ABSTRACT
Previous studies have reported that transcranial direct current stimulation (tDCS) of the frontal polar area (FPA) ameliorated motor disability in patients with Parkinson’s disease (PD). Here we report changes in neuromelanin (NM) imaging of dopaminergic neurons before and after rehabilitation combined with anodal tDCS over the FPA for 2 weeks in a PD patient. After the intervention, the patient showed clinically meaningful improvements while the NM-sensitive area in the SN increased by 18.8%. This case study is the first report of NM imaging of the SN in a PD patient who received tDCS.
Abbreviations FPA: front polar area; PD: Parkinson’s disease; NM: neuromelanin; DCI: DOPA decarboxylase inhibitor; STEF: simple test for evaluating hand function; TUG: timed up and go test; TMT: trail-making test; SN: substantia nigra; NM-MRI: neuromelanin magnetic resonance imaging; MCID: the minimal clinically important difference; SNpc: substantia nigra pars compacta; VTA: ventral tegmental area; LC: locus coeruleus; PFC: prefrontal cortex; M1: primary motor cortex; MDS: Movement Disorder Society; MIBG: 123I-metaiodobenzylguanidine; SBR: specific binding ratio; SPECT: single-photon emission computed tomography; DAT: dopamine transporter; NIBS: noninvasive brain stimulation; tDCS: transcranial direct current stimulation; MAOB: monoamine oxidase B; DCI: decarboxylase inhibitor; repetitive transcranial magnetic stimulation: rTMS; diffusion tensor imaging: DTI; arterial spin labeling: ASL
Introduction
Loss of dopaminergic neurons in the brainstem, including the substantia nigra pars compacta (SNpc), ventral tegmental area (VTA), and locus coeruleus (LC), is characteristic of Parkinson’s disease (PD) (Alberico et al., Citation2015; Giguère et al., Citation2018; Peterson & Li, Citation2018), presenting with progressive motor and non-motor symptoms. The SNpc is involved in voluntary movements through the nigrostriatal pathway, while the VTA is involved in cognition and emotion through the mesolimbic pathway (Van, Domburg & ten, Donkelaar, Citation1991). Dopaminergic neurons in these brainstem regions receive direct and indirect excitatory glutamatergic afferents from the prefrontal cortex (PFC) (Carr & Sesack, Citation2000; Geisler et al., Citation2007; Han et al., Citation2017; Kalivas, Citation1993), and in turn send dopaminergic projections to the primary motor cortex (M1) and basal ganglia (Hosp et al., Citation2011). Thus, dopaminergic depletion induces disturbances in a wide range of the motor circuits (McGregor et al., Citation2019; Underwood & Parr-Brownlie, Citation2021), and noninvasive imaging of dopaminergic activity is useful for PD diagnosis and assessment of PD symptoms (He et al., Citation2018).
Although PD is diagnosed based on clinical symptoms that fulfill the diagnostic criteria published by the UK Parkinson’s Disease Brain Bank (Hughes et al., Citation1992), recent advances in neuroimaging contribute to PD diagnosis, especially in its early stages or in atypical cases. For example, the Movement Disorder Society (MDS) PD criteria include decreased accumulation of 123I-metaiodobenzylguanidine (MIBG) myocardial scintigraphy as a supportive criterion and normal specific binding ratio (SBR) in 123I-N-v-fluoropropyl-2b- carbomethoxy3b-(4-iodophenyl) nortropane single-photon emission computed tomography (SPECT) as an absolute exclusion criterion (Postuma et al., Citation2015). On the other hand, neuromelanin magnetic resonance imaging (NM-MRI) detects iron-NM (catecholamine metabolite) complexes in the SN and LC, and the signal intensity of NM-MRI was reported to reflect the clinical and pathological characteristics of PD (Sasaki et al., Citation2006; Taniguchi et al., Citation2018). This imaging can visualize dopaminergic and noradrenergic neurons in the SNpc and LC in normal subjects and PD patients (Castellanos et al., Citation2015; Matsuura et al., Citation2013; Ohtsuka et al., Citation2014, Citation2013; Sasaki et al., Citation2006). Although sizes of NM-sensitive areas in the SNpc on NM-MRI were correlated with specific binding ratio (SBR) on dopamine transporter-single photon computed emission tomography (DAT-SPECT), NM-MRI was more sensitive to motor fluctuations than DAT-SPECT in advanced PD (Okuzumi et al., Citation2019).
In PD treatment, although pharmacotherapy is effective in the earlier stage of PD, motor fluctuation and dyskinesia, which may be more wasting than non-motor disturbance, gradually develop in most patients (Hauser, Citation2009). Recently, neuro-rehabilitation combined with noninvasive brain stimulation (NIBS) has been considered to safely affect cortical excitability (Nitsche, Fricke et al., Citation2003; Nitsche, Liebetanz et al., Citation2003; Russo et al., Citation2017). For example, transcranial direct current stimulation (tDCS) applies low-intensity electric current from the scalp relatively easily for clinical application, and can safely modulate cortical excitability: anodal and cathodal tDCS raises and diminishes cortical excitability, respectively (Liebetanz, Citation2002; Nitsche, Fricke et al., Citation2003; Nitsche, Liebetanz et al., Citation2003). Recent systematic reviews reported the potential effectiveness of tDCS on motor and non-motor functions in PD, although more evidence is required (Beretta et al., Citation2020; Lefaucheur et al., Citation2017; Simpson & Mak, Citation2020; Suarez-García et al., Citation2020). Rehabilitation with tDCS ameliorated neurological symptoms in various neurological disorders including PD in long-term (Ferrucci et al., Citation2016; Flöel, Citation2014; Khedr et al., Citation2013; Meinzer et al., Citation2016). Especially, anodal tDCS of the primary motor cortex (M1) improved motor symptoms of PD (Benninger et al., Citation2010; Fregni et al., Citation2006; Kaski et al., Citation2014), while anodal stimulation over the dorsolateral PFC improved non-motor functions such as cognitive and executive functions (Boggio et al., Citation2006; Doruk et al., Citation2014; Pereira et al., Citation2013). We have reported that the frontal polar area (FPA) was activated during repetitive hand training with an improvement of motor learning in healthy adults (Ishikuro et al., Citation2014) and tDCS over the FPA significantly ameliorated not only motor symptoms but also non-motor symptoms in PD patients (Ishikuro et al., Citation2018). Although we previously hypothesized that tDCS over the FPA might activate dopaminergic neurons in the midbrain and increase dopamine release since midbrain dopaminergic neurons are functionally coupled with the prefrontal cortex (Ishikuro et al., Citation2018), no evidence has been provided.
