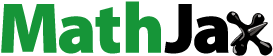
ABSTRACT
Introduction
Type 1 diabetes (T1D) is an autoimmune disease that results from the destruction of insulin-producing beta cells in the pancreas; it leads to the under or nonproduction of insulin. T1D is associated with numerous life-threatening micro- and macro-vascular complications and early deaths, hence the development of preventative strategies is a priority for research.
Areas covered
The authors outline the drawbacks of available treatments for T1D and assess the three key strategies for prevention, including immunomodulatory therapies which hold the most potential. This article examines CTLA4-Ig and its efficacy and safety profiles. Finally, the pharmacokinetic parameters and pharmacodynamic markers of abatacept are shown in vivo and in clinical trials, guiding dosage regimen recommendations for future investigational studies.
Expert opinion
Immunomodulation is one of the promising strategies for decelerating the progression of beta-cell destruction after the onset of T1D. It holds the advantage of specific immune modulation without systemic general immunosuppression. Preclinical and clinical studies have yielded promising data on the use of CTLA4-Ig in T1D. Variations in response to CTLA4-Ig might be partially explained by the existence of multiple T1D subtypes with varying baseline innate inflammatory/regulatory bias and the rate of C-peptide decline.
1. Introduction
Insulin is a glucose reducing hormone that is released in the body by beta cells found in the islets of Langerhans located in the pancreas. Type 1 diabetes is an autoimmune disease that results from the destruction of these insulin-producing beta cells in the pancreas. The loss of beta cells results in little or no production of insulin which necessitates the administration of exogenous insulin throughout the patients’ life. Much about the causing factors of type 1 diabetes remain unknown; however, it is thought to involve genetic and environmental factors. Nevertheless, the prevalence of type 1 diabetes is markedly increasing worldwide. According to the International Diabetes Federation (IDF), the estimated number of children and adolescents (less than 20 years) with type 1 diabetes in 2019 was over 1.1 million and the number of new cases per year is 128,900 with the highest number of type 1 diabetes cases found in Europe [1]. Diabetes is associated with many life-threatening micro- and macro-vascular complications and early deaths; therefore, preventative strategies are warranted.
Our understanding of the pathogenesis of type 1 diabetes has increased significantly, particularly with regard to epidemiology, heterogeneity, pancreatic pathology, and ways of prediction of the disease [Citation2]. The advancement of technology has improved the use of machines such as insulin pumps and glucose monitors, which help type 1 diabetes patients monitor the condition. The existing delivery systems for insulin all, except for two that were recently approved by FDA, suffer from the inability to regulate insulin without patient intervention. However, at present, despite extensive scientific efforts and investments, the current preventative measures or cures for type 1 diabetes are yet to be installed.
There are three current different strategies for the prevention of type 1 diabetes or prevention of the complete loss of beta-cell function after the onset of type 1 diabetes [Citation3]. First strategy is to reduce exposure of genetically prone individuals early in life to environmental factors that can initiate the pathophysiological process that leads to type 1 diabetes. The second prevention strategy is to stop the gradual loss of pancreatic beta cells in individuals with pre-diabetes or individuals who test positive for islet autoantibodies. The third prevention strategy is to preserve residual insulin secretion by protecting the existing beta-cell mass after the onset of type 1 diabetes, since the majority of patients with new-onset type 1 diabetes have residual insulin secretions [Citation4,Citation5]. Currently, screening for type 1 diabetes using multiple autoantibodies is only recommended in the research settings; however, it should also be considered if the individual has first-degree family members with type 1 diabetes because more evidence indicates that the presence of such autoantibodies can be used as an early-stage predictor of clinical hyperglycemia and diabetes [Citation6–Citation9]. However, screening programs for type 1 diabetes are not routinely implemented and patients are often present with acute symptoms of diabetes as the first manifestations of the disease. In addition, to the best of our knowledge, no primary or secondary prevention trial resulted in preventing the progression to clinical type 1 diabetes in at-risk individuals. Therefore, the most practical strategy nowadays is to hinder the progression of beta-cell dysfunction during the early stages of the disease. This review will focus on the available agents used for the prevention of complete loss of beta-cell function to preserve residual insulin secretion after the onset of type 1 diabetes, particularly immunomodulatory agents such as the cytotoxic T lymphocyte-associated antigen 4-immunoglobulin (CTLA4-Ig) or abatacept.
2. Current treatments for type 1 diabetes
Complications associated with the poor treatment of type 1 diabetes or lack thereof, are diverse, debilitating and could lead to early death. These complications range from diabetic ketoacidosis to diseases related to cardiovascular, neuropathy, and retinopathy. Thus, a strict diet regimen and close management on a daily basis are crucial for achieving normal blood sugar levels in type 1 diabetes patients. To ensure this, type 1 diabetes patients are typically provided with a glucose monitor to check blood glucose levels multiple times daily. If glucose levels are too high, the patient is required to self-administer an insulin injection subcutaneously several times a day accordingly. Another method of treatment is an insulin pump, which is a wearable device that continuously provides insulin to the body through a catheter found under the skin. Through this device, the patient can easily modify the amount of insulin injected and change their time intervals [Citation2]. Reports of increased hypoglycemic episodes after continuous use of insulin pumps are sparse and warrants further investigation [Citation10–Citation12]. Although all the above-mentioned insulin delivery systems have proven effective in the regulation of type 1 diabetes, most do not subside the need for patient intervention [Citation2]. In the last two years, two attempts to create ‘artificial pancreas’ consisting of a hybrid closed loop system that monitors glucose and automatically adjusts the delivery of insulin based on the user’s glucose reading were successful and been approved by FDA [Citation13–Citation15]. Despite the effectiveness of continuous glucose monitoring in improving glycemic control in patients with type 1 diabetes, the evidence regarding the effect on reduction of hypoglycemic incidence is moderate and yet to be evaluated using defined and standardized reporting criteria [Citation16]. Other forms of current type 1 diabetes treatment include the transplantation of the pancreas or the islet cells. However, despite ongoing trials, neither of these transplantations have been proven safe or lacking detrimental complications, such as immunosuppression and rejection [Citation10].
