ABSTRACT
Objectives: Newly discovered glutathione transferase omega 1 (GSTO1-1) plays an important role in the glutathionylation cycle, a significant mechanism of protein function regulation. GSTO1-1 expression pattern has not been studied in transitional cell carcinoma (TCC), as yet.
Methods: A total of 56 TCC tumor and corresponding non-tumor specimens were investigated. Glutathione content and thioltransferase activity were measured spectrophotometrically. Protein-glutathione mixed disulfides were measured fluorimetrically. GSTO1-1 expression was determined by immunoblot and qPCR. Immunoprecipitation with GSTO1-1 antibody was followed by immunoblot using anti-GSTO1, GSTP1, c-Jun, JNK, Akt, phospho-Akt, and ASK1 antibody, while for the total S-glutathionylation levels non-reducing electrophoresis was performed.
Results: The contents of reduced glutathione and thioltransferase activity were significantly increased in tumor compared to non-tumor tissue. The increased GSTO1 expression in tumor tissue showed clear correlation with grade and stage. However, decreased total protein glutathionylation level in tumor compared to non-tumor samples was found. Immunoprecipitation has shown an association of GSTO1-1 with GSTP1, Akt, phospho-Akt, and ASK1 proteins.
Conclusions: GSTO1 deglutathionylase activity suggests its potential important role in redox perturbations present in TCC. Increased GSTO1-1 expression might contribute to TCC development and/or progression supporting the notion that GSTO1-1 may be a promising novel cancer target.
1. Introduction
Glutathione transferases (GSTs) are a superfamily of enzymes catalyzing the conjugation of glutathione (GSH) to a wide range of chemical carcinogens, drugs, and products of oxidative stress. Seven cytosolic GST classes have been characterized in humans: alpha, mu, pi, sigma, theta, zeta, and the most recently identified omega class (GSTO) [Citation1]. It is generally accepted that in different tumors, such as bladder, ovarian, non-small cell lung, breast, colon, pancreas, and lymphomas, overexpression of glutathione transferases P1 (GSTP1) isoenzyme represents important characteristic of transformed phenotype [Citation2,Citation3].
These alterations in GSTP1 expression pattern of tumors are related both to its anti-apoptotic role in MAPKs regulation and to newly discovered S-glutathionylation activity [Citation3]. Thus, this post-translational modification of proteins, in which glutathione is reversibly added to proteins (glutathionylation) and removed (deglutathionylation), functions as molecular switch which tunes the activity of many proteins involved in tumor growth [Citation3]. Interestingly, GSTP1 activity and its binding to target proteins are also influenced by the auto-glutathionylation of GSTP1 itself [Citation3]. In that way, glutathionylation of GSTP1 might interfere with its interaction with MAPK-JNK, representing the possible molecular mechanism of redox-sensitive regulation of signaling molecules.
Redox homeostasis with glutathione as its critical determinant is frequently dysregulated in cancers, further implying the important role of glutathionylation in regulation of activity of different signaling molecules involved in cell death and survival [Citation4]. On the basis of increased levels of glutathione, together with upregulated activities of antioxidant enzymes in transitional cell carcinoma (TCC), it can be suggested that during tumor growth important changes in redox balance also occur in this tumor [Citation5].
Omega class GSTs have a range of catalytic activities that are not associated with other GSTs but are typical of the glutaredoxins, enzymes with structural similarity to the N-terminal domain of cytosolic GSTs. Human GSTO1-1 and GSTO2-2 catalyze thioltransferase and dehydroascorbate reductase reactions, and they are involved in the arsenic biotransformation [Citation1]. Recent evidence clearly imply that GSTO1 isoenzyme, which shares only 20% amino acid sequence identity with other GSTs classes, shows significant deglutathionylase activity [Citation6]. Moreover, overexpression of GSTO1-1 is associated with the onset of drug resistance in HeLa cells, as well as in protection against cisplatin-induced apoptosis. Resistance of these tumors to therapy protocols containing cisplatin [Citation7] is a frequent problem in clinical practice. This is probably due to activation of survival pathways (Akt and ERK1/2) and inhibition of apoptotic pathways (JNK1) [Citation8]. However, in TCC of urinary bladder, the data on the activity and expression of this newly discovered and seemingly GST important class are still lacking.
