ABSTRACT
Objectives: Hepatocellular carcinoma (HCC) is characterized by elevated in oxidative stress and inflammatory cytokines, which enhance destructive effects of the tumor. Therefore, we conducted this study to investigate the protective effects of sodium ascorbate against thioacetamide-induced HCC in rats through studying its effect on the apoptotic pathway in rats. In addition, in vitro activity of sodium ascorbate was investigated on HepG2 and compared with cisplatin.
Methods: HCC was experimentally induced by injecting rats with 200 mg/kg thioacetamide intraperitoneally twice weekly for 16 weeks. Part of HCC rats was concomitantly treated with 100 mg/kg sodium ascorbate intraperitoneally during the 16-week period. Hepatic tissues were used for the determination of NFκB, Nrf2, TNF-α, caspase-3, caspase-8 and caspase-9.
Results: Sodium ascorbate significantly attenuated HCC-induced reduction in the expression of NrF2 associated with a reduction in concentrations of hydrogen peroxide and superoxide anion. In addition, sodium ascorbate blocked HCC-induced increase in the expression of NFκB and TNF-α. Sodium ascorbate slightly increased the activity of caspase-3, -8 and -9 in vitro but inhibited their activities in vivo.
Conclusion: In spite of the antioxidant and anti-inflammatory activity of sodium ascorbate, it produced selective cytotoxic activity via direct activation of the apoptotic pathway in cancer cells without affecting the apoptotic pathway in normal hepatic cells.
Highlights
Repeated exposure to thioacetamide results in HCC in rats.
Sodium ascorbate is a strong water-soluble antioxidant with cytotoxic activity.
Sodium ascorbate activates extrinsic and intrinsic apoptotic pathway in tumor cells.
It protects normal hepatic cells by antioxidant, anti-inflammatory and anti-apoptotic effects.
1. Introduction
The incidence of hepatocellular carcinoma (HCC) has significantly elevated worldwide (for review [Citation1]). The expression of both nuclear factors and inflammatory cytokines plays a major role in the incidence of HCC [Citation2–4]. However, the inflammatory microenvironment is the major cause of intrinsic heterogeneity of cancer cells and genomic instability [Citation5]. In addition, extracellular matrix (ECM) is formed of many heterogeneous molecules. It serves as a store for nuclear factors, inflammatory cells, angiogenesis promoters and many biologically active molecules [Citation6]. The release of all these macromolecules activates these pathways leading to pathogenesis and progression of HCC through controlling cells proliferation and differentiation leading to tumor cells invasion and metastasis [Citation7]. HCC has been linked with a high rate of mortality [Citation8]. However, more investigations are needed to find out new therapeutic drugs for the treatment of HCC patients.
Ascorbic acid is considered a strong water-soluble antioxidant vitamin, which was reported recently to produce a highly selective cytotoxic activity against many tumors without affecting normal cells [Citation9]. This selective effect could be explained by several mechanisms such as reduction of the mitochondrial sensitivity to hydrogen peroxide [Citation10], inhibition of catalase and superoxide dismutase activity [Citation11], enhancement of glycolytic metabolism and blockage of the activity of MMP9 [Citation12]. It can propagate cancer cell apoptosis without introducing any changes in cellular redox status in HepG2 cells [Citation13] accompanied by influencing cell proliferation [Citation14].
Recently, we found that sodium ascorbate produced cytotoxic activity through blocking sulfatase-2 and restoring heparan sulfate proteoglycans (HSPGs) associated with reducing glypican-3/IGF-2 and deactivation of the inflammatory pathway [Citation15]. However, there is no available information about the effect of sodium ascorbate on the apoptotic pathway in HCC. Therefore, we aimed to discover the selective cytotoxic activity of sodium ascorbate and its relation to the inflammatory pathway in experimentally induced HCC in rats and in HepG2 cells.
2. Materials and methods
2.1. Animals
The local ethical committee in the University of Tabuk approved the animal protocol under number UT-42-3-2018. Thirty-two male Sprague–Dawely rats were kept under standard conditions and regular 12 h light/12 h dark cycle. Rats were classified into four groups with eight rats in each group.
2.1.1. Control group
Rats were injected with phosphate-buffered saline (PBS, 10 mM, pH 7.4) intraperitoneally (ip).
2.1.2. Ascorbate-treated control group
Rats were treated with 100 mg/kg sodium ascorbate (Sigma Aldrich Chemicals Co., St Louise, MO, USA) twice weekly for 16 weeks via ip injection.
