ABSTRACT
Objectives: The aim of this study was to evaluate the role of the antioxidant barrier in the saliva of children with caries, and its impact on the colonization of cariogenic bacteria.
Methods: This is a cross-sectional study of 81 children aged 1–5 years. Antioxidant levels and salivary bacterial profiles were measured. Patients were divided into two groups as follows: initial stage decay, termed non-cavitated (1–2 in International Caries Detection and Assessment System (ICDAS)), and extensive decay, termed cavitated lesions (5–6 in ICDAS). The control group includes children without caries.
Results: The linear regression model demonstrated that the GSH, GSSG, GSH/GSSG, and total antioxidant capacity levels are influenced (P < 0.05) by: the stage of caries and the dominant bacterial strain. Compared with the other groups (P < 0.001), the highest antioxidant parameters were recorded in the saliva of patients with cavitated lesions.
Discussion: Our results indicate that the high levels of antioxidants in saliva increase significantly in children in line with the salivary cariogenic bacterial profiles and caries progression.
Introduction
Despite technological advancement and the development of new treatment techniques, health requirements for diseases of the oral cavity are increasing. A large number of developing countries struggle with oral cavity health problems [Citation1–3]. A good oral cavity health state is a necessary condition for a healthy lifestyle, which can be influenced by oral cavity diseases such as caries [Citation4]. These problems may affect everyday functions, such as chewing and speech [Citation5]. Initiation of the caries process is asymptomatic, and the consequences of untreated lesions may include missing teeth causing further disorders, such as occlusal abnormalities, temporomandibular complaints including headaches or neuralgias, and even gastrointestinal problems [Citation6]. Untreated carious lesions complicated with chronic disorders of the pulp and periapical tissues constitute a potential focal infection for numerous organs, e.g. the cardiovascular system, nervous system, kidneys, or skeletal system [Citation7–9]. Oral cavity care, including the treatment of carious lesions, is a necessary preliminary condition for the performance of planned procedures, e.g. cardiac surgery and transplantation [Citation10]. The lack of dental care in kindergartens and primary schools, the shortage of children’s dentists, and the wide-ranging indifference of the public health system in this field increase the health problems of the oral cavity, increasing the risk of caries incidence with its early and late complications in the youngest age groups. Currently, the main hypothesis of caries etiology is the ecological plaque hypothesis [Citation11].
The ecological plaque hypothesis assumes that caries development results from a shift in the resident microflora balance driven by changes in local environmental conditions, thus causing the development of more cariogenic species responsible for the advantage of demineralization over remineralization processes.
Bacteria that cause caries, in particular Streptococcus mutans, show a strong response to both environmental acidification and oxidative stress accompanied by, e.g. increased response from bacterial enzyme systems such as the system of intracellular NADH oxidase (Nox; encoded by NOx) as well as the enzymatic systems of the host. An increase in the levels of bacterial antioxidant systems such as NADH oxidase is essential in the regeneration of NAD+ during glycolysis and oxygen reduction, thereby preventing the formation of reactive oxygen species (ROS). On the other hand, in response to the colonization of microorganisms, the host immune system can lead to the development of oxidative stress and increased production of salivary antioxidants. In either case, the role of antioxidants and antioxidant-related mechanisms is not fully understood, although recently the attention of researchers toward those issues is increased.
The part of bacterial antioxidant systems in the regulation and mechanism of action of cariogenic bacteria is strongly associated with acidification of the environment and oxidative stress, through their effects on intracellular levels of NAD+/NADH in Rex-dependent regulation [Citation12,Citation13] (). In the case of a more complex system such as the human body, interactions between microorganisms and the host arising from the role of antioxidants are much more complicated. In the latter case, the antioxidant system of the host acts alongside the antioxidant system of bacteria; moreover, the role of external factors acting on the host, such as diet and hygienic habits, is also not without significance.
Figure 1. Antioxidants’ contribution in regulation and mechanism of action of cariogenic bacteria. Response of S. mutans to oxidative stress caused by ROS produced by neutrophils, fibroblasts, and other cells of the oral cavity is accompanied by, e.g. increased response from the bacterial enzymatic systems as intracellular NADH oxidase (Nox) in the SpxA1/ SpxB/ Rex-dependent modulation, and proteins that affect cellular redox status and are involved in the regulation of S. mutans’s metabolism. Antioxidant systems of the host (e.g. salivary GSH, GSSG, TAC) and exogenous factors (e.g. diet and hygienic habits) act in addition to antioxidant systems of bacteria.
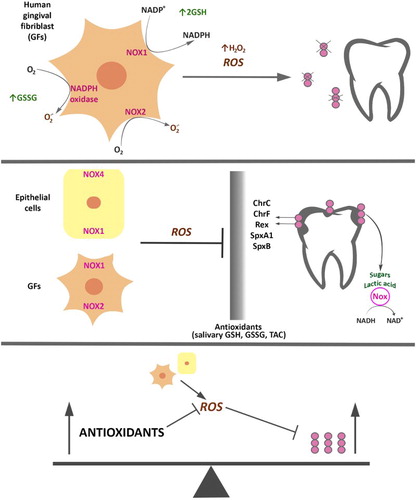
Thus, the number of mechanisms in which oxidative stress can be of pathogenetic significance in caries is very high, and cannot be fully transferred into even the most complex models in vitro. The harmful role of oxidative stress is a result of the direct impact on the basic mechanisms of signal response and transduction, which leads to an increased growth and differentiation of cariogenic bacteria and their increased potency. Strategies for antioxidant therapy of cariogenic bacterial cells in order to protect against damage may be based on an understanding of the molecular aspects of oxidative stress in numerous diseases, including caries and cancer, in which glycolysis is the main source of energy and building block for both cariogenic microorganisms such as S. mutans and cancerous cells, for which low oxygen concentrations (<2%) or hypoxia prevailing in the environment increases the expression of genes encoding glycolytic enzymes and inhibits oxidative phosphorylation. Thus, inhibition of this process seems to be a promising way to effect the eradication of caries [Citation14,Citation15].