Seeing that the current available treatments are associated with many drawbacks, the need for preventative therapies becomes ever more important. Here we show that immunomodulatory therapies, as a secondary or tertiary prevention strategy, hold the potential in slowing down the progression of beta-cell dysfunction early in the disease [Citation17]. The targeted early stages of such strategy are the presymptomatic stage 1 and stage 2 of type 1 diabetes (secondary prevention), in which β-cell autoimmunity demonstrated by the detection of two or more islet autoantibodies is present together with normoglycemia and dysglycemia, respectively; and the symptomatic stage 3 of type 1 diabetes (tertiary prevention), in which hyperglycemia with clinical symptoms starts to appear [Citation9,Citation17]. Examples of immunomodulatory agents are CTLA4-Ig (abatacept), TNF-α antagonist (etanercept), anti-CD3 (teplizumab and otelixizumab) and anti-CD20 (rituximab). This review will focus on CTLA4-Ig as one of the most effective and safe options.
3. Immunomodulatory therapy
In type 1 diabetes, genetic predisposition plays a main role in the autoimmune destruction of beta cells. This is due to the defects in the immune regulation that result from these genetic variants. Type 1 diabetes patients have been found to have different immune regulatory capacities than healthy individuals, rendering the regulation of autoimmunity as an intervention method an important step in restoring immune imbalances [Citation18]. Central to this approach is to understand the mechanism used by the immune system to destruct the pancreatic beta cells. Here, it is important to note that T-cells play a key role in the immunogenesis of type 1 diabetes. For the full activation of T-cells, two signals are required [Citation19]. The first signal results from the interaction between the T-cell receptor (TCR) and an antigen peptide presented on a major histocompatibility complex (MHC) by an antigen-presenting cell (APC). The second and most crucial signal for the full activation of the T-cell results from the interaction between the TCR and specific ligands on the APC (). These ligands are CD80 and CD86, which are found on the APC, and CD28 located on the surface of the T-cell. This interaction results in a strong co-stimulatory signal required for the full activation of the T-cell. Therefore, the inhibition of the second signal to block the stimulation of the T-cell was proposed as a therapeutic strategy for autoimmunity [Citation20]. Unlike immunosuppression, this strategy interferes with the early stages of T-cell activation; therefore, it does not result in heavy general immunosuppression. Consequently, different immunomodulatory agents were tested in patients with new-onset type 1 diabetes in an attempt to arrest autoimmunity to preserve beta cells function [Citation3,Citation21]. C-peptide levels were used as a marker for endogenous insulin production by beta cells in these studies [Citation22]. For the purpose of this paper, the discussion will be about cytotoxic T lymphocyte-associated antigen 4-immunoglobulin (CTLA4-Ig) as an intervention in new-onset type 1 diabetes as it holds the potential for preserving beta-cell function. Information on other potentially promising agents in this disease area is available for the development of a wider perspective [Citation17,Citation23,Citation24].
Figure 1. Schematic representation of the signals involved in the full activation of the T-Cell. (a). Induction of the first and second signals as a result of the interaction between the ligands on the Antigen-Presenting Cell (APC) and the receptors on the T-Cell. Induction of first signal results from the interaction between an antigen present on the major histocompatibility complex (MHC) and the T-cell receptor (TCR), while the second signal is induced by the binding of CD80/CD86 on APC to CD28 on the T-cell. Full activation of the T-cell requires both signals to be activated. Also, T-cell activation can be inhibited with binding of CD80/CD86 on the APC to CTLA4 protein on the T-cell. (b). The effect of CTLA4-Ig (Abatacept) as a blocker of the second costimulatory signal required for full activation of the T-cell.
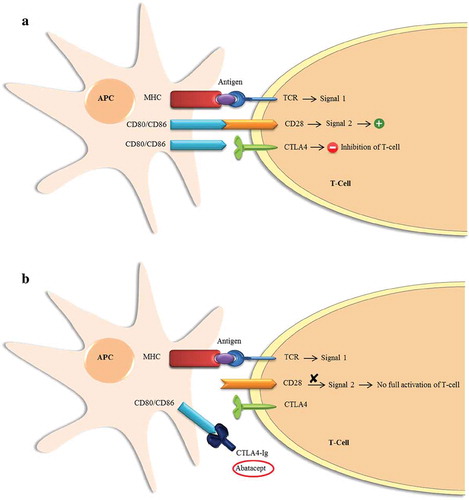
4. Cytotoxic T lymphocyte-associated antigen 4-immunoglobulin (CTLA4-Ig)
4.1. Pharmacological effects of CTLA4-Ig
CTLA4 protein, which was discovered in 1987 [Citation25], has been shown to be related to CD28, the most prominent co-stimulatory receptor for naïve T-cells, by having the same specific sequence of amino acids that are critical for binding with CD80 and CD86 on the APC. CTLA4 protein has a higher affinity to CD80 and CD86 than CD28 [Citation26]. The CTLA4 protein was then found to be a negative regulator of the CD28-mediated T-cell co-stimulation [Citation27,Citation28]. Thus, CTLA4-Ig or abatacept is used for selectively blocking CD28 co-stimulation which interferes with T-cell activation, proliferation, and survival.
The antagonism of CD28 pathway has shown to be effective in transplant settings and autoimmune diseases in preclinical models [Citation20]. In vivo studies revealed that the simultaneous blockade of CD28 and CD40 pathways, which are important regulators of T-cell-dependent immune response, resulted in the long-term survival of allogeneic skin graft and inhibited the rejection of cardiac graft [Citation29]. Lin, et al. have found that cardiac allografts survived for a long time (median 30 days) in mice treated with CTLA4-Ig at the time of transplantation. The survival was further prolonged with the addition of donor-specific transfusion to CTLA4-Ig [Citation30]. CTLA4-Ig therapy was able to inhibit the rejection of human pancreatic islet transplants in mice [Citation31]. In the same context, two studies have demonstrated a new strategy to lengthen the survival of transplanted islets by modifying CTLA4-Ig fusion gene in porcine-derived islet xenografts [Citation32] or expressing the CTLA4-Ig fusion gene in dendritic cells, in diabetic mouse models [Citation33]. Further studies showed that CTLA4-Ig can be more effective when combined with other strategies. For example, Pawlick et al. have shown that CTLA4-Ig, when combined with anti-NKG2D, which is an antagonist of the Natural Killer Group 2, member D (NKG2D) receptor expressed on NK and CD8+ T-cells, resulted in prolonging the islet allograft survival and improved glucose tolerance in mouse models with type 1 diabetes [Citation34]. Another study has indicated that the additional expression of the co-inhibitor of T-cell immunity regulation, B7-H4, significantly prolonged allograft survival of islet transplant mice [Citation35].
