ABSTRACT
Introduction
MARCKS protein, a protein kinase C (PKC) substrate, is known to be at the intersection of several intracellular signaling pathways and plays a pivotal role in cellular physiology. Unlike PKC inhibitors, MARCKS-targeting drug (BIO-11006) has shown early success in clinical trials involving lung diseases. Recent research investigations have identified two MARCKS-targeting peptides which possess multifaceted implications against asthma, cancer, inflammation, and lung diseases.
Areas covered
This review article provides the patent landscape and recent developments on peptides targeting MARCKS for therapeutic purposes. Online free open-access databases were used to fetch out the patent information, and research articles were fetched using PubMed.
Expert opinion
Research studies highlighting the intriguing role of MARCKS in human disease and physiology have dramatically increased in recent years. A similar increasing trend in the number of patents has also been observed related to the MARCKS-targeting peptides. Thus, there is a need to amalgamate and translate such a trend into therapeutic intervention. Our review article provides an overview of such recent advances, and we believe that our compilation will fetch the interest of researchers around the globe to develop MARCKS-targeting peptides in future for human diseases.
1. Introduction
Protein kinase C (PKC), a member of serine/threonine kinase family, is known to orchestrate a wide range of cellular processes, including cell growth, differentiation, and apoptosis, through the phosphorylation of its targeted proteins [Citation1]. The activation of PKC is triggered by the binding of diacylglycerol (DAG) and calcium ions to the C1 domain of the enzyme, leading to its translocation to the cell membrane. DAG is generated by the phospholipase C (PLC) pathway, which is triggered by the activation of G protein-coupled receptors (GPCRs) or tyrosine kinase receptors (RTKs) [Citation2]. The activation of PLC leads to the hydrolysis of phosphatidylinositol 4,5-bisphosphate (PIP2) into DAG and inositol 1,4,5-triphosphate (IP3), which triggers the release of calcium ions from the endoplasmic reticulum (ER) into the cytoplasm. The binding of DAG and calcium ions to PKC results in its activation, which triggers a cascade of intracellular signaling events that lead to the phosphorylation and activation of downstream targets [Citation3,Citation4]. A plethora of research investigations revealed PKC signaling to be highly deregulated in several pathophysiological conditions such as diabetes, neurological disorders, cardiovascular disease, autoimmune diseases, and cancer [Citation3–5].
Myristoylated Alanine-rich C-kinase Substrate (MARCKS), primarily discovered as PKC substrate, is a multifunctional scaffold protein that plays a significant role in various biological processes, including cell migration, membrane trafficking, and cytoskeletal organization [Citation6,Citation7]. MARCKS acts as a molecular switch that stabilizes the actin filament network at the leading edge of migrating cells, thereby promoting cell migration [Citation8,Citation9]. In the membrane trafficking process, MARCKS acts as a scaffold protein that brings together different effector proteins and lipids, thereby regulating the lipid-raft-dependent trafficking of receptors and other signaling molecules [Citation10]. In the event of cytoskeleton regulation, MARCKS binds to and stabilizes the actin filament network, thereby regulating the formation of actin-rich structures such as filopodia and stress fibers [Citation6,Citation11]. Studies have shown that phosphorylation of MARCKS by PKC leads to its displacement from the plasma membrane, thereby regulating its function in the cytoplasm. The phosphorylation of MARCKS by PKC also affects its ability to bind to actin filaments, leading to the regulation of actin polymerization and depolymerization. The role of PKC signaling in physiological and pathological processes has been extensively studied, and it is well established that the activation of PKC can result in both positive and negative effects, depending on the cellular context and the type of PKC isoform involved [Citation6,Citation12–14]. Although significant advancement and understanding have been developed about the therapeutic targeting of PKC, still it is rendered as an elusive target [Citation5]. Rather than considering PKC signaling alone as a therapeutic target, researchers now believe that its substrate protein MARCKS could be a lucrative option. As shown in , in the present review, we have highlighted the recent patent-based progress made related to MARCKS-targeting peptides as therapeutic options in human disease pathophysiology.
2. Peptides as a modality of drug discovery
Peptides are short chains of amino acids that are naturally occurring in the body and are known to be involved in various physiological processes. The major advantage of using peptides as drugs is their specificity, as peptides can target specific receptors or enzymes in the body and have a lower potential for off-target effects compared to small-molecule drugs [Citation15,Citation16]. Peptides also offer a lower toxicity profile due to their rapid metabolism and clearance from the body, which helps reduce the risk of long-term adverse effects [Citation17]. However, this characteristic property is sometimes considered as a limitation for peptide-based drug discovery. Several advancements have been postulated to overcome the rapid clearance or proteolytic degradation of peptide-based drugs, but these are beyond the scope of the present review.
Research investigations revealed that peptides can be designed to cross the blood–brain barrier, which is a significant advantage in the treatment of central nervous system disorders. Their ability to penetrate cell membrane and target intracellular proteins makes them a potent candidate for drug discovery [Citation17,Citation18]. Many peptides can traverse cell membranes due to their hydrophilic nature. For instance, some antimicrobial peptides are effective in killing bacteria, viruses, and fungi by targeting their intracellular components [Citation19]. Additionally, peptides can also be used as prodrugs, which are inactive compounds but can be transformed into active drugs after administration. This approach can be useful in increasing the half-life and stability of drugs, as well as improving their efficacy and safety. In addition, they can also be designed to mimic natural peptides/proteins, and can therefore be used to modulate biological processes in a manner that is similar to that of the natural peptide. Muttenthaler and colleagues have extensively reviewed examples of a few peptide-based drugs, including Humulin (insulin), Byetta (exenatide), and Ozempic (Semaglutide), all of which are approved for the treatment of type 2 diabetes [Citation20]. Other examples are Goserelin (Zoladex) and Leuprolide (Lupron), both are gonadotropin-releasing hormone agonists and approved for the treatment of breast and prostate cancer [Citation20].
