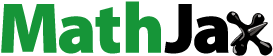
Abstract
Purpose
To study the relationship between inhalation of airborne particles and cobalt in the Swedish hard metal industry and markers of inflammation and coagulation in blood.
Methods
Personal sampling of inhalable cobalt and dust were performed for subjects in two Swedish hard metal plants. Stationary measurements were used to study concentrations of inhalable, respirable, and total dust and cobalt, PM10 and PM2.5, the particle surface area and the particle number concentrations. The inflammatory markers CC16, TNF, IL-6, IL-8, IL-10, SAA and CRP, and the coagulatory markers FVIII, vWF, fibrinogen, PAI-1 and D-dimer were measured. A complete sampling was performed on the second or third day of a working week following a work-free weekend, and additional sampling was taken on the fourth or fifth day. The mixed model analysis was used, including covariates.
Results
The average air concentrations of inhalable dust and cobalt were 0.11 mg/m3 and 0.003 mg/m3, respectively. For some mass-based exposure measures of cobalt and total dust, statistically significant increased levels of FVIII, vWF and CC16 were found.
Conclusions
The observed relationships between particle exposure and coagulatory biomarkers may indicate an increased risk of cardiovascular disease.
Introduction
Occupational exposure to cobalt is well established in the hard metal industry, which produces cutting tools that are mainly used to manufacture industrial products and parts. Hard metal is a group of composite materials that consist predominantly of the hard tungsten carbide (WC) particulate phase tied together with cobalt as a binder (Sadik Citation2015). Nickel in the metallic state could also be added.
Exposure to cobalt in the hard metal industry has been associated with adverse health effects such as rhinitis, sinusitis (Balmes Citation1987), and respiratory effects, including bronchitis and decreased lung function over time (Cugell et al. Citation1990, Rehfisch et al. Citation2012), as well as hard metal lung disease (HMLD) (Nakamura et al. Citation2014). Allergic dermatitis has also been demonstrated (Julander et al. Citation2009). Obstructive lung disease such as asthma has been associated with cobalt exposure (Kusaka et al. Citation1989, Shirakawa et al. Citation1989) and some cases of cardiomyopathy (Barborik and Dusek Citation1972, Kennedy et al. Citation1981). An increased risk of developing lung cancer has also been demonstrated for people working in the hard metal production industry (Lasfargues et al. Citation1994, Wild et al. Citation2000, Moulin et al. Citation1998).
Regarding cardiovascular disease (CVD) and cobalt exposure in the hard metal industry, two French studies failed to present statistically significant excess risk on CVD and ischaemic heart disease (IHD) (Moulin et al. Citation1998, Wild et al. Citation2000). A Swedish cohort of hard metal workers, followed up from 1952 to 1982, presented an increased risk for high level and long term exposed workers regarding IHD (SMR 1.69, 95% CI 0.96–2.75) (Hogstedt and Alexandersson Citation1990). A later Swedish cohort of white and blue-collar workers presented an increased risk of IHD but not CVD (Westberg et al. Citation2017). In a larger study, comprised 32,354 workers from five countries, including the previous Swedish study short-term exposed workers (<1 year) had a higher IHD mortality (SMR 1.41, 95% CI 1.30–1.52) compared with long-term exposed workers (≥1 year) (SMR 1.01, 95% CI 0.94–1.07) (Marsh et al. Citation2017).
Mechanistic evidence from animal and human studies suggests that increases in mortality due to cardiovascular diseases are associated with systemic inflammatory responses. Exposures to ambient particulate matter are linked to this outcome (Brook et al. Citation2010). The inflammatory causal link between the inhalation of particulate matter and cardiovascular disease was first proposed in the mid-1990s (Seaton et al. Citation1995, Sjögren Citation1997). Later publications revealed some of the mechanisms underpinning this link, notably the formation of the NLRP3 inflammasome upon exposure of intracellular receptors to particles, which leads to the activation of the enzyme caspase-1, and subsequently activation of interleukin IL-1β (Dostert et al. Citation2008, Dagenais et al. Citation2012, Peeters et al. Citation2013).
Several inflammatory markers, including IL-6, C-reactive protein (CRP), and fibrinogen, are established risk factors for cardiovascular disease (Danesh Citation2000, Danesh et al. Citation2005, Citation2008). Serum amyloid A (SAA) has also been proposed as a risk factor for cardiovascular disease as a component of a battery of several biomarkers (Arant et al., Citation2009). In a series of studies, we have determined exposure-responses for various inflammatory markers concerning particle exposure in mechanical and welding workshops, cement plants, aluminium foundries, the pulp and paper industry and iron foundries (Ohlson et al. Citation2010, Westberg et al. Citation2016, Citation2019a). In a recent study on respiratory health among workers in the Swedish hard metal industry, increased serum levels of the club cell secretory protein CC16 was found (Andersson et al. Citation2020).
Here, we extend our work to cobalt exposure in the Swedish hard metal industry to investigate possible exposure-response relationships between particulate exposure (quantified in terms of levels of personal inhalable dust and cobalt, particle mass, number, and surface area concentrations in the air) and markers of inflammation and coagulation.
Methods
Study group
The study was performed at two Swedish hard metal industries, employing 130 and 1400 workers, respectively. The two industries use similar processes which cause similar variations in exposures. The production during the measurement days produced 18,500–44,000 pieces and 1,484,000–1,542,000 pieces of hard metal products, respectively. The hard metal details produced are based on tungsten carbide, where cobalt is used as a binder. The companies produce smaller details of cemented carbide materials for cutting work and rock drilling of 10–250 gram/piece and larger products for the car and aeroplane industry of 10–50 kg.
The departments included were forming (prototype), laboratory, pressing, powder and maintenance. The pressing and powder departments represented the majority of the workers. Hard-metal workers, primarily the dayshift, participated in the study, a total of 72 subjects, 63 men and nine women. Their mean age was 42 years, ranging from 20 to 65. The majority of the workers were between 30 and 65 years. A total of 22% were under the age of 30, and only 9% were above 60 years old. They had worked in the same place for 11 years on average, ranging up to 45 years of employment. In total, 62 participants reported their smoking habits: 2 were current smokers, 12 ex-smokers, and 48 participants had never smoked.