Physical rehabilitation may also have beneficial effects on dopaminergic neurons. Previous studies with a PD mouse model using 1-methyl-4-phenyl-1,2,3,6-tetrahydropyridine (MPTP) demonstrated that treadmill exercise could modify dopamine receptor expression in the PFC (Kintz et al., Citation2017; Petzinger et al., Citation2007), improve motor performance, and restore striatal dopaminergic receptors (Vučković et al., Citation2010). Other mouse model studies demonstrated that treadmill exercise restored dendritic spine loss in medium spiny neurons in the striatum (Toy et al., Citation2014; Vučković et al., Citation2010). These reports suggest that repetitive exercise provided by physical rehabilitation can improve motor performance and restore dopaminergic neurons with PD. Many researchers have reported that traditional physical rehabilitation (muscle strength exercise, treadmill exercise, gait exercise, etc.) could improve motor function, including that assessed in the UPDRS-III (Flach et al., Citation2017; Ni et al., Citation2018). Interestingly, high strength exercise could significantly improve PD symptoms and decreased L-dopa more than low-strength exercise after 2 years (Corcos et al., Citation2013). These results suggest that physical rehabilitation itself may activate surviving dopaminergic neurons and induce synaptic or receptor plasticity in the SN.
The above findings suggest that physical rehabilitation combined with tDCS over the FPA would effectively activate dopaminergic neurons. However, the effects of this neuro-rehabilitation on dopaminergic neurons in the SN remain unknown. In the present clinical study, to assess dopaminergic neuronal activity associated with therapeutic efficacy, we investigated changes in NM-sensitive areas in the SN along with PD symptoms after physical rehabilitation combined with anodal tDCS over the FPA in a PD patient.
Materials and methods
Patient report
A 71-year-old woman with resting tremor in her left upper extremity was referred to the Department of Neurology at Toyama University Hospital. The patient was diagnosed with PD according to the clinical diagnostic criteria of the UK Parkinson’s Disease Brain Bank and was prescribed a monoamine oxidase B (MAOB) inhibitor and ropinirole.
Although the resting tremor initially improved, her PD symptoms, including bradykinesia, tremor, rigidity, loss of postural reflex, Pisa syndrome, and freezing gait, gradually worsened, and she fell frequently, despite the increase in pharmacotherapy with levodopa/DOPA decarboxylase inhibitor (DCI), zonisamide, cabergoline, and duloxetine hydrochloride. Her cognitive function remained unimpaired with a Mini Mental Examination score of 30. Since her PD motor symptoms had gradually progressed, we proposed the following neurorehabilitation intervention with tDCS for the treatment of PD symptoms following hospital admission.
This study was conducted under the principles of the Basel Declaration and recommendations of the Medical Ethics Regulations, and ethical guidelines of the Medical Research Ethics Committee at the University of Toyama. The protocol of this clinical study was approved by the Medical Research Ethics Committee of the University of Toyama (Permit No.: Toyama University Hospital 29–106) and publicly registered in UMIN Clinical Trials Registry (UMIN-CTR) (trial registration ID: UMIN000033253). The subject provided written informed consent and approval for the publication of the results before participating in this study. The same pharmacotherapy as before the admission was prescribed during this study.
Intervention procedures with tDCS
Neurorehabilitation in this study included standard physical rehabilitation combined with tDCS. The standard physical rehabilitation consisted of muscle stretching, muscle strengthening exercises, balance exercises, and gait exercises. On each training day, muscle stretching and muscle strengthening exercises of the upper and lower extremities were conducted during tDCS while the patient sat on a chair for 15 min. Balance and gait exercises were performed using parallel bars in an exercise room after tDCS. This standard physical rehabilitation, combined with tDCS (15 min), was administered for approximately 30 min/day, 5 times a week for 2 weeks ().
Figure 1. Experimental design.
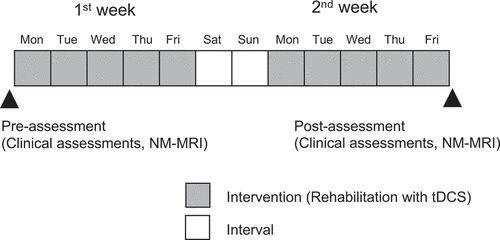
tDCS was performed using a constant current stimulator (DC-stimulator Plus, Neuroconn, Ilmenau, Germany) that delivered a constant current (1.0 mA) for 15 min through a pair of sponge electrodes soaked in physiological saline (5 × 7 cm). An anodal electrode was placed on the forehead over the FPA (FPZ) and a cathodal electrode was placed on the occipital area (OZ) in the international 10–20 EEG system (Herwig et al., Citation2003). The current density was 0.0285 mA/cm2, which was smaller than the safety limits indicated in previous studies (Nitsche, Liebetanz et al., Citation2003; Poreisz et al., Citation2007) and evidence-based guidelines on the therapeutic use of tDCS (Lefaucheur et al., Citation2017).
Clinical assessment
The patient received the same pharmacological medication as that before the intervention during the rehabilitation with tDCS. The same assessor (K.I.) carried out the pre- and post-assessments in the same condition of the patient (on state) at the same time of the day [PM 13:00–14:00 (from 60 to 120 min after the last intake of the medicines)].
