ABSTRACT
Introduction
β-Lactams, which include monobactams, remain the most important class of antibiotics worldwide. Aztreonam, the only monobactam in clinical use, has remarkable activity against many Gram-negative bacteria, but limited activity against some of the most problematic multidrug-resistant (MDR) pathogens, such as MDR Pseudomonas aeruginosa and Acinetobacter baumannii co-expressing extended-spectrum- and metallo-β-lactamases, which can inactivate aztreonam by hydrolysis.
Areas covered
Structurally novel siderophore-conjugated aztreonam derivatives with improved antibacterial properties against several high-priority pathogens are claimed. This invention reports that sidechain extension of aztreonam is tolerated; the coupling of its aminothiazoloxime carboxylic acid part with a siderophore mimetic significantly improved the antibacterial activity against several problematic strains, including MDR A. baumannii isolates with carbapenemase/cephalosporinase activity.
Expert Opinion
Finding new strategies to tackle bacterial resistance to β-lactam antibiotics is critical. Considering that β lactams are validated and safe drugs, this research may stimulate the field to develop new ideas in the arena of antimicrobial drug discovery, particularly with respect to siderophore mimetics.
1. Introduction
The lack of clinically useful antibacterial chemotherapies for treatment of infections caused by multi-drug resistant (MDR) strains of Pseudomonas aeruginosa and Acinetobacter baumannii, which are often associated with life-threatening hospital-acquired infections, pose a significant concern to public health [Citation1–3]. Despite success initially afforded by the continuous chemical modifications of β-lactams (which remain the most commonly prescribed class of antibiotics worldwide) in creating successive new generations of highly optimized β-lactams (e.g. 4th/5th generation cephalosporins [Citation4]), the emergence of resistance mechanisms (in particular bacterial production of β-lactamases, which can hydrolytically inactivate β-lactams [Citation5]) is now significantly limiting treatment options.
More than 40 years ago, stereoselective methodology utilizing hydroxymate-mediated N-C4 cyclization [Citation6,Citation7] has been successfully developed, enabling the preparation and biological evaluation of monocylic β-lactams, such as e.g. oxamazins [Citation8], monosulfactams, monocarbams, and monobactams [Citation9]. The subsequent structure–activity relationship guided development of the monobactam scaffold in the 1980s [Citation10] led to the discovery of aztreonam (1, ATM), which remains the only clinically approved monobactam to date [Citation11], with notable activity against many concerning Gram-negative pathogens and stability toward hydrolysis by challenging metallo-β-lactamases (MBLs). However, some MBLs (e.g. NDM-1 [Citation12]) and extended-spectrum β-lactamases (ESBLs; such as e.g. KPC- and Amp-type enzymes) with carbapenemase/cephalosporinase activity have already evolved to be capable of hydrolyzing 1, thereby limiting its clinical utility in some MDR infections [Citation13].
Targeting bacterial iron acquisition machinery transport systems has proven potential as a new avenue for creating more potent antibiotics [Citation14,Citation15]. The “Trojan-Horse Strategy“ [Citation16,Citation17] has been successfully explored in the past with both, naturally occurring (e.g. sideromycins [Citation18]) and synthetic siderophore conjugates displaying enhanced antibacterial activities both in vitro and in vivo [Citation19,Citation20]. Specifically, siderophore-conjugated monocyclic β-lactams (such as e.g. U-78608 [Citation21,Citation22], BAL30072 [Citation23], and SC23 [Citation24]; ) have been studied extensively over the last decade with an aim to harness a swift balance between their unique mechanism of action, improved antibacterial activity attributable to enhanced antibiotic uptake through siderophore-mediated pathways (at least with respect to analogous structures lacking siderophore moiety) and inherent hydrolytic stability of monocyclic β-lactams against β-lactamases in comparison with bicyclic systems [Citation25,Citation26]. Unfortunately, lengthy synthetic routes and other factors contributed to the fact that none of these compounds were ever used clinically. Encouragingly, the recent FDA approval [Citation27] of siderophore-conjugated cephalosporin cefiderocol [Citation28], which exhibits potent in vitro efficacy against troublesome MDR bacteria (including carbapenemase-producing Enterobacterales [Citation29,Citation30]), warrants this approach.