Study design
The study was performed between March 2017 and October 2018 at six separate 4-day campaigns. The investigation comprised air sampling of inhalable dust and cobalt and biological sampling of blood and urine to analyse cobalt in blood and urine and coagulatory and inflammatory markers in the blood. The air sampling of inhalable dust and cobalt was performed on day 2 or 3 after a work-free weekend. Blood sampling was performed the same day as the air sampling after the work shift and followed up after 2 days, i.e. day 4 or 5 at work after the shift to studying differences between different days in the week. In addition, to estimate cobalt uptake during the current week, cobalt in the blood and urine were sampled after the shift. In total, blood and urine sampling was performed twice for each subject. All participants completed a questionnaire containing items about current and previous working conditions, height and weight, smoking habits, medication, and symptoms of respiratory irritation, infection, or other inflammation, which could influence biomarker levels. All participants were at work during our investigation. Ten workers reported chronic diseases such as mental disorders, heart diseases, hypertension, ulcerative colitis, asthma or multiple sclerosis; these and other findings in our medical investigation were reported to the local company health service after individual consent. All these workers with potential inflammatory-connected diseases were included in the analysis.
Aerosol measurements and cobalt analysis
The respiratory tract comprises three regions: the extrathoracic region (including the nose and throat), the thoracic region, and the alveolar region. Particles that enter the extrathoracic region constitute the inhalable fraction, those that penetrate the thoracic region constitute the thoracic fraction, and particles deposited into the alveolar region constitute the respirable fraction. The PM10 and PM2.5 fractions correspond to the thoracic and respirable fractions, respectively (Vincent Citation2005).
Personal sampling of inhalable dust and cobalt was carried out as 8-h full-shift samples according to standards for measurement of inhalable dust using GSP-samplers (GSA Messgerätebau GmbH, Ratingen, Germany) with a 37 mm cellulose acetate filter 3 µm pore-sized and a sampling pump (SKC AirCheck XR5000, Eighty Four PA, USA or GSA SG5100, GSA Messgerätebau GmbH, Ratingen, Germany) operating at an air flow of 3.5 L/min (HSE Citation2000). For the determination of cobalt in the dust, the filters and dust were then dissolved with acid and analysed by inductively coupled plasma mass spectrometry (ICP-MS) (National Institute of Occupational Health [NIOSH] Citation2014). ICP-MS also analysed cobalt in the blood and urine.
Stationary measurements (area measurements) were acquired using measuring rigs. These measurements were conducted in the departments where the participants worked during their shifts and included a sampling of inhalable dust and cobalt using the same techniques for personal samples. Total dust was determined by collecting 8-h full shift samples according to a modified gravimetric method (NIOSH Citation1994) using 25 mm cellulose acetate filter in open-faced cassettes (OFC) with an airflow of 2.0 L/min. Respirable dust was determined using SKC aluminium cyclones (SKC, Eighty Four PA, USA) with 25 mm cellulose acetate filters, operating at an airflow of 2.5 L/min (Health and Safety Executive [HSE] 2000). Air concentrations of PM10 and PM2.5 were collected on 37 mm cellulose acetate filters using a Chempass system with an airflow of 1.8 L/min (European Standard Citation2014). For PM1.0, a Dusttrak DRX Monitor 8533 (TSI Incorporated, Shoreview MN, USA) was used. The particle surface area air concentrations were determined using a nanoparticle aerosol monitor Aerotrak 9000 (TSI Incorporated, Shoreview MN, USA) instrument, which determines the particle surface area for particles in the size range of 10 to 1000 nm in real-time. Particle number concentrations were measured based on particles in the 20–1000 nm size range using an ultrafine particle counter P-Trak 8525 (TSI Incorporated, Shoreview MN, USA). The number of nanoparticles was measured using a NanoTracerXP (Oxility B.V., Eindhoven, The Netherlands), a real-time instrument detecting ultra-fine particles in the 10–300 nm size range.
Markers of inflammation and coagulation
Blood plasma and serum was collected at the end of a work shift and stored on dry ice until they could be transported and stored at −70° C in a biobank. The concentrations of interleukins (IL-6, IL-8, IL-10 and tumour necrosis factor (TNF) were determined with a meso QuickPlex SQ 120 instrument (Mesoscale Diagnostics, Rockville, MD, USA) using their Vplex immunoassays. High-sensitivity CRP, fibrinogen, D-dimer, and blood groups (ABO) were determined at the Clinical Research Centre, Örebro University Hospital, Sweden. Serum Amyloid A (SAA), an acute-phase protein used as a marker for systemic inflammation, in serum was analysed using a commercial ELISA kit from Invitrogen (Massachusetts, United States). The surfactant protein Club Cell protein 16 (CC16) in serum was analysed using a commercial ELISA-kit from R&D Systems (Minneapolis, United States). Plasminogen activator inhibitor-1 (PAI-1) activity in plasma was analysed using the bio immunoassay Trinilize from Tcoag (Bray, Ireland). The coagulation factor VIII (FVIII) was analysed using a one-stage clotting assay, and the von Willebrand factor antigen (vWf) was determined with immunoturbidimetric, both on the BCS XP analyser from Siemens Healthcare (Marburg, Germany), with reagents from that manufacturer.
SAA and CC16 were analysed in-house at the Department of Occupational and Environmental Medicine, and PAI-1, FVIII and vWf at the Department of Clinical Chemistry, all at Sahlgrenska University Hospital, Göteborg, Sweden.