The Hoehn & Yahr test and unified PD rating scale part III (UPDRS-III) (Martínez-Martín et al., Citation2000) were used to assess parkinsonism. A simple test for evaluating hand function (STEF) (SAKAI Medical Co. Ltd., Hongo, Tokyo, Japan) was used to evaluate hand functions to pinch and grasp with fingers and to move objects (Kaneko & Muraki, Citation1990). The timed up and go (TUG) test assesses walking ability in a general assessment of gait function and balance. This test measures the walking time (sec) and steps (number) for the patient to get up from a chair, walk around a landmark 3 m away, and then return to the chair and sit back. To assess non-motor functions, the Trail Making Test (TMT) was used to evaluate visual attention and task-switching (Bowie & Harvey, Citation2006). The above clinical assessments were performed before the intervention on the first day and after the intervention on the last day of neurorehabilitation.
Imaging assessment (NM-MRI)
A 3-T MRI scanner (VERIO, Siemens, Berlin, Germany) with a 12-channel head coil was used to obtain NM-MRI. T1-weight fast spin-echo images were acquired with the following parameters: repetition time = 550 ms; echo time = 11 ms; echo train length of 3, flip angle = 150°, field of view = 220 × 220 mm; matrix size = 512 × 336 (pixel size = 0.4 × 0.4 mm); number of slices = 12; slice thickness = 2.5 mm; slice gap = 0; and number of excitations = 4. Image slices were aligned with the anterior commissure-posterior commissure line, and the bottom of the center slice was placed on the border between the midbrain and pons, as viewed in the sagittal plane, to scan the entire midbrain. The acquisition time was 7 min 45 s. NM-MRI scans of the patient were performed before the clinical assessment on the first day of the intervention and after the clinical assessment on the last day of the intervention.
NM-MRI data were converted to 8-bit data to analyze NM-sensitive high-contrast areas in the SNpc using ImageJ (http://rsbweb.nih.gov/ij/index.html). According to the previous studies (Ohtsuka et al., Citation2014, Citation2013; Okuzumi et al., Citation2019; Reimão et al., Citation2015), one slice containing the largest NM-sensitive area among the four slices in which the NM-sensitive area was visible in the SN was selected for each scan (Fig. 2Aa, Ba). Then, the boundary lines between the midbrain tegmentum and SN were manually drawn on both sides. Boundary drawing was performed by one blinded physical therapist (S.F.) who had previously observed the SN in NM-MRI photos of the brainstem, but had never met the patient (Fig. 2Ab, Bb). Second, according to the method of Isaias et al. (Citation2016), the mean signal intensity (MCC) and its standard deviation (SDCC) in the circular area (diam., 4 mm) within the cerebral crus (CC) were calculated on each side and taken as the background signal intensity (Ab, Bb). Third, voxels with signal intensity greater than the threshold (MCC + 3 SDCC) were identified in the areas outside the midbrain tegmentum on each side according to previous studies (Isaias et al., Citation2016; Langley et al., Citation2017; Schwarz et al., Citation2017). Finally, voxels with signal intensity greater than the threshold were calculated within the areas outside the midbrain tegmentum as NM-sensitive high-contrast areas (i.e., SNpc).
Figure 2. Analysis of neuromelanin magnetic resonance imaging (NM-MRI).
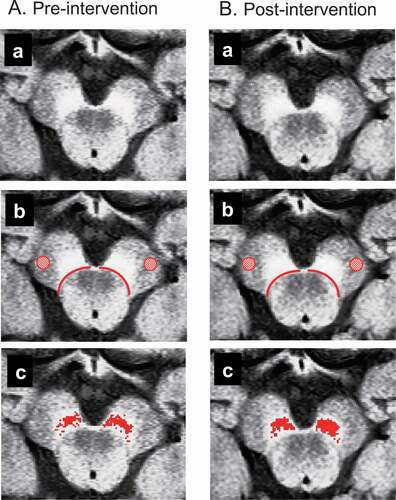
Results
Clinical assessment
The posture balance impairment persisted after the intervention, and Hoehn & Yahr grades did not change (III/III: pre-intervention/post-intervention, same for the following assessments). On the other hand, UPDRS-III scores were greatly improved (27/5 points), in which improvement was observed mainly in the tremor and rigidity assessments. STEF scores were 81/84 points for the right upper extremity and 81/81 points for the left upper extremity. The time to complete the TUG was 13.4/12.5 sec, while the number of steps (pre/post) was 25/19 steps. The time required to complete the TMT was 60.4/54.4 sec for TMT-A and 156.0/153.0 sec for TMT-B. The difference in the time to complete TMT-A and TMT-B was 95.6/98.6 sec ().
Table 1. Assessment of non-motor and motor functions
Imaging assessment
Figure 2Aa and Ba show NM-MRI axial image slices including the SN before and after the intervention, respectively. In Fig. 2Ab and Bb, the background signal intensity was measured within the red circles in the cerebral crus while the areas outside the midbrain tegmentum surrounded by the red boundary lines were analyzed to detect NM-sensitive areas. In Fig. 2Ac and Bc, red areas indicate NM-sensitive areas above the threshold in the outer region of the midbrain tegmentum. The NM-sensitive area in the SNpc changed from 43.2 mm2 (right side, 17.5 mm2; left side, 25.7 mm2) (Fig. 2Ac) to 53.2 mm2 (right side, 23.5 mm2; left side, 29.7 mm2) (Fig. 2Bc), an increase of 18.8%.
Discussion
Previous studies have reported that maneuvers to increase FPA activity, such as tDCS and neurofeedback training, improved motor learning in healthy subjects (Ishikuro et al., Citation2014; Ota et al., Citation2020), and tDCS over the FPA ameliorated deficits in non-motor and motor functions in patients with PD (Ishikuro et al., Citation2018). This case study is the first report demonstrating changes (18.8% increase) in NM-sensitive areas in the SN after neuro-rehabilitation with anodal tDCS of the FPA in a PD patient correlating with improvement in motor function. A previous study using a comparative NM-MRI method (T1-weighted turbo spin echo without a magnetization transfer pulse) reported that the mean differences of SNpc volumes in healthy subjects between the test and retest were less than 7% across three raters (Van, der, Pluijm et al., Citation2021). These findings suggest that the SNpc volume increased by tDCS beyond normal fluctuations in multiple measurements in the present study.