2.1.3. HCC group
Rats received 200 mg/kg thioacetamide (Tocris, Bristol, UK), ip, twice weekly for 16 weeks.
2.1.4. Ascorbate-treated HCC group
Rats were given both 200 mg/kg thioacetamide, ip, and 100 mg/kg sodium ascorbate, ip, twice weekly for 16 weeks.
The doses and time course were in consistent with previous studies [Citation8,Citation12,Citation15–20].
2.2. Morphologic analysis of hepatic tissue
Fixed liver sections were cut into 5 µm thickness sections. The sections were stained with Mayer’s hematoxylin and eosin (H&E). Sections were examined in a masked manner and photographed using Nikon Digital Camera (Japan).
2.3. Assessment of oxidative stress
Oxidative stress was assessed by determining the concentration of hydrogen peroxide by horseradish peroxidase method [Citation21]. Moreover, the concentration of superoxide anion concentration was analyzed by nitroblue tetrazolium method [Citation22].
2.4. Enzyme-linked immunosorbent assay
The levels of biochemical parameters were determined by Enzyme-linked immunosorbent (ELISA) assay using a commercially available TNF-α (eBioscience Inc., San Diego, CA, USA) and α-fetoprotein (USCN Life Science Inc., Houston, TX, USA).
2.5. Quantitative real-time polymerase chain reaction
The PCR analysis was performed as described previously by our group [Citation15]. In HepG2 cells, the sequence of NFκB forward primer 5′-ACCCTGACCTTGCCTATTTG-3′ and reverse primer 5′-GAAAAGCTGTAAACATGAGCCG-3′, for Nrf2 forward primer 5′-CAGCGACGGAAAGAGTATGA-3′ and reverse primer 5′-TGGGCAACCTGGGAGTAG-3′ and for GAPDH the forward primer 5′-AGAAGGCTGGGGCTCATTTG-3′ and reverse primer 5′-GAAGCTTCTGTTGGCTCCC-3′. In rats, for Nrf2, the forward primer 5′-GAGACGGCCATGACTGAT-3′ and reverse primer, 5′-GTGAGGGGATCGATGAGTAA-3′, for NFκB, the forward primer, 5′- GGAAGTGAGAGAGTGAGCGAGAG -3′ and reverse primer 5′-GGGTGGCGAAACCTCCTC -3′; for caspase-3, forward primer 5′-GATCACAGCAAAAGGAGCAGT-3′ and reverse primer 3′-CTCCACTGTCTGTCTCAAT-5′, caspase-8: forward primer 5′-CTGGGAAGGATCGACGATTA-3′ and reverse primer 5′-TGGTCACCTCATCCAAAACA-3′, for caspase-9 forward primer 5′-GAATTCCAGCAATCCGCTAGCCATGGAGG-3′ and reverse primer 5′-GAATTCAACTCATGAAGTTTAAAGAACAG-3′ and for GAPDH, the forward primer 5′- CCATCAACGACCCCTTCATT-3′ and reverse primer 5′- CACGACATACTCAGCACCAGC-3′.
2.6. Estimation of caspases activity
The enzyme activities of caspase-3, -8 and -9 was measured using commercially available kits (Abcam, Cambridge, MA, USA).
2.7. Cell lines
The human HCC, HepG2 (American Type Culture Collection, ATCC, Manassas, VA, USA).
2.7.1. Cell lysates
HepG2 cells were extracted by 50 mM Tris–HCl lysis buffer, pH 7.4, supplemented with 0.5% Triton-X 100, 150 mM NaCl and protease inhibitor cocktail. Protein level in HepG2 cells was determined (Bradford reagent, BioRad, Hercules, CA, USA).
2.7.2. MTT assay
A 96-well plate containing HepG2 cells (1 × 104) were incubated in a humidified CO2 incubator at 37°C for 24 h. Cells were treated with both sodium ascorbate and cisplatin (50, 100 and 250 µM) and kept in humidified CO2 incubator for 48 h. The viability was assessed using MTT (Sigma Aldrich Chemicals Co., St Louise, MO, USA) as described previously [Citation12,Citation16,Citation23,Citation24]. While, time course and doses of sodium ascorbate and cisplatin were in the range of previous studies [Citation12,Citation25,Citation26].
2.7.3. Cytotoxicity with lactate dehydrogenase
Cell cytotoxicity was performed using lactate dehydrogenase reagent as described by our group [Citation15,Citation27]. Triton-X 100 was used as a positive cytotoxicity control.