3. Peptide-based targeting of MARCKS as a therapeutic modality in human disease pathophysiology
As shown in , MARCKS’ N-terminal myristoylated domain (NMD) and phosphorylation site domain (PSD; also known as effector domain, ED) are necessary for its membrane localization and activity. Based on this information, a cell-permeable peptide named MANS was designed and developed which targets NMD and exhibits efficacy against several human malignancies [Citation6,Citation21]. The MANS peptide comprises sequence similar to that of the first 24 amino acids of MARCKS protein. The presence of hydrophobic myristate moiety renders MANS peptide to be cell permeable. Interestingly, MANS inhibits the phosphorylation of MARCKS without affecting its expression. The research investigation on the efficacy of the MANS peptide led Biomarck Pharmaceuticals (North Carolina, U.S.A.) to develop its homolog BIO-11006, which contains the active site of MANS (the first 10 amino acids). BIO-11006 outperforms MANS as an anti-asthmatic and anti-cancer drug, presumably due to its high solubility and small size [Citation22,Citation23]. By reducing MARCKS phosphorylation, BIO-11006 considerably improved the overall response rate of patients receiving standard-of-care treatment as reported in a clinical study on lung cancer [Citation22]. Contrary to popular belief, research studies also revealed that peptides that target PSD are more effective against cancer cells than peptides that target the myristoylation domain [Citation6,Citation21]. Based on this concept, Chen et al designed and developed a 25-mer peptide named MPS which targets PSD of MARCKS (). This peptide has been reported to exhibit advanced anticancer activity through the downregulation of phosphorylated-MARCKS, phosphatidylinositol (3,4,5)-triphosphate, and AKT activity [Citation24]. Recent study further showed that MPS peptide exhibits its anticancer activity via targeting NF-ĸB signaling, pro-inflammatory cytokine production, and other factors [Citation25]. Lastly, Corradin et al designed and developed another highly basic 24-amino-acid peptide named 3DMRPED which they showed to target hydrolysis of MARCKS-related protein (MRP). The 3DMRPED peptide efficiently enters both macrophages and parasites comparable to that with an N-terminal HIV-1 Tat transduction domain (TD). Authors stated that such a peptide could be beneficial for Leishmania biology [Citation26]. In the following sections, we have described in detail the involvement of MARCKS protein in several human disease pathophysiology and how peptide-based targeting is a beneficial therapeutic option.
Figure 2. Schematic diagram showing MARCKS is associated with the plasma membrane and how its phosphorylation by PKC and/or RhoA leads to its dissociation. The diagram also depicts the three domains of MARCKS protein, wherein MANS peptide is known to target the NMD and MPS peptide is known to target PSD (ED). Here PSD means Phosphorylation site domain, ED means effector domain, Myr means Myristoylation, PKC means Protein kinase C, N– means N terminal, C- means C terminal, P means Phosphorylation.
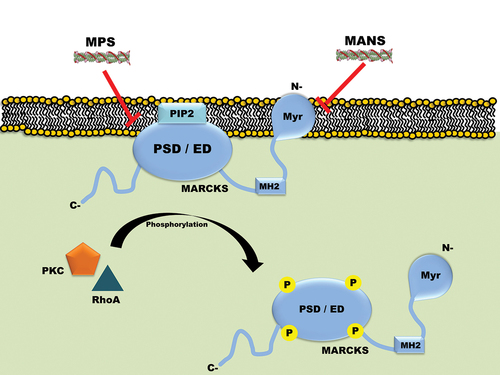
3.1. Role of MARCKS in asthma and inflammatory diseases
MARCKS is an evolutionarily conserved and universally expressed protein which has been linked to controlling cellular processes such as cellular adhesion, migration, and exocytosis that demand dynamic actin remodeling [Citation6]. Sheats et al have extensively reviewed the role of MARCKS in acute lung injury/acute respiratory distress syndrome, lung cancer, chronic obstructive pulmonary disease, and asthma [Citation13]. Other review reports suggest MARCKS as a ubiquitous protein, an important cellular factor responsible for controlling the release of airway mucin (the glycoprotein component of mucus) in both in vitro and in vivo studies [Citation27]. Moreover, the interaction of chaperone cysteine string protein (CSP) and heat shock protein 70 (HSP70) with MARCKS has been reported to be involved in mucin secretion. When either of the chaperones was inhibited, these cells released less mucin [Citation28,Citation29]. Recently, Liu et al found the role of MARCKS in regulating synaptic plasticity of Alzheimer’s disease brain tissue. The authors found that MARCKS regulated the structural and functional plasticity of dendritic spines, which are crucial for synaptic transmission and synaptic plasticity. They also demonstrated that MARCKS deficiency led to impaired learning and memory in mice [Citation30].
In another study, Li and colleagues reported MARCKS as a central regulatory molecule for mucin secretion in normal human bronchial epithelial (NHBE) cells. The activation of both PKC and PKG increases the secretion of mucin from these cells, and MARCKS acts as the point of convergence for coordinating the actions of these two protein kinases to regulate mucin granule release [Citation31]. Additionally, this study for the first time demonstrated the design and development of synthetic peptide MANS (myristoylated N-terminal sequence), the sequence of which was derived from the N-terminus region of MARCKS protein [Citation31]. Prior to the development of MANS peptide (before 2001), physiological aspects of MARCKS were dependent on the traditional RNAi method. Furthermore, the role of MARCKS in the secretion/exocytosis of mucin by the secretory cells of airway epithelium, such as neutrophils and mast cells, was also investigated using MANS peptide [Citation32]. A similar use of MANS peptide was shown by Lee et al, wherein they showed that MANS and other effector domain (ED) peptides were able to reduce the production of TNF-α and IL-6 produced by a lethal dose of LPS. Overall, their study demonstrated that MARCKS controls the expression of pro-inflammatory cytokines in macrophages, and peptides generated from MARCKS can be employed to reduce inflammatory reactions [Citation33]. Through these studies, it is presumed that MARCKS is a possible therapeutic target in airway mucin secretion, from COPD to airway inflammation, ARDS, and even NSCLC, or other lung diseases [Citation13]. Based on the success of MANS peptide in lung airway diseases, BioMarck Pharmaceuticals have developed an inhalable aerosol drug called BIO-11006, which is under clinical trials for several lung diseases [Citation13].