Previous studies reported that the minimal clinically important difference (MCID) was 2.3–2.7 for UPDRS-III (Shulman et al., Citation2010) and 13.0% for TUG (Gallagher et al., Citation2019). Improvement greater than the MCID was found for both the UPDRS-III and TUG (steps). Consistent with the present study, in our previous study using the same protocol (physical rehabilitation combined with tDCS over the FPA), effect sizes of physical rehabilitation combined with anodal tDCS in UPDRS III were 0.29 (ω2) and 0.35 (η2) across the three groups (physical rehabilitation combined with anodal tDCS, physical rehabilitation combined with cathodal tDCS, and physical rehabilitation combined with sham tDCS) (Ishikuro et al., Citation2018). The improvement in UPDRS-III was mainly in tremor and rigidity. These improvements in motor function might be attributed to the increase in NM-sensitive areas. On the other hand, the Hoehn & Yahr grade remained at III, which indicated no significant change in postural balance control. Regarding upper extremity motor function and cognitive function, the mean STEF scores and TMT (sec) of healthy people in the same age range are 90 points in STEF (Kaneko & Muraki, Citation1990), 40.13 ± 14.48 sec in TMT-A, and 86.27 ± 24.07 sec in TMT-B (Tombaugh, Citation2004). The STEF (points) and TMT (sec) of the patient were considerably worse than those of healthy controls, both before and after the intervention, and the change in these scores was slight or negligible. Some motor symptoms, such as tremor and rigidity, might be more sensitive to physical therapy combined with tDCS of the FPA over the short-term (2 weeks in this study) than other motor symptoms, such as bradykinesia and impairment of postural control, and non-motor symptoms.
It has been reported that the PFC sends direct/indirect excitatory projections to dopaminergic neurons (see Introduction) and that PFC stimulation increases dopaminergic neuronal activity (Murase et al., Citation1993; Tong et al., Citation1996). Furthermore, the survival of dopaminergic neurons or expression of a dopaminergic phenotype is activity-dependent (Aumann & Horne, Citation2012; Michel et al., Citation2007): decreased electrical activity in dopaminergic neurons leads to cell death or decreases in the amount of tyrosine hydroxylase (rate-limiting enzyme of dopamine synthesis). In addition, anodal tDCS of the frontal cortex for 3 weeks increased dopamine content in the whole brain in a PD mouse model (Lu et al., Citation2015). The current results, as well as evidence from previous studies, suggest that anodal tDCS of the FPA facilitates the activity of dopaminergic neurons, which may lead to an increase in the NM-sensitive area in the SNpc, with increased dopaminergic neurotransmission. Furthermore, this plastic increase in dopaminergic neurotransmission may facilitate the PFC-SNpc-basal ganglia-M1 circuit, leading to improvement of motor functions.
Accumulating evidence suggests that NM in the SNpc may be an effective biomarker in PD (He et al., Citation2018; Hughes et al., Citation1992; Ohtsuka et al., Citation2014, Citation2013; Postuma et al., Citation2015; Sasaki et al., Citation2006; Taniguchi et al., Citation2018), and NM contrast using NM-MRI is reproducible and reliable for detecting dopaminergic neurons (Wengler et al., Citation2020). As far as we know, no previous studies have compared NM-sensitive areas in the SNpc before and after neuro-rehabilitation. This case study is the first report showing an increase in NM-sensitive areas in the SNpc after neuro-rehabilitation with anodal tDCS on the FPA of a PD patient in line with the improvement in motor symptoms.
Finally, there are several limitations in the present study. First, although the results of this single case study seem promising, they are not yet conclusive: anodal tDCS could induce placebo-like effects. Unfortunately, sham stimulation to assess placebo-like effects could not be tested in this study because there was only one patient. However, our previous study using the same protocol reported that effects of anodal tDCS over the FPA were significantly greater than those of sham stimulation in patients with PD (Ishikuro et al., Citation2018). Furthermore, a previous meta-analysis study reported that effects of placebo treatment as a control for repetitive transcranial magnetic stimulation (rTMS) were not statistically significant in patients with PD (Fregni, Citation2005). To clarify effects of tDCS over the FPA in PD patients, further sham-controlled studies with longer periods of intervention will be required. Second, we assessed only NM-MRI in the present study since we focused on dopaminergic neurons to assess their functional recovery. However, recent studies reported that multimodal MRI assessments including not only NM-MRI, but also structural imaging, nigrosome imaging, iron-sensitive imaging, diffusion tensor imaging (DTI), arterial spin labeling (ASL), and resting-state fMRI to assess functional connectivity would be effective to diagnose PD and understand pathophysiological mechanisms of PD symptoms (Bae et al., Citation2021; Heim et al., Citation2017; Talai et al., Citation2021). Although the present study does not provide information on full pathophysiological changes in the patient, the results suggest that NM-MRI may be useful to assess responses of dopaminergic neurons to treatments.
In conclusion, this case study shows that the NM-sensitive area increased with the improvement of motor functions after rehabilitation with anodal tDCS of the FPA in a patient with PD. These results suggest that neurorehabilitation with anodal tDCS of the FPA may ameliorate motor disturbance in PD by increasing dopaminergic neuronal activity in the SNpc. To prove or disprove this conclusion, further studies with sham controls and multimodal MRI assessments will be required.
Authors’ contributions
KI, NH, YN, and HN designed the experiment. KI performed the physical rehabilitation combined with tDCS. TS, KM, KN, and SK performed the MRI scans. RI, KF, TN, ND, MY, HK, TN, TH, RA, NM, HH, and RT assessed the clinical symptoms. KI and NH and HN analyzed data and wrote the paper. KI, NH, YN, and HN revised the paper. All the authors discussed the results and approved the final manuscript.