Figure 1. Structures of aztreonam (1) and selected examples of siderophore-conjugated monocyclic β-lactams from literature. Note that parts of the molecule containing siderophore mimetics are highlighted in blue.
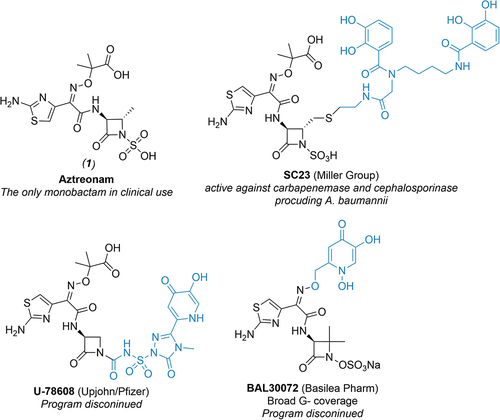
In the patent under review, synthesis, and promising early microbiological data on R2-siderophore conjugated aztreonam derivatives (SCADs) corresponding to the general structure 2 () is reported. Note, however, that all substituents were defined very broadly (R1–R4, see ) as follows: R1 represents H or simple C1–C12 alkyl group, optionally further substituted by (cyclo)alkyl, phenyl or (hetero)aryl via ether, thioether, amide, or amine; R2 contains a comprehensive list of different linkers connected to a wide list of siderophores via oxime oxygen. Note that a rather interesting feature of this patent application is a reported list of more than 100 siderophores from different antibiotics (listed alphabetically from Achromobactin to Yersiniabactin), which could all potentially be used at the R2; R3 can feature different substituents like H, (hetero)(cyclo)alkyl phenyl, (hetero)aryl, connected to the thiazole ring via ether, thioether, amide or an amine; and R4 represents an activating group, typically an (un)substituted sulfate, sulfonate, phosphate, carboxylate, or carboxamide functionality. In all cases, siderophore-like moieties were attached to the aminothiazoloxime (ATMO) carboxylic acid part of the C-3 sidechain of 1, with the aim to further extend the activity of monobactams against MDR Gram-negative pathogens, such as P. aeruginosa and A. baumannii. Hence, only compounds presented in patent examples are discussed herein.
Figure 2. Summary of the present invention and key advantages of the chosen approach. Note that key structural features of aztreonam, including (i) aminothiazoloxime (ATMO) sidechain which enhances Gram-negative activity, (ii) an essential β-lactam ring acylating warhead, (iii) methyl group that improves stability toward β-lactamases, and (iv) N-1 heteroatom activation provided by sulfonic acid are highlighted in orange, violet, cyan, and salmon color, respectively. A site where patent assignees envisaged the installation of siderophore mimetic with a hope to enhance activity against MDR Gram-negative pathogens is shown in a green box.
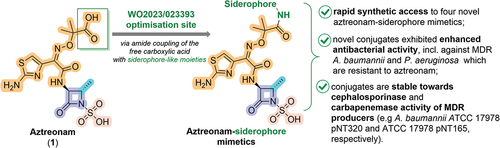
Figure 3. General Markush structure of the new compound series, chemical structure of the control compound, and chemical structures of key novel functionalized monocyclic-β-lactam derivatives from the patent assignees. The siderophore-containing regions are highlighted in green (bis-catechol based ligands).
