ABSTRACT
Background: The cardiovascular crisis is advancing rapidly throughout the world. A large number of studies have shown that plant polyphenols affect major mechanisms involved in cardiovascular events through their action on the antioxidant system, signaling, and transcription pathways. D-limonene, a monocyclic monoterpene obtained from citrus fruits, is reported to possess many pharmacological activities.
Methods: The experiment was designed to determine the protective effect of D-limonene against cardiac injury induced by CCl4 in Wistar rats. Rats were treated with two doses of D-limonene against cardiac injury induced by CCl4. Serum toxicity markers, cardiac toxicity biomarker enzymes, inflammatory mediators, anti-oxidant armory, lipid peroxidation, lipid profile, and histology were done.
Results: CCl4 intoxication resulted in a substantial rise in FFA, TC, TG, PL, LDL, VLDL, and a reduction in HDL, restoring these changes with the administration of D-limonene at a dosage of 200 mg/kg. CCl4 administration also resulted in lipid oxidation and decreased antioxidant activity. At the same time, D-limonene at a dosage of 200 mg/kg body weight inhibited LPO and restored in vivo antioxidant components to normal. CCl4 intoxication also resulted in a significant increase in inflammatory markers like IL-6, TNF-α, high sensitivity Corticotropin Releasing Factor (Hs-CRF), and biomarkers of cardiac toxicity like alanine aminotransferase (ALT), lactate dehydrogenase (LDH), creatine kinase (CK), creatine kinase MB (CKMB), and Troponin I & troponin-t activities. D-limonene reversed all these changes to normal. Histology further confirmed our obtained results.
Conclusion: These findings indicate that D-limonene can ameliorate cardiac injury at a 200 mg/kg body weight dosage. Henceforth, D-Limonene intervenes in mediating CCl4 induced toxicity by various signaling pathways.
GRAPHICAL ABSTRACT
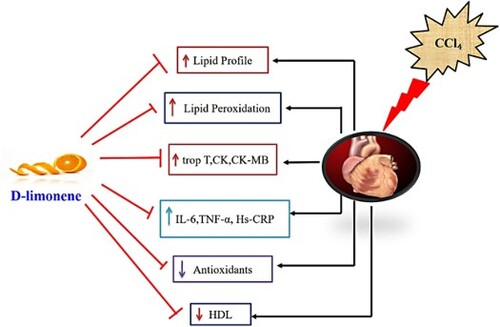
Introduction
The cardiovascular crisis is advancing rapidly throughout the world. Until the next decade, nearly 24 million people are estimated to die from cardiovascular diseases (CVDs) [Citation1]. Nevertheless, some CVD-related fatalities could be prevented. A large number of studies have shown that lifestyle changes, including nutrition and diet, can play a key role in reducing the risk of CVD [Citation2]. Foods such as whole grains, fruit, and vegetables derived from plants have been shown to minimize the risk of mortality for several diseases, including CVD [Citation3,Citation4]. The necessary quantity and period of consumption of plant-derived food were linked to a lower risk of CVD and thus lower CVD mortality. The mechanisms by which plant-derived foods demonstrate their preventive effects are not completely obvious, but preclinical studies have shown that plant polyphenols are the key negotiators. Several studies have reported that plant polyphenols affect major mechanisms involved in cardiovascular events through their action on the antioxidant system, signalling, and transcription pathways [Citation5–7].
Carbon tetrachloride (CCl4) is a highly severe hepatotoxin. Besides harming the liver, it also causes significant damage to other tissues and the heart by producing free radicals [Citation8,Citation9]. CCl4 at higher dosages results in cell death, oxidative strain, and inflammation resulting in serious cellular damage and organ failure. The harmful effects of CCl4 are caused by an increased free radical formation, which plays a crucial role in tissue degeneration and various animal models of heart failure [Citation10,Citation11]. CCl4 intoxication occurs in two steps: first, the production of reactive oxygen species (CCl3* and CCl3OO*) via the interference of the metabolism of cytochrome P450, culminating in lipid peroxidation (LPO); the very next pathway leads to inflammation production and the development of profibrogenic mediators through stimulation of tissue macrophages [Citation12]. Hence, holding the equilibrium between free radical production and antioxidants and restraining inflammation mediators can serve as an essential mechanism for preventing the impact of harmful chemicals. The role of oxidative stress and inflammation in the progression of many health conditions has contributed to the probability that antioxidant and anti-inflammatory compounds may be helpful to well-being. A variety of antioxidant-potential compounds have been reported to reduce the toxicity caused by CCl4 [Citation13–16].