Owing to the fact that glutathionylation and GSTO1 deglutathionylase activity might be important regulators of cell death and survival in TCC, the aim of this study was to determine the total level of glutathionylation, GSTO1 expression in tumor and non-tumor tissue, as well as possible association of GSTO1-1 with some of the signaling molecules involved in cell death and survival pathways, such as c-Jun, c-Jun NH2-terminal kinase (JNK), protein kinase B (Akt), apoptosis signal-regulating kinase 1 (ASK1), and GSTP1.
2. Methods
2.1. Patients, tissue specimens, and sample preparation
We enrolled 56 newly diagnosed patients with TCC of urinary bladder from the Clinic of Urology, Clinical Centre of Serbia, Belgrade. Specimens were obtained from 10 patients after radical cystectomy, and other 46 were obtained after transurethral resection (TUR). TCC samples were staged according to the International Union against Cancer and graded 1–3, according to the World Health Organization criteria [Citation9]. Bladder tumors were considered to be non-muscle-invasive (T1) or muscle-invasive (T2, T3, and T4) based on cystoscopy, TUR, imaging studies, and histopathological findings [Citation10,Citation11].
None of the patients had a history of chemotherapy. All patients provided the written informed consent. Study protocol was approved by the Ethical Committee of the Medical faculty, University of Belgrade, and the research was carried out in compliance with the Declaration of Helsinki.
For the biochemical assays, the samples were washed in cold saline, frozen in liquid nitrogen, and stored at −80°C until use. After thawing, the cytosolic fraction was prepared for the determination of enzyme activity and expression, as described elsewhere [Citation12]. For the determination of glutathione content , the frozen tissues were homogenized in 5′-sulfosalicylic acid (10%, w/v), centrifuged for 20 min at 3000g (4°C), and the supernatant fraction was used. The acid-insoluble pellet was used for the estimation of protein-glutathione mixed disulfides (PrSSG).
2.2. Assays for GSH and GSSG determination
The intracellular oxidized (GSSG) and total glutathione (GSH) were determined spectrophotometrically using the GSH recycling method [Citation13]. Reduced glutathione levels were calculated by subtracting the amount of GSSG from total GSH content and expressed as nmol/mg of protein.
2.3 Assay for protein mixed disulfides
The PrSSG were determined by the non-enzymatic method with ο-phthaldialdehyde. Namely, the reduction of PrSSG by dithiothreitol (DTT) released the bound GSH, which formed a fluorescent complex with ο-phthaldialdehyde that was further measured fluorimetrically. The content of PrSSG was expressed as nmol/mg of protein [Citation13].
2.4. Assay for thioltransferase activity
Thioltransferase activity was measured spectrophotometrically with hydroxyethyl disulfide as a substrate [Citation14]. Specific activities were calculated using an extinction coefficient of 6.22/mM cm and expressed as mU/mg of protein.
2.5 Determination of the total S-glutathionylation and immunoblot analysis
For the determination of total S-glutathionylation levels, tissues were homogenized in radioimmunoprecipitation assay buffer supplemented with protease cocktail inhibitor and N-ethylmaleimide. Equal amounts of proteins (40 μg) from the clarified homogenate were separated on a 10% sodium dodecyl sulfate (SDS)-polyacrylamide gel under non-reducing conditions.
After transfer of proteins to a nitrocellulose membrane, immunodetection was conducted using primary mouse monoclonal anti-glutathione (Abcam, Cambridge, U.K.) or mouse monoclonal anti-GSTO1 (Abcam), followed by incubation with goat anti-mouse antibody (Dako, Glostrup, Denmark). The visualization was performed using chemiluminescent detection system (kit ECL Western blotting analysis system, GE Healthcare/Amersham). All membranes were probed with anti-actin antibody (St. Louis, MO, U.S.A.) to ensure equal loading. Immunoblots were scanned, and densitometric analysis was performed using ImageQuant 5.2 (GE Healthcare, Buckinghamshire, U.K.).