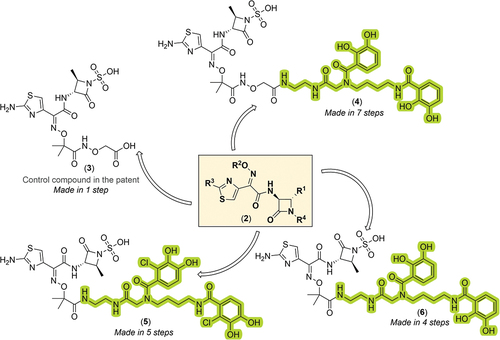
2. Chemistry
To this end, the present patent discloses detailed synthetic and purification conditions required for the preparation of the control compound 3 and SCADs 4–6 (for summary of synthetic procedures see ), which mostly originate from their elaborate earlier reports on the matter in the peer-reviewed literature [Citation31,Citation32]. In all cases, the requisite siderophore mimetics encompassing a free terminal amino functionality (which is required for the later direct attachment of the siderophore ligand to the ATMO carboxylic acid part of the C-3 sidechain of 1 using standard amide coupling procedures) were first prepared in 4–5 synthetic steps (for final compounds 4–6) or commercially obtained (e.g. for control compound 3). For the R2-dihydroxybenzoatebis-catechol structures 4–6, requisite siderophore mimetics were prepared in three consecutive steps, starting with the Cbz-protection of commercially available ethane-1,2-diamine 8 with CbzCl to give benzyl (2-aminoethyl)carbamate 9, which is an integral part of molecular architecture of all three bis-catechol analogues.
Figure 4. Summary of synthetic strategies reported by the patent assignees required to prepare novel analogues 3–6. reagents and conditions: (a)* benzylchloroformate, CH2Cl2, 0°C, 3.5 h; (b) N-hydroxysuccinimide (NHS), EDC·HCl, DMF, rt, then 9, several hours; (c)* 10% Pd/C, MeOH, H2 balloon, rt, 16 h; (d) 1 in DMF, HBTU, DIPEA, 10 min, rt, then 10 in DMF, rt, 16 h; (e)* Et3N, (Boc)2O, CH2Cl2, 0°C to rt; (f) 11b in DMF, HBTU, DIPEA, 10 min, rt, then 10 in DMF, 16 h; (g)* TFA, CH2Cl2, rt; (h) 1, THF-H2O (50/50, v/v; pH = 4.5), EDC·HCl; (i)* NaOH, THF-H2O (2:1; pH = 4.5), rt, 3 h, then 1N HCl; (j) NHS, EDC·HCl, DMF, rt, 3 h, then benzyl (2-aminoethyl)carbamate (9), DIPEA, rt, 16 h; (k)* 10% Pd/C, MeOH, H2 balloon, rt, 16 h; (l) 1 in DMF, HBTU, DIPEA, 10 min, rt, then 16 in DMF, rt, 16 h; (m) NHS, EDC·HCl, DMF, rt, then 11a, DIPEA, rt, several hours. Note that asterisk (*) denotes product used in the next step without further purification. For multi-step reaction sequences, overall yields (in red) were estimated using the longest linear sequence/available data.
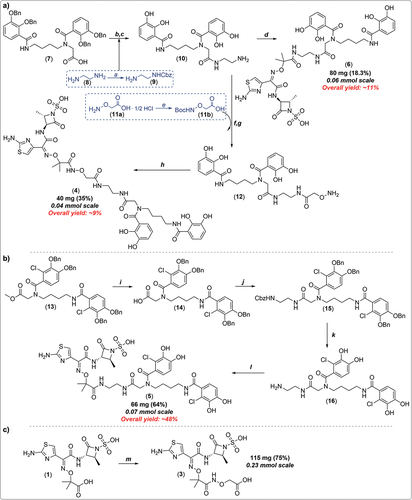
Thereafter, a synthetic sequence composed of (i) conversion of the commercial carboxylic acid 7 into the corresponding activated succinimidyl ester (NHS-ester) using NHS/EDC activation methodology in-situ, (ii) coupling of the NHS-ester with 9 and (iii) subsequent Pd/C-catalyzed hydrogenation of resultant tetrabenzyl bis-cathechol intermediate, gave the key siderophore intermediate 10. Coupling of 10 with aztreonam (1) under basic conditions using HBTU as amide coupling reagent gave conjugate 6. In parallel, intermediate 10 was also coupled with Boc-protected aminooxyacetic acid 11b; the resultant terminal hydroxylamine intermediate 12 was then reacted with 1 using EDC in aqueous THF (at pH 4.5) to give a target conjugate 4 featuring a hydroxamate linkage. Separately, the chlorodihydroxybenzoate conjugate 5 was successfully assembled using very similar synthetic strategy (as depicted in ) in five steps (48% overall yield), utilizing HBTU and DIPEA in anhydrous THF in the final coupling step with 1. Lastly, the control aminooxyacetic acid derivative 3 () was prepared in one-step, using analogous EDC/NHS activation strategy of 1 as discussed above, followed by reaction with the commercially available aminooxyacetic acid, giving control compound 3 in a good yield (75%). All final compounds were purified by reversed-phase C-18 prep-HPLC, before compounds were assayed in microbiological experiments.