There are various methods for induction myocardial infarction, myocarditis, and heart failure in vitro, in vivo in experimental animals and patients. Some of which are genetically modified, electrical methods, chemically induced methods, and many more in experimental animals. CCl4 is used as a chemical agent to induce myocardial infarction/cardiotoxicity. It mimics the pathology close to humans by producing free radicals, a rate-limiting process in cellular peroxidative damage [Citation17]. These free radicals and other related oxidative species cause oxidative stress, which produces significant interrelated changes in cellular metabolism, thus increasing the calcium level inside the cell, which further causes damage to the permeability and transport of ions through the membrane and destroys the cells by LPO. This model has been seen to elevate lactate dehydrogenase (LDH), creatine kinase (CK), and malondialdehyde (MDA) levels and alleviate the level of antioxidant enzyme armoury, which includes SOD, CAT, and GPx levels in the heart, indicating that the heart can be targeted by CCl4 [Citation17,Citation18]. It has also been reported that the release of ROS can lead to myocardial ischaemia with subsequent cell injury and mitochondrial dysfunction. Cell injury in the ischaemic area also causes infiltration of neutrophils, which produce ROS and cytokines. Further cytokines like tumour necrosis factor-α (TNF-α) trigger the mitochondrial release of ROS. In addition, an increase in ROS has been detected in various animal models of heart failure. Reports suggest that an increase in oxidative stress, due to increased ROS production, a relative deficit in the endogenous antioxidant machinery, or both, can cause myocarditis, contractile dysfunction, and cardiomyopathy. Similar results have been found in our study that of increased ROS production, a deficit of anti-oxidant armoury, anomalous increase in biomarkers of cardiac function, increased inflammatory mediators and increased cardiac injury/cardiotoxicity in the disease control group, and alleviation by D-limonene possibly by decreasing ROS, cytokines, and increasing anti-oxidant machinery [Citation18,Citation19].
Natural dietary products with anti-oxidant and anti-inflammatory potential have acquired therapeutic significance due to their protecting prospects against various ailments. D-limonene is a monocyclic monoterpene, a colourless liquid with a citrus odour at room temperature. Due to its lemon-like smell, it has found its application in soaps and perfumes and is found mainly in citrus oils. Oils obtained from citrus species, such as lemon, grapefruits, orange peels, and many more, have large quantities of D-limonene [Citation16,Citation20]. D-Limonene, a monoterpene, was collected from different traditional medicines, such as Curcuma longa, salvia species, Citrullus colosynthis fruits, Adansonnia digitata, finger citron fruits, etc. [Citation21]. D-limonene is the main ingredient in lemon and is mentioned in the Federal Aromatic Substances as safe [Citation22,Citation23]. D-limonene has been categorized as having a broad spectrum of biological activities, such as antioxidant, anti-inflammatory, antibacterial, antiviral, anticancer, anti-fibrotic, vasodilating, and anti-hypertensive. For experimental models, the non-convulsant monoterpenes dose is considered safe. Furthermore, D-limonene does not cause a carcinogenic, mutagenic, or hepatotoxic threat to human life [Citation24–30]. Although there is no evidence of the effects of D-limonene on cardiac toxicity, the current investigation was however designed to decipher the protective function of D-limonene by assessing its influence on multiple signalling pathways like oxidative stress, inflammation, and cardiac mechanisms in cardiac toxicity-induced CCl4 experimental study.
Materials and methods
Chemicals: D-limonene was purchased from Sigma-Aldrich India. The majority of the chemicals and kits used in the study were of high standard. Saline was used as a vehicle for D-limonene [Citation24,Citation29].
Experimental layout
Twenty-four, five- to seven-week-old, albino rats (160–180 g) of Wistar strain were randomly divided into four groups of six rats each. The animals had easy access to diet and water and were housed in a room at 25°C with a light/dark period of 12 h.
Group I: Served normal control and received 1 mL/kg body weight of normal saline twice a week for three weeks.
Group II: Disease control received CCl4 (1 mL/kg body weight 1:1 mixture with olive oil) i.p. twice a week for three weeks.
Group III: Treatment group 1 CCl4 (1 mL/kg body weight 1:1 mixture with olive oil) i.p. twice a week + 100 mg/kg body weight D-limonene for three weeks by oral gavage.
Group IV: Treatment group 2 CCl4 (1 mL/kg body weight 1:1 mixture with olive oil) i.p. twice a week + 200 mg/kg body weight D-limonene for three weeks by oral gavage 28. Doses of CCl4 and D-limonene were based on previous studies from our lab and from another lab reports as well [Citation18,Citation24,Citation31–37].
Sample collection
After the treatment schedule was completed, the animals were euthanized under anaesthesia, blood was collected by cardiac puncture [Citation19] in clean and dry test tubes, left to clot for 30 min, centrifuged at 3000 rpm for serum separation and the serum sample was used to measure lipid profile. The hearts were removed aseptically for the preparation of cell-free extract and were used for the estimation of biomarkers/hallmarks of cardiac function such as creatine kinase (CK), creatine kinase-MB (CK-MB), troponin-t, LPO, inflammatory markers, high-sensitive C-reactive protein (Hs-CRP), interleukin-6 (IL-6), tumour necrosis factor-alpha (TNF-α), in vivo antioxidants like catalase (CAT), superoxide dismutase (SOD), glutathione peroxidase (GPx), etc. The protocols involved were duly approved by the committee constituted for animal ethics vide No. BERC-014-05-21.
Preparation of cell-free extract of cardiac tissue
These were cut into small pieces after removing hearts aseptically and homogenized in Potter–Elvehjem type homogenizer in 100 mM KH2PO4 (potassium dihydrogen phosphate) buffer composed of 1 mM EDTA (ethylenediaminetetraacetic acid), pH 7.4. It had been further centrifuged for 30 min at 12,000g. The supernatant was collected for LPO, inflammatory, cardiac, and in vivo antioxidant marker estimation [Citation38].
Determination of lipid peroxidation
LPO was assayed in cardiac tissue according to Wright et al. [Citation39].