Altogether, the available evidence suggests that the blocking of T-cell co-stimulation by CTLA4-Ig is effective in prolonging the survival of islet transplants in vivo and associated with much lower side effects than general immunosuppression. Also, the addition of other agents can be considered for a synergistic effect in prolonging allograft survival, particularly islet allografts.
4.2. Efficacy of CTLA4-Ig
CTLA4-Ig is clinically available as abatacept, which was approved by Food and Drug Administration (FDA) in 2005 for the treatment of Rheumatoid Arthritis (RA) and in 2009 for the treatment of Juvenile Idiopathic Arthritis (JIA) under the marketed name ‘Orencia.’ A Phase II, multicenter, multinational, placebo-controlled, double-blind, dose-finding clinical trial was conducted to evaluate the safety and preliminary efficacy of two co-stimulatory blocking drugs: CTLA4-Ig (abatacept) and LEA29Y (belatacept) for patients with refractory RA [Citation36]. The study showed that treatment with CTLA4-Ig and LEA29Y resulted in a dose response for the primary outcome to reduce signs and symptoms of RA on day 85 (assessed by the American College of Rheumatology 20% improvement criteria (ACR20)). In addition, the two medications were well tolerated with only a few side effects [Citation36]. After this study, many large clinical trials investigating the safety and efficacy of CTLA4-Ig in RA have trailed which ended with the approval of this agent in 2005 [Citation37]. Since CTLA4-Ig was proven effective in modulating autoimmunity in RA with a satisfactory safety profile, clinical studies have been undertaken to investigate the possibility of using this agent in other autoimmune conditions such as type 1 diabetes. Abatacept is also being studied for the treatment of other autoimmune diseases including Crohn’s, multiple sclerosis, lupus, and psoriasis.
The destruction of pancreatic beta cells is facilitated by T-cell activation. Therefore, CTLA4-Ig has been investigated for its effectiveness in preserving beta cells and preventing the complete loss of insulin production in an attempt to delay the progression of type 1 diabetes and its complications. In 2011, a multicenter, double-blind, phase II, randomized controlled trial was conducted on 112 patients with new-onset type 1 diabetes aged 6–45 years. Patients were administered abatacept solution of 10 mg/kg, max 1000 mg/dose (n = 77) or a matching placebo (n = 35) through IV infusion on days 1, 14, 28 and monthly thereafter totaling to 27 infusions over 2 years. The C-peptide mean concentration at baseline was 0.7430.42 nmol/L in the abatacept group and 0.745
0.31 nmol/L in the placebo group. At 2 years of treatment, stimulated C-peptide concentration was 59% higher in the abatacept group compared to the placebo group (p = 0.0029) (2-h AUC for C-peptide of 0.378 vs. 0.238 nmol/L, respectively; p = 0.0014). However, the analysis of mixed-effects model showed that the reduction in beta-cell functioning in the abatacept group is parallel to that of the placebo group. In general, the study showed that abatacept was capable of delaying the reduction of beta-cell function by 9.6 months [Citation38]. Then, follow-ups were conducted with the patients for 1 year after cessation of treatment to determine if the treatment effect was persistent. The mean simulated C-peptide 2-h AUC was 0.217 nmol/L in the abatacept group vs. 0.141 nmol/L in the placebo group (p = 0.046). Abatacept was also able to slow the decline in beta-cell function by 9.5 months after 1 year of discontinuation of the treatment [Citation39]. A more recent observational study has evaluated insulin sensitivity before and after treatment with abatacept in patients with RA. Insulin sensitivity was evaluated by calculating Matsuda Insulin Stimulation Index (ISI); abatacept significantly improved ISI after 6 months of treatment (p = 0.003) [Citation40]. Altogether, abatacept was able to slow the reduction of beta-cell function early in the development of the disease (within 3 months of diabetes), and the effect was continued 1 year after the cessation of treatment but without much improvement. This might be explained by the aggressive autoimmune response at the onset of the disease which can be targeted with the co-stimulation blockade of T-cell activation by abatacept. These efforts lead in May 2013 that orphan drug designation in the U.S. by Orban Biotech LLC (Massachusetts, USA) was assigned to abatacept (Orencia) for the treatment of type 1diabetes mellitus patients with residual beta-cell function, yet not FDA-approved for orphan indication [Citation41].
Since its launch in the U.S. by Bristol-Myers Squibb in 2005, Abatacept is available as an intravenous formulation. A subcutaneous formulation for the treatment of RA was granted a positive opinion in July 2012 and in 2013, this subcutaneous injection formulation of the product was approved by Bristol-Myers Squibb and an alliance company, Ono, for the treatment of rheumatoid arthritis in Japan. In 2016, a subcutaneous autoinjector formulation was approved in Japan. Prefilled subcutaneous injections are available in the U.S. for the treatment of JIA since 2017. In the same year, a new indication for adult psoriatic arthritis was added to the prescribing information leaflet of the product [Citation42].
