ABSTRACT
Objectives: To evaluate the association between nutritional status, resting energy expenditure (REE), and protein oxidative stress in patients after kidney transplantation (KT).
Methodology: The study evaluated 35 patients transplanted at the time of hospital discharge and 3 months after regarding: body composition, REE (by indirect calorimetry), and injury factor (IF); serum urea, creatinine, glucose, albumin, total protein, advanced oxidation protein products (AOPP), vitamin C.
Results: Three months after discharge, there was an improvement in renal function, nutritional status, and oxidative stress, with a standardization in the REE/kg. There was an increase in body weight, mainly in fat mass. The correlations showed that a greater cold ischemia time resulted in a deeper decline in vitamin C; a longer hospital length stay resulted in a greater reduction in AOPP; the higher preoperative body weight showed greater increases in body fat and glucose after transplantation. For decreases in REE and IF, there were increases in total protein. Finally, at hospital discharge there was a greater gain in weight, lower albumin, and total protein among individuals who had rejection episodes.
Discussion: The KT improves many of metabolic abnormalities, with the improvement of nutritional status, oxidative stress, and normalization of REE.
Introduction
The nutritional status is an important determinant in the clinical course of patients suffering from end-stage renal disease (ESRD). For patients who underwent kidney transplant (KT), it is common to observe alterations in the nutritional status between the following extremes: protein energy malnutrition (PEM) and overweight/obesity [Citation1]. About 25% of transplanted patients are currently estimated to be obese [Citation2], and the body mass index (BMI) increase before transplantation is associated with hypertension, hyperglycemia, and longer hospitalization periods [Citation3].
In general, patients present an increased body weight especially 3 months after transplant, with fat mass (FM) accumulation and maintenance or reduction in lean mass (LM). It is possible to observe an increase in central fat stores (visceral), whereas the peripheral fat stores are normal or increased [Citation4]. This weight gain, especially in body fat, induces changes in lipid metabolism, increasing the risk for diabetes mellitus (DM) and cardiovascular diseases (CVDs) [Citation5].
It is also known that ESRD patients have an imbalance in the redox state as kidney function deteriorates, resulting in oxidative stress (OS) and chronic inflammation, which can be partially reversed after KT [Citation6]. Individuals who underwent KT present a very characteristic OS frame in the post-transplant early stage; however, the oxidant state caused by the surgery is not yet fully characterized [Citation7]. Surgery itself induces oxidative stress during the ischemia–reperfusion period, which may affect the quality of the transplanted kidney and even shorten the graft life [Citation8].
The Advanced Oxidation Protein Products (AOPP) is a biomarker that is very sensitive to protein damage mediated by reactive-oxygen species. It can reflect the oxidative injury in a longer term, as opposed to the lipid oxidation markers [Citation9]. The reduction of the AOPP 1 day after the transplant can be explained by the blood loss during surgery, but its continuous reduction in subsequent moments, corresponding to an increase in antioxidants and a decrease in other oxidative parameters, may confirm that the success of the transplant and the antioxidant defense system can improve the elimination of these reactive species [Citation10].
Considering the situation of the metabolic stress imposed by the transplant, and the changes in the body composition expected for the post-transplant period, the changes in the resting energy expenditure (REE) have not been well established for these patients at different times after surgery. It is believed that the protein metabolism and high doses of immunosuppressant drugs may also interfere with the REE [Citation5].
Therefore, the aim of the present study is to evaluate the changes and to characterize the association between nutritional status, energy expenditure, and protein oxidative stress in patients at discharge after renal transplant and 3 months later.
Methods
Patients
This is a prospective longitudinal study with 35 patients who underwent KT. All subjects were evaluated at two different moments: at hospital discharge after transplant and 3 months later. The participants signed an informed consent and the project was approved by the Hospital’s Research Ethics Committee.
Patients of both genders were included, they were over 18 years old, had a functioning graft, and were transplanted from living donors or cadavers. Individuals with life expectancy of less than 6 months, previous graft loss, and multiple organ transplants were excluded.