2.8. Statistical analysis
For quantitative variables, the mean ± standard error was used. The normality of distribution of samples were determined by the Kolmogorov–Smirnov test. One-way ANOVA was used for comparison between the groups followed by the post hoc Bonferroni correction test was calculated. SPSS version 20 (Chicago, IL, USA) was used. Statistical significance was predefined as p ≤ .05.
3. Results
3.1. Effect of sodium ascorbate on HCC-induced oxidative stress
Treating HCC rats with sodium ascorbate significantly reversed HCC-induced reduction in the gene expression of Nrf2 without affecting the control group. Moreover, treatment of HepG2 cells with sodium ascorbate resulted in elevated gene expression of Nrf2. However, treating HepG2 cells with cisplatin significantly reduced the gene expression of Nrf2 ().
Figure 1. Effect of 100 mg/kg sodium ascorbate on hepatic gene expression of Nrf2 (A). Effect of 50, 100 and 250 μM sodium ascorbate and cisplatin on the gene expression of Nrf2 (B) in HepG2. *Significant difference when compared with the control group of HepG2 cells at p < .05. #Significant difference when compared with the HCC group or HepG2 cells treated with cisplatin at p < .05.
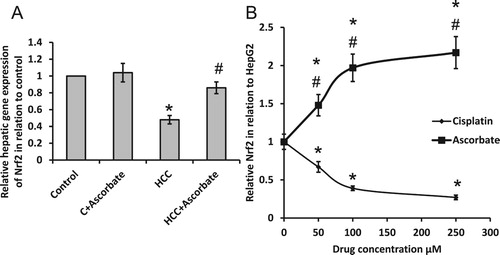
As shown in , we found that sodium ascorbate significantly reduced HCC-induced elevation in both hydrogen peroxide and superoxide anion when compared with the HCC group. However, treatment of HepG2 cell with ascorbate significantly reduced hydrogen peroxide and superoxide anion, while treating HepG2 cells with cisplatin significantly elevated both superoxide anion and hydrogen peroxide.
Figure 2. Effect of 100 mg/kg sodium ascorbate on hepatic hydrogen peroxide (A) and hepatic superoxide anion (C) in vivo. Effect of 50, 100 and 250 μM sodium ascorbate and cisplatin on the concentration of hydrogen peroxide (B) and superoxide anion (D) in HepG2. * Significant difference when compared with the control group or HepG2 cells at p < .05. #Significant difference when compared with the HCC group or HepG2 cells treated with cisplatin at p < .05.
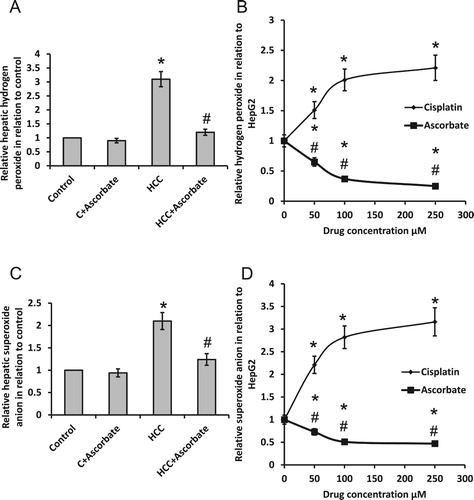
3.2. Sodium ascorbate attenuated HCC-induced activation of inflammatory pathway
Treatment of HCC rats with sodium ascorbate produced a significant reduction in gene expression of NFκB as well as protein expression of TNF-α; however, the levels are still higher than the control group. In parallel, a high dose of sodium ascorbate reduced NFκB gene expression associated with dose-dependent reduction in protein release of TNF-α in HepG2 cells. However, the addition of cisplatin to HepG2 cells caused a dose-dependent elevation in gene expression of NFκB and protein release of TNF-α ().
Figure 3. Effect of 100 mg/kg sodium ascorbate on relative hepatic gene expression of NFκB (A) and TNF-α level (C) in vivo. Effect of 50, 100 and 250 μM sodium ascorbate and cisplatin on the gene expression of NFκB (B) and TNF-α level (D) in HepG2. *Significant difference when compared with the control group or HepG2 cells at p < .05. #Significant difference when compared with the HCC group or HepG2 cells treated with cisplatin at p < .05.