So far, there are few studies on the salivary levels of antioxidants in patients with caries in comparison to healthy individuals without symptoms of disease [Citation16]. Although the role of oxidative stress in patients with caries seems to be documented, the majority of published studies provide no explanation of the observed changes in different biological materials and in various forms of the disease. This aspect seems worthy of laboratory verification, as does the role of the determination of selected salivary antioxidants in the colonization of cariogenic strains, especially in the decay of all levels of progression.
The aim of this study was to determine salivary antioxidants GSH, GSSG, and total antioxidant capacity (TAC) in children with initial and advanced caries in comparison to healthy individuals without any changes and to evaluate the role of the salivary antioxidant barrier and its effect on cariogenic bacterial colonization.
Materials and methods
Study groups
The study was performed in accordance with the Helsinki Declaration of 2013. The protocol was approved by the Bioethics Committee at the Jagiellonian University in Krakow (Nr KBET/281/B/2014). Informed consent was obtained from all participants (parents) included in the study.
The study was conducted between 2014 and 2015 and included 80 pediatric patients from the Department of Pediatric Dentistry, Institute of Dentistry, Jagiellonian University in Krakow, Poland. The study included bacterial strains isolated from the saliva of patients (n = 53; average age = 2.74 ± 1.2) diagnosed with early childhood caries (ECC) of the deciduous teeth. The results, marked with reference numbers, were entered onto a standardized examination chart. Examinations were conducted based on the criteria established by the World Health Organization for epidemiological studies (Oral Health Surveys Basic Data), in artificial light using a dental mirror and a probe [Citation17].
Children were divided into two subgroups: subjects with initial stage decay, defined as the non-cavitated group (1–2 in International Caries Detection and Assessment System (ICDAS) II codes; n = 27; average age = 2.35 ± 1.09); and subjects with extensive decay, defined as the cavitated group (5–6 in ICDAS II codes; n = 27; average age = 3.56 ± 1.25). In the ICDAS II classification, code 1 means the first noticeable change in enamel (seen only after prolonged air drying or restricted to within the confines of a pit or fissure, white opacity or brown discoloration), code 2 – distinct visual change in enamel, white opacity or brown discoloration beyond a fissure with no loss of surface integrity, code 5 – distinct cavity with visible dentin, and code 6 – an extensive distinct cavity with visible dentin [Citation18]. Twenty-seven patients were qualified to the non-cavitated group (13 girls, 14 boys; aged 2.35 ± 1.09 years). The selection criterion for the patients with the non-cavitated form of ECC was the presence of white or brown discolorations on the tooth surface with no visible quantitative loss of enamel tissue. A total of 27 patients were assigned to the cavitated group (16 girls, 11 boys: aged 3.56 ± 1.25 years). The selection criterion for the patients with the cavitated form of ECC was the presence of a clear quantitative enamel lesion, i.e. cariogenic change with lesser or greater advanced dentin exposure. The division criterion between the cavitated and non-cavitated groups was the presence of a visible carious lesion.
The control group comprised children (16 girls, 11 boys; average age = 3.11 ± 1.19) free from caries, who were maintained under clinical control.
The ages of patients in the individual groups with ECC did not differ significantly from the ages of children in the control group. There were no significant differences in sex between the study and control groups.
The exclusion criteria were as follows: aged below 2 or above 6 years; diabetes; periodontal disease; epithelial dysplasia; and inflammatory lesions of the oral mucosa. Antibiotics, non-steroid, anti-inflammatory medications, corticosteroids, and vitamin intake within the last 3 months also resulted in a patient’s exclusion from the study.
Dental plaque was determined using the Simplified Oral Hygiene Index. The Oral Hygiene Index (OHI) assesses the presence of dental plaque on 6 teeth of different groups (55, 53, 51 and 75, 73, 71), and on the labial surfaces of the maxillary teeth and lingual surfaces of the mandibular teeth. 0 – means lack of deposits, 1 – deposits cover up to 1/3 of the surface, 2 – 1/3 to 2/3 of the surface, and 3 – over 2/3 of the surface of the examined tooth [Citation19]. Daily oral hygiene consisted of brushing the teeth 2 times per day with a manual brush and a fluoride toothpaste. The OHI value in all examined children was 0.
This manuscript contains data from clinical studies (including patient data). Details that might disclose the identity of the subjects under study have been removed.
Calibration and reproducibility
The classification of the study group was performed during a routine dental examination. This examination was conducted by a qualified dentist (internal examiner) with many years of experience in epidemiological studies of the oral cavity. The coherence of the diagnosis by the external researcher was tested by the evaluation of variability between researchers: external, internal, and third [Citation20]. All three researchers were independent of each other. The internal researcher conducted the same tasks. In the next step, each researcher was provided with photographs prepared by their counterpart and prepared descriptions for these photographs on three separate occasions, each time working independently of the other two researchers. Furthermore, to ensure the consistency in caries diagnosis, a third researcher participated in the study, carrying out the above tasks in an analogous method. To evaluate the agreement between the researchers, 20% of the samples were selected at random for the study and these were subjected to analysis by the above researchers on different occasions during a one-year trial period.
Saliva collection
After the classification of the patients for the research, unstimulated saliva samples (2 ml) were collected using a Salivette® Cotton Swab system (Sarstedt AG & Co., Numbrecht, Germany). The subjects rinsed their mouths for 1 minute with distilled water, which was then spit out before saliva collection. A cotton swab was placed in the mouth and chewed for 3 minutes to stimulate salivation. Material was collected from fasted participants in the morning (between 8 and 10 am) before toothbrushing and any clinical examination. The collected saliva was put into sterile tubes inhibiting O2 and carried on ice to the laboratory within 1 hour. Saliva samples were then homogenized and clarified by centrifugation at 500 × g for 10 min at 4°C. The aliquots of clarified supernatants were kept at −80°C until needed for the measurements.
