peroxidation and an increase in the level of renal antioxidant defense system.
Conclusion
This review shows that many natural products have antioxidant and anti-inflammatory properties, and they have potential therapeutic properties in DM and DN. Data showed that the natural substances or their active ingredients modulate Keap1/Nrf2/ARE pathway and NFκB. They upregulate Keap1/Nrf2/ARE pathway and stimulate antioxidant status of living cells and tissue. And by downregulating NFκB, the natural substances decrease productions and activities of chemokines, growth factors, cytokines, and adhesion molecules, and ameliorate inflammatory response. Most of the studies, preclinical as well as clinical, included the introduction of herbs, plants or their active ingredient after the establishment of DM and DN. The results showed the obvious therapeutic outcome in preclinical studies that used whole natural substances or the active ingredients, but there is little evidence in clinical settings that taking single antioxidant has a therapeutic effect. There is a lack of randomized controlled trials using whole natural products or combined antioxidants in the management of DN. Furthermore, there was no consistency and subsequent studies following a positive result in animals’ experiments. Also the effective doses of the plants or their derivatives or extracts are still to be determined. Therefore, it is suggested that the use of combined antioxidants or whole natural plant products might demonstrate the expected beneficial results in patients with DM and DN.
A finding of new successful therapeutic intervention for the management of DN is demanded. However, prevention of DM complication such as DN is very important. The natural substances might have strong preventive properties. Such data exploring this potential property are lacking in preclinical and clinical settings. Therefore, studies on using natural substances in the prevention of DM complications and DN are important. We believe that using natural substances in prediabetic status of disease and before development of DN will explore more beneficial outcome in clinical trials.
Since biological systems always are under oxidative and inflammatory insults due to the change in lifestyle and pollution, antioxidant and anti-inflammatory pathways play a major role in keeping healthy environments for cells and organs. Therefore, using natural substances augments antioxidants and anti-inflammatory system before establishment or appearance of diseases. It is important to stress that the future work should include using natural substances as supplementary interventions besides standard therapeutic interventions, using natural substances before the appearance of disease or complication such as DN, and focusing on the development of new ingredients that work directly in Keap1/Nrf2/ARE/NFκB pathways.
Disclaimer statement
No potential conflict of interest was reported by the authors.
Notes on contributors
Noori Al-Waili MD, PhD is a consultant and research scientist. His research interest is in clinical trials, experimental biology, and alternative medicine. His current affiliation is New York Medical Care for Nephrology Queens, New York.
Hamza AL-Waili BSc is a research associate. His research interest is in experimental biology and alternative medicine. His current affiliation is New York Medical Care for Nephrology Queens, New York.
Thia Al-Waili is a researcher in Public health. His research interest is in biostatistics and experimental biology. His current affiliation is New York Medical Care for Nephrology Queens, New York.
Khelod Salom BSc, MD is an Internist and research scientist. Her research interest is in experimental biology and alternative medicine. Her current affiliation is New York Medical Care for Nephrology Queens, New York.
Additional information
Funding
References
- Thorp ML. Diabetic nephropathy: common questions. Am Fam Physician. 2005;72:96–99.
- Vasavada N, Agarwal R, Vasavada N, et al. Role of oxidative stress in diabetic nephropathy. Adv Chronic Kidney Dis. 2005;12(2):146–154.
- Shena F, Gesualdo L. Pathogenetic mechanisms of diabetic nephropathy. JASN. 2005;16:s30–s33.
- Giacco F, Brownlee M. Oxidative stress and diabetic complications. Circ Res. 2010;107:1058–1070.
- Go Y, Jones P. Redox compartmentalization in eukaryotic cells. Biochim Biophys Acta. 2008;1780:1273–1290.
- Scholz R, Graham K, Gumpricht E, et al. Mechanism of interaction of vitamin E and glutathione in the protection against membrane lipid peroxidation. Ann NY Acad Sci. 1989;570:514–517.
- Elmarakby AA, Sullivan JC. Relationship between oxidative stress and inflammatory cytokines in diabetic nephropathy. Cardiovasc Ther. 2012;30:49–59.
- Slatter D, Bolton C, Bailey A. The importance of lipid-derived malondialdehyde in diabetes mellitus. Diabetologia. 2000;43:550–557.
- Cederberg J, Basu S, Eriksson U. Increased rate of lipid peroxidation and protein carbonylation in experimental diabetic pregnancy. Diabetologia. 2001;44:766–774.
- Calvert JW, Jha S, Gundewar S, et al. Hydrogen sulfide mediates cardioprotection through Nrf2 signaling. Circ Res. 2009;105:365–374.
- Tan SM, de Haan JB. Combating oxidative stress in diabetic complications with Nrf2 activators: how much is too much? Redox Rep. 2014;19(3):107–117.
- Huang K, Chen C, Hao J, et al. Polydatin promotes Nrf2-ARE anti-oxidative pathway through activating Sirt1 to resist AGEs-induced upregulation of fibronetin and transforming growth factor-β1 in rat glomerular mesangial cells. Mol Cell Endocrinol. 2015;5(399):178–189.
- Ben-Dor A, Steiner M, Gheber L, et al. Carotenoids activate the antioxidant response element transcription system. Mol Cancer Ther. 2005;4:177–186.
- Yoh K, Hirayama A, Ishizaki K. Hyperglycemia induces oxidative and nitrosative stress and increases renal functional impairment in Nrf2-deficient mice. Genes Cells. 2008;13:1159–1170.
- de Haan JB. Nrf2 activators as attractive therapeutics for diabetic nephropathy. Diabetes. 2011;60(11):2683–2684.
- Xue M, Qian Q, Adaikalakoteswari A, et al. Activation of NF-E2-related factor-2 reverses biochemical dysfunction of endothelial cells induced by hyperglycemia linked to vascular disease. Diabetes. 2008;57:2809–2817.
- Nam J, Cho M, Lee G. The activation of NF-κB and AP-1 in peripheral blood mononuclear cells isolated from patients with diabetic nephropathy. Diabetes Res Clin Prac. 2008;81:25–32.
- Copple IM, Goldring CE, Kitteringham NR, et al. The keap1-Nrf2 cellular defense pathway: mechanisms of regulation and role in protection against drug-induced toxicity. Handb Exp Pharmacol. 2010;196:233–266.
- Itoh K, Chiba T, Takahashi S, et al. An Nrf2/small MAF heterodimer mediates the induction of phase II detoxifying enzyme genes through antioxidant response elements. Biochem Biophys Res Commun. 1997;236:313–322.
- Hagiya Y, Adachi T, Ogura S, et al. Nrf2-dependent induction of human ABC transporter ABCG2 and heme oxygenase-1 in HepG2 cells by photoactivation of porphyrins: biochemical implications for cancer cell response to photodynamic therapy. J Exp Ther Oncol. 2008;7:153–167.
- Jiang T, Huang Z, Lin Y, et al. The protective role of Nrf2 in streptozotocin-induced diabetic nephropathy. Diabetes. 2010;59:850–860.
- Baynes W, Thorpe S. The role of oxidative stress in diabetic complications. Cur Opinion Endocrinol Diabetes. 1996;3:277–284.
- Kim HJ, Vaziri ND. Contribution of impaired Nrf2-Keap1 pathway to oxidative stress and inflammation in chronic renal failure. Am J Physiol Renal Physiol. 2010;298:F662–F671.
- Peng A, Ye T, Rakheja D, et al. Green tea polyphenol, epigallocatechin-3-gallate (EGCG), ameliorates experimental immune-mediated glomerulonephritis. Kidney Int. 2011;80:601–611.
- Fan H, Ynag C, You L, et al. The histone deacetylase, sirt1, contributes to the resistance of young mice to ischemia/reperfusion-induced acute kidney injury. Kidney Int. 2013;83:404–413.
- Gu L, Tao X, Xu Y, et al. Dioscin alleviates BDL- and DMN-induced hepatic fibrosis via Sirt1/Nrf2-mediated inhibition of p38 MAPK pathway. Toxicol Appl Pharmacol. 2016;292:19–29.