4.3. Safety and adverse events of CTLA4-Ig
While immunoregulatory therapies hold great promise for the treatment of type 1 diabetes, their inadequacy and serious toxicity have limited research efforts to find a lifelong immunosuppression approach [Citation43,Citation44]. However, CTLA4-Ig inhibits naïve T-cell activation; therefore, it selectively inhibits T-cell response to specific antigens instead of broad immunosuppression. The safety of abatacept was studied in many clinical trials and post-marketing surveillance studies (). Most of the trials evaluated the safety of subcutaneous (SC) and intravenous (IV) abatacept in patients with RA, with one study evaluating the safety of abatacept in type 1 diabetes patients. In type 1 diabetes patients, there was no difference in adverse effects between abatacept and placebo [Citation38]. The reported infusion-related adverse events were of low frequency and clinically insignificant. In general, long-term abatacept, either IV or SC, was well tolerated with infections such as upper respiratory tract infections, nasopharyngitis, bronchitis, sinusitis, and urinary tract infections as the most common side effects in patients with RA [Citation45,Citation46]. Malignancies, infections, and autoimmune events were of a concern since abatacept modulates the immune function. However, large post-surveillance studies demonstrated that abatacept was not associated with the significant increase in malignancies, serious infections, and autoimmune events [Citation45,Citation46]. Abatacept also demonstrated low immunogenicity rates that occurred transiently [Citation47,Citation48]. It was demonstrated that immunogenicity is similar after subcutaneous and intravenous abatacept or after monotherapy and combination therapy with methotrexate [Citation47,Citation49], and it was low or increased transiently when switching between the two routes of administration [Citation50]. The rate of injection-site reactions including erythema, hematoma, pain, and pruritus was only 3.5% [Citation44]. One limitation for abatacept clinical use is the restriction of using vaccines within 3 months of treatment, which becomes much important in the pediatric population [Citation38]. In addition, episodes of hypoglycemia were reported, some of which were severe [Citation38].
Table 1. Studies evaluated the safety of CTLA4-Ig (abatacept).
4.4. Pharmacokinetics (PK) and pharmacodynamics (PD) of CTLA4-Ig
The PK parameters and PD markers of SC and IV abatacept were evaluated and characterized in in vivo studies in mice, rats, and monkeys. In addition, PK/PD parameters of this agent were also studied in clinical trials on healthy volunteers and patients with RA (). In most studies, serum concentration of CTLA4-Ig was determined using enzyme-linked immunosorbent assay (ELISA). The mean values for selected PK parameters of abatacept are summarized in . The pharmacokinetics of abatacept in children are generally similar to those in adults, taking into account the effect of body weight as a cofounder [Citation54]. The PK of abatacept was described in all studies by a two-compartment model, as expected with large therapeutic proteins, with a first-order absorption process after SC administration. Bioavailability after SC administration ranged between 60% and 110% in the different species studied, with bioavailability decreasing as doses were increased. This is also in agreement with the wide bioavailability range observed after SC administration of other therapeutic proteins. Immunogenic responses to the humanized nature of abatacept may have contributed to its lower SC bioavailability in different animal species compared to that in clinical trials. Its clearance and volume of distribution in the central compartment were found to increase almost linearly with baseline body weight, which is not expected with monoclonal antibodies, so clearance is greater in patients with higher body weight. This may partially be explained by the fact that abatacept elimination occurs by reticuloendothelial catabolism which is believed to be allometrically scalable by body weight. Abatacept is distributed to extracellular fluid rendering it allometrically scalable by body weight also. Clearance values range observed in humans were narrow, 14.4 to 21.7 mL/h. A dose proportional increase in Cmax and AUC parameters was observed after single or multiple doses of abatacept in different animal species studied. Abatacept has a relatively long half-life ranging 18.8–129 h in mice, 64.3–170 h in rats, 90–160 h in monkeys, and around 116 h in humans. After multiple doses of abatacept, achieving steady state is expected to take weeks.
Table 2. Studies evaluated the PK/PD of CTLA4-Ig (abatacept).
Table 3. Mean values for selected pharmacokinetic parameters of CTLA4-Ig (abatacept).
Abatacept administered intravenously was evaluated in healthy volunteers receiving a single dose of 10 mg/kg compared to RA patients receiving multiple doses of 10 mg/kg, and showing linearity over a dose range 1–20 mg/kg. Peak plasma concentration of abatacept (Cmax) was 186.9 24.7 and 247.1
50.3 mg/L (p = 0.006) and volume of distribution (Vss) was 0.138
0.033 and 0.074
0.031 L/kg (p = 0.001) in healthy volunteers and RA patients, respectively [Citation62]. In vivo, treatment of Sprague-Dawley rats with multiple 10 mg/kg doses of SC abatacept resulted in significantly lower terminal half-life (t1/2) compared to IV multiple doses [Citation63].
Pharmacodynamic pro-inflammatory markers that are elevated in RA patients such as TNFα, MMP-3, and IL-6 were significantly reduced at 16-weeks after treatment in RA patients [Citation62]. In addition, mice challenged with sheep red blood cells (SRBC) in addition to the IV or SC single dose of abatacept had a significant percentage of inhibition of anti- SRBC antibodies [Citation64].
5. Conclusion
Abatacept was tested for the prevention of complete loss of beta-cell function in patients recently diagnosed with type 1 diabetes. It showed good efficacy in conserving beta-cell function through the preservation of C–peptide levels and improvement of insulin sensitivity, but only for a transient period of time. Abatacept has a good efficacy profile, as shown in large post-marketing surveillance studies. New routes of administrations should be made available to alleviate the discomfort associated with IV and SC routes of abatacept. In addition, future studies should investigate the use of combinational therapy to preserve the treatment effect for a longer duration.
6. Expert opinion
Immunomodulation is one of the promising strategies in slowing down the progression of beta-cell destruction after the onset of type 1 diabetes. It also holds the advantage of specific immune modulation without systemic general immunosuppression. In a series of The Lancet journals, immunological strategies were shown to fundamentally change the management of type 1 diabetes [Citation65]. Systemic suppression of the immune system results in a long-term serious complication, which is the major concern that limits the use of immunosuppressants such as cyclosporine in recently diagnosed type 1 diabetes [Citation66,Citation67]. Therefore, newer therapeutic agents have emerged to overcome this problem. For example, etanercept, a TNF-α antagonist, has been shown to be effective in increasing beta-cell function compared to the control; however, it is associated with serious infections [Citation68]. In addition, teplizumab and otelixizumab are anti-CD3, which target the destructive T-cells to delete them and regenerate new nondestructive T-cells that have been tested in type 1 diabetes patients. Although teplizumab was unsuccessful in meeting primary end points of insulin use of less than 0.5 unit/kg/day and HbA1c of less than 6.5% at 1 year [Citation69], it preserved the secretion of C-peptide by 30% and showed significant efficacy after 2 years of follow up in young patients with good residual insulin production. Also, there was no substantial increase in adverse events compared to placebo [Citation70]. However, in a recent phase 2 clinical trial of teplizumab treatment in relatives with type 1 diabetes, clinically important and statistically significant adverse events associated with teplizumab were reported, and the beneficial use of teplizumab in delaying progression to clinical type 1 diabetes in high-risk participants is still under debate [Citation71,Citation72].