3.1.1. Evaluation of patent related to anti-asthmatic and anti-inflammatory application of MARCKS-targeting peptides
As mentioned in and , Parikh from Biomarck Pharmaceuticals obtained a patent for the use of MANS peptide as a therapeutic option for asthma in 2008 from several countries including the World Intellectual Property Organization (WIPO). Recently, it was granted U.S.A. patent status with advancements to the previous versions [Citation34]. Through this patent, the patentees aimed to investigate the role of MARCKS in the secretion of chemokines or cytokines from inflammatory cells. The results presented in the patent revealed that MARCKS plays a crucial role in the secretion of myeloperoxidase from neutrophils. Furthermore, the patentees intended to develop a peptide-based method for blocking the release of identified inflammatory mediators from their respective inflammatory cells. Such blocking of inflammatory mediators would be highly beneficial for asthma, COPD, and cystic fibrosis. To achieve this objective, the patentees assessed the active fragments within the 4–23 amino acid sequences of the entire MANS peptide. The patentees believe that their invention could serve as a dynamic model for developing MARCKS-targeting peptides in future. Based on their results, the patentees advocate that the MANS peptide and its active fragments can inhibit the degranulation process and myeloperoxidase secretion from isolated canine inflammatory leukocytes, which were stimulated by either 100 nM Phorbol-12-myristate-13-acetate (PMA) or 10 µM 8-Br-cGMP. They further demonstrated that MANS peptide can compete with the native MARCKS protein to attenuate the release of inflammatory mediators. The peptide can be administered topically, parenterally, rectally, orally, or via inhalation, and can be repeated at intervals to reduce inflammation [Citation34].
Figure 3. Schematic diagram showing patents filed and/or granted related to MANS and MPS peptides with their respective implications.
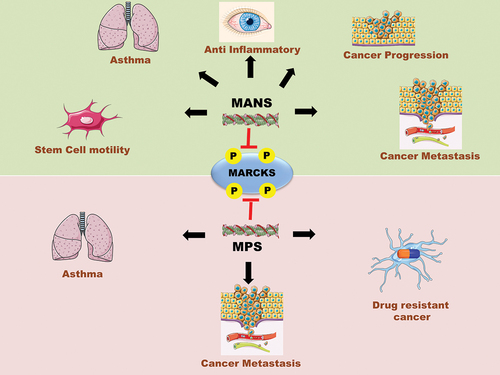
Table 1. List of patents filed or granted related to MANS and MPS peptides with their respective implications.
Wu et al have been granted a patent recently for the use of MPS peptide as a therapeutic option for asthma and other allergic inflammatory diseases [Citation35]. Patentees claim that MARCKS phosphorylation at Ser-159/163 is elevated in human asthmatic airway tissues and OVA-allergic mice model. Patentees claim that MPS peptide and its fragments have the capability to suppress airway hyper-reactivity and inflammatory signaling in the OVA-allergic mice model, as evaluated through invasive body plethysmography. In summary, the in vivo experimental setup involved 8-week-old Balb/c mice that were sensitized with Ova-Alum for 7–8 days. At 10–14 days, the mice were subsequently injected with MPS peptide (154 µg/mouse/day) or PBS each day, followed by OVA challenge at day 15–18 (each day). On the 19th day, the mice were sacrificed and lung/airway tissues were collected and stored for further staining. Masson-trichrome staining and H&E staining of the tissues revealed that MPS treatment suppresses mucus cell metaplasia in the airways of OVA-allergic mice. The suppression in inflammatory cell type was accompanied by decrease in Th2 cytokines (IL-4, IL-5, IL-13, eotaxin-1) and IgE secretion [Citation35]. Previous studies highlighted the critical role of IL-13 in IgE synthesis and airway hyper-responsiveness [Citation28,Citation29]. Since patentees observed enhanced IL-13 secretion in the mouse model, they conducted further investigations to assess the efficacy of the MPS peptide in suppressing the IL-13 signaling pathway in primary normal human bronchial epithelial cells (NHBE). After culturing NHBE cells for 14 days, patentees pretreated them with PBS, or MANS peptide, or MPS peptide, or mutant analogs of MPS peptide (A-MPS or D-MPS; 50 µM each) for 4 h before stimulating them with recombinant IL-13. Notably, the mutant analogs A-MPS and D-MPS peptide were derived by substituting all the serine residues of MPS peptide with either alanine residues or aspartate residues, respectively. The results of this experiment revealed an increase in phospho-STAT3 and phospho-MARCKS proteins, both of which were suppressed with MPS peptide treatment but not with MANS or mutant peptide treatment. This highlights the significance of serine residues in the MPS peptide for relieving asthma symptoms.
Similarly, Parikh and Bhatia from BioMarck Pharmaceuticals obtained a patent for the use of MANS derived peptide MPS and its fragments as an anti-inflammatory drug for the treatment of an ocular disease called uveitis [Citation36]. The plethora of literature suggests that uveitis is caused due to infectious and/or noninfectious reasons. Noninfectious uveitis is presumed to be due to inflammation and is typically treated with steroids or immunosuppressive agents. In their patent, the inventors developed uveitis model by injecting 10 ng of lipopolysaccharide (LPS) into the eye tissue of rabbits and analyzed its characteristics 24 h after injection. Since MARCKS is known to be involved in secretion of inflammatory mediators from infiltrating leukocytes, the patentees explored the efficacy of BIO-11006 (derivative of MPS peptide) in ameliorating LPS-induced uveitis in rabbits. BIO-11006 (100 µM in 50 µL solvent) was intravitreally injected into the rabbits using a regimen of 2 h pre- and post-LPS injection. Histopathological analysis and inflammatory index measurements reveal that BIO-11006 significantly reduces LPS-mediated inflammatory cell infiltration in aqueous humor of rabbits. The patentees further validated their findings with BIO-11006 in a rat model of chronic uveitis.