Microbial
Evaluation of cariogenic bacteria on HLR-S medium
Salivary levels of S. mutans, Lactobacillus rhamnosus, Streptococcus sanguinis, and Streptococcus mitis were determined using selective agar.
The saliva was inoculated on the modified medium of Ritz (HLR-S) agar medium in the laboratory. It was assumed that the role of the medium consisted of support for the growth of the tested microorganisms and inhibition of the remaining species found in the saliva. The incubation time was 48 h under 85% N2, 10% CO2, 5% O2 conditions at 37°C. The HLR-S medium was selected based on our previous study, which had tested media samples described in the literature as selective for specific bacterial species isolated directly from the oral cavity (from smears or saliva samples) [Citation21,Citation22].
Individual cultivated colonies were inoculated on the tryptic soy agar medium with 5% sheep blood, incubated for 24 hours under microaerophilic conditions (85% N2, 10% CO2, 5% O2). Then, the morphological characteristics of individual colonies cultivated on the medium with sheep blood were evaluated, as was the type of hemolysis caused by these colonies. In addition, saliva dilutions were performed, and these were inoculated on the HLR-S medium. The colonies once grown were counted via determination of CFU/ml. The number of microorganisms present in a particular test sample was determined using the formula:
Bacterial identification
Phenotypic identification of the four cultivated bacterial species was performed using a commercial biochemistry identification set: STREPTOtest24 (Lachema, Pliva).
Biotyping from selected enzymatic reactions of the above commercial test was performed for four dominant bacterial species: S. mutans, L. rhamnosus, S. sanguinis, and S. mitis. Two approaches to biotyping criteria based on enzymatic activity profiles were used. The first approach consisted of the selection of appropriate enzymes based on the analysis of their activity in the population of the tested strains. Enzymes found in all the strains (LAP, bGA, Aga, ESL, MAN, SOR, LAC, MLT, RAF, TRE) tested for growth in the presence of 6.5% w/v NaCl, which was negative for all strains, enzymes which were not found in any of the strains (bMN, GLR, RIB, PUL, ARG, AMG, SOE), and enzymes found only in single species were not suitable for differentiating and were rejected per se.
The second method for biotype determination consisted of the use of an unattended (without a priori available knowledge) statistical data analysis. In this method, data were divided into groups (clusters), so that each group was as homogenous as possible and, at the same time, clusters differed from one another (strains from different groups had the lowest possible number of common characters) [Citation23].
GSH/GSSG determination
For determination of thiol status, both reduced (GSH) and oxidized (GSSG) forms of glutathione were measured. Determination of GSH and GSSG levels was based on the reaction of the Griffith [Citation24] method with the Tietze modification [Citation25], as described in Current Protocols in Toxicology by Mustacich [Citation26] and measured at λmax = 412 nm.
The following reagents purchased from Sigma-Aldrich were used: 1% (w/v) picric acid as a protein precipitating reagent, 143 mM sodium phosphate pH = 7.5, 0.3 mM NADPH, 6 mM 5-5′-dithiobis [2-nitrobenzoic acid] (DTNB), 50 U/ml GSH reductase in 143 mM sodium phosphate pH = 7.5, 2-vinylpyridine, and triethanolamine. In order to determine the level of GSSG, 0.1 ml of acidic extract was mixed with 0.002 ml of 2-vinylpyridine and vortexed and the pH value was adjusted to a level of 6–7 with triethanolamine. Samples were derivatized for 1 hour at room temperature. In order to determine total glutathione (GSH), a mixture including 0.7 ml 0.3 mM NADPH, 0.1 ml 6 mM DTNB, 0.1 ml picric acid extract and 0.1 ml metal-free water at a total volume of 1 ml was heated at 30°C for 15 minutes and then transferred to another tube containing 0.010 ml 50 U/ml glutathione reductase, and absorbance at wavelength λmax = 412 nm was monitored every 15 seconds for 2.5 minutes. Measurements were performed in three repetitions. The quantitative determination of the total glutathione (GSH) was obtained with reference to the non-derivatized samples on a calibration curve, which was based on GSH dilutions in 1% (w/v) picric acid. For the determination of the quantitative level of oxidized glutathione (GSSG), a second calibration curve was prepared using samples treated with 2-vinylpyridine and triethanolamine. Quantitative determination of oxidized glutathione (GSSG) was obtained via reference to the derivatized samples on the previously prepared calibration curve.
Result readings were performed on a FLUOstar Omega spectrophotometer – BMG Labtech, Ortenberg, Germany.
TAC determination
Determination of salivary TAC as the reduction of Fe3+ ions was performed using Benzie and Strain’s method [Citation27].
On the day of determination, a working solution was prepared, which contained 100 ml acetate buffer, 10 ml tripyridyltriazine solution, and 10 ml ferrous chloride(III). Wells of a 96-well plate were filled with 0.015 ml of the studied sample, blank test with distilled water or standard solution. Then, 0.300 ml of the working substrate solution was added and the contents were mixed. Absorbance measurements were conducted at a temperature of 37°C, at a wavelength of 593 nm. Result readings were conducted after 10 min on a FLUOstar Omega – BMG Labtech, Ortenberg, Germany. Reagents were purchased from Sigma-Aldrich, St. Louis, MO, USA.
Total protein amount
Total protein (TP) levels were determined in all groups. The protein concentrations of the samples were determined using a bicinchoninic acid assay kit (Sigma-Aldrich, USA), as described by Smith et al. [Citation28]. A standard solution of bovine serum albumin at a range of 200–1000 mg/ml was used. The blind sample was prepared for each series in 10 repetitions. The absorbance of each study sample was determined at a wavelength of λmax = 562 nm, at a temperature of 37°C. The concentration of protein was calculated on the basis of the calibration curve and expressed in mg/ml.