In this selected group of 35 evaluated patients, the main causes for ESRD were arterial hypertension (22%), hypertension and diabetes (12%), and diabetes (3%). Other causes were autosomal dominant polycystic kidney disease (11%), glomerulonephritis (9%), Berger’s disease (6%), focal segmental glomerulosclerosis (3%), systemic lupus erythematosus (3%), and others (8%). In 22% of the cases, the cause was unknown.
All individuals were previously under dialysis, 28 of which were exclusively under hemodialysis (80%), 1 was under peritoneal dialysis (2.86%), and 6 had already been under both modalities (17.14%). The mean time under dialysis before transplant was 52.74 (±26.16 months).
As to the immunosuppression scheme, all patients received a pulse steroid induction (Methylprednisolone 500 mg) during surgery. From the second day on after the surgery, all patients were treated with the following prednisone schedule: 0.5 mg/kg/day, decreasing 5 mg in alternate days until they would reach the dose of 20 mg/day. This dose was maintained until the 30th day after transplant. Between the 31st and the 60th day, the dose of 15 mg/day was maintained. After the 61st day, the dose was reduced to 10 mg/day and after the 91st day to 5 mg/day and kept like this from this day on. Other immunosuppressive drugs used in this center are Tacrolimus at 0.2 mg/kg/day and Mycophenolate sodium at 1440 mg/day. Patients who present adverse effects use smaller doses of the immunosuppressive drugs mentioned.
Clinical data
During hospital admission for transplant, information on the length of hospital stay, cold ischemia time (CIT), the frequency of rejection episodes was gathered.
Biochemical tests used for clinical evolution follow-up were obtained from the patients’ records, such as serum urea, creatinine, glucose, albumin, and total proteins. In order to estimate the glomerular filtration rate (GFR), we used the Modification of Diet in Renal Disease Study equation [Citation11].
Oxidative stress evaluation
The analyses for the evaluation of the oxidative stress were as follows:
AOPP: determined by spectrophotometric microplate reader (SpectraMax model M3, Molecular Devices) [Citation12].
Serum Vitamin C (ascorbic acid): performed by colorimetric reaction and subsequent spectrophotometric reading (SpectraMax model M3, Molecular Devices) [Citation13].
Body composition evaluation
Anthropometric data were pre-transplant dry weight, weight and height at hospital discharge, and weight after 3 months. Weight and height data were used to calculate the BMI at the two moments evaluated.
Since in this Transplant Center, patients are only discharged when they absolutely do not need dialysis anymore and present a renal function which allows them to maintain fluid, electrolyte, and acid–base balance, the first bioelectric impedance evaluation was performed on the day of discharge, precisely because, in this period, the patient presents no edema and has stable weight and clinical conditions. The tetrapolar bioelectrical impedance method was used to evaluate fat mass (FM) and lean mass (LM), with the Bioimpedance Biodynamics model 450 portable device (Biodynamics Corp., Shoreline, WA, U.S.A).
Resting energy expenditure evaluation
All individuals underwent this test at discharge and 3 months later, when they returned to the outpatient clinic. They were all considered sedentary, with no differences in their routine physical activities. This test was always carried out in the morning, after at least an 8-hour fasting. The REE was obtained by indirect calorimetry (IC), with the MedGraphicsVO2000 portable equipment (MedGraphics Corp: St. Paul, Minnesota, U.S.A). By comparing the data on the volumes of inhaled oxygen and exhaled carbon dioxide per minute, and using the Weir Equation (1949) [Citation14], it was possible to estimate the REE for a day.
The result achieved by the IC was compared to the basal energy expenditure (BEE) calculated by the Harris–Benedict (HB) equation [Citation15], so as to estimate the injury factor (IF = REE/HB).
Statistical analysis
Mean and standard deviation of the quantitative variables were calculated using the SAS® 9.0 software (1999) [Citation16].
For the analysis of qualitative variables, the McNemar’s test was performed to verify the effect of time, using PROC FREQ [Citation16]. For cases in which responses were obtained with more than two levels, an extension of the same test was used.
