ABSTRACT
Objectives
The pathogenesis of vitiligo remains unclear. In this review, we comprehensively describe the role of damage associated molecular patterns (DAMPs) during vitiligo pathogenesis.
Methods
Published papers on vitiligo, oxidative stress and DAMPs were collected and reviewed via database searching on PubMed, MEDLINE and Embase, etc.
Results
Oxidative stress may be an important inducer of vitiligo. At high oxidative stress levels, damage-associated molecular patterns (DAMPs) are released from keratinocytes or melanocytes in the skin and induce downstream immune responses during vitiligo. Treatment regimens targeting DAMPs can effectively improve disease severity.
Discussion
DAMPs play key roles in initiating host defenses against danger signals, deteriorating the condition of vitiligo. DAMP levels in serum and skin may be used as biomarkers to indicate vitiligo activity and prognosis. Targeted therapies, incorporating HMGB1, Hsp70, and IL-15 could significantly improve disease etiology. Thus, novel strategies could be identified for vitiligo treatment by targeting DAMPs.
1. Introduction
Vitiligo is an autoimmune disease and is characterized by chronic depigmentation and milk-white lesion in the skin, with a 1% prevalence rate in the general population [Citation1]. Although unaccompanied by distressing symptoms like pruritus or pain, vitiligo negatively affects the self-esteem and may cause anxiety or depression as it tends to occur in exposed skin areas [Citation2]. Vitiligo is currently considered a genetic susceptibility disorder [Citation3]; however, recent studies emphasized the important role of environmental factors in its etiology [Citation4]. From disease initiation, oxidative stress plays significant roles in promoting vitiligo onset [Citation5]. Also, epidermal melanocytes are particularly vulnerable to oxidative stress owing to their pro-oxidant status during melanin synthesis, resulting in melanocyte damage and self-antigen production [Citation6]. In recent years, several studies reported that under adverse stimulus conditions, such as oxidative stress, damage-associated molecular patterns (DAMPs) were released from cells and participated in autoimmune disease onset by inducing sterile inflammation, eventually leading to vitiligo() [Citation7].
Figure 1. Oxidative stress promotes damage-associated molecular pattern secretion, including HMGB1, HSP70, S100B, ATP, the interleukins, and AMPs. These molecules increase cytokine release and accelerate melanocyte death. Abbreviations: HMGB1, high mobility group box 1; HSP70, heat shock protein 70; ATP, adenosine triphosphate; AMPs, antimicrobial peptides. ROS, reactive oxygen species; ERK, extracellular regulated protein kinase; NF-κB, nuclear factor kappa-B; NLRP3, the NOD-like receptor thermal protein domain associated protein 3; pDC, plasmacytoid dendritic cells.
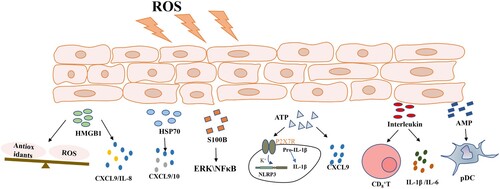
2. Increased skin-based oxidative stress levels in patients with vitiligo
Mediated by disturbed redox homeostasis, oxidative stress is characterized by imbalanced pro-oxidant and antioxidant levels [Citation8], and may be considered a crucial initiator of vitiligo [Citation9]. Reactive oxygen species (ROS) generation is an instant intracellular response when cells are exposed to an external environment [Citation10]. There are three major ROS: superoxide anion, hydrogen peroxide, and hydroxyl radical. Among the great variety of ROS, hydrogen peroxide (H2O2) has a pivotal role in the onset and progression of vitiligo. Excessive ROS production may also be simultaneously generated by melanogenesis and mitochondrial energy metabolism. Other than excessive ROS formation, aberrant ROS removal mechanisms may also account for increased ROS levels in the epidermis. Moreover, downregulated enzymatic or non-enzymatic defenses against oxidants in the epidermis, such as incapacitated catalase and glutathione peroxidase functions and decreased vitamin A and C levels also underpin ROS accumulation [Citation11,Citation12]. Another impaired antioxidant pathway in melanocytes and keratinocytes involves nuclear factor E2-related factor 2 (Nrf2)-antioxidant response element/heme oxygenase-1 (HO-1) activities [Citation13,Citation14].
ROS accumulation induces DNA damage and protein oxidation/fragmentation coupled with lipid peroxidation, thus reducing the function of these biological macromolecules [Citation15]. In recent years, it was reported that oxidative stress in vitiligo lesions promoted DAMP release from surrounding cells and contributed to vitiligo occurrence [Citation7].