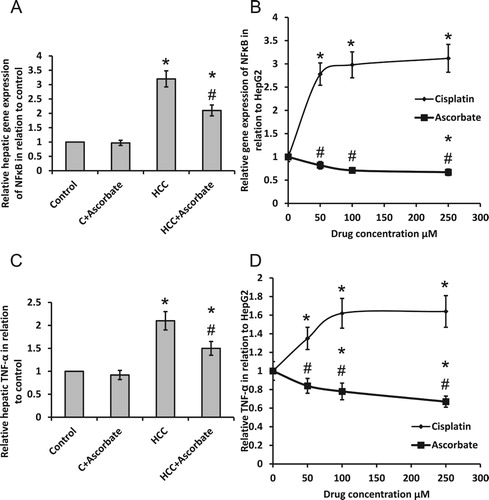
3.3. Effect of sodium ascorbate on HCC-induced elevation in caspases in vivo
As shown in , sodium ascorbate significantly reduced gene expression and enzyme activity of caspase-3, -8 and -9 in the HCC group without affecting the control group. However, sodium ascorbate reduced gene expression and enzyme activity of caspase-9 to levels of control rats.
Figure 4. Effect of 100 mg/kg sodium ascorbate on hepatic gene expression of caspase-3 (A), caspase-8 (C) and caspase-9 (E) in vivo as well as the hepatic enzyme activity of caspase-3 (B), caspase-8 (D) and caspase-9 (F). *Significant difference when compared with the control group at p < .05. # Significant difference when compared with the HCC group at p < .05.
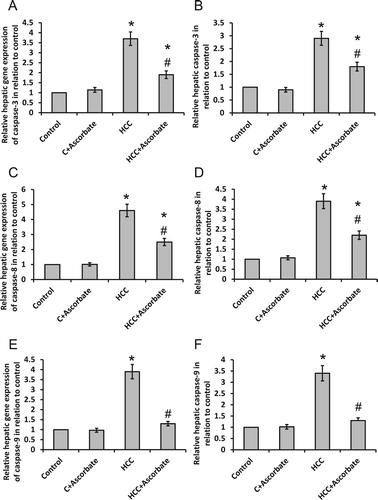
3.4. Effect of sodium ascorbate on apoptosis in HepG2 cells
Treatment of HepG2 cells with sodium ascorbate produced a dose-dependent slight increase in activities of caspase-3, -8 and -9 (). Moreover, cisplatin produced significantly higher effects on the activity of the three caspases in HepG2 cells when compared with sodium ascorbate with the same dose.
3.5. In vivo antitumor activity of sodium ascorbate
Sodium ascorbate significantly reduced the average number of nodules in the liver when compared with HCC rats as well as it reduced serum level of α-fetoprotein but still higher than the control group ((A and B)).
Figure 6. Effect of 100 mg/kg sodium ascorbate on average number of nodules (A) and serum level of α-fetoprotein (B) in vivo. In addition, hepatic sections stained with hematoxylin/eosin in different rat groups (C). Thick black arrow represented ground glass hepatocytes characterized by homogenous pink or vacuolated cytoplasm. Thin black arrows represented apoptotic nuclei. Yellow arrow represented necrotic hepatocytes at the periphery of the nodules. Scale bar 100 µm. *Significant difference when compared with the control group at p < 0.05. #Significant difference when compared with the HCC group at p < .05.
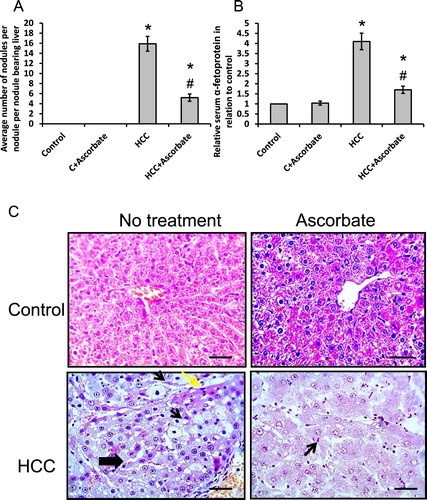
3.6. Hepatoprotective effects of sodium ascorbate
Examination of hepatic sections of HCC rats stained with hematoxylin/eosin showed marked inflammatory cells infiltration, apoptotic changes and vacuolations of hepatocytes. The hepatocytes on the periphery of the nodules are necrotic (yellow arrows). All these changes were significantly ameliorated by sodium ascorbate ((C)).
3.7. Cytotoxic studies of sodium ascorbate
We found that sodium ascorbate decreased HepG2 survival and enhanced HepG2 cytotoxicity in a dose-dependent manner. Cisplatin produced similar effects but significantly higher than sodium ascorbate ().