Determination of in vivo antioxidants
Catalase (CAT) activity was assayed in cardiac tissue according to Claiborne [Citation40].
The superoxide dismutase (SOD) estimation in cardiac tissue was done by Marklund and Maruklund [Citation41].
The glutathione peroxidase (GPx) estimation in cardiac tissue was done by Mohandas et al. [Citation42].
Determination of lipid profile
The estimation of serum-free fatty acids (FFAs), total cholesterol (TC), phospholipids (PLs) and triglycerides (TGs), high density lipoproteins (HDLs), low-density lipoproteins (LDLs), and very low-density lipoprotein (VLDL) estimations were carried out by Roche diagnostics kits (Roche Diagnostics GmbH, Mannheim, Germany) or commercially available kits from Merck India.
Determination of inflammatory and cardiac markers
The inflammatory and cardiac markers like Hs-CRPs, IL-6, TNF-α, CK, CK-MB, and troponin-t were estimated by using ELISA-based kits as per the instruction of manufacturer.
Histology
Slices from the left ventricles of each heart were fixed in a 10% neutral buffered formalin solution and embedded in paraffin, sectioned at 4 µm, subsequently stained with haematoxylin/eosin, and observed under a light microscope mainly for inflammatory, cellular damage, and necrotic changes with respect to control healthy rats’ heart [Citation18].
Statistical analysis
To estimate the effect of treatment, analysis of variance (ANOVA) was carried out followed by Tukey–Kramer multiple comparisons test, and minimum criterion for statistical significance was set at P < .05.
Results
Effect of CCl4 and D-limonene on lipid profile
As shown in , CCl4 intoxication resulted in substantial increases in FFA, TC, PL, TG, LDL, VLDL, and substantial reduction in HDL. D-limonene (200 mg/kg body weight) treatment significantly restored these levels near the normal. However, treatment with D-limonene (100 mg/kg body weight) tends to result in inconsequential changes in lipid profile levels.
Table 1. Effect of different doses of D-limonene on lipid profile.
Effect of CCl4 and D-limonene on biomarkers of cardiac function
CCl4 toxicity showed a significant increase in hallmarks/biomarkers of cardiac function like CK, CK-MB, and Troponin-t in comparison to control. The treatment with D-limonene (200 mg/kg body weight) significantly reversed these markers near to normal while treatment with D-limonene (100 mg/kg body weight) showed significant improvement in CK compared to the CCl4 group ().
Table 2. Effect of different doses of D-limonene on cardiac toxicity markers.
Effect of CCl4 and D-limonene on cardiac antioxidants
CCl4 toxicity resulted in a considerable decrease in CAT, SOD, and GPx activity and an increase in LPO levels in cardiac tissue relative to the control group. D-limonene (200 mg/kg body weight) administration greatly enhanced the activity of cardiac enzymatic antioxidants near to normal and decreased the unusual increase of LPO. D-limonene (100 mg/kg body weight) administration showed insignificant changes in those parameters (). However, it could be possible that other proteins could also contribute to the total activities of the enzymes mentioned above and not just the enzymes mentioned alone, which could be considered as a limitation of the current study.
Table 3. Effect of different doses of D-limonene on lipid peroxidation and enzymatic antioxidants.
Effect of CCl4 and D-limonene on cardiac inflammatory markers
CCl4 toxicity resulted in considerable elevations relative to regulation in cardiac tissue levels of IL-6 (), Hs-CRP (), and TNF-α (). D-limonene (200 mg/kg body weight) treatments have substantially decreased the levels of these inflammatory markers to near normal compared to the CCl4, group but D-limonene 100 mg/kg body weight showed no significant response ().
Figure 1. Effect of different doses of D-limonene on IL-6. Group I: Control; Group II: CCl4 group; Group III: CCl4 + D-limonene (100 mg/kg body weight); values are expressed as mean ± SE, n = 6; *signifies difference with control while #signifies difference with CCl4, ***indicates significance at P < .001 and ##indicates significance at P < .01.
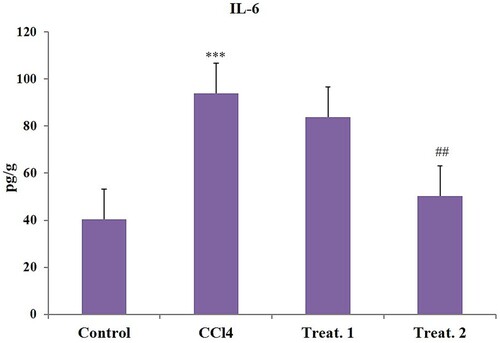
Figure 2. Effect of different doses of D-limonene on Hs-CRP markers. Group I: Control; Group II: CCl4 group; Group III: CCl4 + D-limonene (100 mg/kg body weight); values are expressed as mean ± SE, n = 6; *signifies difference with control while #signifies difference with CCl4, ***indicates significance at P < .001 and ##indicates significance at P < .01.
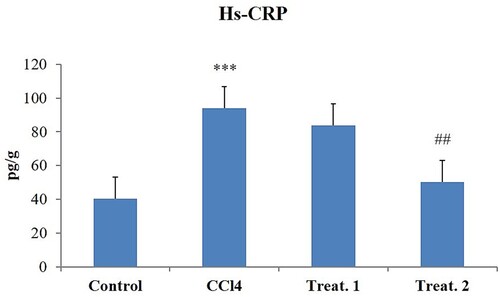
Figure 3. Effect of different doses of D-limonene on TNF-α. Group I: Control; Group II: CCl4 group; Group III: CCl4 + D-limonene (100 mg/kg body weight); values are expressed as mean ± SE, n = 6; *signifies difference with control while #signifies difference with CCl4, ***indicates significance at P< .001 and ##indicates significance at P < .01.