3. Biology
The control compound 3 and newly synthesized SCADs 4–6 were evaluated in in vitro antibacterial assays performed in suitable iron deficient media (which better mimic the biochemical situation during infections), as previously described [Citation20]. The obtained results were benchmarked against the antibacterial activity of 1 and available MIC data on C-4 thiomethyl conjugate SC23 previously reported by the Miller group [Citation24]. Whilst the activity of structurally simpler aminooxyacetic acid analogue 3 was highly comparable to that of 1, bis-catechol based conjugates 4–6 rather unexpectedly exhibited superb MIC values against notorious Gram-negative strains identified by the World Health Organization as pathogens of highest concern (which are resistant to 1; all measured MICs >25 μM), such as MDR P. aeruginosa (MICs = 0.1–3 μM) and MDR A. baumannii, including carbapenemase and cephalosporinase producers (e.g A. baumannii ATCC 17,978 pNT165, MICs = 3–6.25 μM; and TCC 17,978 pNT320, MICs = 0.8–3 μM; respectively). As expected, none of the novel conjugated were active against Gram-positive pathogen S. aureus (MICs >25 μM), but were encouragingly active against several other high-priority pathogens, such as e.g. Gram-negative K. pneumoniae ATCC 8303 × 68carrying novel acquired MBL gene bla(SIM-1)(MICCpd1 = 0.2 μM, MICsCpds4–6 = 0.05–0.8 μM) and pathogenic E. coli DC0 (MICCpd1 0.8/0.2 μM, MICsCpds4–6 = 0.025–1.6 μM). Note however, that no data demonstrating the in vivo efficacy of the newly developed SCADs are included in the patent filing under evaluation.
4. Expert opinion
The fact that most β-lactams are produced by (semi)-fermentation methods has significantly limited the scope for synthetic approaches to tackle the issue of resistance toward these agents, including with respect to rapid preparation and biological evaluation of β-lactam-siderophore libraries, such as SCADs. Hence, fully synthetic approaches as described in the patent under evaluation, which could potentially be extended beyond 1 to other β-lactams (including bicyclic structures), are desirable.
Perhaps, the biggest advantage (at least from a synthetic chemistry standpoint) in the patent is the use of 1 (which is easily commercially available in large quantities) as a starting point for chemical modifications (i.e. attachment of siderophores). The claims, however, are defined very widely, with only a very few examples presented. Hence, further studies will be required to fully determine the overall applicability of this approach, at least with regard to the selection of the most optimal siderophore mimetic with the lowest potential of resistance development.