4. Discussion
Ascorbic acid is required for the control and maintenance of many cellular functions. Many previous reports illustrated the importance of sodium ascorbate in cancer treatment due to its ability to affect cell proliferation associated with its ability to enhance well-being state of patients [Citation14].
Oxidative stress is a key player in both the development and progression of HCC. Oxidative stress was reported to affect cell proliferation, apoptosis and cell cycle [Citation12,Citation28]. Oxidative stress activates mitogen-activated protein kinases with subsequent effect on the regulation of cell growth and transformation [Citation29]. Oxidative stress influences the formation of ECM and cell-to-cell interaction [Citation30]. However, we found that treatment of HCC rats as well as HepG2 cells with sodium ascorbate attenuated elevated levels of oxidative stress in a dose-dependent manner. However, ascorbate was previously reported to induce cell apoptosis without changes in cellular redox status in HepG2 [Citation13].
Interestingly, reactive oxygen species are activators of autophagy and other stress response systems such as nuclear factor (erythroid-derived 2)-like 2 (Nrf2) [Citation31]. Accumulation of Nrf2 in cytoplasm enhances nuclear migration and activates transcription of antioxidant and detoxification compounds that promote cellular response to oxidative stress [Citation32]. We found that sodium ascorbate reversed HCC-induced reduction in gene expression of Nrf2. However, no previous study illustrated the effect of sodium ascorbate on gene expression of Nrf2 in HCC models.
HCC is an inflammation-based carcinogenic process as over than 90% of HCC patients developed chronic liver damage [Citation33,Citation34]. NFκB mediates pro-inflammatory cytokines and is linked with many types of cancer, including HCC [Citation30,Citation35]. It induces the transcription of pro-inflammatory cytokines [Citation36,Citation37]. In addition, many inflammatory cytokines, such as TNF-α, IL-1α, IL-1β, IL-6 and IL-8, have been associated with chronic liver inflammation [Citation38,Citation39]. One of these inflammatory cytokines, TNF-α, is considered as an established marker of DNA damage [Citation40]. However, we found that treatment of HCC rats and HepG2 with sodium ascorbate reduced gene expression of NFκB associated with a reduction in protein levels of TNF-α. On the other hand, cisplatin elevated pro-inflammation cytokines in vitro, because it is well-known inflammation inducer [Citation41,Citation42].
We observed significant elevations in hepatic activities of caspase-3, -8 and -9 in HCC rats, which was inhibited by sodium ascorbate. Caspase-8 is well known in activating extrinsic cell death by activating transmembrane receptor interactions. The extrinsic apoptosis pathway can be initiated by the binding of specific ligands such as TNF-α [Citation43]. When activated, caspase-8 will be released inside the cytoplasm and initiates the cleavage of caspase-3 [Citation44]. However, we discovered a significant elevation in TNF-α associated with elevated caspase-8, in HCC rats. However, caspase-9 is a major part in the intrinsic cell death pathway [Citation45]. In response to mitochondrial stress, caspase-9 is released and activated producing to various pathological conditions with subsequent activation of caspase-3 [Citation46].
We observed an elevated superoxide anion and hydrogen peroxide levels that were associated with high caspase-9 leading to enhancement of the intrinsic cell death pathway. All these effects were attenuated by sodium ascorbate. These findings strongly suggest that sodium ascorbate inhibits oxidant-induced apoptotic cell death in hepatic cells. In addition, sodium ascorbate reduced inflammatory pathway with a subsequent reduction in the activity of caspase-8. Therefore, sodium ascorbate ameliorated the extrinsic apoptotic pathways in vivo. On the other hand, treating HepG2 cells with sodium ascorbate significantly elevated the three illustrating the ability of sodium ascorbate to enhance the apoptotic pathway in tumor cells without affecting normal cells.
Finally, we investigated the cytotoxic effects of sodium ascorbate and cisplatin on HepG2 cell lines by assessing their effect on cell viability and cytotoxicity. The two compounds produced almost similar results, but cisplatin was superior. Sodium ascorbate was previously reported to possess cytotoxic activity against HepG2 [Citation14,Citation47,Citation48]. We have also previously reported the cytotoxic activity of sodium ascorbate both in vivo and in vitro by restoring normal concentration of HSPGs [Citation12,Citation15]. In addition, sodium ascorbate showed hepatoprotective effects, which were demonstrated by its ability to attenuate HCC-induced inflammatory cells infiltration, apoptotic changes and vacuolations of hepatocytes in hepatic sections stained with hematoxylin/eosin.