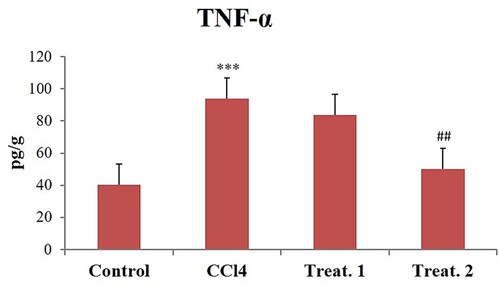
Effect of CCl4 and D-limonene on cardiac tissue histology
The histological examination of the heart tissues of the control rats (group I) showed normal structure in the branching of cardiac fibres having striated eosinophilic sarcoplasm and central vesicular oval nuclei. The interstitial spaces containing fibroblasts and blood capillaries between them are narrow, whereas CCl4 administered rats (group II) show anomalies like increased spacing between cells with congested capillaries surrounded by acidophilic homogenous material and infiltration of inflammatory cells. Deeply stained pyknotic nuclei, necrosis, oedema, and degenerated cardiomyocytes also appear. However, co-treatment with D-limonene led to a marked improvement in these abnormalities like the minimal separation between the cells and few pyknotic nuclei, cellular infiltration in group III, and restoration of histo-architecture as though to near normal in group IV compared to CCl4 group ().
Figure 4. Effect of different doses of D-limonene on histoarchitecture of heart tissue. Microphotograph of H&E-stained sections of the heart. Group I: shows normal histology, the arrow represents striated eosinophilic sarcoplasm, ‘n’ represents central vesicular nuclei, interstitial spaces are represented by ‘s.’ Group II: has CCl4-treated section shows numerous pathological indications, double arrows represent increased spacing between cells with congested capillaries, ‘stars’ indicate periventricular infiltration of inflammatory cells. ‘d’ shows deeply stained pyknotic nuclei. Group III: CCl4 + D-limonene (100 mg/kg body weight); myofibrils appear closer, with less wide interstitial spaces. Group IV: CCl4 + D-limonene (200 mg/kg body weight) showed it decreased nuclear degeneration; normal myofibers with central nuclei represented by (n), and regular narrow interstitial spaces (s).
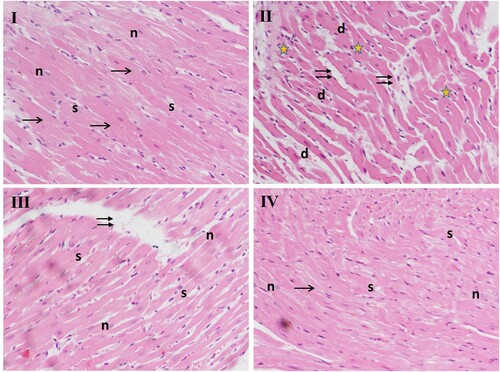
Discussion
CCl4 is an effective agent for evaluating the tissue injury. It is due to a high affinity to CYP450 expressing tissues, and the heart muscle represents the best target for injury by this organic pollutant. It is reported to damage the different organs and blood by creating chemical toxicity through the generation of free radicals. These radicals lead to peroxidation by interacting with membrane lipids resulting in an imbalance in lipid synthesis and degradation [Citation12,Citation43]. The activated metabolites of CCl4 target the lipids very easily resulting in membrane damage [Citation44]. The membrane damage results in the generation of radicals and LPO, thus also in an increased accumulation of lipids, which is considered to be a pathological condition; the excessive accumulation results in impaired organ function [Citation43,Citation44]. In the present study, CCl4 resulted in the elevation of TC, FFA, TG, PL, LDL, and VLDL, and reduced HDL because CCl4 is reported to enhance the synthesis of FFA and TG from acetate, thus providing a substrate for excessive production of these molecules. CCl4 is also reported to inhibit the synthesis of apolipoproteins, thus resulting in the decreased synthesis of these molecules [Citation45,Citation46]. The administration of D-limonene (200 mg/kg body weight) reversed lipid changes in CCl4-treated rats. It has been reported that D-limonene regulates the formation of fatty tissue and production of fat through the involvement of transcriptional factors PPARϒ, C/EPB-α, and CEPB-β and their downstream goals by activating the Akt-signalling pathway and by preventing oxidative stress through inhibition of H2O2-induced caspase-3 and -9 activations and by inhibiting H2O2-activated p38MAPK phosphorylation [Citation30,Citation47,Citation48].