Non-parametric tests were used for comparisons between the two evaluations in relation to the quantitative variables (the Wilcoxon test), and for comparisons between two independent groups (the Mann–Whitney test). The results were obtained with the R software [Citation17].
The difference in values between the two evaluated time periods was called ‘delta’ (Δ), and it was calculated for each individual for comparisons between average deltas. The delta of each quantitative variable was also used in the calculation of correlations using the Spearman correlation (r). The confidence intervals for the Spearman coefficient were obtained using the Fisher’s z transformation [Citation18]. The results were obtained using the PROC CORR [Citation16].
In all cases, the significance level was fixed at 5%.
Results
The sample consisted of 21 men (60%) and 14 women, with a mean age of 46.89 ± 12.7 years, ranging from 20 to 73 years old. A total of 4 out of all subjects studied received their kidneys from living donors (11.43%) and 31 received from cadaver donors (88.57%). The average length of hospital stay after transplantation was 30.29 ± 12.85 days and the cold ischemia time (CIT) average was 1381.71 ± 495.62 minutes. Among all patients, the percentage of diabetics was 14.3 at admission and this prevalence did not change after 3 months.
From hospital discharge until 3 months after transplant, all indicative parameters of renal function significantly improved, with a reduction in serum urea and creatinine and an average increase of 25% in the GFR (). There was no significant correlation between the renal function indicators and the length of hospital stay.
Table 1. Significant changes in biochemical analyses between the two evaluated periods.
The pre-transplant mean dry weight was 64.49 kg (±12.38) and the BMI was calculated for each patient using this weight. According to the BMI classification by age group, of the individuals evaluated, 2 (5.72%) presented undernutrition; 22 (62.86%) presented a healthy weight; 7 (20%) were overweight and 4 (11.42%) were obese.
Taking the entire group into account, a significant increase in the mean body weight between the two evaluations and also in the BMI was observed (). This weight gain was followed by an improvement in the biochemical parameters associated with the nutritional status. A significant increase in serum albumin, and total protein was also noted ().
Table 2. Significant changes in anthropometry and body composition between the two evaluated periods.
The evaluation of the protein oxidative stress used AOPP as a marker. A significant reduction in this parameter between the two evaluations was observed. In order to exclude expected albumin variation interferences in the results of oxidative stress, the ratio of AOPP and albumin was calculated at each study time, observing a significant decrease in this ratio after 3 months (). The vitamin C concentration mean at discharge was 30.61 (±16.82 µmol/l) and it did not change significantly after 3 months (36.85 ± 18.54 µmol/l).
When assessing changes in body composition of transplant patients at the time of hospital discharge and 3 months later, it was possible to note a considerable increase in lean mass (LM) in kilograms (kg). However, there was a further substantial increase in fat mass (FM) in kg and in percentage. In the analysis of the energy expenditure, a significant reduction of REE/kg of body weight was noted ().
During hospital stay, eight individuals (22.86%) presented transplanted kidney rejection and were treated with one of the immunosuppression protocols. For this group, there was a higher weight gain, and at discharge, the values for albumin and total proteins were lower when compared to those who did not suffer rejection ().
Table 3. Comparisons between groups with rejection (WR) and without rejection (WOR).
The individuals who presented some episode of rejection during hospital stay were discharged only when properly treated. This extended the length of their stay in comparison to the other patients. The mean length of hospital stay for the group that presented rejection was 43.38 (±16.24 days), whereas for the rest of the group, it was 26.41 (±8.75 days). Four out of the eight patients lost weight (mean of 3.28 ± 1.77 kg) and four gained weight (mean of 2.85 ± 3.87 kg) during hospital stay. Throughout 3 months, only three patients (8.57%) presented an episode of rejection and none of them lost weight since discharge.
The important correlations found between the admission data and the variables (Δ) of the parameters after 3 months were a negative correlation between CIT and the Δ Vitamin C, demonstrating that the lower the CIT during surgery, the higher was the increase in Vitamin C after 3 months; and a positive correlation between length of hospital stay and the Δ AOPP, showing that the longer the hospital stay, the lower was the decrease in the AOPP (; ).