Furthermore, the patented strategy for novel SCADs takes the advantage of the current lower susceptibility of 1 toward β-lactamase-mediated destruction [Citation33] (at least in comparison to bicyclic β-lactams [Citation34]). Further the advantage of obtaining SCADs via milder and less tedious synthetic routes as described, might enable a rapid generation of novel compound libraries; once siderophore moieties are prepared, the target SCADs can be (i) assembled in 4–7 synthetic steps (compounds 4–6); the control aminooxyacetic acid derivative 3 can be prepared directly in a single step. The most important siderophores, at least from the standpoint of this patent, were the bis-catechol and hydroxamic acid-type ligands. Lastly however, somewhat low overall yields for the e.g. most active bis-catechol conjugates 4–6, ranging between 9% and 48%, necessitate further methodology development to enable routine preparation of such scaffold on an industrial scale. Nevertheless, structurally novel SCADs reported have significantly improved antimicrobial properties in-vitro, with high potential for further optimization. However, the lack of corresponding in-vivo efficacy data for reported SCADs is a significant shortcoming of the current patent application; detailed in-vivo studies will be therefore required to fully understand the real clinical potential of the patented siderophore-conjugated monocyclic β-lactam structures.
Overall, however, the evaluation of the present application clearly highlights that the taken approach possesses a real therapeutic opportunity to create new types of clinically useful and targeted Gram-negative antibiotic conjugates that could be used for treatment of most worrisome MDR bacterial hospital-acquired infections caused by MDR P. aeruginosa and A. baumannii by pharmacologically targeting the bacterial iron-transport machinery [Citation35], while simultaneously circumventing the common β-lactam permeability challenges often observed in MDR infections with Gram-negative pathogens [Citation36,Citation37].
Article highlights
Antibacterial resistance remains one of the most significant concerns to public health.
Several siderophore-conjugated aztreonam derivatives were developed and microbiologically evaluated by the patent authors.
Siderophore-conjugated aztreonam analogues exerted superior antibacterial properties against several high-priority pathogens (i.e. compared to aztreonam alone) and appeared more stable towards beta-lactamase-mediated hydrolysis.
Siderophore-conjugation strategy has considerable clinical potential (i.e. by its application to other beta-lactams) to yield new types of clinically useful Gram-negative antibiotics.
Declaration of interests
The authors have no relevant affiliations or financial involvement with any organization or entity with a financial interest in or financial conflict with the subject matter or materials discussed in the manuscript. This includes employment, consultancies, honoraria, stock ownership or options, expert testimony, grants or patents received or pending, or royalties.
Reviewer disclosures
Peer reviewers on this manuscript have no relevant financial or other relationships to disclose.
Author contribution statement
A.K. wrote the initial draft of the manuscript. Both authors have edited and approved the final version of the manuscript.
Additional information
Funding
References