Acknowledgments
This work was supported partly by JSPS KAKENHI Grant Number JP19K11365.
Disclosure statement
No potential conflict of interest was reported by the author(s).
Additional information
Funding
References
- Alberico, S. L., Casell, M. D., & Narayanan, N. S. (2015). The vulnerable ventral tegmental area in Parkinson’s disease. Basal Ganglia, 5(2-3), 51–55. https://doi.org/https://doi.org/10.1016/j.baga.2015.06.001
- Aumann, T., & Horne, M. (2012). Activity-dependent regulation of the dopamine phenotype in substantia nigra neurons. Journal of Neurochemistry, 121(4), 497–515. https://doi.org/https://doi.org/10.1111/j.1471-4159.2012.07703.x
- Bae, Y. J., Kim, J.-M., Sohn, C.-H., Choi, J.-H., Choi, B. S., Song, Y. S., Nam, Y., Cho, S. J., Jeon, B., & Kim, J. H. (2021). Imaging the substantia Nigra in Parkinson disease and other Parkinsonian syndromes. Radiology, 300(2), 260–278. https://doi.org/https://doi.org/10.1148/radiol.2021203341
- Benninger, D. H., Lomarev, M., Lopez, G., Wassermann, E. M., Li, X., Considine, E., & Hallett, M. (2010). Transcranial direct current stimulation for the treatment of Parkinson’s disease. Journal of Neurology, Neurosurgery, and Psychiatry, 81(10), 1105–1111. https://doi.org/https://doi.org/10.1136/jnnp.2009.202556
- Beretta, V. S., Conceição, N. R., Nóbrega-Sousa, P., Orcioli-Silva, D., Dantas, L. K. B. F., Gobbi, L. T. B., & Vitório, R. (2020). Transcranial direct current stimulation combined with physical or cognitive training in people with Parkinson’s disease: A systematic review. Journal of Neuroengineering and Rehabilitation, 17(1), 74. https://doi.org/https://doi.org/10.1186/s12984-020-00701-6
- Boggio, P. S., Ferrucci, R., Rigonatti, S. P., Covre, P., Nitsche, M., Pascual- Leone, A., & Fregni, F. (2006). Effects of transcranial direct current stimulation on working memory in patients with Parkinson’s disease. Journal of Neurological Sciences, 249(1), 31–38. https://doi.org/https://doi.org/10.1016/j.jns.2006.05.062
- Bowie, C. R., & Harvey, P. D. (2006). Administration and interpretation of the trail making test. Nature Protocols, 1(5), 2277–2281. https://doi.org/https://doi.org/10.1038/nprot.2006.390
- Carr, D. B., & Sesack, S. R. (2000). Projections from the rat prefrontal cortex to the ventral tegmental area: Target specificity in the synaptic associations with mesoaccumbens and mesocortical neurons. The Journal of Neuroscience, 20(10), 3864–3873. https://doi.org/https://doi.org/10.1523/JNEUROSCI.20-10-03864.2000
- Castellanos, G., Fernández-Seara, M. A., Lorenzo-Betancor, O., Ortega-Cubero, S., Puigvert, M., Uranga, J., Vidorreta, M., Irigoyen, F., Lorenzo, E., Munoz-Barrutia, A., Ortiz-de-Solorzano, C., Pastor, P., & Pastor, M. (2015). Automated neuromelanin imaging as a diagnostic biomarker for Parkinson’s disease. Movement Disorders, 30(7), 945–952. https://doi.org/https://doi.org/10.1002/mds.26201
- Corcos, D. M., Robichaud, J. A., David, F. J., Leurgans, S. E., Vaillancourt, D. E., Poon, C., Rafferty, M. R., Kohrt, W. M., & Comella, C. (2013). A two-year randomized controlled trial of progressive resistance exercise for Parkinson’s disease. Movement Disorders, 28(9), 1230–1240. https://doi.org/https://doi.org/10.1002/mds.25380
- Doruk, D., Gray, Z., Bravo, G. L., Pascual-Leone, A., & Fregni, F. (2014). Effects of tDCS on executive function in Parkinson’s disease. Neuroscience Letters, 582, 27–31. https://doi.org/https://doi.org/10.1016/j.neulet.2014.08.043
- Ferrucci, R., Mameli, F., Ruggio, F., & Priori, A. (2016). Transcranial direct current stimulation as treatment for Parkinson’s disease and other movement disorders. Basal Ganglia, 6(1), 53–61. https://doi.org/http://doi.org/10.1016/j.baga.2015.12.002
- Flach, A., Jaegers, L., Krieger, M., Bixler, E., Kelly, P., Weiss, E. P., & Ahmad, S. O. (2017). Endurance exercise improves function in individuals with Parkinson’s disease: A meta-analysis. Neuroscience Letters, 659, 115–119. https://doi.org/https://doi.org/10.1016/j.neulet.2017.08.076
- Flöel, A. (2014). tDCS-enhanced motor and cognitive function in neurological diseases. Neuroimage, 85(Part 3), 934–947. https://doi.org/https://doi.org/10.1016/j.neuroimage.2013.05.098
- Fregni, F. (2005). Non-invasive brain stimulation for Parkinson’s disease: A systematic review and meta-analysis of the literature. Journal of Neurology, Neurosurgery & Psychiatry, 76(12), 1614–1623. https://doi.org/https://doi.org/10.1136/jnnp.2005.069849