Statistical analysis
Statistical analysis was performed using R 3.2.3. (R: Development Core Team (2009); R: A language and environment for statistical computing; R: Foundation for Statistical Computing, Vienna, Austria. ISBN 3-900051-07-0, URL http://www.R-project.org.) [Citation29]. Data are expressed as median ± upper and lower quartiles. To determine the relationship between GSH, GSSG, and TAC, which were not normally distributed, and the stage of diseases and cariogenic bacterial profiles, the Kruskal–Wallis test was used. A value of P < 0.05 was considered statistically significant. Superscripts a, b, c denote groups differing in Bonferroni-corrected Mann–Whitney tests. An analysis of covariance (ANCOVA) was performed to assess the connections between GSSG, TAC, and GSH, and the forms of the disease, and this was corrected to take into account the effect of confounding factors (age, sex).
Results
Calibration for ECC scoring
The Kappa coefficient value for caries evaluation for the external researcher amounted to 0.95 during the evaluation conducted during the first half of the year, and 0.97 for the evaluation carried out in the second half of the year of the study. The Kappa coefficient values for caries evaluation for the internal researcher ranged from 0.92 to 0.96 in the above-mentioned time-period. In reference to the third expert, Kappa coefficient values in the range from 0.94 to 0.95 were recorded.
Microbial characteristics of tested bacterial strains
The isolated bacterial species were Gram-stained and observed under an optical microscope at 40× magnification and confirmed under a scanning electron microscope (SEM) at 11 000× magnification. The sizes of the observed colonies remained in the range of 0.5–1 µm, positive Gram-stained. The spatial distribution of the cells resembled bead-long chains ().
Figure 2. Morphological assessment of Streptococcus sp. bacteria. Photograph shows macro- and micromorphology of Streptococcus sp. 1 – S. mutans colonies with characteristic white coloration on the tested medium; 2 – on blood agar (BA); 3 – S. mutans cells in Gram-stained preparation. Photograph 3 taken with an Olympus CX41 microscope, coupled to a CaMedia C5550 camera; 4 – S. mutans cells in a JEOL JSM-35CF SEM.

The tested bacterial species did not produce catalase. On the medium with sheep blood, α-hemolysis was observed for S. sanguinis, S. mitis, and Lactobacillus acidophilus and γ-hemolysis for S. mutans.
Bacterial identification
Eighty isolated strains were typed biochemically with STREPTOtest 24 with a discrimination rate of more than 95%. In total, 47 (58.75%) strains belonging to three Streptococcus species were isolated, as well as 25 (31.25%) strains belonging to the genus Lactobacillus. Streptococcus was the most frequent species (n = 47; 58.75% strains), followed by S. mutans (n = 12; 15% strains), and then S. sanguinis and S. mitis (n = 23; 28.75% and n = 12; 15% strains) – the percentage of each species is shown in the graph ().
Figure 3. The percentage share of individual bacterial strains isolated from saliva of children with cavitated ECC, non-cavitated ECC, and children without symptoms of the disease.
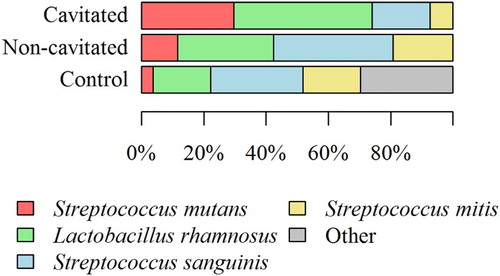
As presented in , many bacterial species form characteristic profiles. Microbial cultures (from the saliva of children with caries and those without symptoms of the disease) on selective medium (HLR-S) and their subsequent phenotypic identification allowed for the determination of the percentage share of individual bacterial strains. Interestingly, the bacterial profile depended on the stage of the disease. In children from the non-cavitated group, the following species were dominant: S. sanguinis (38.46%), L. rhamnosus (30.77%), S. mitis (19.23%), and S. mutans (11.54%) (). In the group of children with cavitated lesions – advanced stage ECC, the following species dominated: L. rhamnosus (44.44%), S. mutans (29.63%), and S. sanguinis (18.52%) (). In the control group, the percentage share of individual bacterial species was as follows: S. mutans (3.70%), S. mitis (18.52%), L. rhamnosus (18.52%), S. sanguinis (29.63%), and other (29.63%).
Figure 4. (a). Salivary reduced-glutathione levels in all groups with ECC and in the control group. The median and interquartile range (box), and percentile 5–95% range (whiskers) are shown; P-values are given for the comparison of groups with each other (***P < 0.001 post-hoc Mann–Whitney test with Bonferroni correction). Cavitated patients with ECC; non-cavitated patients with ECC; control without lesions. (b). Salivary GSSG levels in all groups with ECC and in the control group. The median and interquartile range (box), and percentile 5–95% range (whiskers) are shown; P-values are given for the comparison of groups with each other (***P < 0.001 post-hoc Mann-–Whitney test with Bonferroni correction). Cavitated patients with ECC; non-cavitated patients with ECC; control without lesions. (c). Salivary TAC levels in all groups with ECC and in the control group. The median and interquartile range (box), and percentile 5–95% range (whiskers) are shown; P-values are given for the comparison of groups with each other (***P < 0.001 post hoc Mann–Whitney test with Bonferroni correction). Cavitated patients with ECC; non-cavitated patients with ECC; control without lesions.
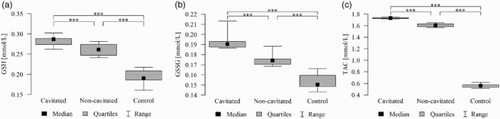
GSH/GSSG
Differences in GSH, GSSG concentration, and GSH/GSSG ratio in the saliva between groups were statistically significant, i.e. higher in cavitated and non-cavitated groups than in the control group ( and ). Differences in GSH and GSSG concentrations in the saliva between groups were also statistically significant, i.e. higher in the cavitated group than in the non-cavitated and control groups ( and ). GSH and GSSG concentrations differed in individual groups (patients with cavitated and non-cavitated forms) ( and ) and these were also statistically significant ((a,b)).
Table 1. Adjusted relationships between reduced glutathione, oxidized glutathione and TAC and salivary bacterial profiles and dental caries progression.