Exposure measures
Exposure was assessed by performing personal sampling to measure inhalable dust and cobalt concentrations and by performing stationary measurements in each department that served as proxies for personal exposure of total and respirable dust, corresponding cobalt concentrations, PM10, PM2.5, PM1.0, nanoparticles, particle surface area, and particle number concentrations in the air. Respirators were used by 26 participants at some time while working. These subjects' raw measurements were adjusted to assess their true exposure better to account for the respirators’ effects. Specifically, it was assumed that these workers experienced zero exposure while wearing a respirator mask; their exposure during the remainder of their shifts was estimated based on either personal sampling or the measured background concentrations for the departments they worked for a while not wearing respirators. In addition to time-weighted averages (TWA) and cumulative exposures, afternoon samples of cobalt in the blood and urine were taken.
Three exposure measures were computed: the non-adjusted exposure (Caverage) as determined by personal sampling; the average adjusted (Caverage adjusted) exposure based on the assumption of null exposure when using a respirator, and the uncorrected exposure determined by personal sampling for the rest of the day; and the background-adjusted (Cbackground adjusted) exposure based on the assumption of null exposure when using a respirator and the background exposure determined by stationary sampling in the relevant departments. For descriptive purposes, we have used non-adjusted cobalt levels. In our linear regression and mixed model analysis, we have used adjusted levels.
Exposures are presented as air concentrations of inhalable dust and cobalt (mg/m3) and cumulative air concentrations (mg/m3year). We used data from a linear regression analysis of cobalt air concentrations to calculate cumulative cobalt exposure measures based on extracted measurement data in our database in hard-metal industries (Westberg et al. Citation2017) and data on dust cobalt from 2006 to 2018 for our two industries. To model exposures for the workers, cobalt exposure concentrations for different 10-year periods were determined at our two companies for the departments' design/prototype, laboratory, pressing, powder, and maintenance (Andersson et al. Citation2020). Exposure proxies based on the stationary samples were presented for the mass based measures as mg/m3, for number of particles as number/cm3 and for particle surface area as µm2/cm3.
Statistical analysis
For descriptive purposes, the measured aerosol concentrations, i.e. the concentrations of inhalable, total, and respirable dust and cobalt, PM10 and PM2.5, as well as the particle surface area (A-Trak), particle number concentration (P-trak) and nanoparticles are reported as 8-h TWAs for the full workday. In addition, afternoon samples of cobalt in blood and urine were analysed. Standard parameters such as arithmetic means (AM), standard deviations (SD), as well as geometric means (GM), geometric standard deviations (GSD), and ranges were calculated based on the log-normal distributions of each measurement, including the stationary samples of the aerosol mass fractions, the particle number and particle surface area concentrations, nanoparticles and cobalt in the blood (). Exposure data by job title for inhalable dust, cobalt and tungsten are presented in . The corresponding descriptions, means, and ranges of the measured blood concentrations of inflammatory and coagulatory markers by sampling day are presented in . The analyses presented below are based on background-adjusted inhalable dust and cobalt particle data.
Table 1. Exposure concentration levels of inhalable dust and cobalt. Stationary concentration levels of inhalable, total and respirable dust, inhalable, total and respirable cobalt, PM10, PM2.5, PM1.0, particle surface area (A-trak), particle number (P-trak) and nanoparticles (Nanotracer). Non-adjusted.
Table 2. Exposure concentration levels of inhalable dust, cobalt and tungsten by job title. Non-adjusted.
Table 3. Blood concentrations of biological markers and cobalt in blood and urine (mean, median minimum and maximum) among 71 participants on different days.
Table 4. A mixed model for inflammatory markers CC16, TNF, IL-6, IL-8, IL-10, SAA and CRP by exposure class of particle mass, particle number, particle area and air concentrations.
A linear mixed model was developed to describe the relationship between the different TWA-exposure metrics and inflammatory markers. Our design and mixed model use made it possible to consider within- and between worker variability based on our repeated measurements of inflammatory markers. We used a mixed model to study the variation in biomarker levels for exposure categories 1–3 (low, mid and high tertiles, ). A linear multiple regression model was used to study the corresponding variation between cumulative exposures and biomarkers (Tables 7–10, Supplemental Appendix 1). Because the distribution of inflammatory markers was skewed, the biomarker data were log-transformed. The estimates (β) of the model's fixed effects allowed us to identify factors affecting inflammatory markers. The equation of the final mixed model is:
Y = ln(X); X and Y are the measured and log-transformed inflammatory marker concentrations, respectively. µ = the overall average inflammatory marker concentration on the log-scale. β1= the fixed effect of the exposure measure in question (i….j) in the given exposure group, with the lowest exposure group (exposure class 1) serving as the reference category. The exposure and stationary measurement data were categorised into three equally sized classes (tertials). The same method was used for cobalt in blood and urine. β2 = the fixed effect of sampling day. β3 = the fixed effect of gender (male or female), with males serving as the reference category. β4 = the fixed effect of BMI, dichotomised by median; the highest level was used as the reference category. β5 = the fixed effect of smoking habits; categories considered were smoker, ex-smoker, and lifetime non-smoker, with the latter being the reference category. β6 = the fixed effect of age dichotomised by median; the oldest group served as the reference category. β7 = the fixed effect of blood group (0), with other blood groups as the reference category. ε = residual
Table 5. A mixed model for coagulatory markers FVIII, vWF, Fibrinogen, PAI-1 and D-dimer by exposure class of particle mass, particle number, particle area and air concentrations.
Table 6. A mixed model for inflammatory (CC16, TNF, IL-6, IL-8, IL-10, SAA, CRP) and coagulatory markers (FVIII, vWF, Fibrinogen, PAI-1, D-dimer) by cobalt in blood and urine.
The results of the mixed model analysis are presented as β values, and 95% confidence intervals and is also presented as significant or non-significant exposure-response when the reference category < the middle tertile < the highest tertile for positive exposure-responses and the reference category > the middle tertile > the highest tertile for negative exposure-responses. The statistical significance threshold was p < 0.05. All analyses were performed with SPSS 22.0.
Results
Exposure
The 8-h TWAs for the inhalable cobalt exposures measured by the personal sampling of the 72 participants ranged from 0.00016 to 0.019 mg/m3, with an arithmetic mean of 0.0034 mg/m3. In contrast, the mean and maximum inhalable cobalt exposures determined by stationary measurement were 0.00076 mg/m3 and 0.0041 mg/m3, respectively (). The highest personal exposures were observed in the powder production, pressing and forming processes, for which the maximum was 0.019, 0.010, and 0.0034 mg/m3, respectively ().