- Fregni, F., Boggio, P. S., Santos, M. C., Lima, M., Vieira, A. L., Rigonatti, S. P., Silva, M. T. A., Barbosa, E. R., Nitsche, M. A., & Pascual-Leone, A. (2006). et al. Noninvasive Cortical Stimulation with Transcranial Direct Current Stimulation in Parkinson’s Disease. Movement Disorders, 21(10), 1693–1702. https://doi.org/https://doi.org/10.1002/mds.21012
- Gallagher, R., Marquez, J., & Osmotherly, P. (2019). Clinimetric properties and minimal clinically important differences for a battery of gait, balance, and cognitive examinations for the tap test in idiopathic normal pressure hydrocephalus. Neurosurgery, 84(6), 378–384. https://doi.org/https://doi.org/10.1093/neuros/nyy286
- Geisler, S., Derst, C., Veh, R. W., & Zahm, D. S. (2007). Glutamatergic afferents of the ventral tegmental area in the rat. The Journal of Neuroscience, 27(21), 5730–5743. https://doi.org/https://doi.org/10.1523/JNEUROSCI.0012-07.2007
- Giguère, N., Burke, N. S., & Trudeau, L.-E. (2018). On cell loss and selective vulnerability of neuronal populations in Parkinson’s disease. Frontiers in Neurology, 9, 455. https://doi.org/https://doi.org/10.3389/fneur.2018.00455
- Han, X., Jing, M. Y., Zhao, T. Y., Wu, N., song, R., & Li, J. (2017). Role of dopamine projections from ventral tegmental area to nucleus accumbens and medial prefrontal cortex in reinforcement behaviors assessed using optogenetic manipulation. Metabolic Brain Disease, 32(5), 1491–1502. https://doi.org/https://doi.org/10.1007/s11011-017-0023-3
- Hauser, R. A. (2009). Levodopa: Past, present, and future. European Neurology, 62(1), 1–8. https://doi.org/https://doi.org/10.1159/000215875
- He, R., Yan, X., Guo, J., Xu, Q., Tang, B., & Sun, Q. (2018). Recent advances in biomarkers for Parkinson’s disease. Frontiers in Aging Neuroscience, 10, 305. https://doi.org/https://doi.org/10.3389/fnagi.2018.00305
- Heim, B., Krismer, F., De Marzi, R., & Seppi, K. (2017). Magnetic resonance imaging for the diagnosis of Parkinson’s disease. J. Neural Transm. (Vienna), 124(8), 915–964. https://doi.org/https://doi.org/10.1007/s00702-017-1717-8
- Herwig, U., Satrapi, P., & Schönfeldt-lecuona, C. (2003). Using the international 10–20 EEG system for positioning of transcranial magnetic stimulation. Brain Topography, 16(2), 95–99. https://doi.org/https://doi.org/10.1023/b:brat.0000006333.93597.9d
- Hosp, J. A., Pekanovic, A., Rioult-Pedotti, M. S., & Luft, A. R. (2011). Dopaminergic projections from midbrain to primary motor cortex mediate motor skill learning. Journal of Neuroscience, 31(7), 2481–2487. https://doi.org/https://doi.org/10.1523/JNEUROSCI.5411-10.2011
- Hughes, A. J., Daniel, S. E., Kilford, L., & Lee, A. J. (1992). Accuracy of clinical diagnosis of idiopathic Parkinson’s disease: A clinic-pathological study of 100 cases. Journal of Neurology, Neurosurgery and Psychiatry, 55(3), 181–184. https://doi.org/https://doi.org/10.1136/jnnp.55.3.181
- Isaias, I. U., Trujillo, P., Summers, P., Marotta, G., Mainardi, L., Pezzoli, G., Zecca, L., & Costa, A. (2016). Neuromelanin imaging and dopaminergic loss in Parkinson’s Disease. Frontiers in Aging Neuroscience, 8, 196. https://doi.org/https://doi.org/10.3389/fnagi.2016.00196
- Ishikuro, K., Dougu, N., Nukui, T., Yamamoto, M., Nakatsuji, Y., Kuroda, S., Matsushita, I., Nishimaru, H., Araujo, M. F. P., & Nishijo, H. (2018). Effects of transcranial direct current stimulation (tDCS) over the frontal polar area on motor and executive functions in Parkinson’s Disease; A pilot study. Frontiers in Aging Neuroscience, 10, 231. https://doi.org/https://doi.org/10.3389/fnagi.2018.00231
- Ishikuro, K., Urakawa, S., Takamoto, K., Ishikawa, A., Ono, T., & Nishijo, H. (2014). Cerebral functional imaging using near-infrared spectroscopy during repeated performances of motor rehabilitation tasks tested on healthy subjects. Frontiers in Human Neuroscience, 8, 292. https://doi.org/https://doi.org/10.3389/fnhum.2014.00292
- Kalivas, P. W. (1993). Neurotransmitter regulation of dopamine neurons in the ventral tegmental area. Brain Research Reviews, 18(1), 75–113. https://doi.org/https://doi.org/10.1016/0165-0173(93)90008-n
- Kaneko, T., & Muraki, T. (1990). Development and standardization of the hand function test. Bulletin of Allied Medical Sciences Kobe, 6, 49–54.