3.2. Role of MARCKS in cancer progression
The role of MARCKS in cancer has been extensively studied, with evidence indicating that it is involved in the regulation of cancer cell behavior. Iyer et al have extensively reviewed the role of aberrant MARCKS expression in hematological malignancies [Citation6]. In the past, several studies have shown that overexpression of MARCKS is associated with an increased risk of cancer progression and a poor prognosis in several types of cancer, including oral squamous cell carcinoma, breast, colorectal, glioma, lung, liver, and ovarian cancer [Citation6,Citation37–40]. However, in glioblastoma multiforme (GBM), clinical studies suggest that there exists an inverse correlation between MARCKS expression and survival rates. The high expression of MARCKS in GBM patients correlates with better patient survival [Citation41,Citation42]. These seemingly contradictory findings may be explained by the fact that phosphorylation of MARCKS, rather than its expression levels, is a decisive factor for clinical and biological effects. As discussed above, MARCKS can be phosphorylated by protein kinases, leading to changes in its localization, interactions with other proteins, and activity. This phosphorylation can have effects on various cellular processes that contribute to cancer progression, such as increased cell migration, invasion, and drug resistance [Citation6,Citation21]. Rao et al showed that elevated MARCKS phosphorylation increases the invasiveness and migratory potential of lung cancer cells in vitro and metastasis in vivo [Citation43]. This study is very well supported by previous clinical reports, which suggest that MARCKS phosphorylation in lung cancer is associated with advanced stage and lymph node metastases and related to reduced survival time in other cancer types [Citation24,Citation39,Citation44–46]. Additionally, HER2 subtype, inflammatory female breast cancer, and male breast cancer have been associated with MARCKS overexpression [Citation47,Citation48]. This association may be due to increased MARCKS binding to Tob, which reduces Tob’s ability to bind with ErbB2, ultimately leading to the activation of ErbB2 signaling [Citation49]. Similarly, a study involving the renal cell carcinoma (RCC) xenograft model suggests elevated MARCKS expression to contribute toward tumor growth and angiogenesis. Along with it, MARCKS phosphorylation is favorably linked with high tumor grade in RCC patients [Citation50]. Similar trends related to the role of MARCKS phosphorylation in regulating invasion and metastasis were observed in cholangiocarcinoma and melanoma cells [Citation8,Citation51].
Few in vitro studies revealed that RNA interference (RNAi)-based silencing of MARCKS inhibits migration and invasion of hepatocellular, colorectal, and melanoma cancer cells [Citation52–54]. Few other studies have demonstrated that MARCKS phosphorylation-inhibiting peptide effectively reduces cell growth, migration, and invasion of cultured cancer cells and lessens metastasis burden in both subcutaneous and orthotopic xenograft models [Citation8,Citation24,Citation25,Citation55,Citation56]. Our group demonstrated that MARCKS phosphorylation-inhibitory peptide MANS reduces WNT5A-mediated melanoma cell invasion, suggesting that it could be a probable anti-metastatic therapy for melanoma patients [Citation8]. Similarly, Chen et al showed MPS peptide to synergize regorafenib in inhibiting the growth and proliferation of RCC [Citation50].
There are strong pieces of evidence suggesting that the development of a cancer stem cell phenotype is responsible for chemotherapy resistance in cancer [Citation57–60]. Numerous studies have shown that the expression levels of MARCKS are associated with the response to chemotherapy and radiation in various cancer malignancies. Elevated levels of MARCKS have been reported in tamoxifen-resistant and paclitaxel-resistant breast cancer cells, oxaliplatin-resistant pancreatic cancer cells, BRAF inhibitor-resistant melanoma cells, and bortezomib-resistant leukemia cells in comparison to cells that are responsive to those treatments [Citation54,Citation61–64]. Moreover, quiescent stem/progenitor cells from chronic myelogenous leukemia had higher MARCKS transcript levels and pancreatic cancer stem cells have been found to release MARCKS protein [Citation65,Citation66].
It is preemptive to speculate that targeting MARCKS expression and/or phosphorylation could have a significant impact on managing cancer patients undergoing chemotherapy. Indeed, several recent studies have advocated such a statement. Chen and colleagues in their study showed that treatment of erlotinib-resistant lung cancer cells with MPS peptide enhances their sensitivity toward erlotinib [Citation24]. Similarly, another study showed that bosutinib-mediated perturbation in MARCKS phosphorylation reduces ABCB1 activity and renders colorectal cancer cells sensitive toward commonly used chemotherapy such as doxorubicin or 5-FU [Citation67]. Both studies highlight the significance of targeting MARCKS signaling as a therapeutic approach to combat cancer therapy resistance and/or stemness. Nevertheless, this does not hold true for gliomas, as overexpression of MARCKS is associated with better survival rates [Citation42]. Conclusively, MARCKS inhibition in drug-resistance seems to be a context-dependent approach and not applicable for all the cancer malignancies.
3.2.1. Evaluation of patents related to anti-cancer application of MARCKS-targeting peptides
As mentioned in and , Parikh & Adler from Biomarck Pharmaceuticals obtained a patent for the use of MANS peptide and its derivative as a therapeutic option for metastatic cancer in 2016 [Citation68]. Patentees further reclaimed their patent with modifications in 2022. The MANS peptide is a 24-amino acid residue peptide with the sequence MA-GAQFSKTAAKGEAAARPGEAAVA, where ‘MA’ represents Myristoylation. The patentees claimed to have designed a series of MANS-related peptides (231 in total) by acylating either the N-terminal or C-terminal of the MANS peptide. For acylating the N-terminus, various groups were used such as amide of trifluoroacetic acid, or benzoic acid, or aliphatic carboxylic acid, or aliphatic alkyl sulfonic acid. In some peptides, N-terminal amine group was alkylated with groups such as linear 2-oxyethyl group, omega-methoxy-ethyl group, and aliphatic alkyl group. The C-terminal amino acid of the peptides was modified using amide of the ammonia, or aliphatic alkyl amine, or omega-methoxy-ethylamine, or linear 2-oxyethylamine group. Additionally, the patentees claimed to have designed a few peptides by altering the C-terminal carboxylic acid of the amino acid at C-termini with an ester of aliphatic alkyl alcohol, or linear PEG-amine, or 2-(omega-methoxy-poly(ethyleneoxy)n)-ethanol group. Out of the 231 designed peptides, BIO-11000, BIO-11002, BIO-11006, BIO-10900, BIO-10901, BIO-11037, BIO-91200, were found to be superior in terms of inhibiting migration of non-small lung cancer cell line CL1–5 in a range of 50–100 µM, when administered for 12 h in a transwell-chamber-based assay. The patentees further stated that BIO-11000 (N-myristoyl-myristoyl-GAQFSKTAAKOH) and BIO-11006 (N-acetyl-GAQFSKTAAKOH) exhibited migration indices of 1.5 and 2, respectively, at 50 µM, advocating the latter peptide for further in vivo testing. To assess the anti-metastatic activity of the peptides in a mice xenograft model, the patentees pretreated PC-9 cells with 100 µM peptides for 4 h, followed by injecting them into the left lobe of the lungs of nude mice. After seven days of seeding the tumor cells, the mice were administered with 50 nMoles of MANS peptide, or BIO-11006 peptide or RNS peptide (control) for 25 days. The experimental results revealed significant reduction in metastatic nodule formation in distant organs of the mice treated with the MANS and BIO-11006 peptides. However, no difference in the average size of the tumor was observed at the injection site of mice treated with any peptide, suggesting that these treatments did not alter tumorigenesis. To optimize the clinical usage of BIO-11006 peptide, the patentees further formulated it as an aerosolized mixture and validated their findings using a lung and melanoma cancer xenograft model. Patentees claim that their aerosolized BIO-11006 is highly effective in inhibiting lung cancer metastasis compared to traditional intraperitoneal administration [Citation68].