3. DAMP characteristics
Danger sensing is a fundamental evolutionary feature enabling multicellular organisms to perceive potential threats, escape from dangerous situations, combat intruders, and repair physiological damage. Most molecules governing these processes are already present in cells (i.e. pre-formed DAMPs), therefore release is immediate, while others are neo-synthesized following injury. DAMPs, also known as alarmins, are endogenous and constitutively expressed proteins/peptides with immune-activating functions [Citation7]. Most DAMPs are passively released from dead cells, but some are actively secreted to indicate an early state of sub-lethal cell stress [Citation16]. In addition to physiological and homeostatic roles inside the cell, DAMPs also deliver, when exposed to the extracellular milieu, danger signals to the host, triggering local inflammatory responses [Citation17]. Apart from their roles in disease initiation, DAMPs also amplify and sustain inflammatory processes, with notable roles in the pathogenesis of inflammatory conditions [Citation18]. In addition to phagocyte activation and proinflammatory cytokine release, DAMPs are important links between the innate and adaptive immune systems, e.g. they activate immature dendritic cells (DCs) which process antigens. DAMPs present antigenic epitopes to naive T cells thereby inducing adaptive immune responses. It is largely accepted that DAMPs initiate immune responses by activating classical Pathogen Recognition Receptors (PRRs), which include not only toll-like receptors, but also multiple germline-encoded receptors, such as NOD-like receptors, retinoic acid-inducible gene I (RIG- I)-like receptors, C- type lectin receptors, and multiple intracellular DNA sensors [Citation19].
In healthy individuals, DAMPs exert important intracellular roles by regulating DNA transcription, calcium homeostasis, cell proliferation, and differentiation. However, high extracellular DAMP levels are present in several pathologies and are related to disease severity in autoimmune conditions and inflammatory disorders, such as sepsis, psoriasis, traumatic brain injury, acute lung injury, inflammatory bowel disease, and arthritis [Citation7,Citation18,Citation20]. In this review, we outline the relationships between different DAMPs and vitiligo pathogenesis.
4. Oxidative stress stimulates DAMP release and worsens vitiligo
As described, oxidative stress may be an important inducer of vitiligo. Also, melanocyte death in vitiligo skin is largely mediated by cytokines and chemokines [Citation4]. Meanwhile, a number of DAMPs are found at high levels extracellularly in vitiligo, enhancing inflammatory responses that contribute to disease progression. These DAMPs include high mobility group box 1, heat shock protein 70, S100B proteins, adenosine triphosphate, the IL family, antimicrobial peptides and other biomolecules. Interestingly, DAMPs appear to bridge the gap between oxidative stress and some proinflammatory factors.
4.1. High mobility group box 1 (HMGB1)
HMGB1 is a highly conserved nuclear protein found in all cells. It is a multi-faceted protein exerting functions both inside and outside cells [Citation21]. Intracellular HMGB1 regulates transcription repair and recombination by affecting chromosome structure [Citation22]. Extracellular HMGB1 is actively released from immune cells (i.e. monocytes or macrophages) after stimulation with lipopolysaccharide, pro-inflammatory cytokines, or nitric oxide, and is passively released from dead or dying cells [Citation22,Citation23]. Extracellular HMGB1 acts as an alarmin which binds to multiple cell-surface receptors to stimulate the innate immune system and trigger inflammatory responses. Signaling pathways activated by HMGB-1 induce nuclear factor kappa-B (NF-κB) phosphorylation, which in turn generates several cytokines and chemokines, such as tumor necrosis factor-α (TNF-α), interleukin-1β (IL-1β), IL-6, macrophage inflammatory protein-1α, and transforming growth factor-β in many cells, including endothelial cells, fibroblasts, macrophages, monocytes, T cells, and B cells [Citation24]. In fact, HMGB1 overexpression in pathological conditions, including septicemia, ischemia-reperfusion injury, arthritis, and cancers indicates key biological roles and clinical importance [Citation25,Citation26].
Vitiligo skin is in a state of high oxidative stress. Several studies reported that hydrogen peroxide (H2O2) stimulation promoted HMGB1 translocation and release from melanocytes. Furthermore, released HMGB1 inhibited the expression of Nrf2 and downstream antioxidant genes, aggravated oxidative stress, and induced melanocyte apoptosis. In fact, HMGB1 is overexpressed in blood samples and lesional specimens from patients with vitiligo [Citation27]. Cui et al. reported that HMGB1 was released from the melanocyte nucleus in vitiligo perilesional skin. Highly expressed HMGB1 also promoted CXCL16 and IL-8 secretion from keratinocytes by binding to the receptor for advanced glycation end products and activating NF-κB and extracellular signal-regulated kinase signal pathways, thus mediating the chemotactic formation of CD8+ T cell migration and accelerating melanocyte death [Citation27-29]. Additionally, HMGB1 promoted DC maturation in patients with vitiligo, contributing to oxidative stress-induced autoimmunity during the condition [Citation27] [Citation30]. In addition to melanocytes, oxidative stress also stimulates HMGB1 release from keratinocytes, drives melanocyte apoptosis and compensatory autophagy activation, and inhibits melanogenesis [Citation29]. In short, HMGB1 worsens vitiligo by impacting melanocyte survival.