The cardiovascular system contains a number of protection mechanisms including enzymatic antioxidants, endogenous free radical scavengers, vitamins E and C, and reduced glutathione to guard the cell against invasion by free radicals and LPO by-products. The varied enzyme actions towards CCl4 insult have been reported by a large number of studies [Citation12–14,Citation29]. Cellular depletion of these enzymes has been linked to LPO caused by toxic substances. The progression of cardiomyopathy induced by adriamycin also involves LPO [Citation49,Citation50]. The present study is in agreement with previous studies that the antioxidant defence system of cardiac muscle was working at lower rates irrespective of oxidative stress in CCl4-induced cardiac injury [Citation29]. The treatment with 200 mg/kg body weight of D-limonene prevented LPO and resulted in enhanced activities of SOD, CAT, and GPx. The findings of the present experiment, therefore, suggest that D-limonene (200 mg/kg body weight) shields the cardiac tissue against CCl4 intoxication and elevates antioxidant tendency. However, it could be possible that other proteins could also contribute to the total activities of the enzymes mentioned above and not just the enzymes mentioned alone, which could be considered a limitation to the current study as we have not used any specific inhibitors in our current study.
The compounds with a basic isoprene structure have been reported to exhibit antioxidant properties since limonene contains two isoprene units which render it a strong antioxidant molecule [Citation27,Citation28,Citation30,Citation51]. Limonene has been reported to saturate the pulmonary membrane and protect the lungs against both exogenous and endogenous ozone and other oxidative oxidant substances; other studies have reported that the essential oil obtained from celery, possessing 74.6% of limonene, has inhibitory activity towards MDA [Citation52].
A cardiac insult measurement involves a battery of cardiac markers including CK, CK-MB, AST, alanine aminotransferase (ALT), ALP, LDH, troponin-t, and TC. However, all of these are not confined to cardiac tissue; only increased serum levels of CK-MB and troponin-t have universally been accepted as a reliable indicator of cardiac injury [Citation53–55]. The membrane peroxidation results in the leakage of these molecules in the plasma, thus enhancing their levels in the serum which acts as a marker of cardiac injury. Lipid and protein peroxidation follows myocardial cell membrane injury resulting in leakage of various enzymes like CK, CK-MB, LDH, Hs-CRP into the bloodstream and are increased after CCl4 administration as reported previously [Citation12,Citation56]. Therefore, mitigating the disturbance of cardiomyocyte cell membrane antioxidants like D-limonene may prevent cardiomyocyte injury induced by CCl4 and subsequent leakage of cellular enzymes [Citation57]. CCl4 toxicity resulted in enhanced leakage of CK, CK-MB, and troponin-t, which are in line with the previous research that CCl4 causes cardiac tissue disruption and secretion of CK-MB and troponin-t into the serum [Citation58,Citation59]. A significant reduction was observed in CK, CK-MB, and troponin-t level biomarkers of cardiac function with D-limonene (200 mg/kg body weight) suggesting improved cardiac function which is in concurrence with the findings that limonene protects against cardiovascular disease by lowering hypertension and cardiac arrhythmias [Citation30,Citation57]. However, these biochemical parameters which include CK, CK-MB, and troponin-t are just biomarkers of cardiac damage/injury. Actual cardiac function relates to the measurement of cardiac output, ventricular pressure, ejection fraction, or electrophysiological test or other functional assays could be considered as a limitation to the current study as it is not clear if function is impacted by CCl4/D-limonene in any case.
The inflammatory response has been identified as a crucial mechanism for causing organ damage during different stresses. CCl4 insult results in tissue damage which is reflected by the increased secretion of inflammatory cytokines Hs-CRP, IL-6, and TNF-α. CRP is predominantly produced by liver, adipocytes, and smooth vascular muscle cells in stimulus to increase the secretion of IL-6 and TNF-α of the tissue [Citation59–61]. The elevation in inflammatory cytokines by CCl4 is a result of the activation of nuclear factor kappa-B (NF-κB) as shown in our previous study [Citation29] and stimulates the release of inflammatory cytokines like IL-6, CRP, and TNF-α. Induction of these markers has a critical role in the CCl4-mediated cardiac injury and dysfunction [Citation13,Citation15]. D-limonene (200 mg/kg body weight) markedly attenuated the increased levels of inflammatory markers TNF-α, IL-6, and Hs-CRP to near normal. The anti-inflammatory properties of D-limonene have previously been proven through the inhibition of redox-dependent NF-κB and other downstream inflammatory cytokines which are key players in exhibition of inflammation as reported previously [Citation26,Citation29]. The limonene is also reported to exhibit its anti-inflammatory activity by saturating the cell membrane being a low molecular weight lipophilic compound, thus providing anti-inflammatory protection to the cell [Citation62]. Control group heart slides stained with haematoxylin and eosin showed healthy cardiac structures. Nevertheless, CCl4 group cardiotoxicity showed inflammatory cell infiltration, widespread necrosis, vacuolization of cytoplasm, swelling, interstitial oedema, and loss of myofibres. Treatment with D-limonene (100 and 200 mg/kg body weight) reversed all the changes induced by CCl4 intoxication, and heart structures appeared normal, suggesting that D-limonene has a potent protective effect in this model of cardiac injury. Alleviation of histopathological changes could be attributed to D-limonene at both the doses as reported earlier [Citation18,Citation63].
Conclusion
In the present research, the findings indicate that D-limonene derived from different citrus fruits can ameliorate cardiac injury induced by CCl4 intoxication through its antioxidant and anti-inflammatory potential. These results reveal that D-limonene has protective potential against the toxic effects of CCl4 by enhancing antioxidant machinery, attenuating LPO, inhibiting inflammatory cascade, and restoring histopathological changes to normal.
Acknowledgement
The authors are thankful to the Faculty of Veterinary Science and Animal Husbandry, SKUAST-Kashmir, J&K, India, for their support.