Wu et al have been granted a patent in 2019 for the use of 25-mer MPS peptide (KKKKKRFSFKKSFKLSGFSFKKNKK) as a therapeutic option for advanced-stage and metastatic lung cancer [Citation69]. The rationale of the patent was based on previous studies which suggest that increased phosphorylation of MARCKS at Ser-159/163 is associated with lung cancer aggressiveness [Citation24,Citation55]. To evaluate the tumor growth inhibition capacity of the MPS peptide, the patentees administered tumor-bearing mice with 50 nMoles of MPS peptide, or RNS peptide (control) or MANS peptide every two days for 21 days. The results revealed that unlike MANS peptide, the MPS peptide was able to significantly reduce the tumorigenesis in mice. To assess the antimetastatic capacity of the MPS peptide, the patentees next developed a lung cancer mice model by orthotopically injecting the highly metastatic PC9 lung cancer cells into the left lobe of the mouse’s lungs. Four days after seeding the tumor cells, the mice were administered with 100 nMoles of MPS peptide, or RNS peptide (control) or PBS for a total of 10 injections over a period of 32 days. The results from the experiments showed that MPS peptide treatment significantly reduced the metastatic burden of lung cancer cells in distant organs compared to the control group. Additionally, the patentees further showed in an in vitro model that MARCKS signaling is at the juncture of crosstalk between the epidermal growth factor receptor (EGFR) and PI3K/AKT pathways. They further claim that erlotinib-resistant lung cancer cells harbor enhanced MARCKS phosphorylation, and MPS peptide sensitizes these cells toward erlotinib. Notably, MPS peptide does not show cytotoxicity toward normal human bronchial epithelial cells but only toward several lung cancer cells. Patentees claim that MPS peptide is comparatively better than MANS peptide, since it inhibits growth and invasiveness, induces apoptosis, and restores the sensitivity of drug-resistant cancer cells. Mechanistically, MPS peptide showed its anticancer effect in PTEN deficient and/or PIK3CA mutant cancer cells suggesting its use for cancer patients harboring enhanced PIP3 levels [Citation69].
4. Expert opinion
Over several years, PKC has been visualized as a lucrative anti-cancer drug target, unfortunately, none of the PKC inhibitors has shown clinical benefits [Citation70]. The failure of PKC inhibitors in clinical trials could be attributed to the presence of various PKC isoforms and their crosstalk with varied signaling molecules [Citation71]. All the preclinical studies related to PKC inhibitors are directed toward its convergent downstream targets, and MARCKS is one such prominent target. Excitingly, all the studies and patents related to MANS and MPS peptides discussed in the present review article strongly justify MARCKS phosphorylation as a promising therapeutic target in cancer and other inflammatory diseases. Fortunately, scientists at BioMarck pharmaceuticals are working on this aspect and their hard work in terms of BIO-11006 has entered the phase-II clinical trials for advanced-stage lung cancer, chronic obstructive pulmonary disease, and respiratory distress syndrome (NCT03472053, NCT00648245, NCT03202394; last assessed on www.clinicaltrials.gov on 31 March 2023). Notably, BioMarck pharmaceuticals have so far developed and formulated BIO-11006 as an aerosolized drug which limits its application only to lung diseases. In order to address drug resistance in blood malignancies and explore its applications in solid tumors, it is necessary to reformulate it for systemic circulation administration. Nano particle-based formulations and encapsulation hold great promise as a leading step in this regard. Unlike MARCKS phosphorylation inhibitory peptides, the potential of MARCKS-ED mimetic peptides has not been explored to date. In a glioblastoma-specific context, the MARCKS-ED mimetic peptide leads to instantaneous cytotoxicity probably mediated through calcium buildup and plasma membrane targeting [Citation72]. Although preexisting literature suggests that MARCKS mimetic peptides could have potential applications for several other human malignancies, not many advances have been made in this aspect. To the best of our knowledge, we could not find any patent or clinical trial of MARCKS ED mimetic peptides.
Among recent patents related to MARCKS-targeting peptides, there has been limited progress regarding one specific patent that claims the application of MANS peptide in modulating the mesenchymal stem cell motility [Citation73]. This patent claims that MARCKS is expressed in mouse bone marrow mesenchymal stem cells (BM-MSCs) and exposure of these cells to chemokines phosphorylates MARCKS (). Patentees further claimed that MANS peptide treatment inhibits migration or chemotaxis of BM-MSCs without inducing cytotoxicity [Citation73]. Although this patent was granted in 2011, no advancements have been made since then in relation to its therapeutic or other implications. This conclusion is based on the extensive scrutinization of databases related to clinical trials and patents. Excitingly, a relatively high number of peptide-based drugs have been approved by the FDA in the last five years (15 out of 208) [Citation74]. Based on these numbers and the clinical success of MARCKS-targeting peptides, we can be highly optimistic that more small molecules and/or peptides targeting MARCKS phosphorylation will be translated from the laboratory to clinical settings.
Article highlights
MARCKS protein is a critical mediator of cellular signaling and physiology.
MARCKS protein is known to play a decisive role in lung injury, asthma, inflammation, and metastatic cancer.