- Kaski, D., Allum, J. H., Bronstein, A. M., & Dominguez, R. O. (2014). Applying anodal tDCS during tango dancing in a patient with Parkinson’s disease. Neuroscience Letters, 568, 39–43. https://doi.org/https://doi.org/10.1016/j.neulet.2014.03.043
- Khedr, E. M., Shawky, O. A., El-Hammady, D. H., Rothwell, J. C., Darwish, E. S., Mostafa, O. M., & Tohamy, A. M. (2013). Effect of anodal versus cathodal transcranial direct current stimulation on stroke rehabilitation: A pilot randomized controlled trial. Neurorehabilitation and Neural Repair, 27(7), 592–601. https://doi.org/https://doi.org/10.1177/1545968313484808
- Kintz, N., Petzinger, G. M., & Jakowec, M. W. (2017). treadmill exercise modifies dopamine receptor expression in the prefrontal cortex of the 1-methyl-4-phenyl-1,2,3,6-tetrahydropyridine-lesioned mouse model of Parkinson’s disease. Neuroreport, 28(15), 987–995. https://doi.org/https://doi.org/10.1097/WNR.0000000000000865
- Langley, J., Huddleston, D. E., Liu, C. J., & Hu, X. (2017). Reproducibility of locus coeruleus and substantia nigra imaging with neuromelanin sensitive MRI. Magnetic Resonance Materials in Physics, Biology and Medicine, 30(2), 121–125. https://doi.org/https://doi.org/10.1007/s10334-016-0590-z
- Lefaucheur, J. P., Antal, A., Ayache, S. S., Benninger, D. H., Brunelin, J., Cogiamanian, F., Cotelli, M., Ridder, D. D., Ferrucci, R., Langguth, B., Marangoko, P., Mylius, V., Nitsche, M., Padberg, F., Palm, U., Poulet, E., Priori, A., Rossi, S., Schecklmann, M., Vanneste, S., … Paulus, W. (2017). Evidence-based guidelines on the therapeutic use of transcranial direct current stimulation(tDCS). Clinical Neurophysiology, 128(1), 56–92. https://doi.org/https://doi.org/10.1016/j.clinph.2016.10.087
- Liebetanz, D. (2002). Pharmacological approach to the mechanisms of transcranial DC-stimulation-induced after-effects of human motor cortex excitability. Brain, 125(Pt 10), 2238–2247. https://doi.org/https://doi.org/10.1093/brain/awf238
- Lu, C., Wei, Y., Hu, R., Wang, Y., Li, K., & Li, X. (2015). Transcranial direct current stimulation ameliorates behavioral deficits and reduces oxidative stress in 1‐methyl‐4‐phenyl‐1, 2, 3, 6‐tetrahydropyridine‐induced mouse model of Parkinson’s disease. Neuromodulation: Technology at the Neural Interface, 18(6), 442–447. https://doi.org/https://doi.org/10.1111/ner.12302
- Martínez-Martín, P., Fontán, C., Frades, P. B., & Petidier, R. (2000). Parkinson’s disease: Quantification of disability based on the unified Parkinson’s disease rating scale. Neurologia, 15(9), 382–387.
- Matsuura, K., Maeda, M., Yata, K., Ichiba, Y., Yamaguchi, T., Kanamaru, K., & Tomimoto, H. (2013). Neuromelanin magnetic resonance imaging in Parkinson’s disease and multiple system atrophy. European Neurology, 70(1-2), 70–77. https://doi.org/https://doi.org/10.1159/000350291
- McGregor, M. M., McKinsey, G. L., Girasole, A. E., Bair-Marshall, C. J., Rubenstein, J. L. R., & Nelson, A. B. (2019). Functionally distinct connectivity of developmentally targeted striosome neurons. Cell Reports, 29(6), 1419–1428.e5. https://doi.org/https://doi.org/10.1016/j.celrep.2019.09.076
- Meinzer, M., Darkow, R., Lindenberg, R., & Flöel, A. (2016). Electrical stimulation of the motor cortex enhances treatment outcome in post-stroke aphasia. Brain, 139(4), 1152–1163. https://doi.org/https://doi.org/10.1093/brain/aww002
- Michel, P. P., Alvarez-Fischer, D., Guerreiro, S., Hild, A., Hartmann, A., & Hirsch, E. C. (2007). Role of activity-dependent mechanisms in the control of dopaminergic neuron survival. Journal of Neurochemistry, 101(2), 289–297. https://doi.org/https://doi.org/10.1111/j.1471-4159.2006.04424.x
- Murase, S., Grenhoff, J., Chouvet, G., Gonon, F. G., & Svensson, T. H. (1993). Prefrontal cortex regulates burst firing and transmitter release in rat mesolimbic dopamine neurons studied in vivo. Neuroscience Letters, 157, 53–56. https://doi.org/https://doi.org/10.1016/0304-3940(93)90641-w
- Ni, M., Hazzard, J. B., Signorile, J. F., & Luca, C. (2018). Exercise guidelines for gait function in Parkinson’s disease: A systematic review and meta-analysis. Neurorehabilitation and Neural Repair, 32, 872–886. https://doi.org/https://doi.org/10.1177/1545968318801558
- Nitsche, M. A., Fricke, K., Henschke, U., Schlitterlau, A., Liebetanz, D., Lang, N., Henning, S., Tergau, F., & Paulus, W. (2003). Pharmacological modulation of cortical excitability shifts induced by transcranial direct current stimulation in humans. The Journal of Physiology, 553(1), 293–301. https://doi.org/https://doi.org/10.1113/jphysiol.2003.049916
- Nitsche, M. A., Liebetanz, D., Lang, N., Antal, A., Tergau, F., & Paulus, W. (2003). Safety criteria for transcranial direct current stimulation (tDCS) in humans. Clinical Neurophysiology, 114(11), 2220–2222. https://doi.org/https://doi.org/10.1016/s1388-2457(03)00235-9
- Ohtsuka, C., Sasaki, M., Konno, K., Kato, K., Takahashi, J., Yamashita, F., & Terayama, Y. (2014). Differentiation of early-stage parkinsonisms using neuromelanin-sensitive magnetic resonance imaging. Parkinsonism & Related Disorders, 20(7), 755–760. https://doi.org/https://doi.org/10.1016/j.parkreldis.2014.04.005