- Kulkarni S, Donepudi A, Xu X, et al. Fasting induces nuclear factor E2-related factor 2 and ATP-binding cassette transporters via protein kinase A and Sirtuin-1 in mouse and human. Antioxid Redox Signal. 2014;20:15–30.
- Baur J, Ungvari Z, Minor R, et al. Are sirtuins viable targets for improving healthspan and lifespan? Nat Rev Drug Discov. 2012;11:443–461.
- Hernandez-Jimenez M, Hurtado O, Cuartero M, et al. Silent information regulator 1 protects the brain against cerebral ischemic damage. Stroke. 2013;44:2333–2337.
- Yu W, Wan Z, Qiu XF, et al. Resveratrol, an activator of SIRT1, restores erectile function in streptozotocin-induced diabetic rats. Asian J Androl. 2013;15:646–651.
- Huang KP, Chen C, Hao J, et al. AGEs-RAGE system down-regulates Sirt1 through the ubiquitin-proteasome pathway to promote FN and TGF-β1 expression in male rat glomerular mesangial cells. Endocrinology. 2015;156(1):268–279.
- Taniyama Y, Griendling K. Reactive oxygen species in the vasculature: molecular and cellular mechanisms. Hypertension. 2003;42:1075–1081.
- Ceriello A, Esposito K, Piconi L, et al. Oscillating glucose is more deleterious to endothelial function and oxidative stress than mean glucose in normal and type 2 diabetic patients. Diabetes. 2008;57(5):1349–1354.
- Cachofeiro V, Goicochea M, de Vinuesa SG, et al. Oxidative stress and inflammation, a link between chronic kidney disease and cardiovascular disease. Kidney Int Suppl. 2008;111:S4–S9.
- Shah S. Oxidants and iron in chronic kidney disease. Kidney Inter Suppl. 2004;66:S50–S55.
- Wardle E. Cellular oxidative processes in relation to renal disease. Am J Nephrol. 2005;25:13–22.
- Ghosh S, Hayden M. New regulators of NF-kappaB in inflammation. Nature Rev Immunol. 2008;8:837–848.
- Nam J, Cho M, Lee G. The activation of NF-κB and AP-1 in peripheral blood mononuclear cells isolated from patients with diabetic nephropathy. Diabetes Res Clin Pract. 2008;81:25–32.
- Zhang X, Dong F, Ren J, et al. High dietary fat induces NADPH oxidase-associated oxidative stress and inflammation in rat cerebral cortex. Exp Neurol. 2005;191:318–312.
- Morino K, Petersen K, Shulman G. Molecular mechanisms of insulin resistance in humans and their potential links with mitochondrial dysfunction. Diabetes. 2006;55:S9–S15.
- Ikeda A, Matsushita S, Sakakibara Y. Inhibition of protein kinase C ameliorates impaired angiogenesis in type I diabetic mice complicating myocardial infarction. Circulation J. 2012;76:943–949.
- Lal M, Brismar H, Eklof A, et al. Role of oxidative stress in advanced glycation end product-induced mesangial cell activation. Kidney Inter. 2002;61:2006–2014.
- Brownlee M. Biochemistry and molecular cell biology of diabetic complications. Nature. 2001;414:813–820.
- Lee H, Yu M, Yang Y, et al. Reactive oxygen species-regulated signaling pathways in diabetic nephropathy. J Am Society Nephrol. 2003;14(Suppl 3):S241–S245.
- Sanz A, Sanchez-Niño M, Ramos A. NF-κB in renal inflammation. J Am Soc Nephrol. 2010;21:1254–1262.
- Das J, Vasan V, Sil PC. Taurine exerts hypoglycemic effect in alloxan-induced diabetic rats, improves insulin-mediated glucose transport signaling pathway in heart and ameliorates cardiac oxidative stress and apoptosis. Toxicol Appl Pharmacol. 2012 Jan 15;258(2):296–308.
- Tessari P. Nitric oxide in the normal kidney and in patients with diabetic nephropathy. J Nephrol. 2015;28(3):257–268.
- Nakagawa T, Segal M, Croker B, et al. A breakthrough in diabetic nephropathy: the role of endothelial dysfunction. Nephrol Dial Transplant. 2007;22:2775–2777.
- Masuda Y, Kikuzaki H, Hisamoto M, et al. Antioxidant properties of gingerol related compounds from ginger. Biofactors. 2004;21:293–296.
- Ojewole J. Analgesic, antiinflammatory and hypoglycaemic effects of ethanol extract of Zingiber officinale (Roscoe) rhizomes (Zingiberaceae) in mice and rats. Phytother Res. 2006;20:764–772.
- Akhani SP, Vishwakarma SL, Goyal RK. Anti-diabetic activity of Zingiber officinale in streptozotocin-induced type I diabetic rats. J Pharm Pharmacol. 2004;56(1):101–105.
- Ahmad N, Sulaiman S, Mukti NA, et al. Effects of ginger extract (Zingiber officinale Roscoe) on antioxidant status of hepatocarcinoma induced rats. Malay J Biochem Mol Biol. 2006;14:7–12.
- Harliansyah H, Murad NA, Ngah ZW, et al. Antiproliferative, antioxidant and apoptosis effects of Zingiber officinale and 6-gingerol on HepG2 cells. Asian J Biochem. 2009;2(6):421–426.
- Morakinyo A, Akindele A, Ahmed Z. Modulation of antioxidant enzymes and inflammatory cytokines: possible mechanism of anti-diabetic effect of ginger extracts. Afr J Biomed Res. 2011;14:195–202.
- Sani NF, Belani LK, Sin CP, et al. Effect of the combination of gelam honey and ginger on oxidative stress and metabolic profile in streptozotocin-induced diabetic Sprague-Dawley rats. Biomed Res Int. 2014;2014:160695.
- Heimes K, Feistel B, Verspohl EJ. Impact of the 5-HT3 receptor channel system for insulin secretion and interaction of ginger extracts. Eur J Pharmacol. 2009;624:58–65.
- Shanmugam KR, Ramakrishna CH, Mallikarjuna K, et al. The impact of ginger on kidney carbohydrate metabolic profiles in STZ induced diabetic rats. Asian J Exp Sci. 2009;23:127–134.
- Al-Qattan K, Thomson M, Ali M. Garlic (Allium sativum) and ginger (Zingiber officinale) attenuate structural nephropathy progression in streptozotocin-induced diabetic rats. ESPEN. 2008;3(2):e62–e71.
- Li F, Wang Y, Parkin K, et al. Isolation of quinone reductase (QR) inducing agents from ginger rhizome and their in vitro anti-inflammatory activity. Food Res Int. 2011;44:1597–1603.
- Tzeng TF, Liou SS, Chang CJ, et al. A tropical ginger sesquiterpene, ameliorates streptozotocin-induced diabetic nephropathy in rats by reducing the hyperglycemia induced inflammatory response. Nutr Metab (Lond). 2013;10(1):64. DOI:10.1186/17437075-10-64.
- Takada Y, Murakami A, Aggarwal B. Zerumbone abolishes NF-κB and IκBα kinase activation leading to suppression of antiapoptotic and metastatic gene expression, upregulation of apoptosis, and downregulation of invasion. Oncogene. 2005;24:6957–6969.
- Ohnishi K, Irie K, Murakami A. In vitro covalent binding of zerumbone, a chemopreventive food factor. Biosci Biotechnol Biochem. 2009;73:1905–1907.
- Singh AB, Akanksha, Singh N, et al. Anti-hyperglycaemic, lipid lowering and anti-oxidant properties of [6]-gingerol in db/db mice. Inter J Med Sci. 2009;1(12):536–544.
- Chakraborty D, Mukherjee A, Sikdar S, et al. [6]-Gingerol isolated from ginger attenuates sodium arsenite induced oxidative stress and plays a corrective role in improving insulin signaling in mice. Toxicol Lett. 2012;210(1):34–43.
- Aggarwal B. Targeting inflammation-induced obesity and metabolic diseases by curcumin and other nutraceuticals. Ann Rev Nutr. 2010;30:173–199.