Recent patents have identified MANS and MPS as MARCKS-targeting peptides with therapeutic efficacy against asthma, uveitis, and metastatic lung cancer.
MANS peptide, MPS peptide, and their fragments have been shown to inhibit the phosphorylation of MARCKS without affecting its total expression.
Treatment with MPS peptide has been shown to sensitize erlotinib-resistant cancer cells toward erlotinib.
MPS peptide-derived drug BIO-11006 has shown clinical efficacy (Phase II) in reducing lung metastasis of Ewing's sarcoma and Osteosarcoma; overproduction of mucus and inflammation in COPD patients.
Declaration of interests
The authors have no relevant affiliations or financial involvement with any organization or entity with a financial interest in or financial conflict with the subject matter or materials discussed in the manuscript. This includes employment, consultancies, honoraria, stock ownership or options, expert testimony, grants or patents received or pending, or royalties.
Reviewer disclosures
Peer reviewers on this manuscript have no relevant financial or other relationships to disclose.
Author contribution statement
V Yadav visualized the current idea and gathered patent-related information. AK Sharma, G Parashar, NC Parashar, and S Ramniwas contributed toward the drafting and finalization of the manuscript. MK Jena, HS Tuli, K Yadav contributed toward the literature survey. V Yadav and HS Tuli supervised and contributed to the final framework of the article. V Yadav created the figures. V Yadav and MK Jena contributed to the manuscript revisions. All authors approved the final version of the manuscript.
Additional information
Funding
References
- Aslam N, Alvi F. Protein kinase C life cycle: explained through systems biology approach. Front Physiol. 2022;13:818688. doi: 10.3389/fphys.2022.818688
- Newton AC. Protein kinase C as a tumor suppressor. Semin Cancer Biol. 2018;48:18–26. doi: 10.1016/j.semcancer.2017.04.017
- Nishizuka Y. Membrane phospholipid degradation and protein kinase C for cell signalling. Neurosci Res. 1992;15(1–2):3–5. doi: 10.1016/0168-0102(92)90013-3
- Kikkawa U. The story of PKC: A discovery marked by unexpected twists and turns. IUBMB Life. 2019;71(6):697–705. doi: 10.1002/iub.1963
- Mochly-Rosen D, Das K, Grimes KV. Protein kinase C, an elusive therapeutic target? Nat Rev Drug Discov. 2012;11(12):937–957. doi: 10.1038/nrd3871
- Iyer DN, Faruq O, Zhang L, et al. Pathophysiological roles of myristoylated alanine-rich C-kinase substrate (MARCKS) in hematological malignancies. Biomark Res. 2021;9(1):34. doi: 10.1186/s40364-021-00286-9
- Fong LWR, Yang DC, Chen CH. Myristoylated alanine-rich C kinase substrate (MARCKS): a multirole signaling protein in cancers. Cancer Metastasis Rev. 2017;36(4):737–747. doi: 10.1007/s10555-017-9709-6
- Mohapatra P, Yadav V, Toftdahl M, et al. Wnt5A-Induced activation of the protein kinase C substrate MARCKS is required for melanoma cell invasion. Cancers (Basel). 2020;12(2):346. doi: 10.3390/cancers12020346
- Iioka H, Ueno N, Kinoshita N. Essential role of MARCKS in cortical actin dynamics during gastrulation movements. J Cell Bio. 2004;164(2):169–174. doi: 10.1083/jcb.200310027
- Yamaguchi H, Shiraishi M, Fukami K, et al. MARCKS regulates lamellipodia formation induced by IGF-I via association with PIP2 and beta-actin at membrane microdomains. J Cell Physiol. 2009;220(3):748–755. doi: 10.1002/jcp.21822
- Eckert RE, Neuder LE, Park J, et al. Myristoylated alanine-rich C-kinase substrate (MARCKS) protein regulation of human neutrophil migration. Am J Respir Cell Mol Biol. 2010;42(5):586–594. doi: 10.1165/rcmb.2008-0394OC
- Brudvig JJ, Weimer JM. X MARCKS the spot: myristoylated alanine-rich C kinase substrate in neuronal function and disease. Front Cell Neurosci. 2015;9:407. doi: 10.3389/fncel.2015.00407
- Sheats MK, Sung EJ, Adler KB, et al. In Vitro Neutrophil migration requires protein kinase C-Delta (δ-PKC)-Mediated Myristoylated Alanine-Rich C-Kinase Substrate (MARCKS) phosphorylation. Inflammation. 2015;38(3):1126–1141. doi: 10.1007/s10753-014-0078-9
- Amri ME, Fitzgerald U, Schlosser G. MARCKS and MARCKS-like proteins in development and regeneration. J Biomed Sci. 2018;25(1):43. doi: 10.1186/s12929-018-0445-1
- Yadav V, Jobe N, Mehdawi L, et al. Targeting oncogenic WNT signalling with WNT signalling-derived peptides. Handb Exp Pharmacol. 2021;269:279–303.