4.2. Heat shock protein 70 (Hsp70)
Hsp70 chaperones have several cell housekeeping activities, including newly synthesized protein-folding, polypeptide translocation into mitochondria, chloroplasts and the endoplasmic reticulum, protein complex disassembly, and protein activity regulation. Furthermore, Hsp70s prevent aggregation and promote the refolding of misfolded and denatured proteins, they solubilize aggregated proteins, and cooperate with cell degradation machinery to remove aberrant proteins and aggregates. Therefore, Hsp70s act as sentinel chaperones, guarding cells from the deleterious effects of a wide range of proteotoxic stresses [Citation31]. Stressed cells halt mainstream protein synthesis in favor of Hsp and/or glucose-regulated protein synthesis pathways [Citation32]. However, in addition to protective roles, Hsp70 promotes antigen uptake, and processes inflammatory responses via the major histocompatibility complex class I pathway and conventional class II pathway in antigen-presenting cells (e.g. DCs) leading to T cell subset activation [Citation33]. Therefore, Hsp molecules exert cytoprotective effects. Once released into the extracellular environment, Hsp70 becomes an alarmin, induces immunity to secretory cells, and triggers pathological inflammatory diseases, such as type 1 diabetes, atherosclerosis, and rheumatoid arthritis, via immunomodulatory pathways [Citation34-36]. In recent years, several studies have focused on Hsp mechanisms during vitiligo.
Diffuse and intense Hsp70 expression patterns occur in vitiligo skin when compared with healthy skin. The Hsp70 nuclear form is expressed in progressive forms of vitiligo [Citation37,Citation38]. Furthermore, in a study using 4-tert-butylphenol as a stress model, melanocytes were very sensitive to environmental stress stimulation and stimulated PIG3V (human vitiligo melanocyte cell line) to secrete Hsp70 [Citation39]. In turn, Hsp70 induced membrane TNF-related apoptosis-inducing ligand expression and activated DC effector functions toward stressed melanocytes. In vitro studies revealed that the plasmacytoid dendritic cells (pDCs) expressing Hsp70 receptor Lox-1 (lectin-like oxidized low-density lipoprotein-receptor-1) aggregated Hsp70. Exogenous Hsp70 induced pDC activation and increased exogenous DNA uptake. Furthermore, Hsp70 potentiated DNA-induced interferon-α (IFN-α) production by pDCs, inducing CXCL9 and CXCL10 expression in keratinocytes and finally leading to melanocyte death [Citation40].
In the vitiligo model of depigmentation which was formed by gene gun vaccination, the inclusion of human and mouse-derived inducible Hsp70 (HSP70i) in the vaccination protocol increased and accelerated depigmentation processes and was accompanied by the induction of lasting humoral responses to Hsp70 [Citation41]. Jeffrey et al. reported that strong and lasting skin depigmentation was not induced in Hsp70i knockout mice, and that in vivo cytolytic assays showed no cytotoxic T-lymphocyte activity, and an absence of T-cell infiltration to the skin and hair follicle melanocyte maintenance. This study demonstrated that Hsp70i was necessary and sufficient to accelerate depigmentation in vitiligo-prone Pmel-1 mice, and was accompanied by lasting phenotypic changes in DC subpopulations [Citation42]. Moreover, research also showed that mutant Hsp70 reversed autoimmune depigmentation in vitiligo [Citation43]. And remarkable repigmentation following mutant Hsp70iQ435A-encoding DNA treatment. Also, repigmentation was accompanied by an initial influx of T cells accompanied by increased CD4/CD8 ratios. Importantly, treatment did not interfere with melanoma immunosurveillance. Therefore, Hsp70 proteins are potential vitiligo promoters [Citation44,Citation45].
4.3. S100B
S100B proteins are implicated in wide number of intracellular and extracellular functions, including apoptosis regulation, proliferation, differentiation, migration, invasion, energy metabolism, calcium ion homeostasis, protein phosphorylation, and inflammation [Citation46]. Some S100B proteins are also secreted and exert extracellular paracrine and autocrine functions [Citation46,Citation47]. After immune cell damage or activation, S100B proteins are released into the extracellular space where they regulate immune and inflammatory processes. They act as DAMP molecules to activate both immune and endothelial cells by binding to toll-like receptors and receptors for advanced-glycation end products [Citation48]. As some S100B proteins are easily identified in body fluids, they are used as biomarkers to detect specific diseases, where increased expression levels indicate pathological conditions [Citation49].
S100B is a DAMP protein expressed in melanocytes and is proposed as a marker of melanocyte cytotoxicity. Serum S100B levels, in patients with active non-segmental vitiligo, were significantly increased and correlated with affected body surface areas, suggesting its potential as a vitiligo biomarker [Citation50,Citation51]. In vitro studies using repeat freeze–thaw procedures identified intracellular S100B upregulation in normal and vitiligo melanocytes prior to its extensive release into the circulation. This phenomenon could explain increased S100B serum levels in the active phase of vitiligo [Citation52]. Once cells become damaged or necrotic, they secrete S100B into the circulation [Citation53]. Extracellular S100B then activates extracellular signal-regulated protein kinase (ERK) and NF-κB by binding to their cell surface receptors [Citation54]. In general, increased S100B levels are closely related to vitiligo activity, but their precise molecular mechanisms require further exploration.