For the personal measurements for the 26 employees using respirators, we calculated adjusted exposure concentrations based on zero exposure while using respirator and background exposure. The adjusted average inhalable cobalt and dust concentrations were 0.0017 and 0.079 mg/m3, respectively. The highest adjusted cobalt exposures were observed in the powder and pressing departments, at least 0.0020 and 0.0019 mg/m3, respectively (not in the table). The Swedish occupational exposure limits (OEL) for inhalable cobalt and dust are 0.02 and 5 mg/m3, respectively.
Markers of inflammation and coagulation
The measured plasma or serum levels of biological markers of inflammation and coagulation are presented in . These results were used to develop the mixed model for inflammatory and coagulatory markers (). The exposure-response analysis was based on personal exposure and stationary particle mass, number, and surface area concentration measurements and TWA of exposure and results of biological sampling of cobalt in blood and urine.
The most consistent findings were a statistically significant exposure-response for FVIII and total dust exposure and corresponding statistically significant increased β-values (representing the fixed effects of exposure) in the highest exposure group for FVIII, vWF and CC16. The particulate fractions associated with these responses were for FVIII inhalable dust, inhalable cobalt, total dust, total dust cobalt, respirable dust, respirable cobalt, PM10 and PM2.5, for vWF respirable cobalt and for CC16 for cobalt in total dust. For cobalt in urine, a significant decrease was noted for fibrinogen at the highest tertile. For cobalt in the blood, a similar finding for the middle tertial for D-dimer was determined. The inflammatory and coagulatory outliers were scrutinised, and none were associated with significant exposure-response relationships.
Non-significant positive exposure-response for the inflammatory markers were determined for CRP and inhalable and total dust, total and respirable cobalt, IL-6 inhalable cobalt, total dust cobalt, for IL-8 and total dust cobalt, for CC16 inhalable dust, inhalable cobalt, total dust cobalt, and P-tra-k. For the coagulatory markers, the following was shown: for PAI-1 for inhalable dust, total dust cobalt, for fibrinogen for inhalable cobalt, inhalable dust, total dust, for vWF for respirable cobalt, PM2.5, for FVIII for almost all exposure variables. The same pattern was determined for CC16 and CRP for cobalt in blood and TNF, vWF, and PAI-1 for cobalt in the urine. A corresponding non-significant negative exposure-response was determined for TNF and PM2.5, A–trak; IL-6 and inhalable dust, PM2.5; IL-8 PM2.5, PM1.0; IL-10 and A-trak; SAA and inhalable dust, PM2.5, cobalt in the blood; TNF, CRP and SAA, cobalt in urine; IL-6, D-dimer, fibrinogen and for inhalable cobalt nanoparticles; PAI-1 and PM2.5; D-dimer and nanoparticles.
Using cumulative exposures for each individual based on the personal sampling of inhalable dust and cobalt showed for inhalable cobalt non-significant exposure-response for SAA, CC16, fibrinogen, FVIII and vWF (Tables 7 and 8, Supplemental Appendix 1). Changing the exposure classes towards higher exposures in the high exposure group strengthened the findings. For CC16, a statistically significant increase in the high exposure group for inhalable dust was shown (Tables 9 and 10, Supplemental Appendix 1).
The mixed model analysis based on personal cobalt exposures showed significantly decreased CRP and TNF levels and increased levels for IL-10 and FVIII when sampling day 1 and 2 was compared. Smokers had significantly increased levels of PAI-1, SAA and CRP compared with non-smokers and ex-smokers. Participants with BMI >26.6 had significantly increased levels of IL-6, IL-8, SAA, FVIII, PAI-1, CRP and fibrinogen. Increased levels of TNF, vWF, FVIII for those aged above 44 was also found. In addition, TNF levels, vWF and FVIII were significantly higher among subjects with blood group O than those with other blood groups. The increase in inflammatory markers was much higher for smokers than for the other markers such as BMI, sex, age, blood group, and measurement time (not in table).
Discussion
Main findings
Our study's main finding was significant relationships between the highest exposure levels of almost all particle mass exposure metrics and FVIII. For vWF and CC16, the corresponding relation was limited to respirable and total cobalt. There were also non-significant positive exposure-response relationships between several exposure metrics and other markers of inflammation and coagulation. In particular, inhalable dust and/or inhalable cobalt exhibited non-significant exposure-response patterns for CRP, IL-6, IL-8, SAA, vWF, fibrinogen, and FVIII for all exposure metrics including nanoparticles. Most of the exposure-response patterns were related to mass-based exposure metrics. However, positive exposure-responses were also observed for some markers for the particle surface area and particle number concentrations. These exposure-response patterns are similar to those reported in earlier studies (Westberg et al. Citation2019a, Andersson et al. Citation2020).
The cobalt exposure was low, with a mean for the personal sampling of 0.003 mg/m3 (range <0.00016–0.019 mg/m3), implying that all cobalt TWAs were below the Swedish OEL, 0.02 mg/m3. An earlier study at one of the plants found the same mean but a wider range of cobalt exposure, up to 0.056 mg/m3 (Westberg et al. Citation2019b). The measured exposures in this study represent typical exposure levels for the Swedish hard metal industry after decades of preventive work within the two sites. A more detailed presentation of the historical measurement data and trends are presented in a previous paper on mortality in the Swedish hard metal industry (Westberg et al. Citation2017). These low levels are also reflected in the exposure tertiles, where the high exposure group ranges from 0.002 to 0.019 mg/m3. Our findings should be interpreted because of these very low concentration levels.