2.6. Immunoprecipitation
For immunoprecipitation, TCC tissue lysates were diluted to 1 μg/μl total cell protein and 100 μg/100 μl of total bladder lysates were incubated with the mouse monoclonal anti-GSTO1 antibody (Abcam) by using the ‘Catch and Release v2.0 reversible immunoprecipitation system’ (Millipore, Darmstadt, Germany). Immunoprecipitated proteins of bladder lysates were separated on a 10% SDS-polyacrylamide gel and transferred to a nitrocellulose membrane. Blots were hybridized with appropriate primary antibodies (anti-GSTP1, c-Jun, JNK, Akt, phospho-Akt (Ser473/Thr308), and ASK1), visualized by chemiluminescent detection system (kit ECL Western blotting analysis system, GE Healthcare/Amersham), and exposed to an X-ray film (GE Healthcare/Amersham Hyperfilm ECL).
2.7. RNA extraction and real-time polymerase chain reaction (RT-PCR)
Total RNA was extracted from bladder tissue using TRIZOL® reagent (Invitrogen, Carlsbad, CA, U.S.A.) and reversely transcribed using reverse transcriptase from Moloney Murine Leukemia Virus (RevertAidTM H Minus M-MuLV RT, Fermentas, Vilnius, Lithuania) with random hexamers (Fermentas). The prepared cDNAs were amplified using TaqMan Universal PCR Master Mix (Perkin–Elmer/Applied Biosystems, Foster City, CA, U.S.A.) in an ABI PRISM 7500 Sequence Detection System (Applied Biosystems). Data were collected and quantitatively analyzed using the SDS 2.1 software (Applied Biosystems). Human 18S gene was used as an endogenous control for sample normalization. Results were presented relative to the expression of 18S. Accumulation of PCR products was detected in real time by monitoring the probe cleavage-induced mobilization of the reporter dye.
2.8. Statistical analysis
Statistical analysis was performed using the Statistical Package for the Social Sciences (SPSS, version 17.0; SPSS, Chicago, IL, U.S.A.). A P value < 0.05 was considered statistically significant. The Kolmogorov–Smirnov test was used to evaluate the normality of the distributions of variables. Parametric continuous variables were presented as mean ± standard deviations. Differences in the continuous variables between two groups were tested by the t-test for variables with normal distribution. Differences in the continuous variables between tree groups were tested the by Kruskal–Wallis test because test of homogeneity of variances (Levene’s test) had a P value of <0.05 and one-way ANOVA could not be used for variables with normal distribution. The Spearman correlation coefficient was computed to examine the association between variables.
3. Results
Tumor samples and surrounding normal uroepithelium were obtained from 56 patients with TCC of urinary bladder. Staging showed superficial growth pattern (T1) in 11 patients, while all other tumors showed invasive characteristics (T2, n = 16; T3, n = 16, and T4, n = 13). Grading was as follows: G1, n = 13; G2, n = 25, and G3, n = 18.
3.1. Increased cellular glutathione status
The content of reduced form of glutathione was significantly higher in tumor tissue compared to surrounding normal uroepithelium (), confirming profound alterations of glutathione metabolism in tumor tissue.
Table 1. Glutathione (GSH, GSSG), protein-glutathione mixed disulfides (PrSSG) content, and thioltransferase activity in patients with TCC.