Figure 1. Graphic presentation of the correlations between CIT and the Δ Vitamin C, and between the time of hospitalization and Δ AOPP.
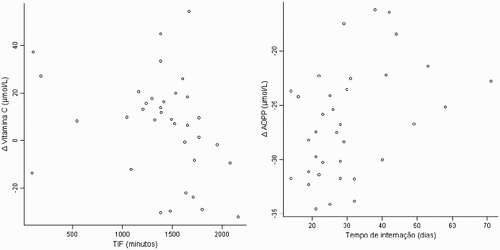
Table 4. Correlations between the studied variables.
In addition, it was possible to observe that the pre-transplant body weight influenced post-transplant outcomes. Positive correlations were also observed between both pre-transplant usual weight (kg) and Δ FM (kg), and Δ glucose after transplant (). It is also possible to observe correlations between deltas of some variables. Significant negative associations were found between Δ total protein and Δ REE, as well as between Δ total protein and Δ IF, demonstrating that the higher the mean total protein, the lower the REE and the IF ().
Discussion
This study found that, after KT, the gradual recovery of the renal function was followed by the improvement of the nutritional status and the oxidative stress, along with a reduction in REE and IF after transplant. As to body composition, an increase in weight was observed, particularly in FM. After transplant, patients are discharged only after they present a renal function that allows them to maintain fluid and electrolyte balance, are edema free, and have a stable body weight and clinical condition. However, the renal function continues to improve after discharge, attaining stability usually 3 months after transplantation.
Post-transplant weight gain, especially over the first year, is well known. Rapid and excessive weight gain contributes to the development of CVDs and other comorbidities, which then lead to less favorable outcomes for renal grafts [Citation19]. In this study, most patients had an increase in body weight over the 3 months evaluated, equivalent to about 1 kg per month, similar to the literature [Citation19].
It is known that the body composition also changes considerably after KT. The increase in food ingestion, combined with corticosteroids therapy [Citation20] and sedentary lifestyles, can lead to FM accumulation, changes in the distribution of body fat, and muscle loss following transplantation [Citation21]. In this study, it was observed that 3 months after discharge, there was a slight increase in muscle mass, but a greater increase in FM, similar to other studies in this population [Citation22,Citation23].
These changes were expected with the restoration of renal function. The GFR is positively related to FM, fat-free mass, and LM, suggesting that the graft function predicts the nutritional status of kidney transplant patients [Citation20]. It was also found in this study that the variation of post-transplant FM was positively and significantly associated with pre-transplant body weight, indicating that the patients with higher previous body weight showed a greater increase in total body fat (kg) at the end of the study.
ESRD creates a hypercatabolic state which is usually recovered in the first year after KT [Citation24]. The recovery of renal function observed immediately after a successful transplant is followed by a general improvement in nutritional status [Citation25]. In this study, the mean increase in serum albumin and total protein after 3 months showed a considerable improvement in the nutritional status, as previously demonstrated in another study [Citation26], probably due to an increase in appetite, an improved renal function, and a correction in anemia.
Acute rejection of the renal graft is another common concern in recent and late kidney transplant patients. In this study, about 23% of the patients presented some rejection during hospitalization for transplant and, in this group, an increased weight gain was also observed, probably due to high doses of steroids used to treat the rejection [Citation27]. It was also noted that individuals who had no rejection episode during hospitalization presented a better nutritional status. It is known that glucocorticoids induce the production of positive acute phase proteins, with a consequent decrease in negative acute phase proteins (such as albumin) [Citation28]. Moreover, the pulse steroid therapy stimulates liver gluconeogenesis, which promotes a protein catabolism for the synthesis of glucose [Citation29].
Another point of interest is the additional energy expenditure attributed to the kidney transplant procedure and its post-transplant phases. It is known that in healthy individuals, kidneys contribute with about 7% of REE. The progression of renal failure is associated with metabolic disorders, such as metabolic acidosis, insulin resistance, and inflammation, thus increasing protein catabolism and energy expenditure [Citation30]. In this study, there was an important reduction in REE after 3 months, when it was adjusted by the body weight. The mean values of REE obtained here were very similar to those estimated in healthy controls [Citation31] and measured in transplanted patients [Citation32].