The other agent, otelixizumab, has also shown efficacy, but it appears to have a narrow therapeutic window. It did not result in the conservation of beta cells when used at a low dose [Citation73]. On the other hand, when the dose was increased, it showed significant preservation of beta-cell function at 18 months; however, it was associated with serious adverse events [Citation74]. Another tested agent is rituximab, an anti-CD20 that downregulates B lymphocytes. It showed some efficacy but for a short period [Citation75,Citation76].
Abatacept, the agent reviewed in this study, is an immunomodulatory agent that blocks the costimulation of T-cells, which help in treating the autoimmune destruction of beta cells in type 1 diabetes. It has been shown to slow the rate of the reduction of beta-cell function in patients with recent onset of type 1 diabetes. In addition, the reduction of central memory CD4+ T cells by abatacept can be used as a replacement marker for C-peptide reduction in recently diagnosed type 1 diabetes patients [Citation77]. Hence, it can be useful for monitoring the efficacy of treatment interventions. This is particularly important, as the change in fasting C-peptide level was limited in the first 48 months compared to baseline, making this outcome of limited reliability in clinical trials to demonstrate benefit of a potential therapy [Citation78]. However, the immune tolerability of abatacept is not long-lasting and may be associated with resistance due to B lymphocyte alterations. Moreover, the short-period effect appears to coincide with the fact that autoimmunity subsides with time in type 1 diabetes patients .
Another strategy, which might be effective in prolonging the effect of the treatment, could be the use of combination therapy. These primary prevention strategies must be safe and well-tolerated because they are administered to healthy individuals who have no clinical signs of autoimmune disease or metabolic impairment, and whose risk of developing type 1 diabetes is unknown. Therefore, an ongoing trial (Type 1 Diabetes TialNet, which is currently Closed to Enrollment – Study Ongoing) is examining the effect of abatacept in preventing progression to clinical type 1 diabetes in Stage 1 & 2 patients with islet autoantibodies [Citation79]. Variations in response to CTLA4-Ig might be partially explained by the new insights of the existence of multiple type 1 diabetes subtypes with varying baseline innate inflammatory/regulatory bias and the rate of C-peptide decline [Citation80]. Heterogeneity of C-peptide decline was observed between individuals after the clinical diagnosis of type 1 diabetes, which was evidenced by the variability in C-peptide slope over time. Factors that may have contributed to such variability included age and number of basophils [Citation78]. Further investigation of the progression of type 1 diabetes in different age groups warrants better understanding and enhancement of population response to immunomodulation therapy.
In a study exploring the possible mechanism of abatacept resistance, abatacept-treated subjects were grouped into respondents (Rs) and non-respondents (NRs) based on the rate of the progression of type 1 diabetes, measured by C-peptide decline [Citation81]. It was concluded that differential expression of B-cell genes, either individually or modularly, can be used to predict disease progression in both R and NR individuals of the abatacept-treated group after 84 days. These differences seemed to be transient and subsided after 2 years and were not evident when the abatacept-treated group was compared to placebo. Furthermore, the transient B-cell gene expression differences were linked to differential expression of T cell costimulatory ligands including ICOSLG, CD40, and CD58 between R and NR groups. This suggests a reprogramming mechanism of the CD28/CD80/CD86 T cell co-stimulation in response to abatacept therapy, leading to the observed resistance in the poor response group. This study together with others suggest the association of B cells, T cells, neutrophils, and other cell types with abatacept response and resistance in R and NR groups, respectively [Citation77,Citation80,Citation81].
A number of other factors contributing to unsuccessful immunomodulation in type 1 diabetes trials have been identified including disease heterogeneity, inappropriate preclinical models, poor understanding of the disease immunological and metabolic conditions, flaws in trial designs, and the need for further understanding of type 1 diabetes pathogenesis [Citation77,Citation78,Citation81]. Despite the above-mentioned factors, our collective and accumulative understanding of the pathogenesis of type 1 diabetes and related physiological status, augmented by the new advances in the designs and models used in preclinical and clinical trials to understand the underlying immunological and metabolic status, get us one step closer to an immunomodulation therapy and increase our hopes for better management of type 1 diabetes.
7. Drug Summary
Drug Summary
Article highlights
CTLA4-Ig slows the rate of the reduction of beta-cell function in patients with recent onset of type 1 diabetes.
Available evidence suggests that T-cell co-stimulation blocking by CTLA4-Ig is effective in prolonging the survival of islet transplants in vivo and associated with much lower side effects than general immunosuppression.
CTLA4-Ig was able to slow the reduction of beta-cell function early in the development of the disease (within 3 months of diabetes), and the effect was continued 1 year after the cessation of treatment but without much improvement.
The addition of other agents can be considered for a synergistic effect in prolonging allograft survival, particularly islet allografts.
The reduction of central memory CD4+ T cells by CTLA4-Ig can be used as a replacement marker for C-peptide reduction in recently diagnosed type 1 diabetes patients.
The immune tolerability of CTLA4-Ig is not long-lasting and may be associated with resistance due to B lymphocyte alterations. The effect appears to be for a short period of time since the autoimmunity subsides with time in type 1 diabetes patients.
Declaration of interest
The authors have no relevant affiliations or financial involvement with any organization or entity with a financial interest in or financial conflict with the subject matter or materials discussed in the manuscript. This includes employment, consultancies, honoraria, stock ownership or options, expert testimony, grants or patents received or pending, or royalties.
Reviewer disclosures
One reviewer has served on advisory boards for Caladrius, Immunomolecular Therapeutics and Tolerion and had an affiliation with Novo Nordisk. Peer reviewers on this manuscript have no other relevant financial or other relationships to disclose.