- Wang L, Wang N, Zhang W, et al. Therapeutic peptides: current applications and future directions. Signal Transduct Target Ther. 2022;7(1):48. doi: 10.1038/s41392-022-00904-4
- Lau JL, Dunn MK. Therapeutic peptides: historical perspectives, current development trends, and future directions. Bioorg Med Chem. 2018;26(10):2700–2707. doi: 10.1016/j.bmc.2017.06.052
- Parrasia S, Szabò I, Zoratti M, et al. Peptides as pharmacological carriers to the brain: promises, shortcomings and challenges. Mol Pharm. 2022;19(11):3700–3729. doi: 10.1021/acs.molpharmaceut.2c00523
- Yadav V, Misra R. A review emphasizing on utility of heptad repeat sequence as a tool to design pharmacologically safe peptide-based antibiotics. Biochimie. 2021;191:126–139. doi: 10.1016/j.biochi.2021.09.001
- Muttenthaler M, King GF, Adams DJ, et al. Trends in peptide drug discovery. Nat Rev Drug Discov. 2021;20(4):309–325. doi: 10.1038/s41573-020-00135-8
- Chiu C-L, Zhao H, Chen C-H, et al. The role of MARCKS in metastasis and treatment resistance of solid tumors. Cancers (Basel). 2022;14(19):4925. doi: 10.3390/cancers14194925
- Yin Q, Fang S, Park J, et al. An inhaled inhibitor of myristoylated alanine-rich C kinase substrate reverses LPS-Induced acute lung injury in mice. Am J Respir Cell Mol Biol. 2016;55(5):617–622. doi: 10.1165/rcmb.2016-0236RC
- Agrawal A, Rengarajan S, Adler KB, et al. Inhibition of mucin secretion with MARCKS-related peptide improves airway obstruction in a mouse model of asthma. J App Physiol. 2007;102(1):399–405. doi: 10.1152/japplphysiol.00630.2006
- Chen CH, Statt S, Chiu CL, et al. Targeting myristoylated alanine-rich C kinase substrate phosphorylation site domain in lung cancer. Mechanisms and therapeutic implications. Am J Respir Crit Care Med. 2014;190(10):1127–1138. doi: 10.1164/rccm.201408-1505OC
- Liu J, Chen SJ, Hsu SW, et al. MARCKS cooperates with NKAP to activate NF-Kb signaling in smoke-related lung cancer. Theranostics. 2021;11(9):4122–4136. doi: 10.7150/thno.53558
- Corradin S, Ransijn A, Corradin G, et al. Novel peptide inhibitors of Leishmania gp63 based on the cleavage site of MARCKS (myristoylated alanine-rich C kinase substrate)-related protein. Biochem J. 2002;367(Pt 3):761–769. doi: 10.1042/bj20020386
- Green TD, Crews AL, Park J, et al. Regulation of mucin secretion and inflammation in asthma: a role for MARCKS protein? Biochim Biophys Acta. 2011;1810(11):1110–1113. doi: 10.1016/j.bbagen.2011.01.009
- Park J, Fang S, Crews AL, et al. MARCKS regulation of mucin secretion by airway epithelium in vitro: interaction with chaperones. Am J Respir Cell Mol Biol. 2008;39(1):68–76. doi: 10.1165/rcmb.2007-0139OC
- Fang S, Crews AL, Chen W, et al. MARCKS and HSP70 interactions regulate mucin secretion by human airway epithelial cells in vitro. Am J Physiol Lung Cell Mol Physiol. 2013;304(8):L511–8. doi: 10.1152/ajplung.00337.2012
- Yu QS, Feng WQ, Shi LL, et al. Integrated analysis of cortex single-cell transcriptome and serum proteome reveals the novel biomarkers in alzheimer’s disease. Brain Sci. 2022;12(8):1022. doi: 10.3390/brainsci12081022
- Li Y, Martin LD, Spizz G, et al. MARCKS protein is a key molecule regulating mucin secretion by human airway epithelial cells in vitro. J Biol Chem. 2001;276(44):40982–40990. doi: 10.1074/jbc.M105614200
- Takashi S, Park J, Fang S, et al. A peptide against the N-terminus of myristoylated alanine-rich C kinase substrate inhibits degranulation of human leukocytes in vitro. Am J Respir Cell Mol Biol. 2006;34(6):647–652. doi: 10.1165/rcmb.2006-0030RC
- Lee SM, Suk K, Lee WH. Myristoylated alanine-rich C kinase substrate (MARCKS) regulates the expression of proinflammatory cytokines in macrophages through activation of p38/JNK MAPK and NF-κB. Cell Immunol. 2015;296(2):115–121. doi: 10.1016/j.cellimm.2015.04.004
- Biomarck Pharmaceuticals Ltd. US20210260154A1. 2021.
- University of California. US20200206308A1. 2021
- Biomarck Pharmaceuticals Ltd. US20220305078A1. 2021
- Li C, Xia R, Xue H, et al. Overexpression of MARCKS indicates a poor prognosis of oral squamous cell carcinoma. Oncol Lett. 2018;16(4):5498–5504. doi: 10.3892/ol.2018.9311
- Manai M, ELBini-Dhouib I, Finetti P, et al. MARCKS as a potential therapeutic target in inflammatory breast cancer. Cells. 2022;11(18):2926. doi: 10.3390/cells11182926
- Hanada S, Kakehashi A, Nishiyama N, et al. Myristoylated alanine-rich C-kinase substrate as a prognostic biomarker in human primary lung squamous cell carcinoma. Cancer Biomark. 2013;13(4):289–298. doi: 10.3233/CBM-130354
- Doghri R, Manai M, Finetti P, et al. Stromal expression of MARCKS protein in ovarian carcinomas has unfavorable prognostic value. Int J Mol Sci. 2017;19(1):41. doi: 10.3390/ijms19010041
- Micallef J, Taccone M, Mukherjee J, et al. Epidermal growth factor receptor variant III-induced glioma invasion is mediated through myristoylated alanine-rich protein kinase C substrate overexpression. Cancer Res. 2009;69(19):7548–7556. doi: 10.1158/0008-5472.CAN-08-4783
- Jarboe JS, Anderson JC, Duarte CW, et al. MARCKS regulates growth and radiation sensitivity and is a novel prognostic factor for glioma. Clin Cancer Res. 2012;18(11):3030–3041. doi: 10.1158/1078-0432.CCR-11-3091
- Rao G, Pierobon M, Kim IK, et al. Inhibition of AKT1 signaling promotes invasion and metastasis of non-small cell lung cancer cells with K-RAS or EGFR mutations. Sci Rep. 2017;7(1):7066. doi: 10.1038/s41598-017-06128-9
- Quan R, Ning Z, Wang Y, et al. Prognostic Value of Upregulation of Myristoylated Alanine-Rich C-Kinase Substrate in Gastric Cancer. Med Sci Monit. 2019;25:279–287. doi: 10.12659/MSM.913558
- Lu H, Zhao R, Qin Q, et al. MARCKS is a New Prognostic Biomarker in Hepatocellular Carcinoma. Int J Gen Med. 2023;16:1603–1619. doi: 10.2147/IJGM.S408651
- Button E, Shapland C, Lawson D. Actin, its associated proteins and metastasis. Cell Motil Cytoskeleton. 1995;30(4):247–251. doi: 10.1002/cm.970300402
- Manai M, Thomassin-Piana J, Gamoudi A, et al. MARCKS protein overexpression in inflammatory breast cancer. Oncotarget. 2017;8(4):6246–6257. doi: 10.18632/oncotarget.14057
- Manai M, Abdeljaoued S, Goucha A, et al. MARCKS protein overexpression is associated with poor prognosis in male breast cancer. Cancer Biomark. 2019;26(4):513–522. doi: 10.3233/CBM-190637
- Cho SJ, La M, Ahn JK, et al. Tob-mediated cross-talk between MARCKS phosphorylation and ErbB-2 activation. Biochem Biophys Res Commun. 2001;283(2):273–277. doi: 10.1006/bbrc.2001.4773
- Chen C-H, Fong LWR, Yu E, et al. Upregulation of MARCKS in kidney cancer and its potential as a therapeutic target. Oncogene. 2017;36(25):3588–3598. doi: 10.1038/onc.2016.510
- Techasen A, Loilome W, Namwat N, et al. Myristoylated alanine-rich C kinase substrate phosphorylation promotes cholangiocarcinoma cell migration and metastasis via the protein kinase C-dependent pathway. Cancer Sci. 2010;101(3):658–665. doi: 10.1111/j.1349-7006.2009.01427.x
- Rombouts K, Carloni V, Mello T, et al. Myristoylated Alanine-Rich protein Kinase C Substrate (MARCKS) expression modulates the metastatic phenotype in human and murine colon carcinoma in vitro and in vivo. Cancer Lett. 2013;333(2):244–252. doi: 10.1016/j.canlet.2013.01.040
- Song J, Wang Q, Luo Y, et al. MiR-34c-3p inhibits cell proliferation, migration and invasion of hepatocellular carcinoma by targeting MARCKS. Int J Clin Exp Pathol. 2015;8(10):12728–12737.