- Meng B, Li J, Cao H. Antioxidant and antiinflammatory activities of curcumin on diabetes mellitus and its complications. Curr Pharm Des. 2013;19(11):2101–2113.
- Reddy S, Aggarwa B. Curcumin is a non-competitive and selective inhibitor of phosphorylase kinase. FEBS Lett. 1994;341:19–22.
- Ak T, Gülçin I. Antioxidant and radical scavenging properties of curcumin. Chem Biol Int. 2008;174:27–37.
- Das K, Das C. Curcumin (diferuloylmethane), a singlet oxygen (1O2) quencher. Biochem Biophys Res Commun. 2002;295:62–66.
- Barzegar A, Moosavi-Movahedi A. Intracellular ROS protection efficiency and free radical scavenging activity of curcumin. PLoS One. 2011;6:e26012.
- Panchal H, Vranizan K, Lee C, et al. Early anti-oxidative and antiproliferative curcumin effects on neuroglioma cells suggest therapeutic targets. Neurochem Res. 2008;33:1701–1710.
- Trujillo J, Chirino YI, Molina-Jijón E, et al. Renoprotective effect of the antioxidant curcumin: recent findings. Redox Biol. 2013;1(1):448–456.
- Sun LN, Yang ZY, Lv SS, et al. Curcumin prevents diabetic nephropathy against inflammatory response via reversing caveolin-1 Tyr14 phosphorylation influenced TLR4 activation. Int Immunopharmacol. 2014;23:236–246.
- Sun LN, Chen ZX, Liu XC, et al. Curcumin ameliorates epithelial-to-mesenchymal transition of podocytes in vivo and in vitro via regulating caveolin-1. Biomed Pharmacother. 2014;68:1079–1088.
- Liu J, Feng L, Zhu M. The in vitro protective effects of curcumin and demethoxycurcumin in Curcuma longa extract on advanced glycation end products-induced mesangial cell apoptosis and oxidative stress. Planta Med. 2012;78:1757–1760.
- Lu M, Tao L, Mei W. Effect of curcumin on the expression of p-STAT3 and IκB in db/db mice. J Central South Univ (Med Sci). 2014;39(6):591–597.
- Yang H, Xu W, Zhou Z, et al. Curcumin attenuates urinary excretion of albumin in type II diabetic patients with enhancing nuclear factor erythroid-derived 2-like 2 (Nrf2) system and repressing inflammatory signaling efficacies. Exp Clin Endocrinol Diabetes. 2015;123(6):360–367.
- Wu W, Geng H, Liu Z, et al. Effect of curcumin on rats/mice with diabetic nephropathy: a systematic review and meta-analysis of randomized controlled trials. J Tradit Chin Med. 2014;34(4):419–429.
- Zhang X, Liang D, Guo L, et al. Curcumin protects renal tubular epithelial cells from high glucose-induced epithelial-to-mesenchymal transition through Nrf2-mediated upregulation of heme oxygenase-1. Mol Med Rep. 2015;12:1347–1355.
- Soetikno V, Sari FR, Lakshmanan AP, et al. Curcumin alleviates oxidative stress, inflammation, and renal fibrosis in remnant kidney through the Nrf2-keap1 pathway. Mol Nutr Food Res. 2013;57(9):1649–1659.
- Tapia E, Soto V, Ortiz-Vega K, et al. Curcumin I induces Nrf2 nuclear translocation and prevents glomerular hypertension, hyperfiltration, oxidant stress, and the decrease in antioxidant enzymes in 5/6 nephrectomized rats. Oxid Med Cell Longev. 2012;2012:269039.
- Pae O, Jeong S, Jeong O, et al. Roles of heme oxygenase-1 in curcumin-induced growth inhibition in rat smooth muscle cells. Exp Mol Med. 2007;39:267–277.
- Rosenfeldt FL, Haas SJ, Krum H. Coenzyme Q10 in the treatment of hypertension: a meta-analysis of the clinical trials. J Hum Hypertens. 2007;21(4):297–306.
- Sourris K, Harcourt B, Tang P, et al. Ubiquinone (coenzyme Q10) prevents renal mitochondrial dysfunction in an experimental model of type 2 diabetes. J Free Radic Biol Med. 2012;52:716–723.
- Wold L, Muralikrishnan D, Albano CB, et al. Insulin-like growth factor I (IGF-1) supplementation prevents diabetes-induced alterations in coenzymes Q9 and Q10. Acta Diabetol. 2003;40(2):85–90.
- Ahmadvand H, Tavafi M, Khosrowbeygi A. Amelioration of altered antioxidant enzymes activity and glomerulosclerosis by coenzyme Q10 in alloxan-induced diabetic rats. J Diabetes Complications. 2012;26:476–482.
- Persson MF, Franzén S, Catrina SB, et al. Coenzyme Q10 prevents GDP-sensitive mitochondrial uncoupling, glomerular hyperfiltration and proteinuria in kidneys from db/db mice as a model of type 2 diabetes. Diabetologia. 2012;55(5):1535–1543.
- Maheshwari R, Balaraman R, Sen A, et al. Effect of coenzyme Q10 alone and its combination with metformin on streptozotocin-nicotinamide-induced diabetic nephropathy in rats. Indian J Pharmacol. 2014;46:627–632.
- Li L, Du J, Lian Y, et al. Protective effects of Coenzyme Q10 against hydrogen peroxide-induced oxidative stress in PC12 cell: the role of Nrf2 and antioxidant enzymes. Cell Mol Neurobiol. 2016;36:103–111.
- Choi HK, Pokharel YR, Lim SC, et al. Inhibition of liver fibrosis by solubilized coenzyme Q10: role of Nrf2 activation in inhibiting transforming growth factor-beta1 expression. Toxicol Appl Pharmacol. 2009;240:377–384.
- Rafieian-Kopaie M, Baradaran A. Plants antioxidants: from laboratory to clinic. J Nephropathol. 2013;2:152–153.
- Rehman H, Krishnasamy Y, Haque K, et al. Green tea polyphenols stimulate mitochondrial biogenesis and improve renal function after chronic cyclosporin a treatment in rats. PLoS One. 2013;8:1–12.
- Ryu HH, Kim HL, Chung JH, et al. Renoprotective effects of green tea extract on renin-angiotensin-aldosterone system in chronic cyclosporine-treated rats. Nephrol Dial Transplant. 2011;26:1188–1193.
- Kang M, Park Y, Kim B, et al. Preventive effects of green tea (Camellia sinensis var. Assamica) on diabetic nephropathy. Yonsei Med J. 2012;53:138–144.
- Renno WM, Abdeen S, Alkhalaf M, et al. Effect of green tea on kidney tubules of diabetic rats. Br J Nutr. 2008;100:652–659.
- Yokozawa T, Nakagawa T, Oya T, et al. Green tea polyphenols and dietary fibre protect against kidney damage in rats with diabetic nephropathy. J Pharm Pharmacol. 2005;57(6):773–780.
- Li J, Sapper TN, Mah E, et al. Green tea extract provides extensive Nrf2-independent protection against lipid accumulation and NFκB pro-inflammatory responses during nonalcoholic steatohepatitis in mice fed a high-fat diet. Mol Nutr Food Res. 2016;60(4):858–870.
- Kim H, QUan M, Kim J. New insights into the mechanisms of polyphenols beyond antioxidant properties; lessons from the green tea polyphenol, epigallocatechin-3-gallate. Redox Biol. 2014;2:187–195.
- Varatharajan R, Sattar MZ, Chung I, et al. Antioxidant and pro-oxidant effects of oil palm (Elaeis guineensis) leaves extract in experimental diabetic nephropathy: a duration-dependent outcome. BMC Complement Altern Med. 2013;13:242–246.
- Chennasamudram SP, Kudugunti S, Boreddy PR, et al. Renoprotective effects of (+)-catechin in streptozotocin-induced diabetic rat model. Nutr Res. 2012;32(5):347–356.
- Wang Y, Wang B, Du F, et al. Epigallocatechin-3-gallate attenuates oxidative stress and inflammation in obstructive nephropathy via NF-κB and Nrf2/HO-1 signalling pathway regulation. Basic Clin Pharmacol Toxicol. 2015;117(3):164–172.