Additional information
Funding
References
- Federation ID. IDF diabetes atlas brussels. 8th edn. Belgium: 2017 [updated 2019 Nov 30]. Available from: http://www.diabetesatlas.org.
- Atkinson MA, Eisenbarth GS, Michels AW. Type 1 diabetes. Lancet. 2014;383(9911):69–82.
- Brooks-Worrell B, Palmer JP. Prevention versus intervention of type 1 diabetes. Clin Immunol. 2013;149(3,Part A):332–338.
- Bonfanti R, Bazzigaluppi E, Calori G, et al. Parameters associated with residual insulin secretion during the first year of disease in children and adolescents with type 1 diabetes mellitus. Diabetic Med. 2004;15(10):844–850.
- Sochett EBDD, Clarson C, Ehrlich RM. Factors affecting and patterns of residual insulin secretion during the first year of type 1 (insulin-dependent) diabetes mellitus in children. Diabetologia. 1987 Jul;30(7):453–459.
- Association AD. 2. Classification and diagnosis of diabetes: standards of medical care in diabetes—2018. Diabetes Care. 2018;41(Supplement 1):S13–S27.
- Narendran P. Screening for type 1 diabetes: are we nearly there yet? Diabetologia. 2019;62(1):24–27.
- Skyler JS, Bakris GL, Bonifacio E, et al. Differentiation of diabetes by pathophysiology, natural history, and prognosis. Diabetes. 2017;66(2):241–255.
- Insel RA, Dunne JL, Atkinson MA, et al. Staging presymptomatic type 1 diabetes: a scientific statement of JDRF, the endocrine society, and the american diabetes association. Diabetes Care. 2015;38(10):1964–1974.
- Fiorina P, Shapiro AMJ, Ricordi C, et al. The clinical impact of islet transplantation. Am J Transplant. 2008;8(10):1990–1997.
- Sora ND, Shashpal F, Bond EA, et al. Insulin pumps: review of technological advancement in diabetes management. Am J Med Sci. 2019;358(5):326–331.
- Pease AJ, Lo C, Earnest A, et al. The efficacy of technology in type 1 diabetes: a systematic review, network meta-analysis, and narrative synthesis. Diabetes Technol Ther. 2020 Feb 5. doi:10.1089/dia.2019.0417. [Epub ahead of print]
- FDA. MiniMed 670G system - P160017/S017: U.S. food & drug administration. 2018 [updated 2019 Nov 30]. Available from: https://www.fda.gov/medical-devices/recently-approved-devices/minimed-670g-system-p160017s017.
- FDA. FDA authorizes first interoperable, automated insulin dosing controller designed to allow more choices for patients looking to customize their individual diabetes management device system. U.S. Food & Drug Administration. 2019 [updated 2019 Dec 13]. Available from: https://www.fda.gov/news-events/press-announcements/fda-authorizes-first-interoperable-automated-insulin-dosing-controller-designed-allow-more-choices.
- Brown SA, Kovatchev BP, Raghinaru D, et al. Six-month randomized, multicenter trial of closed-loop control in type 1 diabetes. N Engl J Med. 2019;381(18):1707–1717.
- Gandhi GY, Kovalaske M, Kudva Y, et al. Efficacy of continuous glucose monitoring in improving glycemic control and reducing hypoglycemia: a systematic review and meta-analysis of randomized trials. J Diabetes Sci Technol. 2011;5(4):952–965.
- Simmons KM, Gottlieb PA, Michels AW. Immune intervention and preservation of pancreatic beta cell function in type 1 diabetes. Curr Diab Rep. 2016;16(10):97.
- Roep BO, Tree TIM. Immune modulation in humans: implications for type 1 diabetes mellitus. Nat Rev Endocrinol. 2014;10:229.
- Bluestone JA, St Clair EW, Turka LA. CTLA4Ig: bridging the basic immunology with clinical application. Immunity. 2006;24(3):233–238.
- Salomon B, Bluestone JA. Complexities of CD28/B7: CTLA-4 costimulatory pathways in autoimmunity and transplantation. Annu Rev Immunol. 2001;19(1):225–252.
- Ludvigsson J. Therapies to preserve β-cell function in type 1 diabetes. Drugs. 2016;76(2):169–185.
- Palmer JP, Fleming GA, Greenbaum CJ, et al. C-peptide is the appropriate outcome measure for type 1 diabetes clinical trials to preserve β-cell function. Diabetes. 2004;53(1):250.
- Cabello-Olmo M, Arana M, Radichev I, et al. New Insights into immunotherapy strategies for treating autoimmune diabetes. Int J Mol Sci. 2019;20:19.
- Couri CEB, Malmegrim KCR, Oliveira MC. New horizons in the treatment of type 1 diabetes: more intense immunosuppression and beta cell replacement. Front Immunol. 2018;9:1086.
- Brunet J-F, Denizot F, Luciani M-F, et al. A new member of the immunoglobulin superfamily—CTLA-4. Nature. 1987;328:267.
- Lindsten T, Lee KP, Harris ES, et al. Characterization of CTLA-4 structure and expression on human T cells. J Immunol. 1993;151(7):3489.
- Krummel MF, Allison JP. CD28 and CTLA-4 have opposing effects on the response of T cells to stimulation. J Exp Med. 1995;182(2):459.
- Walunas TL, Lenschow DJ, Bakker CY, et al. Pillars article: CTLA-4 can function as a negative regulator of T cell activation. Immunity. 1994;1:405–413. The Journal of Immunology. 2011;187(7):3466.
- Larsen CP, Elwood ET, Alexander DZ, et al. Pillars article: long-term acceptance of skin and cardiac allografts after blocking CD40 and CD28 pathways. Nature. 1996;381:434–438, 1996. The Journal of Immunology. 2011;186(5):2693.
- Lin H, Bolling SF, Linsley PS, et al. Long-term acceptance of major histocompatibility complex mismatched cardiac allografts induced by CTLA4Ig plus donor-specific transfusion. J Exp Med. 1993;178(5):1801.
- Lenschow DJ, Zeng Y, Thistlethwaite, et al. Long-term survival of xenogeneic pancreatic islet grafts induced by CTLA4lg. Science. 1992;257(5071):789.