5. Conclusion
Sodium ascorbate possesses cytotoxic activity both in vivo and in vitro. These effects can be partially explained by direct activation of both extrinsic and intrinsic apoptotic pathway in tumor cells independent on its antioxidant and anti-inflammatory activities. However, in normal cells, sodium ascorbate produced protective effects in normal hepatic cells by producing antioxidant, anti-inflammatory and anti-apoptotic effects ().
Acknowledgements
Research Ethics Committee in the University of Tabuk approved the animal protocol (UT-42-3-2018).
Disclosure statement
No potential conflict of interest was reported by the author(s).
References
- Stefaniuk P, Cianciara J, Wiercinska-Drapalo A. Present and future possibilities for early diagnosis of hepatocellular carcinoma. World J Gastroenterol. 2010;16(4):418–424. doi: 10.3748/wjg.v16.i4.418
- Tseng CS, Tang KS, Lo HW, et al. UDP-glucuronosyltransferase 1A7 genetic polymorphisms are associated with hepatocellular carcinoma risk and onset age. Am J Gastroenterol. 2005;100(8):1758–1763. doi: 10.1111/j.1572-0241.2005.41857.x
- Sergio A, Cristofori C, Cardin R, et al. Transcatheter arterial chemoembolization (TACE) in hepatocellular carcinoma (HCC): the role of angiogenesis and invasiveness. Am J Gastroenterol. 2008;103(4):914–921. doi: 10.1111/j.1572-0241.2007.01712.x
- Metwaly HA, Al-Gayyar MM, Eletreby S, et al. Relevance of serum levels of interleukin-6 and syndecan-1 in patients with hepatocellular carcinoma. Sci Pharm. 2012;80(1):179–188. doi: 10.3797/scipharm.1110-07
- Jia Q, Dong Q, Qin L. CCN: core regulatory proteins in the microenvironment that affect the metastasis of hepatocellular carcinoma? Oncotarget. 2016;7(2):1203–1214. doi: 10.18632/oncotarget.6209
- Jain RK. Molecular regulation of vessel maturation. Nat Med. 2003;9(6):685–693. doi: 10.1038/nm0603-685
- Wu XZ, Chen D, Xie GR. Extracellular matrix remodeling in hepatocellular carcinoma: effects of soil on seed? Med Hypotheses. 2006;66(6):1115–1120. doi: 10.1016/j.mehy.2005.12.043
- Elewa MA, Al-Gayyar MM, Schaalan MF, et al. Hepatoprotective and anti-tumor effects of targeting MMP-9 in hepatocellular carcinoma and its relation to vascular invasion markers. Clin Exp Metastasis. 2015;32(5):479–493. doi: 10.1007/s10585-015-9721-6
- Chen Q, Espey MG, Krishna MC, et al. Pharmacologic ascorbic acid concentrations selectively kill cancer cells: action as a pro-drug to deliver hydrogen peroxide to tissues. Proc Natl Acad Sci U S A. 2005;102(38):13604–13609. doi: 10.1073/pnas.0506390102
- Chen Q, Espey MG, Sun AY, et al. Ascorbate in pharmacologic concentrations selectively generates ascorbate radical and hydrogen peroxide in extracellular fluid in vivo. Proc Natl Acad Sci U S A. 2007;104(21):8749–8754. doi: 10.1073/pnas.0702854104
- Li Y, Schellhorn HE. New developments and novel therapeutic perspectives for vitamin C. J Nutr. 2007;137(10):2171–2184. doi: 10.1093/jn/137.10.2171
- Darweish MM, Abbas A, Ebrahim MA, et al. Chemopreventive and hepatoprotective effects of Epigallocatechin-gallate against hepatocellular carcinoma: role of heparan sulfate proteoglycans pathway. J Pharm Pharmacol. 2014;66(7):1032–1045. doi: 10.1111/jphp.12229
- Lee YS. Role of intracellular Ca2+ signal in the ascorbate-induced apoptosis in a human hepatoma cell line. Arch Pharm Res. 2004;27(12):1245–1252. doi: 10.1007/BF02975889
- Lu N, Ding Y, Tian R, et al. Effects of pharmacological ascorbate on hemoglobin-induced cancer cell proliferation. Int J Biol Macromol. 2016;92:1215–1219. doi: 10.1016/j.ijbiomac.2016.08.036