3.2. Increased thioltransferase activity and GSTO1 expression
The mean thioltransferase activity in tumor tissue was increased 2.5-fold when compared with normal uroepithelium (). We also tested whether upregulation of thioltransferase activity depends on the malignant potential of the tumors. The enzyme activity in patients divided into the groups according to tumor grade was significantly increasing across grade, with the special emphasis on grade 3 (P = 0.041) ((a)). Furthermore, we stratified the tumor samples according to stage into muscle non-invasive (T1) and muscle invasive (T2, T3, and T4) ((b)). A significantly increased activity was observed in muscle-invasive in comparison with non-invasive tumors, and the activity in normal uroepithelium was lower than the one in non-invasive and invasive tumors (4.83 ± 2.48; 8.65 ± 5.09; 13.37 ± 5.78 mU/mg of protein, respectively; P < 0.001) ((b)). We analyzed the differences in thioltransferase activity among all four stages of disease (T1, T2, T3, and T4) as well and found that the thioltransferase activity was significantly increasing across stages (8.65 ± 5.09; 10.82 ± 3.95; 18.34 ± 6.34; 19.18 ± 7.02 mU/mg of protein, respectively; P = 0.014). GSTO1 expression profile was also examined by immunoblot and RT-PCR in tumor and the corresponding non-tumor uroepithelial tissues. The presence of GSTO1 was confirmed in all tumor and non-tumor specimens at a protein level. Densitometry analysis of the immunoblot data showed that the expression of GSTO1 in tumor tissue was increased approximately 2.3-fold when compared with normal uroepithelial specimens (P = 0.041) ((a)). Furthermore, we tested the correlation statistics between GSTO1 expression and TCC tumor stage (T1–T4) and grade (G1–G3) and have shown statistically significant moderate correlation between GSTO1 expression with stage and grade (P = 0.038, P = 0.040; respectively) (). Moreover, GSTO1 expression at mRNA level was increasing with the tumor grade (expressed as dCt (delta threshold cycle) in G1: 18.19 ± 3.02; dCt in G2: 17.83 ± 1.38; dCt in G3: 15.43 ± 4.47), although it did not reach statistical significance (P = 0.484) ((b)).
Figure 1. (a) Thioltransferase activity in TCC of urinary bladder according to grade. The activity is measured in tumor tissue of TCC patients and expressed as mU/mg of protein. Samples were stratified according to the tumor grade (G1, n = 13; G2, n = 25; G3, n = 18) and activity presented as mean ± standard deviation (SD). The differences in the variables between groups were assessed by the Kruskal–Wallis test. P values < 0.05 were considered statistically significant. The measurements were conducted in triplicate; n, number of tissue samples, G, tumor grade. (B). Thioltransferase activity in TCC of urinary bladder according to stage. The activity is expressed as mU/mg of protein. Tumor samples were stratified according to the tumor stage: stage 1 (non-invasive, n = 11), stages 2, 3 and 4 (invasive, n = 45) and activity presented as mean ± SD. Differences in the variables between groups were assessed by the Kruskal–Wallis test. A P value < 0.05 was considered statistically significant. The measurements were conducted in triplicate; n, number of samples
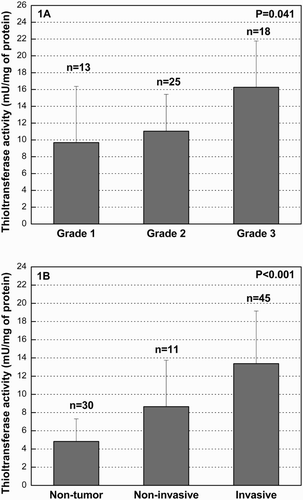
Figure 2. (a) Western blot of GSTO1 expression in TCC tumor and non-tumor tissue. Total amount of protein per sample (40 μg) was separated by SDS/PAGE and analyzed with monoclonal anti-GSTO1 antibody. Equal loading of total protein amount was ensured by β-actin. Proteins were densitometrically quantified and expressed as the mean percentage increase or decrease, compared to β-actin ± SD (graph). Differences in the variables between groups were assessed by the t-test. A P value of <0.05 was considered statistically significant. For western blots reported in the figure, the representative samples are shown. (B) RT-PCR analysis of GSTO1 expression in TCC according to the tumor grade. Total RNA was extracted from TCC tissue specimens and subjected to RT-PCR. Data were normalized by the 18S ribosomal RNA expression levels in each sample and presented as mean dCt ± SD of three independent RT-PCR analyses. Ct levels are inversely proportional to the amount of target GSTO1 in the sample (i.e. the lower the Ct level the greater the expression of target GSTO1 in the sample). dCt, delta threshold cycle.
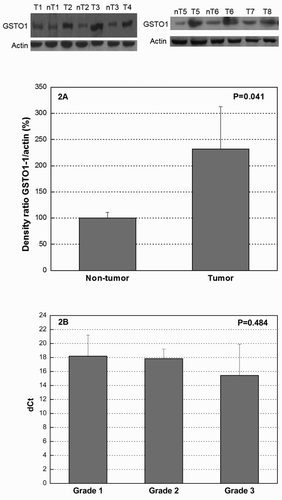
Table 2. Correlation between GSTO1 expression and TCC tumor stage/grade.