4.4. Adenosine triphosphate (ATP)
ATP is a signal transmitter in non-adrenergic innervation stimulation, and its production is driven by the electron transport chain in the mitochondria [Citation55]. ATP produced by glycolysis and oxidative phosphorylation usually exists at very high cellular concentrations, creating strong outward gradients, including chemical and elevator gradients, on negatively charged plasma membranes [Citation56]. Extracellular ATP is a key DAMP molecule and is released into the extracellular medium during inflammation-induced injury to parenchymal cells, dying leukocytes, and activated platelets. Also, ATP directly activates the plasma membrane channel P2X7 receptor (P2X7R), leading to an intracellular influx of potassium ions, key triggers which activate the NOD-like receptor thermal protein domain associated protein 3(NLRP3) inflammasome. Simultaneously in the mitochondria, ROS and ATP production are coupled to regulate cellular redox reactions [Citation57]. In healthy tissue stroma, levels of ATP and its metabolite adenosine are negligible, while their accumulation is significantly increased in inflammatory or tumor microenvironments [Citation58].
Inflammasomes are key components of the host-defense system. They are essential inflammatory signaling platforms which detect injury mediators released during infection and tissue damage to activate inflammatory responses [Citation59]. Genome-wide association analysis of patients with vitiligo showed the inflammasome pathway was involved in vitiligo pathogenesis, and that ATP was the most important link during inflammasome activation [Citation59]. During vitiligo, the hyperoxidative stress state stimulates keratinocytes to release ATP outside the cell [Citation60,Citation61]. Treatment with ATP induces inflammasome and caspase-1 activation, and the production of active IL-1β and IL-18 forms via P2X7Rs in keratinocytes and melanocytes [Citation62] [Citation63-65]. High extracellular ATP levels also induce ROS production and cell death in melanocytes. Some vitiligo studies suggested that extracellular ATP, as a danger signal, activated the inflammasome pathway and increased the cutaneous chemotaxis of CD8 + T cells via CXCL9 [Citation59]. Therefore, targeting ATP-P2X7 signaling could be a potential strategy for treating vitiligo. In other studies, vitiligo epidermal melanocytes displayed impaired ATP production when compared with healthy melanocytes, which further affected melanocyte migration abilities and led to pigment regeneration disorder [Citation66,Citation67]. In short, ATP deteriorates vitiligo by activating inflammasomes.
4.5. The IL family
IL-15 expression in the epidermis and serum of patients with vitiligo was significantly higher when compared with healthy controls, and highly correlated with H2O2 levels [Citation68]. Oxidative stress promoted IL-15 and IL-15Rα expression, and also IL-15 trans-presentation by activating NF-κB signaling in keratinocytes, thereby contributing to effector memory T cell (CD8 + TEM) activation by IL-15-JAK-STAT signaling pathway [Citation69]. Additionally, IL-15 was important for tissue resident memory T cell (TRM) production in viral infections and cutaneous lymphomas [Citation70]. Moreover, IL-15 deficient-mice reportedly displayed impaired TRM formation, while IL-15 promoted TRM function ex vivo. Also, targeting IL-15 signaling using an anti-CD122 antibody reversed established vitiligo in mice. Short-term treatment with anti-CD122 also inhibited the TRM production of IFN-γ and long-term treatment depleted TRM from skin lesions [Citation71].
Th17 cells are a subset of CD4+ T cells and secrete a variety of immunomodulatory molecules, including IL-17, which is increasingly implicated in the pathogenesis of several immune-mediated diseases [Citation72]. Several studies reported that the frequency of peripheral blood Th17 cells and serum IL-17A levels in patients with vitiligo was higher than in healthy controls [Citation73,Citation74]. Vitiligo lesion biopsies also revealed Th17 cell infiltration [Citation75]. An in vitro analysis also showed that the expression of microphthalmia-associated transcription factor (MITF) and downstream genes was downregulated in melanocytes post IL-17A treatment [Citation76]. This treatment also induced morphological shrinking in melanocytes, resulting in decreased melanin production. In terms of the local cytokine network in the skin, IL-17A dramatically induced IL-1β, IL-6, and TNF-α production in skin-resident cells, such as keratinocytes and fibroblasts, to promote inflammation via a positive feedback loop [Citation76].
IL-33 and ST2 expression were both increased in lesional skin, with serum IL-33 levels increased in patients with vitiligo. Further research showed that IL-33 was secreted by keratinocytes and functioned as an alarmin [Citation77]. IL-33 also increased both IL-6 and TNF-α expression levels in primary keratinocytes and potentially induced melanocyte death by regulating cytokines in the cell microenvironment [Citation78].