- Yoon SP, Maeng YH, Hong R, et al. Protective effects of epigallocatechin gallate (EGCG) on streptozotocin-induced diabetic nephropathy in mice. Acta Histochem. 2014;116:1210–1215.
- Ye T, Zhen J, Du Y, et al. Green tea polyphenol (–)-epigallocatechin-3-gallate restores Nrf2 activity and ameliorates crescentic glomerulonephritis. PLoS One. 2015. DOI:10.1371/journal.pone.0119543.
- Sahin K, Tuzcu M, Gencoglu H, et al. Epigallocatechin-3-gallate activates Nrf2/HO-1 signaling pathway in cisplatin-induced nephrotoxicity in rats. Life Sci. 2010;87:240–245.
- Tsai PY, Ka SM, Chang JM, et al. Epigallocatechin-3-gallate prevents lupus nephritis development in mice via enhancing the Nrf2 antioxidant pathway and inhibiting NLRP3 inflammasome activation. Free Radic Biol Med. 2011;51:744–754.
- Jiménez-Escrig A, Rincón M, Pulido R, et al. Guava fruit (Psidium guajava L.) as a new source of antioxidant dietary fiber. J Agric Food Chem. 2001;49(11):5489–5493.
- Lin CY, Yin MC. Renal protective effects of extracts from guava fruit (Psidium guajava L.) in diabetic mice. Plant Foods Hum Nutr. 2012;67(3):303–308.
- Adesida A, Farombi EO. Free radical scavenging activities of guava extract in vitro. Afr J Med Med Sci. 2012;41(Suppl):81–90.
- Kuang QT, Zhao JJ, Ye CL, et al. Nephroprotective effects of total triterpenoids from Psidium guajava leaves on type 2 diabetic rats. Zhong Yao Cai. 2012;35(1):94–97.
- Sen SS, Sukumaran V, Giri SS, et al. Flavonoid fraction of guava leaf extract attenuates lipopolysaccharide-induced inflammatory response via blocking of NF-κB signalling pathway in Labeo rohita macrophages. Fish Shellfish Immunol. 2015;47(1):85–92.
- Zhang S, Zheng L, Dong D, et al. Effects of flavonoids from Rosa laevigata Michx fruit against high-fat diet-induced non-alcoholic fatty liver disease in rats. Food Chem. 2013;141:2108–2116.
- Zhou Y, Liao Q, Luo Y, et al. Renal protective effect of Rosa laevigata Michx. by the inhibition of oxidative stress in streptozotocin-induced diabetic rats. Mol Med Rep. 2012;5(6):1548–1554.
- Zhao L, Xu L, Tao X, et al. Protective effect of the total flavonoids from Rosa laevigata Michx fruit on renal ischemia-reperfusion injury through suppression of oxidative stress and inflammation. Molecules. 2016;21(7):952. DOI:10.3390/molecules21070952.
- Anwar M, Meki A. Oxidative stress in streptozotocin-induced diabetic rats: effects of garlic oil and melatonin. Comp Biochem Physiol A. 2003;135:539–547.
- Thomson M, Al-Amin ZM, Al-Qattan KK, et al. Anti-diabetic and hypolipidaemic properties of garlic (Allium sativum) in streptozotocin-induced diabetic rats. Int J Diabetes Metab. 2006;15:108–115.
- Drobiova H, Thomson M, Al-Qattan K, et al. Garlic increases antioxidant levels in diabetic and hypertensive rats determined by a modified peroxidase method. Evid Based Complement Altern Med. 2011;2011:703049. DOI:10.1093/ecam/nep011.
- Eidi A, Eidi M, Esmaeli E. Antidiabetic effect of garlic (Allium sativum L.) in normal and streptozotocin-induced diabetic rats. Phytomedicine. 2006;13:624–629.
- Al-Qattan KK, Mansour MH, Thomson M, et al. Garlic decreases liver and kidney receptor for advanced glycation end products expression in experimental diabetes. Pathophysiology. 2016;23(2):135–145.
- Shiju TM, Rajkumar R, Rajesh NG, et al. Aqueous extract of Allium sativum L bulbs offer nephroprotection by attenuating vascular endothelial growth factor and extracellular signal-regulated kinase-1 expression in diabetic rats. Indian J Exp Biol. 2013;51(2):139–148.
- Mariee AD, Abd-Allah GM, El-Yamany MF. Renal oxidative stress and nitric oxide production in streptozotocin-induced diabetic nephropathy in rats: the possible modulatory effects of garlic (Allium sativum L.). Biotechnol Appl Biochem. 2009;52(Pt 3):227–232.
- Amagase H, Petesch BL, Matsuura H, et al. Intake of garlic and its bioactive components. J Nutr. 2001;131:955S–9562.
- Thomson M, Al-Qattan KK, Ali M. Anti-diabetic and anti-oxidant potential of aged garlic extract (AGE) in streptozotocin-induced diabetic rats. BMC Complement Altern Med. 2016 Jan 19;16:17. DOI:10.1186/s12906–016-0992-5.
- Maldonado PD, Barrera D, Medina-Campos ON, et al. Aged garlic attenuates gentamicin-induced renal damage and oxidative stress in rats. Life Sci. 2003;73:2543–2556.
- Colin-Gonzalez AL, Santana RA, Silva-Islas CA, et al. The antioxidant mechanisms underlying the aged garlic extract- and S-allylcysteine-induced protection. Oxid Med Cell Longev. 2012;2012:907162. DOI:10.1155/2012/907162.
- Dillon SA, Burmi RS, Lowe GM, et al. Antioxidant properties of aged garlic extract: an in vitro study incorporating human low density lipoprotein. Life Sci. 2003;72:1583–1594.
- Hiramatsu K, Tsuneyoshi T, Ogawa T, et al. Aged garlic extract enhances heme oxygenase-1 and glutamate-cysteine ligase modifier subunit expression via the nuclear factor erythroid 2-related factor 2-antioxidant response element signaling pathway in human endothelial cells. Nutr Res. 2016;36(2):143–149.
- Huang H, Jiang Y, Mao G, et al. Protective effects of allicin on streptozotocin-induced diabetic nephropathy in rats. J Sci Food Agric. 2016. DOI:10.1002/jsfa.7874.
- Gómez-Sierra T, Molina-Jijón E, Tapia E, et al. S-allylcysteine prevents cisplatin-induced nephrotoxicity and oxidative stress. J Pharm Pharmacol. 2014;66(9):1271–1281.
- Shi H, Jing X, Wei X, et al. S-allyl cysteine activates the Nrf2-dependent antioxidant response and protects neurons against ischemic injury in vitro and in vivo. J Neurochem. 2015;133(2):298–308.
- Tsai CY, Wang CC, Lai TY, et al. Antioxidant effects of diallyl trisulfide on high glucose-induced apoptosis are mediated by the PI3K/Akt-dependent activation of Nrf2 in cardiomyocytes. Int J Cardiol. 2013;168(2):1286–1297.
- Hadad GM, Salam RA, Soliman RM, et al. High-performance liquid chromatography quantification of principal antioxidants in black seed (Nigella sativa L.) phytopharmaceuticals. J AOAC Int. 2012;95:1043–1047.
- Kaleem M, Kirmani D, Asif M, et al. Biochemical effects of Nigella sativa L seeds in diabetic rats. Indian J Exp Biol. 2006;44(9):745–748.
- Saleem U, Ahmad B, Rehman K, et al. Nephro-protective effect of vitamin C and Nigella sativa oil on gentamicin associated nephrotoxicity in rabbits. Pak J Pharm Sci. 2012;25(4):727–730.
- Mousavi G. Study on the effect of black cumin (Nigella sativa Linn.) on experimental renal ischemia-reperfusion injury in rats. Acta Cir Bras. 2015;30(8):542–550.
- Rchid H, Chevassus H, Nmila R, et al. Nigella sativa seed extracts enhance glucose-induced insulin release from rat-isolated Langerhans islets. Fundam Clin Pharmacol. 2004;18(5):525–529.