- Alyoussef A, Al-Gayyar MMH. Cytotoxic and partial hepatoprotective activity of sodium ascorbate against hepatocellular carcinoma through inhibition of sulfatase-2 in vivo and in vitro. Biomed Pharmacother. 2018;103:362–372. doi: 10.1016/j.biopha.2018.04.060
- Al-Gayyar MM, Abbas A, Hamdan AM. Chemopreventive and hepatoprotective roles of adiponectin (SULF2 inhibitor) in hepatocelluar carcinoma. Biol Chem. 2016;397(3):257–267. doi: 10.1515/hsz-2015-0265
- Zaghloul RA, El-Shishtawy MM, El Galil KH, et al. Evaluation of antiglypican-3 therapy as a promising target for amelioration of hepatic tissue damage in hepatocellular carcinoma. Eur J Pharmacol. 2015;746:353–362. doi: 10.1016/j.ejphar.2014.11.008
- Tayel A, Abd El Galil KH, Ebrahim MA, et al. Suramin inhibits hepatic tissue damage in hepatocellular carcinoma through deactivation of heparanase enzyme. Eur J Pharmacol. 2014;728:151–160. doi: 10.1016/j.ejphar.2014.02.001
- Al-Gayyar MMH, Bagalagel A, Noor AO, et al. The therapeutic effects of nicotinamide in hepatocellular carcinoma through blocking IGF-1 and effecting the balance between Nrf2 and PKB. Biomed Pharmacother. 2019;112:108653. doi: 10.1016/j.biopha.2019.108653
- Nazmy EA, El-Khouly OA, Zaki MMA, et al. Targeting p53/TRAIL/caspase-8 signaling by adiponectin reverses thioacetamide-induced hepatocellular carcinoma in rats. Environ Toxicol Pharmacol. 2019;72:103240. doi: 10.1016/j.etap.2019.103240
- Pick E, Keisari Y. A simple colorimetric method for the measurement of hydrogen peroxide produced by cells in culture. J Immunol Methods. 1980;38(1–2):161–170. doi: 10.1016/0022-1759(80)90340-3
- Baehner RL, Boxer LA, Davis J. The biochemical basis of nitroblue tetrazolium reduction in normal human and chronic granulomatous disease polymorphonuclear leukocytes. Blood. 1976 Aug;48(2):309–313. doi: 10.1182/blood.V48.2.309.309
- Tayel A, Ebrahim MA, Ibrahim AS, et al. Cytotoxic effects of suramin against HepG2 cells through activation of intrinsic apoptotic pathway. J BUON. 2014;19(4):1048–1054.
- Sherif IO, Al-Gayyar MMH. Oleuropein potentiates anti-tumor activity of cisplatin against HepG2 through affecting proNGF/NGF balance. Life Sci. 2018;198:87–93. doi: 10.1016/j.lfs.2018.02.027
- Darwish WS, Ikenaka Y, Nakayama SM, et al. Constitutive effects of lead on aryl hydrocarbon receptor gene battery and protection by beta-carotene and ascorbic acid in human HepG2 cells. J Food Sci. 2016;81(1):T275–T281. doi: 10.1111/1750-3841.13162
- Kumar DJ, Santhi RJ. Antioxidant and cytotoxic effects of hexane extract of Morinda pubescens leaves in human liver cancer cell line. Asian Pac J Trop Med. 2012;5(5):362–366. doi: 10.1016/S1995-7645(12)60060-1
- Gonzales M, Mitsumori LM, Kushleika JV, et al. Cytotoxicity of iron oxide nanoparticles made from the thermal decomposition of organometallics and aqueous phase transfer with Pluronic F127. Contrast Media Mol Imaging. 2010;5(5):286–293. doi: 10.1002/cmmi.391
- Marra M, Sordelli IM, Lombardi A, et al. Molecular targets and oxidative stress biomarkers in hepatocellular carcinoma: an overview. J Transl Med. 2011;9:171. doi: 10.1186/1479-5876-9-171
- Finkel T, Holbrook NJ. Oxidants, oxidative stress and the biology of ageing. Nature. 2000;408(6809):239–247. doi: 10.1038/35041687
- Block TM, Mehta AS, Fimmel CJ, et al. Molecular viral oncology of hepatocellular carcinoma. Oncogene. 2003;22(33):5093–5107. doi: 10.1038/sj.onc.1206557
- Bartolini D, Dallaglio K, Torquato P, et al. Nrf2-p62 autophagy pathway and its response to oxidative stress in hepatocellular carcinoma. Transl Res. 2018;193:54–71. doi: 10.1016/j.trsl.2017.11.007