In the early stages of post-kidney transplant, both post-surgical stress and the increased doses of immunosuppressive drugs can lead to protein catabolism and an increase in energy expenditure [Citation33]. In this study, the reduction in REE and, therefore, in the IF, was followed by improvements in serum concentrations of total proteins. Thus, the recovery of the kidney function and the normalization of REE may help in the improvement of the nutritional status. Consequently, patients with a higher REE are apparently more inflamed and malnourished [Citation34].
The restoration of renal function after transplant reduces OS, contributing to a better survival rate of these patients [Citation9]. The transplant restores the process of glomerular filtration, thus eliminating toxic solutes that contribute to the OS [Citation35]. Our results show a significant reduction in AOPP between the two evaluations. Several studies indicate that dialysis alone promotes repeated inflammations and therefore promotes an increased production of oxidants [Citation9]. Consequently, eliminating the dialysis would be a strong determinant for the reduction in OS markers.
The serum concentration of AOPP may be sensitive to assess OS after KT. In one study, their levels decreased significantly 1 year after transplantation, in comparison to before surgery [Citation36]. In another study, comparing the AOPP values between transplanted and healthy subjects, it was observed that patients who underwent transplant had a significantly higher level of AOPP before surgery compared to controls and that transplanted patients showed AOPP levels persistently higher than the controls at all times [Citation37]. Individuals who were hospitalized for a shorter period presented a greater reduction in AOPP levels, possibly due to a faster improvement in renal function, in the inflammatory process, and in OS.
Another important factor in organ transplantation which is related to the patients’ prognosis is the cold ischemia time (CIT). The prolonged CIT is a significant risk factor for early graft dysfunction and loss [Citation38]. The severity of tissue injury influences the levels of ischemia/reperfusion, subsequent graft dysfunction, and probably an increase in acute rejection rates in kidney transplant patients [Citation39]. The correlation between Vitamin C and CIT found in this study, despite being weak, but statistically significant, can suggest that Vitamin C has a higher absorption rate in situations in which the CIT is longer, when the formation of free radicals is usually greater.
Mainly due to the restoration of the renal function, it is possible to conclude that the KT improves some metabolic abnormalities, improving the nutritional status and the OS, and also normalizing the REE. However, new metabolic abnormalities are introduced due to the use of immunosuppressive therapy and new eating habits, demanding a permanent nutritional follow-up for this group.
Disclosure statement
No potential conflict of interest was reported by the authors.
Notes on contributors
Larissa Vieira Marino obtained bachelor’s degree in nutrition and metabolism and master’s degree in clinic nutrition at Department of Internal Medicine, Ribeirao Preto Medical School, University of Sao Paulo, Ribeirao Preto, Brazil.
Elen Almeida Romão is a professor doctor at the Department of Internal Medicine, Division of Nephrology, Ribeirao Preto Medical School, University of Sao Paulo, Ribeirao Preto, Brazil.
Paula Garcia Chiarello is a professor doctor in clinic nutrition at the Department of Internal Medicine, Ribeirao Preto Medical School, University of Sao Paulo, Ribeirao Preto, Brazil.
ORCID
Larissa Vieira Marino http://orcid.org/0000-0002-0137-2864
Additional information
Funding
References
- Moreira TR. Alterações nutricionais em transplantados renais: prevalência, fatores de risco e complicações. 2010. 131f. Tese (Mestrado em Ciências Médicas) – Faculdade de Medicina, Universidade Federal do Rio Grande do Sul, Porto Alegre, RS; 2010.
- Ditonno P, Lucarelli G, Impedovo SV, et al. Obesity in kidney transplantation affects renal function but not graft and patient survival. Transplant Proc. 2011;43(1):367–372. doi: 10.1016/j.transproceed.2010.12.022
- Abou-Jaoude MM, Nawfal N, Najm R, et al. Effect of pretransplantation body mass index on allograft function and patient survival after renal transplantation. Transplant Proc. 2010;42(3):785–788. doi: 10.1016/j.transproceed.2010.03.026
- Iş I, Akin O, Demirağ A, et al. Effects of renal transplantation on body composition. Transplant Proc. 1998;30(3):831–832. doi: 10.1016/S0041-1345(98)00067-0
- Martins C, Furukawa L. Nutrição e transplante renal. In: Riella MC, Martins C, editors. Nutrição e o Rim. 1st ed. Rio de Janeiro: Guanabara Koogan; 2001. p. 149–161.