3.3. GSTO1-1 association with GSTP1, MAPK, and PI3K signaling molecules
We examined possible association of GSTO1-1 with GSTP1 and some of the MAPK and PI3K pathway signaling molecules, c-Jun, JNK, Akt, phospho-Akt (Ser473/Thr308), and ASK1 proteins by immunoprecipitation. We have shown an association of GSTO1-1 with GSTP1, Akt, phospho-Akt (Ser473/Thr308), and ASK1 proteins. No association of GSTO1-1 and JNK or c-Jun was detected ().
Figure 3. Immunoprecipitation of GSTO1 and associated proteins. TCC tissue lysates were diluted to 1 μg/μl total cell protein and 100 μg/100 μl of total bladder lysates were loaded in each well. GSTO1-associated proteins were immunoprecipitated with the mouse monoclonal anti-GSTO1 antibody and then immunoblotted using appropriate primary antibodies (anti-GSTP1, c-Jun, JNK, Akt, phospho-Akt (Ser473/Thr308), and ASK1). No association of GSTO1-1 and JNK or c-Jun was detected. For western blots reported in the figure, the representative samples are shown.
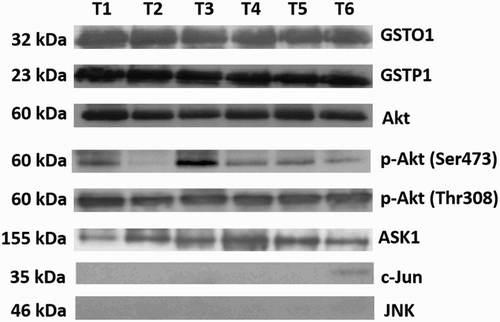
3.4. Decreased total protein glutathionylation in tumor samples
In our study, we used the non-enzymatic method with ο-phthaldialdehyde to measure PrSSG. The obtained results indicated that the content of mixed disulfides was significantly decreased in tumor tissue in comparison with non-tumor tissue (P = 0.034) ().
The level of total protein glutathionylation was assessed by western blot, as well in 19 tumor samples and surrounding normal uroepithelium from patients with TCC. Monoclonal anti-glutathione antibody was used to investigate the total protein glutathionylation ex vivo ((a) and (b)). Equal amounts of loaded protein were verified by Coomassie Blue staining of an equivalent SDS gel (data not shown). (b) shows a number of bands, with molecular masses ranging from 7.2 to 205 kDa, reacting with anti-glutathione antibody, with more intense staining in non-tumor in comparison to the corresponding tumor samples. Densitometric analysis showed significantly decreased levels of protein glutathionylation in tumor tissue in comparison with non-tumor tissue (P = 0.001) ((a)).
Figure 4. (a) Total protein glutathionylation in tumor and the corresponding non-tumor uroepithelial samples of TCC. Representative western blots of glutathionylated proteins are shown (arrows indicating bands of glutathionlylated proteins). The values of densitometric analysis are presented as mean ± SD and the data analyzed by the t-test (graph). Lane T (tumor tissue), lane nT (non-tumor tissue). Lane 1 (left), sample prepared in non-reducing conditions; lane 2 (right), sample treated with DTT presents negative control. (b) Total protein glutathionylation in tumor and the corresponding non-tumor uroepithelial samples of TCC. Representative western blots with anti-glutathione antibody for the purpose of visualizing glutathionylated proteins are shown with arrows indicating bands of glutathionlylated proteins.
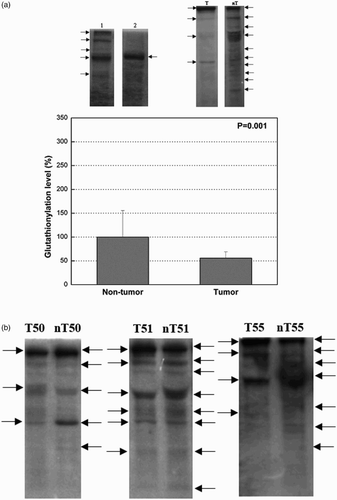
4. Discussion
In the present study, we have shown, for the first time, the upregulated expression of glutathione transferase omega-1 in TCC of urinary bladder, correlating with the malignant phenotype. Furthermore, we have found the decreased level of the total protein S-glutathionylation in TCC tumor tissue compared to surrounding non-tumor uroepithelium. On the basis of immunoprecipitation analysis, we have shown the association of GSTO1-1 with GSTP1, Akt, and ASK1.