The fact that the markers of coagulation were affected more than markers of inflammation, and the weak findings for cumulative exposures, may indicate that the cardiovascular health effects of occupational dust and cobalt exposure is mediated to a greater extent through short-term effects (increased risk of thrombosis by current exposure) rather than long-term effects (low-grade systemic inflammation promoting the progression of atherosclerosis), and would thus decrease rapidly after cessation of exposure. This result could explain the relatively low-risk cardiovascular event estimates due to occupational cobalt and dust exposure (Sjögren et al. Citation2020).
To our knowledge, this is the first published data on cobalt particle exposure and inflammatory and coagulatory markers among hard metal workers. A strength of this study is the use of parallel blood sampling and the determination of multiple measures of exposure, including measures based on particle mass, surface area, and number. The end-of-shift blood sampling protocol was designed to minimise the influence of diurnal variation (Rudnicka et al. Citation2007). The participants’ blood groups were recorded since it has been suggested that exposure to air pollutants may be associated with IHD among individuals with blood type O (Suadicani et al. Citation2002).
The study lacks a completely non-exposed referent group because the use of such a group could introduce socioeconomic bias. In previous studies, the air and blood sampling started after the participants returned to work after a long vacation (Ohlson et al. Citation2010). This approach was not adopted here because of logistical difficulties. Our experience suggests that a single week’s absence from exposed work is insufficient to establish a true baseline level of inflammatory markers (Westberg et al. Citation2016, Andersson et al. Citation2019). However, we believe that even without establishing such a baseline, analyses of exposure-response relationships for inflammatory markers can reveal important effects of particle and cobalt exposure at levels below the OEL (Swedish Work Environment Authority [SWEA] Citation2018).
The overall cobalt exposures and those for specific job titles at the two hard metal plants are comparable to previously reported exposure measurements (Klasson et al. Citation2016, Westberg et al. Citation2017). In the current study, 36% of the participating workers used respirators during some of their working time. Two particulate exposure measures were computed for these workers: the non-adjusted measure ignoring the effect of the respirators and an adjusted measure assuming zero exposure while the respirator was worn. We consider the latter measure to be the most reliable. These considerations are described in detail in an earlier paper reporting on data from iron foundries (Westberg et al. Citation2019a).
Workers in the hard metal industry may also be exposed to chemical agents, particularly metal state nickel and or chromium. However, these were not present in the alloys used during our field campaigns, and exposure to these metals is not firmly associated with CVD (Sjögren et al. Citation2020). The present and historical exposure levels of nickel and chromium in specific hard metal alloys were low compared to existing OELs (Westberg et al. Citation2017).
We used log-linear modelling to study the exposure-response relationships between particle exposure and inflammatory and coagulatory markers, adjusted for possible confounders. For reasons of sensitivity, mixed model analyses were performed using all three adjusted and non-adjusted measures of exposure. The mixed model analysis revealed that the levels of the inflammatory and coagulatory markers IL-10 and FVIII in the second samples were significantly higher than in the first samples. The levels of TNF, FVIII and vWF were significantly higher among subjects with blood group 0 than those with other blood groups. Levels of SAA, CRP and PAI-1 among smokers differed significantly from non-smokers. These findings are in line with findings in our previous studies in the pulp and paper industry and iron foundries (Westberg et al. Citation2016, Andersson et al. Citation2019), as well as other studies generally reporting higher levels of CRP and other inflammatory markers in smokers (Gallus et al. Citation2018).
Notably, the associations with FVIII, as well the marginal tendencies of exposure-response associations for some other biomarkers, were stronger for measures of total and respirable dust levels, and PM2.5 and PM10, than for measurements of nanoparticles, particle numbers or total particle area for which we found weak or null associations. This result contrasts with the common theory of greater cardiovascular health effects of ultrafine particles than coarser particles, mostly based on experimental studies (Brook et al. Citation2010). In our previous studies of occupational exposure (Ohlson et al. Citation2010, Westberg et al. Citation2016, Andersson et al. Citation2019), we could not see any strong support for this hypothesis. Similar findings of stronger associations for mass-based measurements have also been reported in previous occupational exposure studies (Westberg et al. Citation2019a).
Inflammatory markers
CC16 is secreted into the epithelial lining fluid of the lungs by club cells with a fraction normally leaking into the blood, where increased levels can be used as a marker of lung injury (McAuley and Matthay Citation2009). Serum CC16 levels may also increase due to increased airway production during inflammation or decreased renal clearance (Broeckaert et al. Citation2000). The biological function of CC16 is not fully understood, but many studies suggest that it has anti-inflammatory and anti-oxidative effects (Almuntashiri et al. Citation2020). Serum CC16 has been shown to be increased after acute airway exposures and in lung disorders with increased airway permeability and decreased after chronic particle exposure such as tobacco smoking (Broeckaert et al. Citation2000, Lakind et al. Citation2007).
We found a statistically significant relationship between cumulative inhalable dust exposure and CC16 in the highest tertile of exposure compared to the lowest. A non-significant exposure-response was determined for cross shift exposure to inhalable dust. In a recently published paper on respiratory health and inflammatory markers, based on the same investigation and study group, a more detailed discussion on CC16 is presented (Andersson et al. Citation2020).
We have in previous studies in other industries (aluminium and iron foundries and the paper and pulp industry) with much higher dust exposure levels found no relationship or weak non-significant relationships to pro-inflammatory cytokines (i.e. IL-1b, IL-6, IL-8 and TNF), in line with the results obtained in the present study. An exception was a significant exposure-response relationship between particulate matter and IL-6 in workers returning from a long vacation exposed to average total dust levels of 0.93 mg/m3 (Ohlson et al. Citation2010). Findings were similar after exposure to organic dust (Palmberg Citation2002) and zinc oxide, suggesting that an adaptation or low-grade inflammatory process occurs after prolonged exposure, with comparatively low levels of cytokines (Fine et al. Citation2000).
SAA, an acute-phase protein comparable to CRP, has few studies in occupational environments. However, there have been some studies on its responses in ambient air (Ruckerl et al. Citation2011) and of wood smoke showing both increasing and null results (Barregård et al. Citation2006, Stockfelt et al. Citation2013). SAA may play a role in atherosclerosis because it has been causally related to plaque formation in the aorta in animal studies (Vogel and Cassee Citation2018).