- Kunz Coyne AJ, El Ghali A, Holger D, et al. Therapeutic strategies for emerging multidrug-resistant Pseudomonas aeruginosa. Infect Dis Ther. 2022;11(2):661–682. doi: 10.1007/s40121-022-00591-2
- Perez F, Hujer AM, Hujer KM, et al. Global challenge of multidrug-resistant Acinetobacter baumannii. Antimicrob Agents Chemother. 2007;51(10):3471–3484. doi: 10.1128/AAC.01464-06
- Tacconelli E, Carrara E, Savoldi A, et al. Discovery, research, and development of new antibiotics: the WHO priority list of antibiotic-resistant bacteria and tuberculosis. Lancet Infect Dis. 2018;18(3):318–327. doi: 10.1016/S1473-3099(17)30753-3
- Bush K, Bradford PA. β-lactams and β-lactamase inhibitors: an overview. Cold Spring Harb Perspect Med. 2016;6(8). doi: 10.1101/cshperspect.a025247
- Bonomo RA, Tolmasky M, Bonomo RA, et al. Enzyme-mediated resistance to antibiotics: mechanisms, dissemination, and prospects for inhibition. ASM Press; 2007. doi: 10.1128/9781555815615
- Miller MJ, Mattingly PG, Morrison MA, et al. Synthesis of beta-lactams from substituted hydroxamic acids. J Am Chem Soc. 1980;102(23):7026–7032. doi: 10.1021/ja00543a021
- Floyd DM, Fritz AW, Cimarusti CM. MonobactamS. StereoSpecific SyntheSiS of (S)-3-amino-2-oxoazetidine-1-Sulfonic acidS. J Org Chem. 1982;47(1):176–178. doi: 10.1021/jo00340a048
- Woulfe SR, Miller MJ. Synthesis and biological activity of substituted [[3(S)-(acylamino)-2-oxo-1-azetidinyl]oxy]acetic acids. A new class of heteroatom-activated beta-lactam antibiotics. J Med Chem. 1985;28(10):1447–1453. doi: 10.1021/jm00148a013
- Cimarusti CM, Sykes RB. Monocyclic β-lactam antibiotics. Med Res Rev. 1984;4(1):1–24. doi: 10.1002/med.2610040103
- Sykes RB, Bonner DP. Discovery and development of the monobactams. Rev Infect Dis. 1985;7(4):S579–93. doi: 10.1093/clinids/7.Supplement_4.S579
- Butler MS, Gigante V, Sati H, et al. Analysis of the clinical pipeline of treatments for drug-resistant bacterial infections: despite progress, more action is needed. Antimicrob Agents Chemother. 2022;66(3):e0199121. doi: 10.1128/aac.01991-21
- Lohans CT, Brem J, Schofield CJ. New Delhi metallo-β-lactamase 1 catalyzes avibactam and aztreonam hydrolysis. Antimicrob Agents Chemother. 2017;61(12). doi: 10.1128/AAC.01224-17
- Morroni G, Bressan R, Fioriti S, et al. Antimicrobial activity of aztreonam in combination with old and new β-Lactamase inhibitors against MBL and ESBL co-producing gram-negative clinical isolates: possible options for the treatment of complicated infections. Antibiotics. 2021;10(11):1341. doi: 10.3390/antibiotics10111341
- Sargun A, Gerner RR, Raffatellu M, et al. Harnessing iron acquisition machinery to target enterobacteriaceae. J Infect Dis. 2021;223(12):S307–s313. doi: 10.1093/infdis/jiaa440
- Stelitano G, Cocorullo M, Mori M, et al. Iron acquisition and metabolism as a promising target for antimicrobials (bottlenecks and opportunities): where do we stand? Int J Mol Sci. 2023;24(7):6181. doi: 10.3390/ijms24076181
- Möllmann U, Heinisch L, Bauernfeind A, et al. Siderophores as drug delivery agents: application of the “trojan horse” strategy. Biometals. 2009;22(4):615–624. doi: 10.1007/s10534-009-9219-2
- Rayner B, Verderosa AD, Ferro V, et al. Siderophore conjugates to combat antibiotic-resistant bacteria. RSC Med Chem. 2023;14(5):800–822. doi: 10.1039/D2MD00465H
- Wencewicz TA, Miller MJ. Sideromycins as pathogen-targeted antibiotics. Antibacterials. 2018;II:151–183.