Table 2. Relationship between salivary glutathione and TAC levels, and salivary bacterial profiles and dental caries.
The highest GSH concentrations were recorded in the saliva of patients from the cavitated group: 0.287 (0.274–0.288) mmol/l (median (lower quartile – upper quartile)), whereas GSSG concentrations were 0.191 (0.187–0.193) mmol/l and 1.49 for the GSH/GSSG ratio, respectively ( and ). The differences were statistically significant for the discussed parameters (P < 0.001).
Concentrations of GSH and GSSG were significantly higher in boys and these were positively correlated with age (older age = higher levels). The concentration of glutathione showed a correlation with age (r = 0.294; P = 0.008) and differed between the groups of girls and boys (P = 0.007; Mann–Whitney test) (). The concentration of glutathione disulfide correlated with age (r = 0.287; P = 0.01) and differed between the groups of girls and boys (P = 0.04; Mann–Whitney test) (). For the GSH/GSSG ratio, no relationship with age was observed (r = 0.036; P = 0.75), but the girls and the boys demonstrated different GSH/GSSG ratios (P = 0.032; Mann–Whitney test) ().
Total antioxidant capacity
Differences in TAC concentrations in the saliva between groups were statistically significant, i.e. higher in cavitated and non-cavitated groups than in the control group (; ). Differences in TAC concentrations in the saliva between groups were also statistically significant, i.e. higher in the cavitated group than in the non-cavitated and control groups (; ). TAC concentrations differed in individual groups (patients with cavitated, and non-cavitated forms) ().
The highest TAC concentrations were recorded in the saliva of patients from the cavitated group: 1.73 (1.72–01.74) mmol/l (median (lower quartile – upper quartile) ( and ). The differences were statistically significant for the discussed parameters (P < 0.001). Concentrations of TAC were significantly higher in boys and these were positively correlated with age (older age = higher levels). In terms of TAC value, its concentration positively correlated with age (r = 0.234; P = 0.036) and the ratio was different in the groups of girls and boys (P = 0.022; Mann–Whitney test) ().
TP content
Inter-group differences in salivary protein concentrations were statistically significant, i.e. in cavitated and non-cavitated groups (1.86 (1.52–2.30) mg/ml and 1.79 (1.40–2.42) mg/ml, respectively), and these were higher than in the control group 1.65 (1.41–2.44) mg/ml. However, there was no significant correlation between the concentrations of the studied markers and the age and gender of the study participants.
Antioxidants (GSH/GSSG, TAC), cariogenic profiles and caries
After considering age, sex, eating habits (i.e. daily consumption of fruit juices and carbonated drinks), and feeding type (natural, artificial, mixed), the antioxidants in the saliva (GSH, GSSG, and TAC) demonstrated a certain dependency on the occurrence of cariogenic profiles and also on the stage of caries in children. The linear regression model demonstrated that the GSH level is influenced (P < 0.05) by the stage of caries and the dominant bacterial strain. In comparison to the controls, caries from the non-cavitated group increased GSH by an average of 0.061 mmol/l; on the other hand, the cavitated form increased GSH by an average of 0.075 mmol/l. Regarding dominant bacterial species, as compared with dominance of S. mutans, S. sanguinis dominance decreased GSH by an average of 0.018 mmol/l. Streptococcus mitis dominance decreased GSH by an average of 0.327 mmol/l, whereas the dominance of the so-called ‘other’ strains decreased GSH by an average of 0.321 mmol/l. For GSSG, the linear regression model demonstrated that the GSSG level is influenced (P < 0.05) by the stage of caries. In comparison to the control, the non-cavitated form increased GSSG by an average of 0.171 mmol/l, and the cavitated form increased GSSG by an average of 0.316 mmol/l. For the dominant bacterial strain, it was demonstrated that the L. rhamnosus dominance decreased GSSG by an average of 0.011 mmol/l, S. sanguinis dominance decreased GSSG by an average of 0.150 mmol/l, S. mitis dominance decreased GSSG by an average of 0.202 mmol/l, and the ‘other’ strain dominance decreased GSSG by an average of 0.240 mmol/l. For TAC, the linear regression model demonstrated that the TAC level is influenced (P < 0.05) by the stage of caries and bacterial strain dominance. In comparison to the control, the non-cavitated level increased TAC by an average of 1.036 mmol/l, and the cavitated level increased TAC by an average of 1.153 mmol/l. On the other hand, for a dominant bacterial strain and its relationship with the level of the TAC expressed as TAC in comparison to the S. mutans dominance, S. sanguinis dominance decreased TAC by an average of 0.023 mmol/l, S. mitis decreased TAC by an average of 0.046 mmol/l, and the ‘other’ strain dominance decreased TAC by an average of 0.053 mmol/l. In the analysis of the GSH/GSSG ratio, the linear regression model demonstrated that the GSH/GSSG level is influenced (P < 0.05) by the caries stage and dominant bacterial strain. Compared with the control, the non-cavitated form of caries increased GSH/GSSG by an average of 0.222, whereas the cavitated form increased GSH/GSSG by an average of 0.183. For a dominant bacterial species and in comparison to the dominance of S. mutans, the dominance of L. rhamnosus increased GSH/GSSG by 0.05 on average.
Discussion
ROS are produced during a bacterial infection and are involved in intracellular signaling. However, the effect of oral bacteria on the ROS induction and associated interspecies influence is the subject of many studies and has yet to be clarified [Citation30].