In a study on the pulp and paper industry, significant increases in SAA levels (similar to those observed for CRP) was associated with most mass-based exposure measures, particularly in the high exposure group (Westberg et al. Citation2016). The effects of SAA in iron foundry workers with quartz exposure were much stronger compared to the current study (Westberg et al. Citation2019a).
CRP is a sensitive acute-phase reactant widely used as a clinical biomarker of inflammation. Elevated levels of CRP have been observed among welders (Kim et al. Citation2005, Ohlson et al. Citation2010), steel production workers (Bonzini et al. Citation2010), and pulp and paper production workers (Westberg et al. Citation2016). Thus, several occupational studies have identified increased CRP levels among workers exposed to dust concentrations of the same order of magnitude or higher as observed in this study.
Coagulatory markers
For total dust, a statistically significant positive exposure-response was determined for FVIII at concentration levels ranging from 0.075 to 0.11 mg/m3. Almost all cobalt and dust exposure measures were significantly associated with increased concentrations of coagulation FVIII when the highest tertiles were compared to the lowest. For inhalable dust and cobalt, the high exposure levels were above 0.044 mg/m3 and above 0.47 µg/m3, respectively. FVIII is a marker of inflammation and hemostasis and a risk factor for coronary heart disease (Folsom et al. Citation1997, Haverkate Citation2002, Lacroix-Desmazes et al. Citation2008). Previous studies of ambient air pollution (PM10) (Liao et al. Citation2005), wood smoke exposure (Barregård et al. Citation2006), and exposure in Swedish iron foundries observed increased plasma levels of FVIII (Westberg et al. Citation2019a).
Previous studies have reported increased plasma concentrations of fibrinogen among pulp and paper mill workers (Westberg et al. Citation2016), iron foundry workers (Westberg et al. Citation2019a) and volunteers exposed to dust in a swine farm building (Sjögren et al. Citation1999), but reductions in fibrinogen levels have been reported among welders (Kim et al. Citation2005). In the present study, no significant increases in fibrinogen were found.
Downregulation of coagulation markers
Previous studies of hard metal workers have observed higher urine concentrations of cobalt at the end of the workweek indicating cumulating exposure (Lison Citation2015). For cobalt in urine, a significant decrease was noted for fibrinogen at the highest tertile of urinary cobalt. The middle tertial of cobalt in blood was associated with a significant decrease of D-dimer, however.
As mentioned before CC16 may have anti-inflammatory effects (Almuntashiri et al. Citation2020) and IL-10 is the most important cytokine in suppressing pro-inflammatory responses in all kinds of autoimmune diseases and limiting excessive immune responses (Wei et al. Citation2019, Saraiva et al. Citation2020). IL-10 can down-regulate the synthesis of fibrinogen (Vasse et al. Citation1996), and IL-10 is a powerful inhibitor of CRP-induced tissue factor expression in vitro, which may play an important regulatory role in preventing thrombosis (Ernofsson et al. Citation1996). IL-10 also inhibits fibrinolysis by blunting the release of D-dimer after endotoxin administration (Pajkrt et al. Citation1997). In the current study, CC16 showed a significantly increased concentration at the highest tertile of total cobalt particulate exposure. CC16 and IL-10 also increased significantly in the middle tertiles of two exposure measures, respectively.
Taken together, these findings may indicate a downregulating pathway of coagulation factors mediated by IL-10 or CC16.
Conclusion
Low average exposure levels were measured for workers in the Swedish hard metal industry with an inhalable dust concentration of 0.11 mg/m3 and inhalable cobalt of 0.003 mg/m3. The study observed statistically significant positive exposure-response for FVIII and total dust. It increased levels of the inflammation and coagulation markers FVIII, vWF and CC16 in the highest exposure groups for several exposure measures. These relationships between particle exposure and coagulatory markers may indicate an increased short-term risk of cardiovascular disease.
Informed consent
Informed consent was obtained for all individual participants included in the study. The samples from the study are stored in a biobank (Dnr 13OLL718-5). The study was approved by the Regional Ethical Review Board, Uppsala, DNR. 2015/066, including the informed consent procedures.
Response_to_the_reviewers.docx
Download MS Word (24.4 KB)Appendix_1.docx
Download MS Word (50.9 KB)Acknowledgements
We would like to acknowledge the cooperation and assistance of the industry representatives and their Swedish sites. In particular, we would like to thank all the employees who provided assistance throughout the study.
We would also acknowledge Annette Ericsson, Ina Lindell, Anders Johansson, Jessica Westerlund, Louise Fornander and Lennart Andersson at the Department of Occupational and Environmental Medicine, Örebro, for valuable assistance during the air and blood sampling, and Daniel Eklund and Kristine Midtbö at Inflammatory Response and Infection Susceptibility Centre (iRiSC), Örebro University for valuable support with blood sampling, storage and analysis.
Disclosure statement
The authors declare that they have no conflict of interest, commercial or non-commercial.
Data availability statement
Data cannot be shared publicly due to legal restrictions imposed by Swedish Law regarding identifiable data. Data access requests can be directed to Etikprövningsmyndigheten i Uppsala (Swedish Ethical Review Authority in Uppsala): https://etikprovningsmyndigheten.se/ or [email protected].
Additional information
Funding
References
- Almuntashiri, S., et al., 2020. Club cell secreted protein CC16: potential applications in prognosis and therapy for pulmonary diseases. Journal of clinical medicine, 9 (12), 4039.
- Andersson, L., et al., 2019. Respiratory health and inflammatory markers - exposure to respirable dust and quartz and chemical binders in Swedish iron foundries. PLoS one, 14 (11), e0224668.
- Andersson, L., et al., 2020. Respiratory health and inflammatory Markers-Exposure to Cobalt in the Swedish hard metal industry. Journal of occupational and environmental medicine, 62 (10), 820–829.
- Arant, C.B., et al., 2009. Multimarker approach predicts adverse cardiovascular events in women evaluated for suspected ischemia: results from the National Heart, Lung, and Blood Institute-sponsored Women's Ischemia Syndrome Evaluation. Clinical cardiology, 32 (5), 244–250.