- Aveles PR, Criminácio CR, Gonçalves S, et al. Association between biomarkers of carbonyl stress with increased systemic inflammatory response in different stages of chronic kidney disease and after renal transplantation. Nephron Clin Pract. 2010;116(4):c294–c299. doi: 10.1159/000318792
- Zahmatkesh M, Kadhodaee M, Mahdavi-Mazdeh M, et al. Oxidative stress status in renal transplant recipients. Exp Clin Tranplant. 2010;8(1):38–44.
- Ha H, Park J, Kim YS, et al. Oxidative stress and chronic allograft nephropathy. Yonsei Med J. 2004;45(6):1049–1052. doi: 10.3349/ymj.2004.45.6.1049
- Simmons EM, Langone A, Sezer MT, et al. Effect of renal transplantation on biomarkers of inflammation and oxidative stress in end-stage renal disease patients. Transplantation. 2005;79(8):914–919. doi: 10.1097/01.TP.0000157773.96534.29
- Vostálová J, Galandáková A, Svobodová AR, et al. Time-course evaluation of oxidative stress-related biomarkers after renal transplantation. Renal Fail. 2012;34(4):413–419. doi: 10.3109/0886022X.2011.649658
- Levey AS, Greene T, Kusek JW, et al. A simplified equation to predict glomerular filtration rate from serum creatinine (abstract). J Am Soc Nephrol. 2000;11:155A.
- Valli A, Suliman ME, Meert N, et al. Overestimation of advanced oxidation protein products in uremic plasma due to presence of triglycerides and other endogenous factors. Clin Chim Acta. 2007;379(1–2):87–94. doi: 10.1016/j.cca.2006.12.026
- Bessey OA. Ascorbic acid: microchemical methods. In: Vitamin methods. New York (NY): Academic Press; 1960. p. 303.
- Weir JB. New methods for calculating metabolic rate with special reference to protein metabolism. J Physiol. 1949;109(1–2):1–9. doi: 10.1113/jphysiol.1949.sp004363
- Harris JA, Benedict FG. A biometric study of human basal metabolism. Proc Natl Acad Sci USA. 1918;4(12):370–373. doi: 10.1073/pnas.4.12.370
- SAS Institute Inc. SAS/STAT® user’s guide. Version 9.0. Cary (NC): SAS Institute Inc; 1999.
- R Core Team. R: A language and environment for statistical computing. R Foundation for Statistical Computing, Vienna, Austria; 2013. Available from: http://www.Rproject.org/.
- Fisher RA. Statistical methods for research workers. 14th ed. Davien (CT): Hafner Publishing Company; 1970.
- Cashion A, Stanfill A, Thomas F, et al. Expression levels of obesity-related genes are associated with weight change in kidney transplant recipients. PLoS One. 2013;8(3):e59962. doi: 10.1371/journal.pone.0059962
- Tutal E, Sezer S, Uyar ME, et al. Evaluation of nutritional status in renal transplant recipients in accordance with changes in graft function. Transplant Proc. 2013;45(4):1418–1422. doi: 10.1016/j.transproceed.2013.01.104
- Mantoo S, Abranham G, Pratap GB, et al. Nutritional status in renal transplant recipients. Saudi J Kidney Dis Transpl. 2007;18(3):382–386.