One of the concepts that is verified in our study concerns the impact of enzymatic and non-enzymatic antioxidants on ROS induction, and thus, the response of bacterial cells. Our study demonstrated higher concentrations of GSH, GSSG, and TAC in saliva of patients with the advanced form of caries (cavitated group) in comparison to patients with non-cavitated forms. Additionally, the changes in the given parameters (GSH, GSSG, and TAC) are higher in children with caries in comparison to those without; moreover, cariogenic bacterial profiles were significantly higher in saliva of ECC children in comparison to healthy volunteers. The observed changes can be linked to a protective effect of antioxidants on pathogenic bacterial strains. When the antioxidant concentration is high, ROS produced by neutrophils, fibroblasts, and other oral cells are not active and thus, cariogenic bacteria are more likely to multiply and divide, consequently leading to the destruction of the hard tissues of the tooth. The antioxidant system of saliva, such as glutathione GSH, GSSG, and TAC, additionally (except for antioxidant defense systems of host immune system cells) participates in the weakening of resistance to the bacteria colonizing the oral cavity, ensuring their increased vulnerability to different kinds of stress. Our research confirms this hypothesis as a result of the relationship between the increased levels of the above antioxidant parameters and caries progression in children, which have been illustrated in and and . As can be seen, the GSH/GSSG level is closely related to the bacterial profiles and exhibits a significant correlation with the developmental stage of the ECC (). Similarly, TAC values in the saliva are 2.86 times higher in children with the advanced form of caries (cavitated lesions group) and 3.09 times higher in children with the initial form (non-cavitated lesions group) than in children without symptoms of the disease. The authors also suggest that TAC values exhibit a relationship with age (). These results are consistent with the results of Uberos et al. [Citation31], who demonstrated that the salivary TAC level in patients with caries is 2.89 times higher than that in control groups. A review of the literature on the topic of ‘different TAC determination methods in the saliva of persons with caries’ can be found in Kumar et al.’s study [Citation32]. Our study is the first to demonstrate the dependency of increases in TAC values on the progression of the disease and cariogenic profiles. This study is also the first to show the positive correlation between the TAC level and the age of children (r = 0.234; P = 0.036) (c)).
Confirmation of the hypothesis of the role of antioxidants in cariogenic bacterial colonization can also be seen in our results, i.e. the GSH/GSSG ratio is strongly related to the developmental stage of caries in children. Compared with the control, GSH/GSSG in the non-cavitated group is increased on average by 0.222 (95% confidence interval is 0.184–0.260), whereas in the cavitated group – this increase is 0.183 (95% confidence interval is 0.147–0.220).
The correlation adjusted factor R² for this model was 77.56%, which means that 77.56% of GSH/GSSG variability was explained by the bacterial profile and stage of caries. The remaining 20.45% depends both on variables not included in the model and on random factors. Further study on the mechanisms of the occurring changes is necessary to confirm our hypothesis. Our study is one of few providing evidence for the presence of a relationship between the level of antioxidant markers (GSH, GSSG, GSH/GSSG, and TAC) and the level of cariogenic bacteria in the saliva and the developmental stage of caries in children. Moreover, those salivary profiles of bacteria considered to be cariogenic positively correlate with the stage of caries in children, which corroborates the results of Han et al. [Citation33].
Our results are also confirmed by the results of Mahjoub et al. [Citation34], where attention was paid to the increased TAC value in children with caries compared with children without symptoms of the disease. The authors suggest that increased salivary TAC values occur in advanced caries, which is also corroborated by our study, where these changes are statistically significant even between groups, i.e. non-cavitated and cavitated lesions groups (c)).
Comparing our results with those obtained by other researchers, it can be seen that species frequencies observed in our study in the group of children with early stage of caries (non-cavitated form), i.e. S. sanguinis (38.46%), L. rhamnosus (30.77%), S. mitis (19.23%) and S. mutans (11.54%) () are consistent with the results of Aas et al. [Citation35] and van Hout et al. [Citation36], who demonstrated that 10% of children and teenagers (aged 2–21) did not have S. mutans at all. This also corroborates our earlier study, in which S. mutans constituted less than 9% in this group [Citation23].
Regarding the advanced stage of caries, S. mutans was not dominant, unlike Lactobacillus, Bifidobacterium, and Prevotella species. In our study, L. rhamnosus dominated in the cavitated group (44.44%). The virulence of populations of cariogenic bacteria correlates with the phenotype accepted for a given environment related to the acidogenic potential of bacteria that may induce changes in the environment leading to dental caries development [Citation37]. Our results (S. mutans 29.63%) are consistent with the results of Gross et al. [Citation38] and Sampaio-Maia and Monteiro-Silva [Citation37], in which the participation of S. mutans in dentin damage amounted to approximately 30% of total microflora.
In the case of initial caries (non-cavitated form), the percentage of S. mutans was higher than that in the control group (11.54% white spot >3.70% control), which confirms van Hout et al.’s study, in which S. mutans constituted from 0.001 to 10% [Citation36]. The Streptococcus species (except for S. mutans) and Actinomyces are considered the main culprits of enamel damage. In the presence of S. mutans and the absence of Lactobacillus, initial demineralization of the enamel may be induced by early colonizers (S. sanguinis, S. mitis, and S. oralis), which was also presented in this study, where S. sanguinis dominated (38.46%) in children from the non-cavitated lesions group. The participation and presence of species considered to be early colonizers and their dominance over other species seem to be indirectly associated with the antioxidant-associated mechanism discussed above.
As has been shown by Ahn et al. [Citation39], colonization of Porphyromonas gingivalis can only occur in the presence of Fusobacterium nucleatum, which initiates activation of the NADPH oxidase in a host cell, leading to a positive impact on P. gingivalis growth. This concept also indirectly explains the presence of so-called early and late colonizers in dental plaque and the dominance of one species over another.
The reduction in oxidative stress resulting from increased expression of the salivary antioxidant enzymes may be a consequence of dietary habits and food components (e.g. probiotics and antioxidants contained in food, sucrose supplementation, smoking, alcohol intake, etc.), which could increase the absorption of micro- and macro-nutrients, including antioxidants [Citation40]. Similar observations were made by Hathout et al. [Citation41], who showed that oral administration of Lactobacillus casei and Lactobacillus reuteri resulted in an increase in the TAC, expressed as TAC. On the other hand, other studies have observed a significant increase in the activity of antioxidant enzymes (CAT, SOD, and GPx) after 90 days of supplementation with L. casei ssp [Citation42].