Our results on increased glutathione content in tumor in comparison with non-tumor tissue confirmed the existence of dysregulations of glutathione homeostasis in TCC, observed in our earlier reports and the reports of other groups [Citation5]. In addition, thioltransferase activity was significantly higher in tumor compared to non-tumor tissue, showing clear correlation with tumor grade and stage. It is important to note that apart from GSTO1-1, other enzymes, such as glutaredoxins and GSTO2-2, also possess thioltransferase activity and thus could contribute to the observed increase of the activity in TCC tumor tissue [Citation6]. When we compared the level of S-glutathionylation between tumor and the corresponding non-tumor tissue, more intense S-glutathionylation was obtained in the non-tumor tissue. Similar results were obtained in another urological tumor, prostate cancer, where glutathionylation of tumor suppressor protein p53 in tumor tissue was significantly lower, compared to the corresponding normal tissue, both within a single specimen and among different specimens [Citation15]. On the basis of the recent evidence of Menon and Board [Citation6] on the GSTO1-1 role in glutathionylation cycle, we speculated that enhanced deglutathionylase activity of GSTO1 might contribute to the process of deglutathionylation, resulting in decreased total S-glutathionylation level in TCC tumor tissue. Until now, the regulatory role of deglutathionylation (reduction of PrSSG) in redox signal transduction was investigated concerning mainly glutaredoxins as the major intracellular deglutathionylating enzymes in the cells [Citation16]. Moreover, it has been shown that manipulation of glutaredoxin levels affects downstream signaling events by changing the protein glutathionylation status of the cells [Citation16]. Until now, only one study showed increased expression of glutaredoxin 3 in bladder cancer in comparison to the surrounding normal tissue. Interestingly, in contrast to other human isoforms, glutaredoxin 3 has no enzymatic activity but inhibits apoptosis through their interaction with signaling molecules, such as JNK (c-jun N-terminal kinase) [Citation17].
Among potential candidates in TCC cells, which are subjected to redox regulation, we recognized GSTP1, which is known to be overexpressed in TCC [Citation5,Citation18]. This highly expressed tumor protein possesses an intriguing anti-apoptotic role, possibly supported by its protein:protein interaction with JNK [Citation2]. Apart from this, it seems that GSTP1-1 also provides an important link between cellular redox potential and the regulation of kinase pathways involved in apoptosis. Molecular basis which potentially carries the explanation how oxidative stress causes the change in signaling transduction and why GSTP1 represents molecular ‘redox switch’ may be found in the process of S-glutathionylation. Indeed, Tew et al. [Citation3] showed that S-glutathionylation can significantly influence GSTP1:JNK interaction, causing disassociation of the complex leading to apoptosis activation. In this context, the glutathionylation of GSTP1 may represent the important step in apoptosis activation, especially in relation to JNK-MAPK signaling pathway. In TCC, it can be speculated that increased GSTO1 expression through its deglutathionylase activity keeps the GSTP1 in complex with JNK, contributing to the apoptosis resistance. Indeed, the existence of GSTP1:JNK interaction in both TCC 5637 cell line and TCC tumor tissue was confirmed in our earlier investigation [Citation19], while in the current study, we have shown, for the first time, the association between GSTO1 and GSTP1 by immunoprecipitation. Additionally, we have looked for the possible association of GSTO1-1 with some of the signaling molecules of PI3K and MAPK pathways known to be regulated by S-glutathionylation. We have found the association of GSTO1-1 with Akt (total/phosphorylated) and ASK1 proteins. The Akt kinase family is considered to be the main survival pathway in a various cell types, whereas ASK1 is a member of MAP kinase family and as such is involved in proapoptotic signaling [Citation8,Citation20]. We hypothesised that, similarly to GSTP1, which has a 2wide variety of substrate for S-glutathionylase activity [Citation21], the above-mentioned proteins also might be the targets for GSTO1 deglutathionylase activity. Our results further imply a potential role of GSTO1-1 in regulating the activity of different signaling molecules involved in cell death and survival.