- Balbaa M, El-Zeftawy M, Ghareeb D, et al. Nigella sativa relieves the altered insulin receptor signaling in streptozotocin-induced diabetic rats fed with a high-fat diet. Oxid Med Cell Longev. 2016;2016:2492107. DOI:10.1155/2016/2492107.
- Elsherbiny NM, El-Sherbiny M. Thymoquinone attenuates doxorubicin-induced nephrotoxicity in rats: role ofNrf2 and NOX4. Chem Biol Interact. 2014 Nov 5;223:102–108.
- Khattab MM, Nagi MN. Thymoquinone supplementation attenuates hypertension and renal damage in nitric oxide deficient hypertensive rats. Phytother Res. 2007;21(5):410–414.
- Ulu R, Dogukan A, Tuzcu M, et al. Regulation of renal organic anion and cation transporters by thymoquinone in cisplatin induced kidney injury. Food Chem Toxicol. 2012;50:1675–1679.
- El-Mahmoudy A, Matsuyama H, Borgan MA, et al. Thymoquinone suppresses expression of inducible nitric oxide synthase in rat macrophages. Int Immunopharmacol. 2002;2:1603–1611.
- Ayed-Ahmed MM, Nagi MN. Thymoquinone supplementation prevents the development of gentamicin-induced acute renal toxicity in rats. Clin Exp Pharmacol Physiol. 2007;34:399–405.
- Basarslan F, Yilmaz N, Ates S, et al. Protective effects of thymoquinone on vancomycin-induced nephrotoxicity in rats. Hum Exp Toxicol. 2012;31:726–733.
- Vaillancourt F, Silva P, Shi Q, et al. Elucidation of molecular mechanisms underlying the protective effects of thymoquinone against rheumatoid arthritis. J Cell Biochem. 2011;112:107–117.
- Sankaranarayanan C, Pari L. Thymoquinone ameliorates chemical induced oxidative stress and beta-cell damage in experimental hyperglycemic rats. Chem Biol Interact. 2011;190:148–154.
- Al-Trad B, Al-Batayneh K, El-Metwally S, et al. Nigella sativa oil and thymoquinone ameliorate albuminuria and renal extracellular matrix accumulation in the experimental diabetic rats. Eur Rev Med Pharmacol Sci. 2016;20(12):2680–2688.
- Sethi G, Ahn KS, Aggarwal BB. Targeting nuclear factor-kappa B activation pathway by thymoquinone: role in suppression of antiapoptotic gene products and enhancement of apoptosis. Mol Cancer Res. 2008;6:1059–1070.
- Bolton WK, Cattran DC, Williams ME. Randomized trial of an inhibitor of formation of advanced glycation end products in diabetic nephropathy. Am J Nephrol. 2004;24:32–40.
- Tilton RG, Chang K, Hasan KS. Prevention of diabetic vascular dysfunction by guanidines. Inhibition of nitric oxide synthase versus advanced glycation end-product formation. Diabetes. 1993;42:221–232.
- Attele AS, Wu JA, Yuan CS. Ginseng pharmacology: multiple constituents and multiple actions. Biochem Pharmacol. 1999;58:1685–1693.
- Baek SH, Piao XL, Lee UJ, et al. Reduction of cisplatin-induced nephrotoxicity by ginsenosides isolated from processed ginseng in cultured renal tubular cells. Biol Pharm Bull. 2006;29:2051–2055.
- Sen S, Chen S, Feng B, et al. Preventive effects of North American ginseng (Panax quinquefolium) on diabetic nephropathy. Phytomedicine. 2012;19(6):494–505.
- Kang K, Ham J, Kim Y, et al. Heat-processed Panax ginseng and diabetic renal damage: active components and action mechanism. J Ginseng Res. 2013;37(4):379–388.
- Halim M, Yee DJ, Sames D. Imaging induction of cytoprotective enzymes in intact human cells: coumberone, a metabolic reporter for human AKR1C enzymes reveals activation by panaxytriol, an active component of red ginseng. J Am Chem Soc. 2008;130(43):14123–14128.
- Lee LS, Stephenson KK, Fahey JW, et al. Induction of chemoprotective phase 2 enzymes by ginseng and its components. Planta Med. 2009;75(10):1129–1133.
- Jin Y, Shi Y, Zou Y, et al. Fenugreek prevents the development of STZ-induced diabetic nephropathy in a rat model of diabetes. Evid Based Complement Altern Med. 2014;2014.
- Sayed AA, Khalifa M, Abd el-Latif FF. Fenugreek attenuation of diabetic nephropathy in alloxan-diabetic rats: attenuation of diabetic nephropathy in rats. J Physiol Biochem. 2012;68(2):263–269.
- Koya D, Jirousek MR, Lin YW. Characterization of protein kinase C beta isoform activation on the gene expression of transforming growth factor-beta, extracellular matrix components, and prostanoids in the glomeruli of diabetic rats. J Clin Invest. 1997;100:115–126.
- Hegazy MG, Emam MA. Ethanolic extract of Trigonella foenum graecum attenuates cisplatin-induced nephro- and hepatotoxicities in rats. Cell Mol Biol (Noisy-le-grand). 2015;61(7):81–87.
- Abdel-Daim MM, Abd Eldaim MA, Hassan AG. Trigonella foenum-graecum ameliorates acrylamide-induced toxicity in rats: roles of oxidative stress, proinflammatory cytokines, and DNA damage. Biochem Cell Biol. 2015;93(3):192–198.
- Puri D, Prabhu KM, Dev G, et al. Mechanism of antidiabetic action of compound GII purified from fenugreek (Trigonella foenum graecum) seeds. Indian J Clin Biochem. 2011;26(4):335–346.
- Xue W, Lei J, Li X, et al. Trigonella foenum graecum seed extract protects kidney function and morphology in diabetic rats via its antioxidant activity. Nutr Res. 2011;31(7):555–562.
- Kandhare AD, Bodhankar SL, Mohan V, et al. Effect of glycosides based standardized fenugreek seed extract in bleomycin-induced pulmonary fibrosis in rats: decisive role of Bax, Nrf2, NF-κB, Muc5ac, TNF-α and IL-1β. Chem Biol Interact. 2015;237:151–165.
- Ozturk H, Ozturk H, Terzi EH, et al. Anti-inflammatory effect of rosmarinic acid and an extract of Rosmarinus officinalis in rat models of local and systemic inflammation. Basic Clin Pharmacol Toxicol. 2014. DOI:10.1111/bcpt.12335.
- Jiang WL, Xu Y, Zhang SP, et al. Effect of rosmarinic acid on experimental diabetic nephropathy. Basic Clin Pharmacol Toxicol. 2012;110(4):390–395.
- Ozturk H, Ozturk H, Terzi EH, et al. Protective effects of rosmarinic acid against renal ischaemia/reperfusion injury in rats. J Pak Med Assoc. 2014;64(3):260–265.
- Mushtaq N, Schmatz R, Ahmed M, et al. Protective effect of rosmarinic acid against oxidative stress biomarkers in liver and kidney of strepotozotocin-induced diabetic rats. J Physiol Biochem. 2015;71(4):743–751.
- Erkan N, Ayranci G, Ayranci E. Antioxidant activities of rosmary extract, black seed essential oil, carnosic acid, rosmarinic acid and sesamol. Food Chem. 2008;110:76–82.
- Peterson M, Simmonds M. Rosmarinic acid. Phytochemistry. 2003;62:121–125.
- Jeanette S, Alex K, Adviye E. Oxidative stress and the use of antioxidants in diabetes. Cardiovasc Diabetol. 2005;4:5–9.
- Tursun A, Astumi N, Hiroyuki M, et al. A natural scavenger of peroxynitrites, protects against impairment of memory induced by Ab25–35. Behav Brain Res. 2007;180:139–145.
- Tavafi M, Ahmadvand H, Tamjidipoor A. Rosmarinic acid ameliorates diabetic nephropathy in uninephrectomized diabetic rats. Iran J Basic Med Sci. 2011;14(3):275–283.