- Zhai C, Yu L, Zhu H, et al. Porcine CTLA4‐Ig prolong islet xenografts in rats by downregulating the direct pathway of T‐cell activation. Xenotransplantation. 2011;18(1):40–45.
- Yang D-F, Qiu W-H, Zhu H-F, et al. CTLA4-Ig-modified dendritic cells inhibit lymphocyte-mediated alloimmune responses and prolong the islet graft survival in mice. Transpl Immunol. 2008;19(3):197–201.
- Pawlick R, Gala‐Lopez B, Pepper AR, et al. The combination of anti‐NKG2D and CTLA‐4 Ig therapy prolongs islet allograft survival in a murine model. Am J Transplant. 2014;14(10):2367–2374.
- Wang X, Hao J, Metzger DL, et al. Blockade of both B7-H4 and CTLA-4 co-signaling pathways enhances mouse islet allograft survival. Islets. 2012;4(4):284–295.
- Moreland LW, Alten R, Van Den Bosch F, et al. Costimulatory blockade in patients with rheumatoid arthritis: A pilot, dose-finding, double-blind, placebo-controlled clinical trial evaluating CTLA-4Ig and LEA29Y eighty-five days after the first infusion. Arthritis Rheumatism. 2002;46(6):1470–1479.
- Ruderman EM, Pope RM. The evolving clinical profile of abatacept (CTLA4–ig): a novel co-stimulatory modulator for the treatment of rheumatoid arthritis. Arthritis Res Ther. 2005;7(2):S21.
- Orban T, Bundy B, Becker DJ, et al. Co-stimulation modulation with abatacept in patients with recent-onset type 1 diabetes: a randomised double-masked controlled trial. Lancet. 2011;378(9789):412–419.
- Orban T, Bundy B, Becker DJ, et al. Costimulation modulation with abatacept in patients with recent-onset type 1 diabetes: follow-up 1 year after cessation of treatment. Diabetes Care. 2014;37(4):1069–1075.
- Ursini F, Russo E, Letizia Hribal M, et al. Abatacept improves whole-body insulin sensitivity in rheumatoid arthritis: an observational study. Medicine (Baltimore). 2015;94(21):e888.
- Administration USFD. Orphan drug designations and approvals. 2013 [updated 2019 Nov 30]. Available from: https://www.accessdata.fda.gov/scripts/opdlisting/oopd/detailedIndex.cfm?cfgridkey=395713.
- FDA. Drugs@FDA: FDA-Approved drugs: U.S. Food & drug administration. 2005. [updated 2019 Mar 21]. Available from: https://www.accessdata.fda.gov/scripts/cder/daf/index.cfm?event=BasicSearch.process.
- Luo X, Herold KC, Miller SD. Immunotherapy of type 1 diabetes: where are we and where should we be going? Immunity. 2010;32(4):488–499.
- Rakieh C, Conaghan PG. Comparative clinical utility of once-weekly subcutaneous abatacept in the management of rheumatoid arthritis. Ther Clin Risk Manag. 2014;10:313–320.
- Weinblatt ME, Moreland LW, Westhovens R, et al. Safety of abatacept administered intravenously in treatment of rheumatoid arthritis: integrated analyses of up to 8 years of treatment from the abatacept clinical trial program. J Rheumatol. 2013;40(6):787.
- Alten R, Kaine J, Keystone E, et al. Long-term safety of subcutaneous abatacept in rheumatoid arthritis: integrated analysis of clinical trial data representing more than four years of treatment. Arthritis Rheumatol (Hoboken). 2014;66(8):1987–1997.
- Nash P, Nayiager S, Genovese Mark C, et al. Immunogenicity, safety, and efficacy of abatacept administered subcutaneously with or without background methotrexate in patients with rheumatoid arthritis: results from a phase iii, international, multicenter, parallel‐arm, open‐label study. Arthritis Care Res (Hoboken). 2013;65(5):718–728.
- Iwahashi M, Inoue H, Matsubara T, et al. Efficacy, safety, pharmacokinetics and immunogenicity of abatacept administered subcutaneously or intravenously in Japanese patients with rheumatoid arthritis and inadequate response to methotrexate: a phase II/III, randomized study. Mod Rheumatol. 2014;24(6):885–891.
- Genovese MC, Covarrubias A, Leon G, et al. Subcutaneous abatacept versus intravenous abatacept: a phase IIIb noninferiority study in patients with an inadequate response to methotrexate. Arthritis Rheum. 2011;63(10):2854–2864.
- Kaine J, Gladstein G, Strusberg I, et al. Evaluation of abatacept administered subcutaneously in adults with active rheumatoid arthritis: impact of withdrawal and reintroduction on immunogenicity, efficacy and safety (phase Iiib ALLOW study). Ann Rheum Dis. 2012;71(1):38–44.
- Lahaye CSM, Mulliez A, Bardin T, et al. French society of rheumatology. Effectiveness and safety of abatacept in elderly patients with rheumatoid arthritis enrolled in the French society of rheumatology’s ORA registry. Rheumatology (Oxford). 2016;55(5):874–882.
- Mease PJ, Gottlieb AB, van der Heijde D, et al. Efficacy and safety of abatacept, a T-cell modulator, in a randomised, double-blind, placebo-controlled, phase III study in psoriatic arthritis. Ann Rheum Dis. 2017;76(9):1550–1558.
- Westhovens R, Robles M, Ximenes AC, et al. Clinical efficacy and safety of abatacept in methotrexate-naive patients with early rheumatoid arthritis and poor prognostic factors. Ann Rheum Dis. 2009;68(12):1870–1877.
- Zhou Z, Tay LK, Pfister M, et al. Characterizing abatacept exposure in juvenile an d adult arthritis patients using a unified population exposure model. Clin Pharmacol Ther. 2008;83(Abst PIII–64):S91.
- Lon H-K, Liu D, DuBois DC, et al. Modeling pharmacokinetics/pharmacodynamics of abatacept and disease progression in collagen-induced arthritic rats - A population approach. J Pharmacokinet Pharmacodyn. 2013;40(6):701–712.