4.6. Antimicrobial peptides (AMPs)
AMPs are a diverse group of small bioactive proteins which are part of the body’s first line of defense against pathogen activation. They function by disrupting bacterial membranes, modulating immune responses, and regulating inflammation [Citation79,Citation80]. Some mammals have multiple cathelicidin genes, but in humans, cathelicidin antimicrobial peptide is the only cathelicidin gene which encodes the 18-kDa proprotein hCAP18. LL37 is one form of a mature cathelicidin peptide derived from hCAP18 by enzymatic cleavage with kallikreins in the epidermis [Citation80]. LL37 modifies host immune responses, cell growth, migration, and differentiation [Citation81]. Recently, abnormal LL37 expression was identified in several diseases such as psoriasis, atopic dermatitis, and rosacea [Citation82,Citation83]. Clinically, some patients will develop vitiligo in injured skin. Previous research also showed that keratinocytes co-expressed IFN, LL37, and MAVS in skin wounds and chronic inflammatory disease. LL37 enabled keratinocytes to produce IFN in response to double-stranded RNA from dying cells, and also LL37 functioned through MAVS-dependent activation of TBK1-AKT-IRF3 signaling pathway, meanwhile, IFN secreted by activated keratinocytes promoted DC maturation [Citation84]. After interfering with LL37-DNA complexes, inflammation was significantly improved [Citation85]. In vitiligo, IFN influenced chemokine secretion by surrounding keratinocytes and further recruited T cells through a positive feedback pathway, resulting in melanocyte death [Citation86]. Atazadeh et al. reported that average blood LL37 levels in patients with vitiligo were significantly higher than in control groups, suggesting LL37 could be a potential threat during vitiligo; however, the mechanisms remain unclear [Citation87].
5. Conclusion
DAMPs and their receptors play key roles in initiating host defenses against danger signals from innate and adaptive immune responses, deteriorating the condition of vitiligo (). DAMP levels in serum and skin may be used as biomarkers to indicate vitiligo activity and prognosis. Because most DAMPs are expressed locally and directly released during tissue injury, targeted therapies, incorporating HMGB1, Hsp70, and IL-15 could significantly improve disease etiology in animal models with abnormal inflammatory and autoimmune responses. Thus, novel strategies could be identified for vitiligo treatment by targeting DAMPs.
Disclosure statement
No potential conflict of interest was reported by the author(s).
Additional information
Funding
References
- Ezzedine K, Eleftheriadou V, Whitton M, et al. Vitiligo. The Lancet. 2015;386(9988):74–84.
- Dabas G, Vinay K, Parsad D, et al. Psychological disturbances in patients with pigmentary disorders: a cross-sectional study. J Eur Acad Dermatol Venereol. 2020 Feb;34(2):392–399.
- Bergqvist C, Ezzedine K. Vitiligo: A focus on pathogenesis and its therapeutic implications. J Dermatol. 2021 Mar;48(3):252–270.
- Bergqvist C, Ezzedine K. Vitiligo: A Review. Dermatology. 2020;236(6):571–592.
- Xuan Y, Yang Y, Xiang L, et al. The Role of Oxidative Stress in the Pathogenesis of Vitiligo: A Culprit for Melanocyte Death. Oxid Med Cell Longev. 2022;2022:8498472.
- Li S, Zhu G, Yang Y, et al. Oxidative stress drives CD8(+) T-cell skin trafficking in patients with vitiligo through CXCL16 upregulation by activating the unfolded protein response in keratinocytes. J Allergy Clin Immunol. 2017 Jul;140(1):177–189. e9.
- Roh JS, Sohn DH. Damage-Associated Molecular Patterns in Inflammatory Diseases. Immune Netw. 2018 Aug;18(4):e27.
- Bertero E, Maack C. Calcium Signaling and Reactive Oxygen Species in Mitochondria. Circ Res. 2018 May 11;122(10):1460–1478.
- Mathachan SR, Khurana A, Gautam RK, et al. Does oxidative stress correlate with disease activity and severity in vitiligo? An analytical study. J Cosmet Dermatol. 2021 Jan;20(1):352–359.
- Huchzermeyer B, Menghani E, Khardia P, et al. Metabolic Pathway of Natural Antioxidants, Antioxidant Enzymes and ROS Providence. Antioxidants (Basel). 2022 Apr 11;11(4):761.
- Speeckaert R, Dugardin J, Lambert J, et al. Critical appraisal of the oxidative stress pathway in vitiligo: a systematic review and meta-analysis. J Eur Acad Dermatol Venereol. 2018 Jul;32(7):1089–1098.
- Huo J, Liu T, Huan Y, et al. Serum level of antioxidant vitamins and minerals in patients with vitiligo, a systematic review and meta-analysis. J Trace Elem Med Biol. 2020 Dec;62:126570.
- Liu S, Pi J, Zhang Q. Mathematical modeling reveals quantitative properties of KEAP1-NRF2 signaling. Redox Biol. 2021 Sep 22;47:102139.
- Qiu L, Song Z, Setaluri V. Oxidative stress and vitiligo: the Nrf2-ARE signaling connection. J Invest Dermatol. 2014 Aug;134(8):2074–2076.
- Zhou S, Zeng H, Huang J, et al. Epigenetic regulation of melanogenesis. Ageing Res Rev. 2021 Aug;69:101349.
- Zindel J, Kubes P. DAMPs, PAMPs, and LAMPs in Immunity and Sterile Inflammation. Annu Rev Pathol. 2020 Jan 24;15:493–518.