- Balmes, J.R., 1987. Respiratory effects of hard-metal dust exposure. Occupational medicine, 2 (2), 327–344.
- Barborik, M., and Dusek, J., 1972. Cardiomyopathy accompanying industrial cobalt exposure. British heart journal, 34 (1), 113–116.
- Barregård, L., et al., 2006. Experimental exposure to wood-smoke particles in healthy humans: effects on markers of inflammation, coagulation, and lipid peroxidation. Inhalation toxicology, 18 (11), 845–853.
- Bonzini, M., et al., 2010. Effects of inhalable particulate matter on blood coagulation. Journal of thrombosis and haemostasis, 8 (4), 662–668.
- Broeckaert, F., et al., 2000. Clara cell secretory protein (CC16): features as a peripheral lung biomarker. Annals of the New York academy of sciences, 923, 68–77.
- Brook, R.D., et al., 2010. American Heart Association Council on Epidemiology and Prevention, Council on the Kidney in Cardiovascular Disease, and Council on Nutrition, Physical Activity and Metabolism Particulate matter air pollution and cardiovascular disease: an update to the scientific statement from the American Heart Association. Circulation, 121, 2331–2378.
- Cugell, D.W., et al., 1990. The respiratory effects of cobalt. Archives of internal medicine, 150 (1), 177–183.
- Dagenais, M., Skeldon, A., and Saleh, M., 2012. The inflammasome: in memory of Dr. Jurg Tschopp. Cell death and differentiation, 19 (1), 5–12.
- Danesh, J., 2000. Low grade inflammation and coronary heart disease: prospective study and updated meta-analyses. BMJ, 321 (7255), 199–204.
- Danesh, J., et al., 2005. Fibrinogen Studies Collaboration. Plasma fibrinogen level and the risk of major cardiovascular diseases and nonvascular mortality: an individual participant meta-analysis. JAMA, 294 (14), 1799–1809.
- Danesh, J., et al., 2008. Long-term interleukin-6 levels and subsequent risk of coronary heart disease: two new prospective studies and a systematic review. PLoS medicine, 5 (4), e78.
- Dostert, C., et al., 2008. Innate immune activation through Nalp3 inflammasome sensing of asbestos and silica. Science, 320 (5876), 674–677.
- Ernofsson, M., Tenno, T., and Siegbahn, A., 1996. Inhibition of tissue factor surface expression in human peripheral blood monocytes exposed to cytokines. British journal of haematology, 95 (2), 249–257.
- European Standard, 2014. EN 12341:2014 Ambient air – standard gravimetric measurement method for the determination of the PM10 or PM2.5 mass concentration of suspended particulate matter. Brussels, Belgium: CEN - European Committee for Standardization.
- Fine, J.M., et al., 2000. Characterisation of clinical tolerance to inhaled zinc oxide in naive subjects and sheet metal workers. Journal of occupational and environmental medicine, 42, 1085–1091.
- Folsom, A.R., et al., 1997. Prospective study of hemostatic factors and incidence of coronary heart disease: the Atherosclerosis Risk in Communities (ARIC) Study. Circulation, 96 (4), 1102–1108.
- Gallus, S., et al., 2018. Effect of tobacco smoking cessation on c-reactive protein levels in a cohort of low-dose computed tomography screening participants. Scientific reports, 8 (1), 12908.
- Haverkate, F., 2002. Levels of haemostatic factors, arteriosclerosis and cardiovascular disease. Vascular pharmacology, 39 (3), 109–112.
- Health and Safety Executive (HSE), 2000. MDHDS: general methods for sampling and gravimetric analysis of inhalable and respirable dust. Report no 14/3, February. Suffolk, UK: Health and Safety Executive.
- Hogstedt, C., and Alexandersson, R., 1990. Mortality among hard metal workers. Work and Health 1990:21. (In Swedish). Solna, Sweden: National Institute of Occupational Health.
- Julander, A., et al., 2009. Cobalt-containing alloys and their ability to release cobalt and cause dermatitis. Contact dermatitis, 60 (3), 165–170.
- Kennedy, A., Dornan, J.D., and King, R., 1981. Fatal myocardial disease associated with industrial exposure to cobalt. Lancet, 1 (8217), 412–414.
- Kim, J.Y., et al., 2005. Exposure to welding fumes is associated with acute systemic inflammatory responses. Occupational and environmental medicine, 62 (3), 157–163.
- Klasson, M., et al., 2016. Occupational exposure to cobalt and tungsten in the Swedish hard metal industry – air concentrations of particle mass, number and surface area. Annals of occupational hygiene, 60 (6), 684–699.
- Kusaka, Y., et al., 1989. Lymphocyte transformation with cobalt in hard metal asthma. Industrial health, 27 (4), 155–163.
- Lacroix-Desmazes, S., et al., 2008. Dynamics of factor VIII interactions determine its immunologic fate in hemophilia A. Blood, 112 (2), 240–249.
- Lakind, J.S., et al., 2007. A critical review of the use of Clara cell secretory protein (CC16) as a biomarker of acute or chronic pulmonary effects. Biomarkers, 12 (5), 445–467.
- Lasfargues, G., et al., 1994. Lung cancer mortality in a French cohort of hard-metal workers. American journal of industrial medicine, 26 (5), 585–595.
- Liao, D., et al., 2005. Association of criteria pollutants with plasma hemostatic/inflammatory markers: a population-based study. Journal of exposure science & environmental epidemiology, 15 (4), 319–328.
- Lison, D., 2015. 34. Cobalt. In: G.F. Nordberg, B.A. Fowler, and M. Nordberg, eds. Handbook on the toxicology of metals. 4th ed. London, United Kingdom: Elsevier Academic Press Inc., 743–763.
- Marsh, G.M., et al., 2017. Mortality among hardmetal production workers: pooled analysis of cohort data from an international investigation. Journal of occupational and environmental medicine, 59 (12), e342–e364.