Disclosure statement
No potential conflict of interest was reported by the author(s).
Data availability statement
The data that support the findings of this study are available from the corresponding author, upon reasonable request.
References
- Virani SS, Alonso A, Benjamin EJ, et al. Heart disease and stroke statistics-2020 update: a report from the American Heart Association. Circulation. 2020;141(9):e139–e596. DOI:10.1161/CIR.0000000000000757
- Billingsley HE, Hummel SL, Carbone S. The role of diet and nutrition in heart failure: a state-of-the-art narrative review. Prog Cardiovasc Dis. 2020;63(5):538–551. DOI:10.1016/j.pcad.2020.08.004
- Man AWC, Li H, Xia N. Impact of lifestyles (diet and exercise) on vascular health: oxidative stress and endothelial function. Oxid Med Cell Longev. 2020;2020:1496462. DOI:10.1155/2020/1496462
- Barkas F, Nomikos T, Liberopoulos E, et al. Diet and cardiovascular disease risk among individuals with familial hypercholesterolemia: systematic review and meta-analysis. Nutrients. 2020;12(8):2436. DOI:10.3390/nu12082436
- Trautwein EA, McKay S. The role of specific components of a plant-based diet in management of dyslipidemia and the impact on cardiovascular risk. Nutrients. 2020;12(9):2671. DOI:10.3390/nu12092671
- Feldman F, Koudoufio M, Desjardins Y, et al. Efficacy of polyphenols in the management of dyslipidemia: a focus on clinical studies. Nutrients. 2021;13(2):672. DOI:10.3390/nu13020672
- Zhang K, Cao H, Gao Y, et al. Marchantia polymorpha L. flavonoids protect liver from CCl4-induced injury by antioxidant and gene-regulatory effects. Altern Ther Health Med. 2020;28(3):34–41.
- Botsoglou NA, Taitzoglou IA, Botsoglou E, et al. Effect of long-term dietary administration of oregano and rosemary on the antioxidant status of rat serum: liver, kidney and heart after carbon tetrachloride-induced oxidative stress. J Sci Food Agric. 2009;89:1397–1406.
- Islam MA, Al Mamun MA, Faruk M, et al. Astaxanthin ameliorates hepatic damage and oxidative stress in carbon tetrachloride-administered rats. Pharmacognosy Res. 2017;9(Suppl. 1):S84–S91. DOI:10.4103/pr.pr_26_17
- Lee S, Won KY, Joo S. Protective effect of polydeoxyribonucleotide against CCl4-induced acute liver injury in mice. Int Neurourol J. 2020;24(Suppl. 2):88–95. DOI:10.5213/inj.2040430.215
- Lee IC, Kim SH, Baek HS, et al. The involvement of Nrf2 in the protective effects of diallyl disulfide on carbon tetrachloride-induced hepatic oxidative damage and inflammatory response in rats. Food Chem Toxicol. 2014;63:174–185. DOI:10.1016/j.fct.2013.11.006
- Unsal V, Cicek M, Sabancilar İ. Toxicity of carbon tetrachloride, free radicals and role of antioxidants. Rev Environ Health. 2020. DOI:10.1515/reveh-2020-0048
- Wang Y, Wang S, Wang R, et al. Neferine exerts antioxidant and anti-inflammatory effects on carbon tetrachloride-induced liver fibrosis by inhibiting the MAPK and NF-κB/IκBα pathways. Evid Based Complement Alternat Med. 2021;2021:4136019. DOI:10.1155/2021/4136019
- Rahman MM, Muse AY, Khan DMIO, et al. Apocynin prevented inflammation and oxidative stress in carbon tetra chloride induced hepatic dysfunction in rats. Biomed Pharmacother. 2017;92:421–428. DOI:10.1016/j.biopha.2017.05.101
- Zhao J, Hu H, Wan Y, et al. Pien Tze Huang Gan Bao ameliorates carbon tetrachloride-induced hepatic injury: oxidative stress and inflammation in rats. Exp Ther Med. 2017;13(5):1820–1826. DOI:10.3892/etm.2017.4174
- Amini R, Asle-Rousta M, Aghazadeh S. Hepatoprotective effect of limonene against chronic immobilization induced liver damage in rats. Naunyn Schmiedebergs Arch Pharmacol. 2020;393(11):2053–2059. DOI:10.1007/s00210-020-01915-0
- Ahsan F, Mahmood T, Usmani S, et al. A conglomeration of preclinical models related to myocardial infarction. Braz J Pharm Sci. 2020;56. DOI:10.1590/s2175-97902019000418365
- Masoud E, Samad Z, Nafiseh B, et al. Cardioprotective effect of Cornus mas fruit extract against carbon tetrachloride induced-cardiotoxicity in albino rats. J Basic Appl Sci Res. 2012;2(11):11106–11114.
- Abdel-Wahab BA, Metwally ME. Clozapine-induced cardiotoxicity: role of oxidative stress: tumour necrosis factor alpha and NF-κβ. Cardiovasc Toxicol. 2015;15(4):355–365. DOI:10.1007/s12012-014-9304-9
- Anandakumar P, Kamaraj S, Vanitha MK. D-limonene: a multifunctional compound with potent therapeutic effects. J Food Biochem. 2021;45(1):e13566. DOI:10.1111/jfbc.13566
- Erasto P, Viljoen AM. Limonene: a review: biosynthetic: ecological and pharmacological relevance. Nat Prod Comm. 2008;3(7):1193–1202.