- Gong T, Liu L, Jiang W, et al. DAMP-sensing receptors in sterile inflammation and inflammatory diseases. Nat Rev Immunol. 2020 Feb;20(2):95–112.
- Yang HZ, Oppenheim JJ. Alarmins and immunity. Immunol Rev. 2017 Nov;280(1):41–56.
- Hudson BI, Lippman ME. Targeting RAGE Signaling in Inflammatory Disease. Annu Rev Med. 2018 Jan 29;69:349–364.
- Sarrand J, Baglione L, Parisis D, et al. The Involvement of Alarmins in the Pathogenesis of Sjogren's Syndrome. Int J Mol Sci. 2022 May 18;23(10):5671.
- Satoh TK. The role of HMGB1 in inflammatory skin diseases. J Dermatol Sci. 2022 Jul 13. doi:10.1016/j.jdermsci.2022.07.005.
- Andersson U, Tracey KJ, Yang H. Post-Translational Modification of HMGB1 Disulfide Bonds in Stimulating and Inhibiting Inflammation. Cells. 2021 Nov 26;10(12):3323.
- Murai S, Yamaguchi Y, Shirasaki Y, et al. A FRET biosensor for necroptosis uncovers two different modes of the release of DAMPs. Nat Commun. 2018 Oct 26;9(1):4457.
- Deng M, Tang Y, Li W, et al. The Endotoxin Delivery Protein HMGB1 Mediates Caspase-11-Dependent Lethality in Sepsis. Immunity. 2018 Oct 16;49(4):740–753. e7.
- Raucci A, Di Maggio S, Scavello F, et al. The Janus face of HMGB1 in heart disease: a necessary update. Cell Mol Life Sci. 2019 Jan;76(2):211–229.
- Kaur I, Behl T, Bungau S, et al. Exploring the therapeutic promise of targeting HMGB1 in rheumatoid arthritis. Life Sci. 2020 Oct 1;258:118164.
- Cui T, Zhang W, Li S, et al. Oxidative Stress-Induced HMGB1 Release from Melanocytes: A Paracrine Mechanism Underlying the Cutaneous Inflammation in Vitiligo. J Invest Dermatol. 2019 Oct;139(10):2174–2184. e4.
- Mou K, Liu W, Miao Y, et al. HMGB1 deficiency reduces H2 O2 -induced oxidative damage in human melanocytes via the Nrf2 pathway. J Cell Mol Med. 2018 Dec;22(12):6148–6156.
- Kim JY, Lee EJ, Seo J, et al. Impact of high-mobility group box 1 on melanocytic survival and its involvement in the pathogenesis of vitiligo. Br J Dermatol. 2017 Jun;176(6):1558–1568.
- Zhang K, Anumanthan G, Scheaffer S, et al. HMGB1/RAGE Mediates UVB-Induced Secretory Inflammatory Response and Resistance to Apoptosis in Human Melanocytes. J Invest Dermatol. 2019 Jan;139(1):202–212.
- Rosenzweig R, Nillegoda NB, Mayer MP, et al. The Hsp70 chaperone network. Nat Rev Mol Cell Biol. 2019 Nov;20(11):665–680.
- Pockley AG, Henderson B. Extracellular cell stress (heat shock) proteins-immune responses and disease: an overview. Philos Trans R Soc Lond B Biol Sci. 2018 Jan 19;373(1738):0522.
- Veglia F, Tyurin VA, Mohammadyani D, et al. Lipid bodies containing oxidatively truncated lipids block antigen cross-presentation by dendritic cells in cancer. Nat Commun. 2017 Dec 14;8(1):2122.
- Tukaj S. Heat Shock Protein 70 as a Double Agent Acting Inside and Outside the Cell: Insights into Autoimmunity. Int J Mol Sci. 2020 Jul 26;21(15):5298.
- Moin ASM, Nandakumar M, Diane A, et al. The Role of Heat Shock Proteins in Type 1 Diabetes. Front Immunol. 2020;11:612584.
- van Eden W, Jansen MAA, Ludwig IS, et al. Heat Shock Proteins Can Be Surrogate Autoantigens for Induction of Antigen Specific Therapeutic Tolerance in Rheumatoid Arthritis. Front Immunol. 2019;10:279.
- Abdou AG, Maraee AH, Reyad W. Immunohistochemical expression of heat shock protein 70 in vitiligo. Ann Diagn Pathol. 2013 Jun;17(3):245–249.
- Mosenson JA, Flood K, Klarquist J, et al. Preferential secretion of inducible HSP70 by vitiligo melanocytes under stress. Pigment Cell Melanoma Res. 2014 Mar;27(2):209–220.
- Hariharan V, Klarquist J, Reust MJ, et al. Monobenzyl ether of hydroquinone and 4-tertiary butyl phenol activate markedly different physiological responses in melanocytes: relevance to skin depigmentation. J Invest Dermatol. 2010 Jan;130(1):211–220.
- Jacquemin C, Rambert J, Guillet S, et al. Heat shock protein 70 potentiates interferon alpha production by plasmacytoid dendritic cells: relevance for cutaneous lupus and vitiligo pathogenesis. Br J Dermatol. 2017 Nov;177(5):1367–1375.