- Lin YM, Ghosh M, Miller PA, et al. Synthetic sideromycins (skepticism and optimism): selective generation of either broad or narrow spectrum Gram-negative antibiotics. Biometals. 2019;32(3):425–451. doi: 10.1007/s10534-019-00192-6
- Ghosh M, Miller PA, Möllmann U, et al. Targeted antibiotic delivery: selective siderophore conjugation with daptomycin confers potent activity against multidrug resistant Acinetobacter baumannii both in vitro and in vivo. J Med Chem. 2017;60(11):4577–4583. doi: 10.1021/acs.jmedchem.7b00102
- Zurenko GE, Truesdell SE, Yagi BH, et al. In vitro antibacterial activity and interactions with beta-lactamases and penicillin-binding proteins of the new monocarbam antibiotic U-78608. Antimicrob Agents Chemother. 1990;34(5):884–888. doi: 10.1128/AAC.34.5.884
- Barbachyn MR, Tuominen TC. Synthesis and structure–activity relationships of monocarbams leading to U-78608. J Antibiot (Tokyo). 1990;43(9):1199–1203. doi: 10.7164/antibiotics.43.1199
- Page MG, Dantier C, Desarbre E. In vitro properties of BAL30072, a novel siderophore sulfactam with activity against multiresistant gram-negative bacilli. Antimicrob Agents Chemother. 2010;54(6):2291–2302. doi: 10.1128/AAC.01525-09
- Carosso S, Liu R, Miller PA, et al. Methodology for monobactam diversification: syntheses and studies of 4-thiomethyl substituted β-lactams with activity against Gram-negative bacteria, including carbapenemase producing Acinetobacter baumannii. J Med Chem. 2017;60(21):8933–8944. doi: 10.1021/acs.jmedchem.7b01164
- Bush K. Bench-to-bedside review: the role of β-lactamases in antibiotic-resistant Gram-negative infections. Crit Care. 2010;14(3):224. doi: 10.1186/cc8892
- Almeida MC, da Costa PM, Sousa E, et al. Emerging target-directed approaches for the treatment and diagnosis of microbial infections. J Med Chem. 2023;66(1):32–70. doi: 10.1021/acs.jmedchem.2c01212
- FDA approves new antibacterial drug to treat complicated urinary tract infections as part of ongoing efforts to address antimicrobial resistance. [2023 Apr 14]. Available from: https://www.fda.gov/news-events/press-announcements/fda-approves-new-antibacterial-drug-treat-complicated-urinary-tract-infections-part-ongoing-efforts
- Aoki T, Yoshizawa H, Yamawaki K, et al. Cefiderocol (S-649266), a new siderophore cephalosporin exhibiting potent activities against Pseudomonas aeruginosa and other gram-negative pathogens including multi-drug resistant bacteria: structure activity relationship. Eur J Med Chem. 2018;155:847–868. doi: 10.1016/j.ejmech.2018.06.014
- Kaye KS, Naas T, Pogue JM, et al. Cefiderocol, a siderophore cephalosporin, as a treatment option for infections caused by Carbapenem-Resistant Enterobacterales. Infectious diseases and therapy. Infect Dis Ther. 2023;12(3):777–806. doi: 10.1007/s40121-023-00773-6
- Sansone P, Giaccari LG, Coppolino F, et al. Cefiderocol for carbapenem-resistant bacteria: handle with care! A review of the real-world evidence. Antibiotics. 2022;11(7):904. doi: 10.3390/antibiotics11070904
- Liu R, Miller PA, Miller MJ. Conjugation of aztreonam, a synthetic monocyclic β-lactam antibiotic, to a siderophore mimetic significantly expands activity against Gram-negative bacteria. ACS Infect Dis. 2021;7(11):2979–2986. doi: 10.1021/acsinfecdis.1c00458
- Miller MJ, Liu R. Design and syntheses of new antibiotics inspired by nature’s quest for iron in an oxidative climate. Acc Chem Res. 2021;54(7):1646–1661. doi: 10.1021/acs.accounts.1c00004
- Decuyper L, Jukič M, Sosič I, et al. Antibacterial and β-lactamase inhibitory activity of monocyclic β-lactams. Med Res Rev. 2018;38(2):426–503. doi: 10.1002/med.21443
- Tooke CL, Hinchliffe P, Bragginton EC, et al. β-lactamases and β-lactamase inhibitors in the 21st Century. J Mol Biol. 2019;431(18):3472–3500. doi: 10.1016/j.jmb.2019.04.002
- Page MGP. The role of iron and siderophores in infection, and the development of siderophore antibiotics. Clinl Infect Dis. 2019;69(Supplement_7):S529–S537. doi: 10.1093/cid/ciz825
- King DT, Sobhanifar S, Strynadka NCJ. One ring to rule them all: current trends in combating bacterial resistance to the β-lactams. Protein Sci. 2016;25(4):787–803. doi: 10.1002/pro.2889
- Zgurskaya HI, Rybenkov VV. Permeability barriers of Gram-negative pathogens. Ann N Y Acad Sci. 2020;1459(1):5–18. doi: 10.1111/nyas.14134