- Yadav V, Jobe N, Satapathy SR, et al. Increased MARCKS activity in BRAF inhibitor-resistant melanoma cells is essential for their enhanced metastatic behavior independent of elevated WNT5A and IL-6 signaling. Cancers (Basel). 2022;14(24):6077. doi: 10.3390/cancers14246077
- Chen CH, Thai P, Yoneda K, et al. A peptide that inhibits function of Myristoylated Alanine-Rich C Kinase Substrate (MARCKS) reduces lung cancer metastasis. Oncogene. 2014;33(28):3696–3706. doi: 10.1038/onc.2013.336
- Rohrbach TD, Shah N, Jackson WP, et al. The effector domain of MARCKS is a nuclear localization signal that regulates cellular PIP2 levels and nuclear PIP2 localization. Plos One. 2015;10(10):e0140870. doi: 10.1371/journal.pone.0140870
- Chatterjee N, Bivona TG. Polytherapy and targeted cancer drug resistance. Trends Cancer. 2019;5(3):170–182. doi: 10.1016/j.trecan.2019.02.003
- Dianat-Moghadam H, Heidarifard M, Jahanban-Esfahlan R, et al. Cancer stem cells-emanated therapy resistance: implications for liposomal drug delivery systems. J Control Release. 2018;288:62–83. doi: 10.1016/j.jconrel.2018.08.043
- Huang T, Song X, Xu D, et al. Stem cell programs in cancer initiation, progression, and therapy resistance. Theranostics. 2020;10(19):8721–8743. doi: 10.7150/thno.41648
- Pattabiraman DR, Weinberg RA. Tackling the cancer stem cells - what challenges do they pose? Nat Rev Drug Discov. 2014;13(7):497–512. doi: 10.1038/nrd4253
- Browne BC, Hochgraffe F, Wu J, et al. Global characterization of signalling networks associated with tamoxifen resistance in breast cancer. FEBS J. 2013;280(21):5237–5257. doi: 10.1111/febs.12441
- Chen CH, Cheng CT, Yuan Y, et al. Elevated MARCKS phosphorylation contributes to unresponsiveness of breast cancer to paclitaxel treatment. Oncotarget. 2015;6(17):15194–15208. doi: 10.18632/oncotarget.3827
- Kim YE, Kim EK, Song MJ, et al. SILAC-Based quantitative proteomic analysis of oxaliplatin-resistant pancreatic cancer cells. Cancers (Basel). 2021;13(4):724. doi: 10.3390/cancers13040724
- Muguruma Y, Yahata T, Warita T, et al. Jagged1-induced notch activation contributes to the acquisition of bortezomib resistance in myeloma cells. Blood Cancer J. 2017;7(12):650. doi: 10.1038/s41408-017-0001-3
- Gerber JM, Gucwa JL, Esopi D, et al. Genome-wide comparison of the transcriptomes of highly enriched normal and chronic myeloid leukemia stem and progenitor cell populations. Oncotarget. 2013;4(5):715–728. doi: 10.18632/oncotarget.990
- Brandi J, Pozza ED, Dando I, et al. Secretome protein signature of human pancreatic cancer stem-like cells. J Proteomics. 2016;136:1–12. doi: 10.1016/j.jprot.2016.01.017
- Wenzel T, Büch T, Urban N, et al. Restoration of MARCKS enhances chemosensitivity in cancer. J Cancer Res Clin Oncol. 2020;146(4):843–858. doi: 10.1007/s00432-020-03149-2
- North Carolina State University, Biomarck Pharmaceuticals Ltd. US11466054B2. 2022.
- University of California. US10189881B2. 2019.
- Kawano T, Inokuchi J, Eto M, et al. Activators and inhibitors of Protein Kinase C (PKC): their applications in clinical trials. Pharmaceutics. 2021;13(11):1748. doi: 10.3390/pharmaceutics13111748
- Mackay HJ, Twelves CJ. Targeting the protein kinase C family: are we there yet? Nat Rev Cancer. 2007;7(7):554–562. doi: 10.1038/nrc2168
- Eustace NJ, Anderson JC, Warram JM, et al. A cell-penetrating MARCKS mimetic selectively triggers cytolytic death in glioblastoma. Oncogene. 2020;39(46):6961–6974. doi: 10.1038/s41388-020-01511-9
- North Carolina State University, Biomarck Pharmaceuticals Ltd. WO2011060349A1. 2011.
- de la Torre BG, Albericio F. The pharmaceutical industry in 2021. An analysis of FDA drug approvals from the perspective of molecules. Molecules. 2022;27(3):1075. doi: 10.3390/molecules27031075