Fluctuations in the salivary antioxidants suggest that saliva may be a proper marker for the diagnosis of oral pathologies similar to conventional invasive antioxidant serum markers. As a facilitating fluid, saliva is also an important medium for the exchange of different micro-elements, as well as taking part in oxidative stress and antioxidant activity. Therefore, it is important to conduct studies in varying disciplines with respect to understanding the process of releasing micro-elements into saliva which might aid effective pharmacological treatment and enhance host resistance therapy.
Conclusions
Antioxidants are necessary for our health, but we do not know their exact mechanism and how to action this in oral pathologies. Therefore, further research is required to enable a better understanding of salivary antioxidants and their role in health and disease, and not only in in vitro models.
Based on the results of the present study, we can conclude that in the group of patients with ECC, both in the non-cavitated and in the cavitated form, the salivary antioxidant potency, cariogenic bacterial profiles, and caries progression are increased. Further studies are recommended to clarify whether these markers may play an essential role in the pathogenesis of ECC.
Ethical approval
Consents were obtained from all patients, and the study procedure was approved by the Bioethical Committee of the Jagiellonian University in Krakow (Opinion No. KBET/281/B/2014).
Geolocation information
Krakow, Poland.
Disclosure statement
No potential conflict of interest was reported by the author(s).
Notes on contributors
Dr Anna Jurczak is the head of Department of Paediatric Dentistry, Institute of Dentistry, Jagiellonian University, Krakow, Poland. She is the chairman of Polish Society of Paediatric Dentistry. Her searching field is ECC.
Dr Dorota Kościelniak is an academic and scientific assistant professor at the Departure of Pediatric Dentistry Institute of Dentistry, Jagiellonian University, Krakow, Poland. She is a member of Polish Dental Association, Polish Society of Paediatric Dentistry, International Association of Paediatric Dentistry. She has written over 41 articles in Polish and international scientific journals.
Dr Anna Skalniak is a biotechnologist, medical analyst, and biostatistician and has been working in the field of molecular genetic diagnostics since 2010. Dr Anna Skalniak is a member of the Polish Society of Human Genetics, and the Polish and European Societies of Endocrinology, and the ambassador of the European Association for Cancer Research.
Dr Papież is an assistant professor in the Department of Cytobiology, Faculty of Pharmacy, Medical College, Jagiellonian University in Krakow, Poland. She is an expert in redox signaling and oxidative stress in cancer. She published 30 papers in peer-review journals on cancer pathomechanism and therapy. She is a member of Polish Society of Oncology and Polish Society for Histochemistry and Cytochemistry.
Palina Vyhouskaya is a student of Laboratory Medicine at Jagiellonian University, Poland. She is a member of The Scientific Students Association of Laboratory Diagnosticians, where she gains experience and undergoes practice connected with research methods used in medicine.
Dr Krzyściak is an assistant professor in the Department of Medical Diagnostics, Faculty of Pharmacy, Medical College, Jagiellonian University in Krakow, Poland. She is an expert in Clinical Biochemistry. She published 20 papers in peer-review journals on caries pathogenesis and redox signaling. She is a member of Polish Society of Microbiologists and Polish Society of Biochemistry.
Additional information
Funding
References
- Olerud E, Hagman-Gustavsson ML, Gabre P. Oral health status in older immigrants in a medium-sized Swedish city. Spec Care Dentist. 2016;36:328–334.
- Tarvonen PL, Sipilä K, Yang GS, et al. Comparison of two preventive interventions on dental caries among children in Democratic People's Republic of Korea. Int J Dent Hyg. 2016;14(4):301–306.
- Natapov L, Sasson A, Zusman SP. Does dental health of 6-year-olds reflect the reform of the Israeli dental care system? Isr J Health Policy Res. 2016;5:5–26.
- Yoshioka M, Hinode D, Yokoyama M, et al. Relationship between subjective oral health status and lifestyle in elderly people: a cross-sectional study in Japan. ISRN Dent. 2013;2013:687139. Available from: https://www.hindawi.com/journals/isrn/2013/687139/
- Castrejón-Pérez RC, Borges-Yáñez SA, Gutiérrez-Robledo LM, et al. Oral health conditions and frailty in Mexican community-dwelling elderly: a cross sectional analysis. BMC Public Health. 2012;12:773.
- Thekiso M, Yengopal V, Rudolph MJ, et al. Caries status among children in the West Rand District of Gauteng Province, South Africa. SADJ. 2012;67:318–320.
- Siahi-Benlarbi R, Nies SM, Sziegoleit A, et al. Caries-, Candida- and Candida antigen/antibody frequency in children after heart transplantation and children with congenital heart disease. Pediatr Transplant. 2010;14:715–721.
- Oliveira FA, Forte CP, Silva PG, et al. Molecular analysis of oral bacteria in heart valve of patients with cardiovascular disease by real-time polymerase chain reaction. Medicine. 2015;94:e2067. Available from: https://www.ncbi.nlm.nih.gov/pubmed/26632711
- Cotti E, Dessì C, Piras A, et al. Can a chronic dental infection be considered a cause of cardiovascular disease? A review of the literature. Int J Cardiol. 2011;148:4–10.
- Rauschmann MA, Thomann KD, Schwetlick G, et al. From “wet caries” to controllable complication. History of post-traumatic and postoperative osteitis. Orthopade. 2004;33:389–396.
- Usha C, Sathyanarayanan R. Dental caries – a complete changeover (Part I). J Conserv Dent. 2009;12:46–54.
- Baker JL, Derr AM, Faustoferri RC, et al. Loss of NADH oxidase activity in Streptococcus mutans leads to rex-mediated overcompensation in NAD+ regeneration by lactate dehydrogenase. J Bacteriol. 2015;197:3645–3657.
- Bitoun JP, Wen ZT. Transcription factor Rex in regulation of pathophysiology in oral pathogens. Mol Oral Microbiol. 2016;31(2):115–124.