- Ravichandran C, Badgujar PC, Gundev P, et al. Review of toxicological assessment of D-limonene, a food and cosmetics additive. Food Chem Toxicol. 2018;120:668–680. DOI:10.1016/j.fct.2018.07.052
- Kim YW, Kim MJ, Chung BY, et al. Safety evaluation and risk assessment of D-limonene. J Toxicol Environ Health B Crit Rev. 2013;16(1):17–38.
- Babaeenezhad E, Hadipour Moradi F, Rahimi Monfared S, et al. D-limonene alleviates acute kidney injury following gentamicin administration in rats: role of NF-κB pathway: mitochondrial apoptosis, oxidative stress, and PCNA. Oxid Med Cell Longev. 2021;2021:6670007. DOI:10.1155/2021/6670007
- Bai J, Zheng Y, Wang G, et al. Protective effect of D-limonene against oxidative stress-induced cell damage in human lens epithelial cells via the p38 pathway. Oxid Med Cell Long. 2016;5962832:1–12.
- de Vasconcelos Cerqueira Braz J, de Carvalho FO, de Vasconcelos Cerqueira Meneses D, et al. Mechanism of action of limonene in tumor cells: a systematic review and metanalysis. Curr Pharm Des. 2020. DOI:10.2174/1381612826666201026152902
- Chaudhary S, Siddiqui M, Athar M, et al. d-Limonene modulates inflammation: oxidative stress and Ras-ERK pathway to inhibit murine skin tumorigenesis. Hum Exp Toxicol. 2012;31(8):798–811. DOI:10.1177/0960327111434948
- Yu L, Yan J, Sun Z. D-limonene exhibits anti-inflammatory and antioxidant properties in an ulcerative colitis rat model via regulation of iNOS, COX-2, PGE2 and ERK signaling pathways. Mol Med Rep. 2017;15:2339–2346.
- Ahmad SB, Rehman MU, Fatima B, et al. Antifibrotic effects of D-limonene (5(1-methyl-4-[1-methylethenyl]) cyclohexane) in CCl4 induced liver toxicity in Wistar rats. Environ Toxicol. 2018;33(3):361–369.
- Santiago JVA, Jayachitra J, Shenbagam M, et al. D-limonene attenuates blood pressure and improves the lipid and antioxidant status in high fat diet and L-NAME treated rats. J Pharm Sci Res. 2010;2(11):752–758.
- Mohamed A. Prophylatic role of L-carnitine and ubiquinone in combating the cardiotoxicity induced by carbon tetrachloride in rat. Int J Acad Res. 2010;2:52–59.
- Jayakumar T, Sakthivel M, Thomas PA, et al. Pleurotus ostreatus, an oyster mushroom: decreases the oxidative stress induced by carbon tetrachloride in rat kidneys, heart and brain. Chem Biol Interact. 2008;176(2-3):108–120. DOI:10.1016/j.cbi.2008.08.006
- Botsoglou NA, Taitzoglou IA, Botsoglou E, et al. Effect of long-term dietary administration of oregano on the alleviation of carbon tetrachloride-induced oxidative stress in rats. J Agric Food Chem. 2008;56(15):6287–6293. DOI:10.1021/jf8003652
- Botsoglou N, Taitzoglou I, Zervos I, et al. Potential of long-term dietary administration of rosemary in improving the antioxidant status of rat tissues following carbon tetrachloride intoxication. Food Chem Toxicol. 2010;48(3):944–950. DOI:10.1016/j.fct.2010.01.004
- Elbaky NAA, El-Orabi NF, Fadda LM, et al. Role of N-acetylcysteine and coenzyme Q10 in the amelioration of myocardial energy expenditure and oxidative stress,: induced by carbon tetrachloride intoxication in rats. Dose Response. 2018;16(3):1559325818790158. DOI:10.1177/1559325818790158
- Emam NM, Anjum S, Okail HA, et al. Pomegranate peel extract protects against carbon tetrachloride-induced nephrotoxicity in mice through increasing antioxidants status. Biomed Rep. 2020;13(3):13. DOI:10.3892/br.2020.1320
- Murali R, Saravanan R. Antidiabetic effect of d-limonene, a monoterpene in streptozotocin-induced diabetic rats. Biomed Preventive Nutr. 2012;2(4):269–275.
- Ćirić Zdravković S, Kostić T, Marcetić ZP, et al. Melatonin modulates acute cardiac muscle damage induced by carbon tetrachloride – involvement of oxidative damage, glutathione, and arginine and nitric oxide metabolism. Can J Physiol Pharmacol. 2021;99(4):360–367. DOI:10.1139/cjpp-2020-0201
- Wright JR, Colby HD, Miles PR. Cytosolic factors which affect microsomal lipid peroxidation in lung and liver. Arch of Biochem and Biophy. 1981;206(2):296–304.
- Claiborne A. Catalase activity. In: Greenwald RA, editor. Handbook of methods in oxygen radical research. Boca Raton (CO): CRC Press; 1986. pp. 283–284.
- Marklund S, Maruklund G. Involvement of the superoxide anion radical in the autoxidation of pyrogallol and a convenient assay for superoxide dismutase. Eur J Biochem. 1974;47:469–474.