- Bakirel T, Bakirel U, Keleş OU, et al. In vivo assessment of antidiabetic and antioxidant activities of rosmary (Rosmarinus officinalis) in alloxan – diabetic rabbits. J Ethnopharmacol. 2008;116:64–73.
- Tavafi M, Ahmadvand H. Effect of rosmarinic acid on inhibition of gentamicin induced nephrotoxicity in rats. Tissue Cell. 2011;43(6):392–397.
- Kosaka K, Mimura J, Itoh K, et al. Role of Nrf2 and p62/ZIP in the neurite outgrowth by carnosic acid in PC12 h cells. J. Biochem. 2010;147:73–81.
- Mimura J, Kosaka K, Maruyama A, et al. Nrf2 regulates NGF mRNA induction by carnosic acid in T98G glioblastoma cells and normal human astrocytes. J. Biochem. 2011;150:209–217.
- de Oliveira MR, Ferreira GC, Schuck PF. Protective effect of carnosic acid against paraquat-induced redox impairment and mitochondrial dysfunction in SH-SY5Y cells: role for PI3 K/Akt/Nrf2 pathway. Toxicol In Vitro. 2016;32:41–54.
- de Oliveira MR, Ferreira GC, Schuck PF, et al. Role for the PI3K/Akt/Nrf2 signaling pathway in the protective effects of carnosic acid against methylglyoxal-induced neurotoxicity in SH-SY5Y neuroblastoma cells. Chem Biol Interact. 2015;242:396–406.
- Seymour E, Bennink M, Bolling S. Diet-relevant phytochemical intake affects the cardiac AhR and nrf2 transcriptome and reduces heart failure in hypertensive rats. J Nutr Biochem. 2013;24:1580–1586.
- Chiou Y, Tsai M, Nagabhushanam K, et al. Pterostilbene is more potent than resveratrol in preventing azoxymethane (AOM)-induced colon tumorigenesis via activation of the NF-E2-related factor 2 (Nrf2)-mediated antioxidant signaling pathway. J Agric Food Chem. 2011;59:2725–2733.
- Bagchi D, Swaroop A, Preuss HG, et al. Free radical scavenging, antioxidant and cancer chemoprevention by grape seed proanthocyanidin: an overview. Mutat Res. 2014;768:69–73.
- Bladé C, Aragonès G, Arola-Arnal A, et al. Proanthocyanidins in health and disease. Biofactors. 2016;42(1):5–12.
- Mansouri E, Panahi M, Ghaffari MA, et al. Effects of grape seed proanthocyanidin extract on oxidative stress induced by diabetes in rat kidney. Iran Biomed J. 2011;15(3):100–106.
- Li X, Xiao Y, Gao H, et al. Grape seed proanthocyanidins ameliorate diabetic nephropathy via modulation of levels of AGE, RAGE and CTGF. Nephron Exp Nephrol. 2009;111(2):e31–e41.
- Liu YN, Shen XN, Yao GY. Effects of grape seed proanthocyanidins extracts on experimental diabetic nephropathy in rats. Wei Sheng Yan Jiu. 2006;35(6):703–705.
- Bao L, Zhang Z, Dai X, et al. Effects of grape seed proanthocyanidin extract on renal injury in type 2 diabetic rats. Mol Med Rep. 2015;11(1):645–652.
- Gao Z, Liu G, Hu Z, et al. Grape seed proanthocyanidin extract protects from cisplatin-induced nephrotoxicity by inhibiting endoplasmic reticulum stress induced apoptosis. Mol Med Rep. 2014;9:801–807.
- Bao L, Cai X, Zhang Z, et al. Grape seed procyanidin B2 ameliorates mitochondrial dysfunction and inhibits apoptosis via the AMP-activated protein kinase-silent mating type information regulation 2 homologue 1-PPARγ co-activator-1α axis in rat mesangial cells under high-dose glucosamine. Br J Nutr. 2015;113(1):35–44.
- Nazima B, Manoharan V, Miltonprabu S. Grape seed proanthocyanidins ameliorates cadmium-induced renal injury and oxidative stress in experimental rats through the up-regulation of nuclear related factor 2 and antioxidant responsive elements. Biochem Cell Biol. 2015;93(3):210–226.
- Akisu M, Kultursay N, Coker I, et al. Platelet-activating factor is an important mediator in hypoxic ischemic brain injury in the newborn rat. Flunarizine and Ginkgo biloba extract reduce PAF concentration in the brain. Biol Neonate. 1998;74:439–444.
- Li X, Yan H, Wang J. Extract of Ginkgo biloba and alpha-lipoic acid attenuate advanced glycation end products accumulation and RAGE expression in diabetic nephropathy rats. Zhongguo Zhong Xi Yi Jie He Za Zhi. 2011;31(4):525–531.
- Tang D, Zhang Z, Gao Y, et al. Protective effects of serum containing Ginkgo biloba extract on glomerulosclerosis in rat mesangial cells. J Ethnopharmacol. 2009 6;124(1):26–33.
- Lu Q, Yin XX, Wang JY, et al. Effects of Ginkgo biloba on prevention of development of experimental diabetic nephropathy in rats. Acta Pharmacol Sin. 2007;28(6):818–828.
- Zhang L, Wei W, Guo X, et al. Ginkgo biloba extract for patients with early diabetic nephropathy: a systematic review. Evid Based Complement Altern Med. 2013; 2013, article id 689142, 17 p., DOI:10.1155/2013/689142.
- Galasso G, Schiekofer S, Sato K, et al. Impaired angiogenesis in glutathione peroxidase-1-deficient mice is associated with endothelial progenitor cell dysfunction. Circ Res. 2006;98:254–261.
- Zhao M, Wang XX, Wan WH. Effects of the Ginkgo biloba extract on the superoxide dismutase activity and apoptosis of endothelial progenitor cells from diabetic peripheral blood. Genet Mol Res. 2014;13(1):220–227.
- Wang J, Zhang L, Zhang Y, et al. Transcriptional upregulation central of HO-1 by EGB via the MAPKs/Nrf2 pathway in mouse C2C12 myoblasts. Toxicol In Vitro. 2015;29(2):380–338.
- Chen JS, Huang PH, Wang CH, et al. Nrf-2 mediated heme oxygenase-1 expression, an antioxidant-independent mechanism, contributes to anti-atherogenesis and vascular protective effects of Ginkgo biloba extract. Atherosclerosis. 2011;214(2):301–309.
- Prasad K. Flaxseed: a source of hypocholesterolemic and antiatherogenic agents. Drug News Perspect. 2000;13:99–102.
- Akpolat M, Kanter M, Topcu Y, et al. Protective effect of flaxseed oil on renal injury in hyperlipidemic rats: the effect of flaxseed oil on hyperlipidemia. Phytother Res. 2011;25:796–802.
- Rizwan S, Naqshbandi A, Farooqui Z, et al. Protective effect of dietary flaxseed oil on arsenic-induced nephrotoxicity and oxidative damage in rat kidney. Food Chem Toxicol. 2014;68:99–107.
- Velasquez MT, SJ, T, et al. Dietary flaxseed meal reduces proteinuria and ameliorates nephropathy in an animal model of type II diabetes mellitus. Kidney Int. 2003;64(6):2100–2107.
- Haliga R, Mocanu V, Păduraru I, et al. Effects of dietary flaxseed supplementation on renal oxidative stress in experimental diabetes. Rev Med Chir Soc Med Nat Iasi. 2009;113(4):1200–1204.
- Makni M, Sefi M, Fetoui H, et al. Flax and pumpkin seeds mixture ameliorates diabetic nephropathy in rats. Food Chem Toxicol. 2010;48(8–9):2407–2412.
- Bays H. Clinical overview of omacor: a concentrated formulation of omega-3 polyunsaturated fatty acids. Am J Cardiol. 2006;98:71i–76i.
- Hong S, Lu Y. Omega-3 fatty acid-derived resolvins and protectins in inflammation resolution and leukocyte functions: targeting novel lipid mediator pathways in mitigation of acute kidney injury. Front Immunol. 2013;4:13–18.
- Parinyasiri U, Ong-Ajyooth L, Parichatikanond P, et al. Effect of fish oil on oxidative stress, lipid profile and renal function in IgA nephropathy. J Med Assoc Thai. 2004;87:143–149.