- Hasegawa M, Imai Y, Hiraoka M, et al. Model-based determination of abatacept exposure in support of the recommended dose for Japanese rheumatoid arthritis patients. J Pharmacokinet Pharmacodyn. 2011;38(6):803–832.
- Weiner RS, Srinivas NR, Calore JD, et al. A sensitive enzyme immunoassay for the quantitation of human CTLA4Ig fusion protein in mouse serum: pharmacokinetic application to optimizing cell line selection. J Pharm Biomed Anal. 1997;15(5):571–579.
- Srinivas NRWR, Shyu WC, Calore JD, et al. A pharmacokinetic study of intravenous CTLA4Ig, a novel immunosuppressive agent, in mice. J Pharm Sci. 1996;85(3):296–298.
- Srinivas NRWR, Warner G, Shyu WC, et al. Pharmacokinetics and pharmacodynamics of CTLA4lg (BMS-188667), a novel immunosuppressive agent, in monkeys following multiple doses. J Pharm Sci. 1996;85(1):1–4.
- Srinivas NR, Shyu WC, Weiner RS, et al. Pharmacokinetics of CTLA4Ig (BMS-188667), a novel immunosuppressive agent, following intravenous and subcutaneous administration to mice. J Pharm Sci. 1995;84(12):1488–1489.
- Li X, Roy A, Murthy B. Population pharmacokinetics and exposure-response relationship of intravenous and subcutaneous abatacept in patients with rheumatoid arthritis. J Clin Pharmacol. 2019;59(2):245–257.
- Ma Y, Lin B-R, Lin B, et al. Pharmacokinetics of CTLA4Ig fusion protein in healthy volunteers and patients with rheumatoid arthritis. Acta Pharmacol Sin. 2009;30(3):364–371.
- Srinivas NR, Shyu WC, Weiner RS, et al. Assessment of dose proportionality, absolute bioavailability, and immunogenicity response of CTLA4Ig (BMS-188667), a novel immunosuppressive agent, following subcutaneous and intravenous administration to rats. Pharm Res. 1997;14(7):911–916.
- Srinivas NR, Weiner RS, Berry K, et al. Pharmacokinetics and pharmacodynamics of CTLA4Ig (BMS‐188667), a novel immunosuppressive agent, after subcutaneous and intravenous administration to mice. Pharm. Pharmacol. Commun. 1998;4(7):355–360.
- Dayan CM, Korah M, Tatovic D, et al. Changing the landscape for type 1 diabetes: the first step to prevention. Lancet. 2019;394(10205):1286–1296.
- Assan R, Debray-Sachs M, Laborie C, et al. Metabolic and immunological effects of cyclosporin in recently diagnosed type 1 diabetes mellitus. Lancet. 1985;325(8420):67–71.
- Stiller CR, Dupre J, Gent M, et al. Effects of cyclosporine immunosuppression in insulin-dependent diabetes mellitus of recent onset. Science. 1984;223(4643):1362.
- Mastrandrea L, Yu J, Behrens T, et al. Etanercept treatment in children with new-onset type 1 diabetes: pilot randomized, placebo-controlled, double-blind study. Diabetes Care. 2009;32(7):1244–1249.
- Sherry N, Hagopian W, Ludvigsson J, et al. Teplizumab for treatment of type 1 diabetes (Protégé study): 1-year results from a randomised, placebo-controlled trial. Lancet. 2011;378(9790):487–497.
- Hagopian W, Ferry RJ, Sherry N, et al. Teplizumab preserves C-peptide in recent-onset type 1 diabetes: two-year results from the randomized, placebo-controlled protégé trial. Diabetes. 2013;62(11):3901–3908.
- Herold KC, Bundy BN, Long SA, et al. An anti-CD3 antibody, teplizumab, in relatives at risk for type 1 diabetes. N Engl J Med. 2019;381(7):603–613.
- Herold KC, Bundy BN, Krischer JP. Type 1 diabetes trialnet study G. Teplizumab in relatives at risk for type 1 diabetes. Reply. N Engl J Med. 2019;381(19):1880–1881.
- Aronson R, Gottlieb PA, Christiansen JS, et al. Low-dose otelixizumab anti-CD3 monoclonal antibody DEFEND-1 study: results of the randomized phase III study in recent-onset human type 1 diabetes. Diabetes Care. 2014;37(10):2746–2754.
- Keymeulen B, Candon S, Fafi-Kremer S, et al. Transient Epstein-Barr virus reactivation in CD3 monoclonal antibody-treated patients. Blood. 2010;115(6):1145.
- Herold KC, Pescovitz MD, McGee P, et al. Increased T cell proliferative responses to islet antigens identify clinical responders to anti-CD20 monoclonal antibody (rituximab) therapy in type 1 diabetes. J Immunol. 2011;187(4):1998–2005.
- Pescovitz MD, Greenbaum CJ, Krause-Steinrauf H, et al. Rituximab, B-lymphocyte depletion, and preservation of beta-cell function. N Engl J Med. 2009;361(22):2143–2152.
- Orban T, Beam CA, Xu P, et al. Reduction in CD4 central memory T-cell subset in costimulation modulator abatacept-treated patients with recent-onset type 1 diabetes is associated with slower C-peptide decline. Diabetes. 2014;63(10):3449–3457.
- Greenbaum CJ, Beam CA, Boulware D, et al. Fall in C-peptide during first 2 years from diagnosis: evidence of at least two distinct phases from composite type 1 diabetes trialnet data. Diabetes. 2012;61(8):2066–2073.
- TrialNet TD. Abatacept prevention study. 2018 [updated 2019 Nov 30]. Available from: https://www.trialnet.org/our-research/prevention-studies/abatacept.
- Cabrera SM, Engle S, Kaldunski M, et al. Innate immune activity as a predictor of persistent insulin secretion and association with responsiveness to CTLA4-Ig treatment in recent-onset type 1 diabetes. Diabetologia. 2018;61(11):2356–2370.
- Linsley PS, Greenbaum CJ, Speake C, et al. B lymphocyte alterations accompany abatacept resistance in new-onset type 1 diabetes. JCI Insight. 2019;4:4.