- Mohandas M, Marshall JJ, Duggin GG, et al. Differential distribution of glutathione and glutathione-related enzymes in rabbit kidney: possible implications in analgesic nephropathy. Biochem Pharmacol. 1984;33(11):1801–1807.
- Adewole SA, Salako AA, Doherty OW, et al. Effect of melatonin on carbon tetrachloride-induced kidney injury in Wistar rats. Afric J Biomed Res. 2007;10:153–164.
- Upur H N, Amat B, Blazekovi AT. Protective effects of Cichorium glandulosum root extract on carbon tetrachloride-induced and galactosamine-induced hepatotoxicity in mice. Food Chem Toxicol. 2009;47:2022–2030.
- Ogunmoyole T, Awodooju M, Idowu S, et al. Phyllanthus amarus extract restored deranged biochemical parameters in rat model of hepatotoxicity and nephrotoxicity. Heliyon. 2020;6(12):e05670. DOI:10.1016/j.heliyon.2020.e05670
- Alrashood ST, Al-Asmari AK, Alotaibi AK, et al. Protective effect of lyophilized sapodilla (Manilkara zapota) fruit extract against CCl4-induced liver damage in rats. Saudi J Biol Sci. 2020;27(9):2373–2379. DOI:10.1016/j.sjbs.2020.05.010
- Soundharrajan I, Kim DH, Srisesharam S, et al. R-limonene enhances differentiation and 2-deoxy-D-glucose uptake in 3T3-L1 preadipocytes by activating the AKt signaling pathway. Evd Based Compl Alt Med. 2018;4573254:1–10.
- Jing L, Zhang Y, Fan S, et al. Preventive and ameliorating effects of citrus D-limonene on dyslipidemia and hyperglycemia in mice with high-fat diet-induced obesity. Eur J Pharmacol. 2013;715(1-3):46–55. DOI:10.1016/j.ejphar.2013.06.022
- Hijazi MA, Jambi HA, Aljehany BM, et al. Potential protective effect of Achillea fragrantissima against adriamycin-induced cardiotoxicity in rats via an antioxidant and anti-inflammatory pathway. Biomed Res Int. 2019;2019:5269074. DOI:10.1155/2019/5269074
- Liu X, Chen Z, Chua CC, et al. Melatonin as an effective protector against doxorubicin-induce cardiotoxicity. Am J Physiol Heart Circ Physiol. 2002;283:254–263.
- Younis NS. D-limonene mitigate myocardial injury in rats through MAPK/ERK/NF-κB pathway inhibition. Korean J Physiol Pharmacol. 2020;24(3):259–266. DOI:10.4196/kjpp.2020.24.3.259
- Wei A, Shibamoto T. Antioxidant activities and volatile constituents of various essential oils. J Agr Food Chem. 2007;55:1737–1742.
- Mair J. Cardiac troponin I and troponin T are enzymes still relevant as cardiac markers. Clin Chim Acta. 1997;257:99–115.
- Warings WS, Webb DJ, Maxwell SRJ. Uric acid as a risk for cardiovascular disease. O J Med. 2000;93:707–713.
- Adams JE, Abendschein DR, Jaffe AS. Biochemical markers of myocardial injury: Is MB creatine kinase choice for 1990s? Circulation. 1993;88:750–763.
- Al-Rasheed NM, Faddah LM, Mohamed AM, et al. Potential impact of silymarin in combination with chlorogenic acid and/or melatonin in combating cardiomyopathy induced by carbon tetrachloride. Saud J Biol Sci. 2014;21:265–274.
- Nascimento GAD, Souza DSD, Lima BS, et al. Bradycardic and antiarrhythmic effects of the D-limonene in rats. Arq Bras Cardiol. 2019;113(5):925–932.
- Poling J, Gajawada P, Richter M, et al. Therapeutic targeting of the oncostatin M receptor-β prevents inflammatory heart failure. Basic Res Cardiol. 2014;109:396.
- Calabro P, Willerson JT, Yeh ET. Inflammatory cytokines stimulated C-reactive protein production by human coronary artery smooth muscle cells. Circulation. 2003;108:1930–1932.
- Li CC, Hsiang CY, Wu SL, et al. Identification of novel mechanisms of silymarin on the carbon tetrachloride-induced liver fibrosis in mice by nuclear factor-κB bioluminescent imaging-guided transcriptomic analysis. Food Chem Toxicol. 2012;50(5):1568–1575.
- Patel DN, King CA, Bailey SR. Interleukin 17 stimulates C-reactive protein expression in hepatocytes and smooth muscle cells via p38 MAPK and ERKI/2-dependant NF-kappaB and C/EBPbeta activation. J Biol Chem. 2007;282:27229–27238.
- Frum Y, Viljoen AM. In vitro 5-lipoxygenase activity of three indigenous South African aromatic plants used in traditional healing and the stereospecific activity of limonene in the 5-lipoxygenase assay. J Ess Oil Res. 2006;18:85–88.
- Coballase-Urrutia E, Pedraza-Chaverri J, Cárdenas-Rodríguez N, et al. Acetonic and methanolic extracts of Heterotheca inuloides, and quercetin, decrease CCl(4)-oxidative stress in several rat tissues. Evid Based Complement Alternat Med. 2013: 659165. DOI:10.1155/2013/659165