- Chin H, Fu J, Ahn Y, et al. Omacor, N-3 polyunsaturated fatty acid, attenuated albuminuria and renal dysfunction with decrease of SREBP-1 expression and triglyceride amount in the kidney of type II diabetic animals. Nephrol. Dial. Transplant. 2010;25:1450–1457.
- Garman J, Mulroney S, Manigrasso M, et al. Omega-3 fatty acid rich diet prevents diabetic renal disease. Am J Physiol Renal Physiol. 2009;296:F306–F316.
- Zhang M, Hagiwara S, Matsumoto M, et al. Effects of eicosapentaenoic acid on the early stage of type 2 diabetic nephropathy in KKA(Y)/Ta mice: involvement of anti-Inflammation and antioxidative stress. Metabolism. 2006;55:1590–1598.
- Katakura M, Hashimoto M, Inoue T, et al. Omega-3 fatty acids protect renal functions by increasing docosahexaenoic acid-derived metabolite levels in SHR.Cg-Lepr(cp)/NDmcr rats, a metabolic syndrome model. Molecules. 2014;19(3):3247–3363.
- Coppo R, Amore A, Peruzzi L, et al. Angiotensin antagonists and fish oil for treating IgA nephropathy. Contrib Nephrol. 2007;157:27–36.
- An W, Kim H, Cho K, et al. Omega-3 fatty acid supplementation attenuates oxidative stress, inflammation, and tubulointerstitial fibrosis in the remnant kidney. Am J Physiol. 2009;297:F895–F903.
- Ooi L, Li Y, Kam S, et al. Antimicrobial activities of cinnamon oil and cinnamaldehyde from the Chinese medicinal herb Cinnamomum cassia Blume. Am J Chin Med. 2006;34:511–522.
- Lin C, Wu S, Chang C. Antioxidant activity of Cinnamomum cassia. Phytother Res. 2003;17:726–730.
- Khan A, Safdar M, Khan M, et al. Cinnamon improves glucose and lipids of people with type 2 diabetes. Diabetes Care. 2003;26:3215–3218.
- Chericoni S, Prieto JM, Iacopini P, et al. In vitro activity of the essential oil of Cinnamomum zeylanicum and eugenol in peroxynitrite-induced oxidative processes. J Agric Food Chem. 2005;53(12):4762–4765.
- Cheng D, Kuhn P, Poulev A, et al. In vivo and in vitro antidiabetic effects of aqueous cinnamon extract and cinnamon polyphenol-enhanced food matrix. Food Chem. 2012;135:2994–3002.
- Anderson R, Broadhurst C, Polansky M, et al. Isolation and characterization of polyphenol type-A polymers from cinnamon with insulin-like biological activity. J Agric Food Chem. 2004;52:65–70.
- Mishra A, Bhatti R, Singh A, et al. Ameliorative effect of the cinnamon oil from Cinnamomum zeylanicum upon early stage diabetic nephropathy. Planta Med. 2010;76(5):412–417.
- Muthenna P, Raghu G, Kumar PA, et al. Effect of cinnamon and its procyanidin-B2 enriched fraction on diabetic nephropathy in rats. Chem Biol Interact. 2014;222C:68–76.
- Wondrak GT, Villeneuve NF, Lamore SD, et al. The cinnamon-derived dietary factor cinnamic aldehyde activates the Nrf2-dependent antioxidant response in human epithelial colon cells. Molecules. 2010;15(5):3338–3355.
- Liao B, Hsieh C, Liu Y, et al. Cinnamaldehyde inhibits the tumor necrosis factor-α-induced expression of cell adhesion molecules in endothelial cells by suppressing NF-κB activation: effects upon IκB and Nrf2. Toxicol Appl Pharmacol. 2008;229:161–171.
- Lai PK, Chan JY, Cheng L, et al. Isolation of anti-inflammatory fractions and compounds from the root of Astragalus membranaceus. Phytother Res. 2013;27(4):581–587.
- Li J, Li F, Xu Y, et al. Chemical composition and synergistic antioxidant activities of essential oils from Atractylodes macrocephala and Astragalus membranaceus. Nat Prod Commun. 2013;8(9):1321–1324.
- Li M, Wang W, Xue J, et al. Meta-analysis of the clinical value of Astragalus membranaceus in diabetic nephropathy. J Ethnopharmacol. 2011;133:412–419.
- Zhang J, Xie X, Li C, et al. Systematic review of the renal protective effect of Astragalus membranaceus (root) on diabetic nephropathy in animal models. J Ethnopharmacol. 2009;126:189–196.
- Mou S, Ni Z, Zhang Q. Expression of c-met in human kidney fibroblasts induced by high glucose in vitro and the regulation of Radix astragali. J Chinese Integrat Med. 2008;6:482–487.
- Tang D, He B, Zheng ZG, et al. Inhibitory effects of two major isoflavonoids in Radix astragali on high glucose-induced mesangial cells proliferation and AGEs-induced endothelial cells apoptosis. Planta Med. 2011;77:729–732.
- Wang Q, Shao X, Xu W. Astragalosides IV inhibits high glucose-induced cell apoptosis through HGF activation in cultured human tubular epithelial cells. Renal Failure. 2014;36:400–406.
- Zhang YW, Wu CY, Cheng JT. Merit of Astragalus polysaccharide in the improvement of early diabetic nephropathy with an effect on mRNA expressions of NF-κB and IκB in renal cortex of streptozotoxin-induced diabetic rats. J Ethnopharmacol. 2007;114:387–392.
- Gui D, Huang J, Guo Y, et al. Astragaloside IV ameliorates renal injury in streptozotocin-induced diabetic rats through inhibiting NF-κB mediated inflammatory genes expression. Cytokine. 2013;61:970–977.
- Gu H, Ni Z, Gu L. Effects of Astragalus on expression of renal angiopoietin receptor Tie-2 in diabetic rats. J Chinese Integrat Med. 2007;5(5):536–540.
- Nie Y, Li S, Yi Y. Effects of Astragalus injection on the TGFβ/Smad pathway in the kidney in type 2 diabetic mice. BMC Complement Altern Med. 2014;14. DOI:10.1186/1472-6882-14-148.
- Varma V, Varma M, Sarkar P, et al. Correlation of vitamin C with HBA1C and oxidative stress in diabetes mellitus with or without nephropathy. Nat J Med Res. 2014;4:151–155.
- Chen L, Jia RH, Qiu CJ, et al. Hyperglycemia inhibits the uptake of dehydroascorbate in tubular epithelial cell. Am J Nephrol. 2005;25:459–465.
- Lee EY, Lee MY, Hong SW, et al. Blockade of oxidative stress by vitamin C ameliorates albuminuria and renal sclerosis in experimental diabetic rats. Yonsei Med J. 2007;48:847–855.
- Qin QJ, Deng HC, Zhao TF, et al. Study on the effect and mechanism of ascorbic acid on renal podocytes in diabetes. Zhongguo Ying Yong Sheng Li Xue Za Zhi. 2008;24(1):112–115.
- Kedziora-Kornatowska K, Szram S, Kornatowski T, et al. Effect of vitamin E and vitamin C supplementation on antioxidative state and renal glomerular basement membrane thickness in diabetic kidney. Nephron Exp Nephrol. 2003;95(4):e134–e143.
- Li Q, Ao X, Du Y, et al. Effects of aminoguanidine and vitamin C on collagen type IV in diabetic nephropathy rats. Endocrine. 2011;39(3):251–258.
- Kim SR, Ha YM, Kim YM, et al. Ascorbic acid reduces HMGB1 secretion in lipopolysaccharide-activated RAW 264.7 cells and improves survival rate in septic mice by activation of Nrf2/HO-1 signals. Biochem Pharmacol. 2015;95(4):279–289.
- Gaede P, Poulsen HE, Parving HH, et al. Double-blind, randomized study of the effect of combined treatment with vitamin C and E on albuminuria in type 2 diabetic patients. Diabetes Med. 2001;18(9):756–760.