- Ko YH, Domingo-Vidal M, Roche M, et al. TP53-inducible glycolysis and apoptosis regulator (TIGAR) metabolically reprograms carcinoma and stromal cells in breast cancer. J Biol Chem. 2016;291(51):26291–26303.
- Cvitkovitch DG, Hamilton IR. Biochemical change exhibited by oral streptococci resulting from laboratory subculturing. Oral Microbiol Immunol. 1994;9(4):209–217.
- Silva PV, Troiano JA, Nakamune AC, et al. Increased activity of the antioxidants systems modulate the oxidative stress in saliva of toddlers with early childhood caries. Arch Oral Biol. 2016;70:62–66.
- WHO: Oral Health Surveys. Basic methods. 4th ed. Geneva: World Health Organization; 1997.
- International Caries Detection and Assessment System Coordinating Committee Rationale and Evidence for the International Caries Detection and Assessment System (ICDAS II). 2012.
- Wei SH, Lang NP. Periodontal epidemiological indices for children and adolescents: II. Evaluation of oral hygiene; III. Clinical applications. Pediatr Dent. 1982;4:64–73.
- Oliveira AFB, Rosenblatt A. Prevalência de defeitos do esmalte e cárie dentária em crianças pré-escolares em João Pessoa ⁄ Brasil. Rev ABO Nac. 2004;12:107–110.
- Hildebrandt GHl, Bretz WA. Comparison of culture media and chairside assays for enumerating mutans streptococci. J Appl Microbiol. 2006;100:1339–1347.
- Wan AK, Seow WK, Walsh LJ, et al. Comparison of five selective media for the growth and enumeration of Streptococcus mutans. Aust Dent J. 2002;47:21–26.
- Krzyściak W, Pluskwa KK, Piątkowski J, et al. The usefulness of biotyping in the determination of selected pathogenicity determinants in Streptococcus mutans. BMC Microbiol. 2014;14:194. Available from: https://www.ncbi.nlm.nih.gov/pubmed/25096795
- Griffith OW. Determination of glutathione and glutathione disulfide using glutathione reductase and 2-vinylpyridine. Anal Biochem. 1980;106:207–212.
- Tietze F. Enzymic method for quantitative determination of nanogram amounts of total and oxidized glutathione: Applications to mammalian blood and other tissues. Anal Biochem. 1969;27:502–522.
- Mustacich D. Measurement of glutathione and glutathione disulfide. Curr Protoc Toxicol. 2005;6:1027–1029.
- Benzie IF, Strain JJ. The ferric reducing ability of plasma (FRAP) as a measure of “antioxidant power”: the FRAP assay. Anal Biochem. 1996;239:70–76.
- Smith PK, Krohn RI, Hermanson GT, et al. Spectrophotometric total protein assay with copper(II)–neocuproine reagent in alkaline medium. Talanta. 2006;68:1601–1609.
- R: Development Core Team. R: A language and environment for statistical computing; R: Foundation for Statistical Computing, Vienna, Austria. 2009. http://www.R-project.org.
- Tóthová L, Kamodyová N, Červenka T, et al. Salivary markers of oxidative stress in oral diseases. Front Cell Infect Microbiol. 2015;5:73. Available from: https://www.ncbi.nlm.nih.gov/pubmed/26539412
- Uberos J, Alarcón JA, Peñalver MA, et al. Influence of the antioxidant content of saliva on dental caries in an at-risk community. Br Dent J. 2008;205(2):E5. Available from: https://www.ncbi.nlm.nih.gov/pubmed/18545268
- Kumar D, Pandey RK, Agrawal D. An estimation and evaluation of total antioxidant capacity of saliva in children with severe early childhood caries. Int J Paediatr Dent. 2011;21:459–464.
- Han DH, Kim MJ, Jun EJ, et al. The role of glutathione metabolism in cariogenic bacterial growth and caries in Korean children. Arch Oral Biol. 2013;58:493–499.
- Mahjoub S, Ghasempour M, Gharage A, et al. Comparison of total antioxidant capacity in saliva of children with severe early childhood caries and caries-free children. Caries Res. 2014;48:271–275.
- Aas JA, Griffen AL, Dardis SR, et al. Bacteria of dental caries in primary and permanent teeth in children and young adults. J Clin Microbiol. 2008;46:1407–1417.
- Van Houte J, Sansone C, Joshipura K, et al. Mutans streptococci and non-mutans streptococci acidogenic at low pH, and in vitro acidogenic potential of dental plaque in two different areas of the human dentition. J Dent Res. 1991;70:1503–1507.
- Sampaio-Maia B, Monteiro-Silva F. Acquisition and maturation of oral microbiome throughout childhood: an update. Dent Res J. 2014;11:291–301.
- Gross EL, Beall CJ, Kutsch S, et al. Beyond Streptococcus mutans: dental caries onset linked to multiple species by 16S rRNA community analysis. PLoS One. 2012;7(10):e47722. Available from: https://www.ncbi.nlm.nih.gov/pubmed/23091642
- Ahn SH, Song JE, Kim S, et al. NOX1/2 activation in human gingival fibroblasts by Fusobacterium nucleatum facilitates attachment of Porphyromonas gingivalis. Arch Microbiol. 2016;198(6):573–583.
- Kleniewska P, Hoffmann A, Pniewska E, et al. The influence of probiotic Lactobacillus casei in combination with prebiotic inulin on the antioxidant capacity of human plasma. Oxid Med Cell Longev. 2016;2016:1340903. Available from: https://www.hindawi.com/journals/omcl/2016/1340903/
- Hathout S, Mohamed SR, El-Nekeety AA, et al. Ability of Lactobacillus casei and Lactobacillus reuteri to protect against oxidative stress in rats fed aflatoxins-contaminated diet. Toxicon. 2011;58(2):179–186.
- Kapila S, Kapila R, Reddi S, et al. Oral administration of probiotic Lactobacillus casei spp. casei ameliorates oxidative stress in rats. Int J Curr Microbiol Appl Sci. 2014;3(9):670–684.