In addition to findings on GSTO1-1 upregulation in TCC of urinary bladder, our results clearly demonstrated positive correlation of GSTO1 expression with an ascending histopathological grade and tumor invasiveness. Apart from the similar results obtained in esophageal squamous cell carcinoma [Citation22], the prognostic value of GSTO1 overexpression has been shown even for Barrett’s esophagus, as premalignant lesion [Citation23]. In the future, examination of GSTO1 expression might be used as additional molecular marker for identifying those TCC patients at increased risk of tumor progression.
What is more, the significance of GSTO1 upregulation in the malignant phenotype of TCC should also be considered in relation to chemotherapy resistance. Although there are no data whether GSTO1-1 is active toward the agents used in the chemotherapy of TCC, its upregulation in TCC, together with its new ‘anti-apoptotic’ role [Citation8], may be relevant to the ability of the tumor to develop drug resistance. Moreover, the high toxicity of cisplatin, due to its preferential binding to non-DNA targets, depends on the cellular redox state. Namely, it is known that only 5–10% intracellular concentration of cisplatin is found in DNA fraction, while 75–85% binds to nucleophilic sites of intracellular constituents, such as thiol-containing peptides, proteins, replication enzymes, and RNA [Citation24]. It can be speculated that disturbances in redox status of tumor cells and increased GSTO1 deglutathionylase activity could affect this process through the changes in glutathionylation/deglutathionylation balance, in which case high thiol groups favor cisplatin binding to proteins. Despite the difficulties in this field related to the complexity of biochemical pathways, an understanding of the relationship between redox homeostasis and TCC progression could lead to the development of drugs able to interact with such molecular processes, thereby expanding the therapeutic strategy against TCC. Ramkumar et al. [Citation25] have reported on the development of series of chloroacetamide-containing GSTO1 inhibitors that suppress cancer cell growth, increase the cytotoxic effects of cisplatin, and inhibit tumor growth in colon cancer models as single agent, supporting the notion of GSTO1 as a potential new target in oncology.
5. Conclusions
In this study, we described, for the first time, the mRNA and protein expression pattern of GSTO1 in TCC. Our results provide a basis for the concept that high GSTO1 expression in TCC may be important in the acquisition of an aggressive phenotype, due to its newly discovered deglutathionylase activity which might contribute to redox perturbations present in TCC.
Certain limitations could be considered in our study. A specific assay for GSTO1-1 activity should be used in our future experiments, especially regarding TCC malignant phenotype. In addition, the correlation of GSTO1 expression with other significant clinical and pathologic factors of disease recurrence and progression besides tumor stage and grade will improve the notion of GSTO1 as a potential molecular marker of TCC progression. Nevertheless, this study may offer some essential information that could be the base for future research, especially regarding the GST class omega role in drug resistance.
Disclosure statements
No potential conflict of interest was reported by the authors.
Acknowledgements
We thank technician Mrs Sanja Sekulic for collecting data and support in the manuscript preparation.
ORCID
Tatjana Djukic http://orcid.org/0000-0003-1959-5115
Sonja Suvakov http://orcid.org/0000-0002-3743-1213
Additional information
Funding
References
- Whitbread AK, Masoumi A, Tetlow N, et al. Characterization of the omega class of glutathione transferases. Methods Enzymol. 2005;401:78–99.
- Simic T, Savic-Radojevic A, Pljesa-Ercegovac M, et al. Glutathione S-transferases in kidney and urinary bladder tumors. Nat Rev Urol. 2009;6:281–289.
- Tew KD, Manevich Y, Grek C, et al. The role of glutathione S-transferase P in signaling pathways and S-glutathionylation in cancer. Free Radic Biol Med. 2011;51:299–313.
- Tew KD, Townsend DM. Redox platforms in cancer drug discovery and development. Curr Opin Chem Biol. 2011;15:156–161.
- Savic-Radojevic A, Mimic-Oka J, Pljesa-Ercegovac M, et al. Glutathione S-transferase-P1 expression correlates with increased antioxidant capacity in transitional cell carcinoma of the urinary bladder. Eur Urol. 2007;52:470–477.