- McAuley, D., and Matthay, M., 2009. Clara cell protein CC16. A new lung epithelial biomarker for acute lung injury. Chest, 135 (6), 1408–1410.
- Moulin, J.J., et al., 1998. Lung cancer risk in hard-metal workers. American journal of epidemiology, 148 (3), 241–248.
- Nakamura, Y., et al., 2014. Hard metal lung disease diagnosed on a transbronchial lung biopsy following recurrent contact dermatitis. Internal medicine, 53 (2), 139–143.
- National Institute of Occupational Health (NIOSH), 1994. Manual of analytical methods. 4th ed. Particulates not otherwise regulated: method 0500. Cincinnati: National Institute of Occupational Safety and Health.
- National Institute of Occupational Health (NIOSH), 2014. Manual of Analytical methods method 7300. Elements by ICPMS In: NIOSH Manual of analytical methods. 5th ed. DHHS Pub. No 94-113. Cincinnati: National Institute of Occupational Health. Modified.
- Ohlson, C.G., et al., 2010. Inflammatory markers and exposure to occupational air pollutants. Inhalation toxicology, 22 (13), 1083–1090.
- Pajkrt, D., et al., 1997. Interleukin-10 inhibits activation of coagulation and fibrinolysis during human endotoxemia. Blood, 89 (8), 2701–2705.
- Palmberg, L., et al., 2002. Airway responses of healthy farmers and nonfarmers to exposure in a swine confinement building. Scandinavian journal of work, environment & health, 28 (4), 256–263.
- Peeters, P.M., et al., 2013. Silica induces NLRP3 inflammasome activation in human lung epithelial cells. Particle and fibre toxicology, 10, 3.
- Rehfisch, P., et al., 2012. Lung function and respiratory symptoms in hard metal workers exposed to cobalt. Journal of occupational and environmental medicine, 54 (4), 409–413.
- Ruckerl, R., et al., 2011. Health effects of particulate air pollution: a review of epidemiological evidence. Inhalation toxicology, 23 (10), 555–592.
- Rudnicka, A.R., et al., 2007. Diurnal, seasonal, and blood-processing patterns in levels of circulating fibrinogen, fibrin D-dimer, C-reactive protein, tissue plasminogen activator and von Willebrand factor in a 45 year-old population. Circulation, 115 (8), 996–1003.
- Sadik, M., 2015. An introduction to cutting tools materials and application. 2nd ed. Sweden: Sandvik.
- Saraiva, M., Vieira, P., and O’Garra, A., 2020. Biology and therapeutic potential of interleukin-10. Journal of experimental medicine, 217 (1), e20190418.
- Seaton, A., et al., 1995. Particulate air pollution and acute health effects. Lancet, 345 (8943), 176–178.
- Shirakawa, T., et al., 1989. Occupational asthma from cobalt sensitivity in workers exposed to hard metal dust. Chest, 95 (1), 29–37.
- Sjögren, B., 1997. Occupational exposure to dust: inflammation and ischaemic heart disease. Occupational and environmental medicine, 54 (7), 466–469.
- Sjögren, B., Bigert, C., and Gustavsson, P., 2020. 153 Occupational chemical exposures and cardiovascular disease. Work and Health Scientific Serial No 2020;54(2). The Nordic Expert Group for Criteria Documentation of Health Risks of Chemicals. Göteborg, Sweden: Work and Health. (www.nordicexpertgroup.org).
- Sjögren, B., et al., 1999. Increase in interleukin-6 and fibrinogen in peripheral blood after swine dust inhalation. Scandinavian journal of work, environment & health, 25 (1), 39–34.
- Stockfelt, L., et al., 2013. Short-term chamber exposure to low doses of two kinds of wood smoke does not induce systemic inflammation, coagulation or oxidative stress in healthy humans. Inhalation toxicology, 25 (8), 417–425.
- Suadicani, P., Hein, H.O., and Gyntelberg, F., 2002. Airborne occupational exposure, ABO phenotype and risk of ischaemic heart disease in the Copenhagen Male Study. Journal of cardiovascular risk, 9 (4), 191–198.
- Swedish Work Environment Authority (SWEA), 2018. Hygienic limit values, statute book 2018:1. Solna, Sweden: Swedish Work Environment Authority. (In Swedish).
- Vasse, M., et al., 1996. Down-regulation of fibrinogen biosynthesis by IL-4, IL-10 and IL-13. British journal of haematology, 93 (4), 955–961.
- Vincent, J., 2005. Health-related aerosol measurement: a review of existing sampling criteria and proposals for new ones. Journal of environmental monitoring, 7 (11), 1037–1053.
- Vogel, U., and Cassee, F.R., 2018. Editorial: dose-dependent ZnO particle-induced acute phase response in humans warrants re-evaluation of occupational exposure limits for metal oxides. Particle and fibre toxicology, 15 (1), 7.
- Wei, H., et al., 2019. Interleukin-10 family cytokines immunobiology and structure. Advances in experimental medicine and biology, 1172, 79–96.
- Westberg, H., et al., 2016. Inflammatory markers and exposure to airborne particles among workers in a Swedish pulp and paper mill. International archives of occupational and environmental health, 89 (5), 813–822.
- Westberg, H., et al., 2017. Mortality among hardmetal production workers: Swedish measurement data and exposure assessment. Journal of occupational & environmental medicine, 59 (12), e327–e341.
- Westberg, H., et al., 2019a. Inflammatory and coagulatory markers and exposure to different size fractions of particle mass, number and surface area air concentrations in Swedish iron foundries, in particular respirable quartz. International archives of occupational and environmental health, 92 (8), 1087–1098.
- Westberg, H., et al., 2019b. Airway and dermal effects-biomarkers for inflammation and coagulation for cobalt exposure in a hard metal plant. (In Swedish). Report AMM 2019;1, no 19RS8319-1, 38 pp.
- Wild, P., et al., 2000. Lung cancer mortality in a site producing hard metals. Occupational and environmental medicine, 57 (8), 568–573.