- Denman CJ, McCracken J, Hariharan V, et al. HSP70i accelerates depigmentation in a mouse model of autoimmune vitiligo. J Invest Dermatol. 2008 Aug;128(8):2041–2048.
- Mosenson JA, Zloza A, Klarquist J, et al. HSP70i is a critical component of the immune response leading to vitiligo. Pigment Cell Melanoma Res. 2012 Jan;25(1):88–98.
- Mosenson JA, Zloza A, Nieland JD, et al. Mutant HSP70 reverses autoimmune depigmentation in vitiligo. Sci Transl Med. 2013 Feb 27;5(174):174ra28.
- Henning SW, Fernandez MF, Mahon JP, et al. HSP70iQ435A-Encoding DNA Repigments Vitiligo Lesions in Sinclair Swine. J Invest Dermatol. 2018 Dec;138(12):2531–2539.
- Frisoli ML, Harris JE. Treatment with Modified Heat Shock Protein Repigments Vitiligo Lesions in Sinclair Swine. J Invest Dermatol. 2018 Dec;138(12):2505–2506.
- Gonzalez LL, Garrie K, Turner MD. Role of S100 proteins in health and disease. Biochim Biophys Acta Mol Cell Res. 2020 Jun;1867(6):118677.
- Gross SR, Sin CGT, Barraclough R, et al. Joining S100 proteins and migration: for better or for worse, in sickness and in health. Cell Mol Life Sci. 2013;71(9):1551–1579.
- Xia C, Braunstein Z, Toomey AC, et al. S100 Proteins As an Important Regulator of Macrophage Inflammation. Front Immunol. 2018;8:01908.
- Turnier JL, Fall N, Thornton S, et al. Urine S100 proteins as potential biomarkers of lupus nephritis activity. Arthritis Res Ther. 2017 Oct 24;19(1):242.
- Birlea SA. S100B: Correlation with Active Vitiligo Depigmentation. J Invest Dermatol. 2017 Jul;137(7):1408–1410.
- Shabaka FH, Rashed LA, Said M, et al. Sensitivity of serum S100B protein as a disease activity marker in Egyptian patients with vitiligo (case-control study). Arch Physiol Biochem. 2020 Mar 19;8:1–8.
- Speeckaert R, Voet S, Hoste E, et al. S100B Is a Potential Disease Activity Marker in Nonsegmental Vitiligo. J Invest Dermatol. 2017;137(7):1445–1453.
- Tomas-Velazquez A, Lopez-Gutierrez JC, Ceballos C, et al. S-100B serum protein is elevated in children with medium-to-giant congenital melanocytic nevi: An exploratory case-control study. J Am Acad Dermatol. 2020 Jul;83(1):222–224.
- Leclerc E, Fritz G, Weibel M, et al. S100B and S100A6 differentially modulate cell survival by interacting with distinct RAGE (receptor for advanced glycation end products) immunoglobulin domains. J Biol Chem. 2007 Oct 26;282(43):31317–31331.
- Vercellino I, Sazanov LA. The assembly, regulation and function of the mitochondrial respiratory chain. Nat Rev Mol Cell Biol. 2021 Oct 7;23(2):141–161.
- Giuliani AL, Sarti AC, Di Virgilio F. Extracellular nucleotides and nucleosides as signalling molecules. Immunol Lett. 2019 Jan;205:16–24.
- Savio LEB, Leite-Aguiar R, Alves VS, et al. Purinergic signaling in the modulation of redox biology. Redox Biol. 2021 Sep 22;47:102137.
- Di Virgilio F, Sarti AC, Falzoni S, et al. Extracellular ATP and P2 purinergic signalling in the tumour microenvironment. Nat Rev Cancer. 2018 Oct;18(10):601–618.
- Wang W, Hu D, Feng Y, et al. Paxillin mediates ATP-induced activation of P2X7 receptor and NLRP3 inflammasome. BMC Biol. 2020 Nov 26;18(1):182.
- Li Z, Gu Y, Wen R, et al. Lysosome exocytosis is involved in astrocyte ATP release after oxidative stress induced by H2O2. Neurosci Lett. 2019;705:251–258.
- Srisomboon Y, Ohkura N, Iijima K, et al. Airway Exposure to Polyethyleneimine Nanoparticles Induces Type 2 Immunity by a Mechanism Involving Oxidative Stress and ATP Release. Int J Mol Sci. 2021;22(16):9071.
- Di Virgilio F, Dal Ben D, Sarti AC, et al. The P2X7 Receptor in Infection and Inflammation. Immunity. 2017 Jul 18;47(1):15–31.
- Riteau N, Baron L, Villeret B, et al. ATP release and purinergic signaling: a common pathway for particle-mediated inflammasome activation. Cell Death Dis. 2012;3(10):e403–e403.
- Rogers C, Erkes DA, Nardone A, et al. Gasdermin pores permeabilize mitochondria to augment caspase-3 activation during apoptosis and inflammasome activation. Nat Commun. 2019 Apr 11;10(1):1689.