- Menon D, Board PG. A role for glutathione transferase Omega 1 (GSTO1-1) in the glutathionylation cycle. J Biol Chem. 2013;288:25769–25779.
- Djukic TI, Savic-Radojevic AR, Pekmezovic TD, et al. Glutathione S-transferase T1, O1 and O2 polymorphisms are associated with survival in muscle invasive bladder cancer patients. PLoS One. 2013;8:e74724.
- Piaggi S, Raggi C, Corti A, et al. Glutathione transferase omega 1-1 (GSTO1-1) plays an anti-apoptotic role in cell resistance to cisplatin toxicity. Carcinogenesis. 2010;31:804–811.
- Eble JN, Sauter G, Epstein JI, et al., editors. World Health Organization classification of tumours. Pathology and genetics of tumours of the urinary system and male genital organs. Lyon: IARC Press; 2004.
- Babjuk M, Burger M, Zigeuner R, et al. EAU guidelines on non-muscle-invasive urothelial carcinoma of the bladder: update 2013. Eur Urol. 2013;64:639–653.
- Witjes JA, Compérat E, Cowan NC, et al. EAU guidelines on muscle-invasive and metastatic bladder cancer: summary of the 2013 guidelines. Eur Urol. 2014;65:778–792.
- Simic T, Mimic-Oka J, Savic-Radojevic A, et al. Glutathione S-transferase T1-1 activity upregulated in transitional cell carcinoma of urinary bladder. Urology. 2005;65:1035–1040.
- Ravindranath V. Animal models and molecular markers for cerebral ischemia-reperfusion injury in brain. Methods Enzymol. 1994;233:610–619.
- Holmgren A, Aslund F. Glutaredoxin. Methods Enzymol. 1995;252:283–292.
- Yusuf MA, Chuang T, Bhat GJ, et al. Cys-141 glutathionylation of human p53: studies using specific polyclonal antibodies in cancer samples and cell lines. Free Radic Biol Med. 2010;49:908–917.
- Gallogly MM, Starke DW, Mieyal JJ. Mechanistic and kinetic details of catalysis of thiol-disulfide exchange by glutaredoxins and potential mechanisms of regulation. Antioxid Redox Signal. 2009;11:1059–1081.
- Cha M-K, Kim I-H. Preferential overexpression of glutaredoxin3 in human colon and lung carcinoma. Cancer Epidemiol. 2009;33:281–287.
- Berendsen CL, Mulder TP, Peters WH. Plasma glutathione S-transferase pi 1-1 AND alpha 1-1 levels in patients with bladder cancer. J Urol. 2000;164:2126–2128.
- Pljesa-Ercegovac M, Savic-Radojevic A, Kravic-Stevovic T, et al. Co-localization of GSTP1 and JNK in transitional cell carcinoma of urinary bladder. Genet Mol Biol. 2010;33:460–462.
- Board PG, Menon D. Glutathione transferases, regulators of cellular metabolism and physiology. Biochim Biophys Acta. 2013;1830:3267–3288.
- Zhang J, Grek C, Ye Z-W, et al. Pleiotropic functions of glutathione S-transferase P. Adv Cancer Res. 2014;122:143–175.
- Li Y, Zhang Q, Peng B, et al. Identification of glutathione S-transferase omega 1 (GSTO1) protein as a novel tumor-associated antigen and its autoantibody in human esophageal squamous cell carcinoma. Tumour Biol. 2014;35:10871–10877.
- Piaggi S, Marchi S, Ciancia E, et al. Nuclear translocation of glutathione transferase omega is a progression marker in Barrett’s esophagus. Oncol Rep. 2009;21:283–287.
- Gómez-Ruiz S, Maksimović-Ivanić D, Mijatović S, et al. On the discovery, biological effects, and use of cisplatin and metallocenes in anticancer chemotherapy. Bioinorg Chem Appl. 2012;2012:1–14.
- Ramkumar K, Samanta S, Kyani A, et al. Mechanistic evaluation and transcriptional signature of a glutathione S-transferase omega 1 inhibitor. Nat Commun. 2016;7:13084.