- van den Ham EC, Kooman JP, Christiaans MH, et al. Relation between steroid dose, body composition and physical activity in renal transplant patients. Transplantation. 2000;69(8):1591–1598. doi: 10.1097/00007890-200004270-00013
- Habedank D, Kung T, Karhausen T, et al. Exercise capacity and body composition in living-donor renal transplant recipients over time. Nephol Dial Transplant. 2009;24(12):3854–3860. doi: 10.1093/ndt/gfp433
- Netto MCAS, Alves-Filho G, Mazzali M. Nutritional status and body composition in patients early after renal transplantation. Transplant Proc. 2012;44(8):2366–2368. doi: 10.1016/j.transproceed.2012.07.041
- Martins C, Pecoits-Filho R, Riella MC. Nutrition for the post–renal transplant recipients. Transplant Proc. 2004;36(6):1650–1654. doi: 10.1016/j.transproceed.2004.06.065
- du Plessis AS, Randall H, Escreet E, et al. Nutritional status of renal transplant patients. S Afr Med J. 2002;92(1):68–74.
- Rogers CC, Alloway RR, Buell JF, et al. Body weight alterations under early corticosteroid withdrawal and chronic corticosteroid therapy with modern immunosuppression. Transplantation. 2005;80(1):26–33. doi: 10.1097/01.TP.0000164290.17030.BC
- Kasiske BL, Adeva-Andany M. Manejo nutricional do transplante renal. In: Koople, JD, Massry SG, editors. Cuidados Nutricionais das Doenças Renais. 2nd ed. Rio de Janeiro: Guanabara Koogan; 2006. p. 464–475.
- Goral S, Bleicher MB. Nutritional requirements of kidney transplant patients. In: Mitch WE, Ikizler TA, editors. Handbook of nutrition and the kidney. 6th ed. Philadelphia (PA): Lippincott Williams & Wilkins; 2010. p. 208–223.
- Avesani CM, Draibe SA, Kamimura MA, et al. Decreased resting energy expenditure in non-dialysed chronic kidney disease patients. Nephol Dial Transplant. 2004;19(12):3091–3097. doi: 10.1093/ndt/gfh547
- Plauth M, Schütz T, Buckendahl DP, et al. Weight gain after transjugular intrahepatic portosystemic shunt is associated with improvement in body composition in malnourished patients with cirrhosis and hypermetabolism. J Hepatol. 2004;40(2):228–233. doi: 10.1016/j.jhep.2003.10.011
- Schütz T, Hudjetz H, Roske AE, et al. Weight gain in long-term survivors of kidney or liver transplantation – another paradigm of sarcopenic obesity? Nutrition. 2012;28(4):378–383. doi: 10.1016/j.nut.2011.07.019
- Saxena A, Sharma RK. Nutritional Surviellance after renal transplant: review. Indian J Transplant. 2009;3:5–12. doi: 10.1016/S2212-0017(11)60087-7
- Wang AY, Sea MM, Tang N, et al. Resting energy expenditure and subsequent mortality risk in peritoneal dialysis patients. J Am Soc Nephrol. 2004;15(12):3134–3143. doi: 10.1097/01.ASN.0000144206.29951.B2
- Antolini F, Valente F, Ricciardi D, et al. Normalization of oxidative stress parameters after kidney transplant is secondary to full recovery of renal function. Clin Nephrol. 2004;62(2):131–137. doi: 10.5414/CNP62131
- Zadrazil J, Horak P, Strebl P, et al. In vivo oxidized low-density lipoprotein (ox-LDL) aopp and tas after kidney transplantation: a prospective, randomized one year study comparing cyclosporine A and tacrolimus based regiments. Biomed Pap Med Fac Univ Palacky Olomouc Czech Repub. 2012;156(1):14–20. doi: 10.5507/bp.2012.008
- de cal M, Silva S, Cruz D, et al. Oxidative stress and ‘monocyte reprogramming’ after kidney transplant: a longitudinal study. Blood Purification. 2008;26(1):105–110. doi: 10.1159/000110575
- Salahudeen AK, Haider N, May W. Cold ischemia and the reduced long-term survival of cadaveric renal allografts. Kidney Int. 2004;65(2):713–718. doi: 10.1111/j.1523-1755.2004.00416.x
- van der Vliet JA, Warlé MC. The need to reduce cold ischemia time in kidney transplantation. Curr Opin Organ Transplant. 2013;18(2):174–178. doi: 10.1097/MOT.0b013e32835e2a08