- Di A, Xiong S, Ye Z, et al. The TWIK2 Potassium Efflux Channel in Macrophages Mediates NLRP3 Inflammasome-Induced Inflammation. Immunity. 2018 Jul 17;49(1):56–65. e4.
- Dell'Anna ML, Ottaviani M, Kovacs D, et al. Energetic mitochondrial failing in vitiligo and possible rescue by cardiolipin. Sci Rep. 2017 Oct 20;7(1):13663.
- Xu P, Xue YN, Ji HH, et al. H2 O2 -induced oxidative stress disrupts mitochondrial functions and impairs migratory potential of human epidermal melanocytes. Exp Dermatol. 2020 Aug;29(8):733–741.
- Atwa MA, Ali SMM, Youssef N, et al. Elevated serum level of interleukin-15 in vitiligo patients and its correlation with disease severity but not activity. J Cosmet Dermatol. 2020;20(8):2640–2644.
- Chen X, Guo W, Chang Y, et al. Oxidative stress-induced IL-15 trans-presentation in keratinocytes contributes to CD8+ T cells activation via JAK-STAT pathway in vitiligo. Free Radical Biol Med. 2019;139:80–91.
- Adachi T, Kobayashi T, Sugihara E, et al. Hair follicle-derived IL-7 and IL-15 mediate skin-resident memory T cell homeostasis and lymphoma. Nat Med. 2015 Nov;21(11):1272–1279.
- <Antibody blockade of IL-15 signaling has the potential to durably reverse vitiligo..pdf>.
- Zhong Z, Su G, Kijlstra A, et al. Activation of the interleukin-23/interleukin-17 signalling pathway in autoinflammatory and autoimmune uveitis. Prog Retin Eye Res. 2021 Jan;80:100866.
- Speeckaert R, Speeckaert M, De Schepper S, et al. Biomarkers of disease activity in vitiligo: A systematic review. Autoimmun Rev. 2017 Sep;16(9):937–945.
- Acharya P, Mathur M. Interleukin-17 level in patients with vitiligo: A systematic review and meta-analysis. Australas J Dermatol. 2020 May;61(2):e208–e212.
- Bhardwaj S, Rani S, Kumaran MS, et al. Expression of Th17- and Treg-specific transcription factors in vitiligo patients. Int J Dermatol. 2020 Apr;59(4):474–481.
- Kotobuki Y, Tanemura A, Yang L, et al. Dysregulation of melanocyte function by Th17-related cytokines: significance of Th17 cell infiltration in autoimmune vitiligo vulgaris. Pigment Cell Melanoma Res. 2012 Mar;25(2):219–230.
- Li P, Ma H, Han D, et al. Interleukin-33 affects cytokine production by keratinocytes in vitiligo. Clinical & Experimental Dermatology. 2015;40(2):163–170.
- Vaccaro M, Cicero F, Mannucci C, et al. IL-33 circulating serum levels are increased in patients with non-segmental generalized vitiligo. Arch Dermatol Res. 2016;308(7):527–530.
- Magana M, Pushpanathan M, Santos AL, et al. The value of antimicrobial peptides in the age of resistance. Lancet Infect Dis. 2020;20(9):e216–e230.
- Gan BH, Gaynord J, Rowe SM, et al. The multifaceted nature of antimicrobial peptides: current synthetic chemistry approaches and future directions. Chem Soc Rev. 2021;50(13):7820–7880.
- Mookherjee N, Anderson MA, Haagsman HP, et al. Antimicrobial host defence peptides: functions and clinical potential. Nat Rev Drug Discov. 2020 May;19(5):311–332.
- Deng Z, Chen M, Liu Y, et al. A positive feedback loop between mTORC1 and cathelicidin promotes skin inflammation in rosacea. EMBO Mol Med. 2021 May 7;13(5):e13560.
- Nguyen HLT, Trujillo-Paez JV, Umehara Y, et al. Role of Antimicrobial Peptides in Skin Barrier Repair in Individuals with Atopic Dermatitis. Int J Mol Sci. 2020 Oct 14;21(20):7607.
- Zhang LJ, Sen GL, Ward NL, et al. Antimicrobial Peptide LL37 and MAVS Signaling Drive Interferon-beta Production by Epidermal Keratinocytes during Skin Injury. Immunity. 2016 Jul 19;45(1):119–130.
- Liang H, Yan Y, Wu J, et al. Topical nanoparticles interfering with the DNA-LL37 complex to alleviate psoriatic inflammation in mice and monkeys. Sci Adv. 2020 Jul;6(31):eabb5274.
- Frisoli ML, Essien K, Harris JE. Vitiligo: Mechanisms of Pathogenesis and Treatment. Annu Rev Immunol. 2020 Apr 26;38:621–648.
- Atazadeh F, Fazeli Z, Vahidnezhad H, et al. Increased level of cathelicidin (LL-37) in vitiligo: Possible pathway independent from vitamin D receptor gene polymorphism. Exp Dermatol. 2020 Dec;29(12):1176–1185.