- Dakhale GN, Chaudhari HV, Shrivastava M. Supplementation of vitamin C reduces blood glucose and improves glycosylated hemoglobin in type 2 diabetes mellitus: a randomized, double-blind study. Adv Pharmacol Sci. 2011;2011:195271.
- Das SK, Vijayakumar PA, Senthil R, et al. Antioxidant effect of vitamin C on type 2 diabetes mellitus patients along with two different oral hypoglycemic agents for smooth glycemic control. WJPPS. 2012;1:1113–1122.
- Kuhad A, Chopra K. Attenuation of diabetic nephropathy by tocotrienol: involvement of NF KB signaling pathway. Life Sci. 2009;84(9–10):296–301.
- Haidara MA, Mikhailidis DP, Rateb MA, et al. Evaluation of the effect of oxidative stress and vitamin E supplementation on renal function in rats with streptozotocin-induced Type 1 diabetes. J Diabetes Complicat. 2009;23:130–136.
- Bursell S, Clermont A, Aiello L, et al. High-dose vitamin E supplementation normalizes retinal blood flow and creatinine clearance in patients with type 1 diabetes. Diabetes Care. 1999;22(8):1245–1251.
- Akbar S, Bellary S, Griffits H. Dietary antioxidant interventions in type 2 diabetes patients: a meta-analysis. Br J Diabetes Vasc Dis. 2011;11:62–68.
- Hirnerova E, Krahulec B, Strbova L, et al. Effect of vitamin E supplementation on microalbuminuria, lipid peroxidation and blood prostaglandins in diabetic patients. Bratisl Lek Listy. 2004;105:408–413.
- Khan MR, Siddiqui S, Parveen K, et al. Nephroprotective action of tocotrienol-rich fraction (TRF) from palm oil against potassium dichromate (K2Cr2O7)induced acute renal injury in rats. Chem Biol Interact. 2011;186:228–238.
- Hirnerová E, Krahulec B, Strbová L, et al. Effect of vitamin E therapy on progression of diabetic nephropathy. Vnitr Lek. 2003;49(7):529–534.
- Yang H, Sun N, Li Y, et al. Regulation of α-tocopherol on NFκB and Nrf2 signaling pathway at early stage of N-nitrosomethylbenzylamine-induced human esophageal cell carcinogenesis. Zhonghua Yu Fang Yi Xue Za Zhi. 2015;49:546–553.
- Brandao-Neto J, Silva C, Figueiredo N. Zinc kinetics in insulin-dependent diabetes mellitus patients. Biometals. 2000;13:141–145.
- Capdor J, Foster M, Petocz P. Zinc and glycemic control: a meta-analysis of randomized placebo controlled supplementation trials in humans. J Trace Elem Med Biol. 2013;27:137–142.
- Tang Y, Yang Q, Lu J, et al. Zinc supplementation partially prevents renal pathological changes in diabetic rats. J Nutr Biochem. 2010;21(3):237–246.
- Özcelik D, Nazıroglu M, Tunçdemir M, et al. Zinc supplementation attenuates metallothionein and oxidative stress changes in kidney of streptozotocin-induced diabetic rats. Biol Trace Elem Res. 2012;150(1–3):342–349.
- Khan M, Siddique K, Ashfaq F, et al. Effect of high-dose zinc supplementation with oral hypoglycemic agents on glycemic control and inflammation in type-2 diabetic nephropathy patients. J Nat Sci Biol Med. 2013;4:336–340.
- Li B, Cui W, Tan Y, et al. Zinc is essential for the transcription function of Nrf2 in human renal tubule cells in vitro and mouse kidney in vivo under the diabetic condition. J Cell Mol Med. 2014;18(5):895–906.
- Ha KN, Chen Y, Cai J. Increased glutathione synthesis through an ARE-Nrf2-dependent pathway by zinc in the RPE: implication for protection against oxidative stress. Invest Ophthalmol Vis Sci. 2006;47:2709–2715.
- Miao X, Wang Y, Sun J, et al. Zinc protects against diabetes-induced pathogenic changes in the aorta: roles of metallothionein and nuclear factor (erythroid-derived 2)-like 2. Cardiovasc Diabetol. 2013;12:1–13.
- Emmons CL, Peterson DM, Paul GL. Antioxidant capacity of oat (Avena sativa L.) extracts. 2. In vitro antioxidant activity and contents of phenolic and tocol antioxidants. J Agric Food Chem. 1999;47(12):4894–4898.
- Al-Malki A. Oat protects against diabetic nephropathy in rats via attenuating advanced glycation end products and nuclear factor kappa B. Evid Based Complement Altern Med. 2013;2013:609745.
- Liu M, Zhang Y, Zhang H, et al. The anti-diabetic activity of oat β-d-glucan in streptozotocin-nicotinamide induced diabetic mice. Int J Biol Macromol. 2016;91:1170–1176.
- Shen XL, Zhao T, Zhou Y, et al. Effect of oat β-glucan intake on glycaemic control and insulin sensitivity of diabetic patients: a meta-analysis of randomized controlled trials. Nutrients. 2016;8(1). DOI:10.3390/nu8010039.
- Fu J, Zhu Y, Yerke A, et al. Oat avenanthramides induce heme oxygenase-1 expression via Nrf2-mediated signaling in HK-2 cells. Mol Nutr Food Res. 2015;59(12):2471–2479.
- Yao Y, Yang J, Wang D, et al. The aqueous extract of Lycopus lucidus Turcz ameliorates streptozotocin-induced diabetic renal damage via inhibiting TGF-β1 signaling pathway. Phytomedicine. 2013;20:1160–1167.
- Lee YJ, Kang DG, Kim JS, et al. Lycopus lucidus inhibits high glucose-induced vascular inflammation in human umbilical vein endothelial cells. Vascul Pharmacol. 2008;48(1):38–46.
- Li W, Wang G, Lu X, et al. Lycopene ameliorates renal function in rats with streptozotocin-induced diabetes. Int J Clin Exp Pathol. 2014;7(8):5008–5015.
- Yao Y, Yang J, Wang D, et al. The aqueous extract of Lycopus lucidus Turcz ameliorates streptozotocin-induced diabetic renal damage via inhibiting TGF-β1 signaling pathway. Phytomedicine. 2013;20(13):1160–1167.
- Fallahzadeh MK, Dormanesh B, Sagheb MM, et al. Effect of addition of silymarin to renin-angiotensin system inhibitors on proteinuria in type 2 diabetic patients with overt nephropathy: a randomized, double-blind, placebo-controlled trial. Am J Kidney Dis. 2012;60(6):896–903.
- Khazim K, Gorin Y, Cavaglieri RC, et al. The antioxidant silybin prevents high glucose-induced oxidative stress and podocyte injury in vitro and in vivo. Am J Physiol Renal Physiol. 2013;305(5):F691–700.
- Sheela N, Jose MA, Sathyamurthy D, et al. Effect of silymarin o streptozotocin-induced diabetic nephropathy. Iran J Kidney Dis. 2013;7(2):117–123.
- Wang Q, Liu M, Liu WW, et al. In vivo recovery effect of silibinin treatment on streptozotocin-induced diabetic mice is associated with the modulations of Sirt-1 expression and autophagy in pancreatic β-cell. J Asian Nat Prod Res. 2012;14(5): 41(5):413–423.
- Ebrahimpour S, Gargari BP, Mobasseri M, et al. Effects of Silybum marianum (L.) Gaertn. (silymarin) extract supplementation on antioxidant status and hs-CRP in patients with type 2 diabetes mellitus: a randomized, triple-blind, placebo-controlled clinical trial. Phytomedicine. 2015;22(2):290–296.
- Omidreza O, Khajehrezaei S, Ezzatzadegan S, et al. Effects of silymarin on biochemical and oxidative stress markers in end-stage renal disease patients undergoing peritoneal dialysis. Hemodial Inter. 2016;20:558–563.
- Prabu SM, Muthumani M. Silibinin ameliorates arsenic induced nephrotoxicity by abrogation of oxidative stress, inflammation and apoptosis in rats. Mol Biol Rep. 2012;39(12):11201–11216.