- Taguchi K, Motohashi H, Yamamoto M. Molecular mechanisms of the Keap1-Nrf2 pathway in stress response and cancer evolution. Genes Cells. 2011;16(2):123–140. doi: 10.1111/j.1365-2443.2010.01473.x
- Bruno S, Zuin M, Crosignani A, et al. Predicting mortality risk in patients with compensated HCV-induced cirrhosis: a long-term prospective study. Am J Gastroenterol. 2009;104(5):1147–1158. doi: 10.1038/ajg.2009.31
- Reid AE, Koziel MJ, Aiza I, et al. Hepatitis C virus genotypes and viremia and hepatocellular carcinoma in the United States. Am J Gastroenterol. 1999;94(6):1619–1626. doi: 10.1111/j.1572-0241.1999.01153.x
- Ribeiro PS, Cortez-Pinto H, Sola S, et al. Hepatocyte apoptosis, expression of death receptors, and activation of NF-kappaB in the liver of nonalcoholic and alcoholic steatohepatitis patients. Am J Gastroenterol. 2004;99(9):1708–1717. doi: 10.1111/j.1572-0241.2004.40009.x
- Rutledge AC, Adeli K. Fructose and the metabolic syndrome: pathophysiology and molecular mechanisms. Nutr Rev. 2007;65(6 Pt 2):S13–S23. doi: 10.1301/nr.2007.jun.S13-S23
- Al-Gayyar MM, Abdelsaid MA, Matragoon S, et al. Thioredoxin interacting protein is a novel mediator of retinal inflammation and neurotoxicity. Br J Pharmacol. 2011;164(1):170–180. doi: 10.1111/j.1476-5381.2011.01336.x
- Berasain C, Castillo J, Perugorria MJ, et al. Inflammation and liver cancer: new molecular links. Ann N Y Acad Sci. 2009;1155:206–221. doi: 10.1111/j.1749-6632.2009.03704.x
- Budhu A, Wang XW. The role of cytokines in hepatocellular carcinoma. J Leukoc Biol. 2006;80(6):1197–1213. doi: 10.1189/jlb.0506297
- Wheelhouse NM, Chan YS, Gillies SE, et al. TNF-alpha induced DNA damage in primary murine hepatocytes. Int J Mol Med. 2003;12(6):889–894.
- Huang TH, Chiu YH, Chan YL, et al. Antrodia cinnamomea alleviates cisplatin-induced hepatotoxicity and enhances chemo-sensitivity of line-1 lung carcinoma xenografted in BALB/cByJ mice. Oncotarget. 2015;6(28):25741–25754. doi: 10.18632/oncotarget.4348
- El-Naga RN. Pre-treatment with cardamonin protects against cisplatin-induced nephrotoxicity in rats: impact on NOX-1, inflammation and apoptosis. Toxicol Appl Pharmacol. 2014;274(1):87–95. doi: 10.1016/j.taap.2013.10.031
- Al-Gayyar MM, Elsherbiny NM. Contribution of TNF-alpha to the development of retinal neurodegenerative disorders. Eur Cytokine Netw. 2013;24(1):27–36. doi: 10.1684/ecn.2013.0334
- Lee EW, Seo J, Jeong M, et al. The roles of FADD in extrinsic apoptosis and necroptosis. BMB Rep. 2012;45(9):496–508. doi: 10.5483/BMBRep.2012.45.9.186
- Al-Gayyar MM, Al Youssef A, Sherif IO, et al. Protective effects of arjunolic acid against cardiac toxicity induced by oral sodium nitrite: effects on cytokine balance and apoptosis. Life Sci. 2014;111(1–2):18–26. doi: 10.1016/j.lfs.2014.07.002
- Chwa M, Atilano SR, Hertzog D, et al. Hypersensitive response to oxidative stress in keratoconus corneal fibroblasts. Invest Ophthalmol Vis Sci. 2008 Oct;49(10):4361–4369. doi: 10.1167/iovs.08-1969
- Fu XB, Liu DD, Lin Y, et al. Water-soluble DNA minor groove binders as potential chemotherapeutic agents: synthesis, characterization, DNA binding and cleavage, antioxidation, cytotoxicity and HSA interactions. Dalton Trans. 2014;43(23):8721–8737. doi: 10.1039/c3dt53577k
- Vasquez DR, Verrax J, Valderrama JA, et al. Aminopyrimidoisoquinolinequinone (APIQ) redox cycling is potentiated by ascorbate and induces oxidative stress leading to necrotic-like cancer cell death. Invest New Drugs. 2012 Jun;30(3):1003–1011. doi: 10.1007/s10637-011-9661-1