- Ohtsuka, C., Sasaki, M., Konno, K., Koide, M., Kato, K., Takahashi, J., Takahashi, S., Kudo, K., Yamashita, F., & Terayama, Y. (2013). Changes in substantia nigra and locus coeruleus in patients with early-stage Parkinson’s disease using neuromelanin-sensitive MR imaging. Neuroscience Letters, 541, 93–98. https://doi.org/https://doi.org/10.1016/j.neulet.2013.02.012
- Okuzumi, A., Hatano, T., Kamagata, K., & Hattori, N. (2019). Neuromelanin or DaT-SPECT : Which is the better marker for discriminating advanced Parkinson’s disease? European Journal of Neurology, 26(11), 1408–1416. https://doi.org/https://doi.org/10.1111/ene.14009
- Ota, Y., Takamoto, K., Urakawa, S., Nishimaru, H., Matsumoto, J., Takamura, Y., Mihara, M., Ono, T., & Nishijo, H. (2020). Motor Imagery Training with Neurofeedback from the Frontal Pole Facilitated Sensorimotor Cortical Activity and Improved Hand Dexterity. Frontiers in Neuroscience, 14, 34. https://doi.org/https://doi.org/10.3389/fnins.2020.00034
- Pereira, J. B., Junqué, C., Bartrés-Faz, D., Martí, M. J., Sala-Llonch, R., Compta, Y., Falcon, C., Vendrell, P., Pascul-Leone, A., Valls-Sole, J., & Tolosa, E. (2013). Modulation of verbal fluency networks by transcranial direct current stimulation (tDCS) in Parkinson’s disease. Brain Stimulation, 6(1), 16–24. https://doi.org/https://doi.org/10.1016/j.brs.2012.01.006
- Peterson, A. C., & Li, C. S. R. (2018). Noradrenergic Dysfunction in Alzheimer’s and Parkinson’s diseases—an overview of imaging studies. Frontiers in Aging Neuroscience, 10, 127. https://doi.org/https://doi.org/10.3389/fnagi.2018.00127
- Petzinger, G. M., Walsh, J. P., Akopian, G., Hogg, E., Abernathy, A., Arevalo, P., Turnquist, P., Vuckovic, M., Fisher, B. E., Togasaki, D. M., & Jakowec, M. W. (2007). Effects of treadmill exercise on dopaminergic transmission in the 1-methyl-4-phenyl-1,2,3,6-tetrahydropyridine-lesioned mouse model of basal ganglia injury. The Journal of Neuroscience, 27(20), 5291–5300. https://doi.org/https://doi.org/10.1523/JNEUROSCI.1069-07.2007
- Poreisz, C., Boros, K., Antal, A., & Paulus, W. (2007). Safety aspects of transcranial direct current stimulation concerning healthy subjects and patients. Brain Research Bulletin, 72(4-6), 208–214. https://doi.org/https://doi.org/10.1016/j.brainresbull.2007.01.004
- Postuma, R. B., Berg, D., Stern, M., Poewe, W., Olanow, C. W., Oertel, W., Obeso, J., Marek, K., Litvan, I., Lang, A. E., Halliday, G., Goetz, C. G., Gasser, T., Dubois, B., Chan, P., Bloom, B. R., Adler, C. H., & Deuschi, G. (2015). MDS clinical diagnostic criteria for Parkinson’s disease. Movement Disorders, 30(12), 1591–1601. https://doi.org/https://doi.org/10.1002/mds.26424
- Reimão, S., Pita, L., Neutel, P., Correia, D., Guedes, L., Coelho, M., Campos, M. M., Ferreira, J. J., Ferreira, J., Abreu, D., Gonçalves, N., Morgado, C., Nunes, R. G., Campos, M. M., & Ferreira, J. J. (2015). Substantia nigra neuromelanin magnetic resonance imaging in de novo Parkinson’s disease patients. European Journal of Neurology, 22(3), 540–546. https://doi.org/https://doi.org/10.1111/ene.12613
- Russo, C., Souza, C. M., Bolognini, I., & Fregni, F. (2017). Safety review of transcranial direct current stimulation in stroke. Neuromodulation, 20(3), 215–222. https://doi.org/https://doi.org/10.1111/ner.12574
- Sasaki, M., Shibata, E., Tohyama, K., Takahashi, J., Otsuka, K., Tsuchiya, K., Takahashi, S., Ehara, S., Terayama, Y., & Sasaki, A. (2006). Neuromelanin magnetic resonance imaging of locus ceruleus and substantia nigra in Parkinson’s disease. Neuroreport, 17(11), 1215–1218. https://doi.org/https://doi.org/10.1097/01.wnr.0000227984.84927.a7
- Schwarz, S. T., Xing, Y., Tomar, P., Bajaj, N., & Auer, D. P. (2017). In vivo assessment of brainstem depigmentation in Parkinson disease: potential as a severity marker for multicenter studies. Radiology, 283(3), 789–798. https://doi.org/https://doi.org/10.1148/radiol.2016160662
- Shulman, L. M., Gruber-Baldini, A. L., Anderson, K. E., Fishman, P. S., Reich, S. G., & Weiner, W. J. (2010). The clinically important difference on the unified Parkinson’s disease rating scale. Archives of Neurology, 67(1), 64–70. https://doi.org/https://doi.org/10.1001/archneurol.2009.295
- Simpson, M. W., & Mak, M. (2020). The effect of transcranial direct current stimulation on upper limb motor performance in Parkinson’s disease: A systematic review. Journal of Neurology, 267(12), 3479–3488. https://doi.org/https://doi.org/10.1007/s00415-019-09385-y
- Suarez-García, D. M. A., Grisales-Cárdenas, J. S., Zimerman, M., & Cardona, J. F. (2020). Transcranial direct current stimulation to enhance cognitive impairment in Parkinson’s Disease: A systematic review and meta-analysis. Frontiers in Neurology, 11, 597955. https://doi.org/https://doi.org/10.3389/fneur.2020.597955
- Talai, A. S., Sedlacik, J., Boelmans, K., & Forkert, N. D. (2021). Utility of multi-modal MRI for differentiating of Parkinson’s disease and progressive supranuclear palsy using machine learning. Frontiers in Neurology, 12, 648548. https://doi.org/https://doi.org/10.3389/fneur.2021.648548
- Taniguchi, D., Hatano, T., Kamagata, K., Okuzumi, A., Oji, Y., Mori, A., Hori, M., Aoki, S., & Hattori, N. (2018). Neuromelanin imaging and midbrain volumetry in progressive supranuclear palsy and Parkinson’s disease. Movement Disorders, 33(9), 1488–1492. https://doi.org/https://doi.org/10.1002/mds.27365
- Tombaugh, T. N. (2004). Trail making test A and B: Normative data stratified by age and education. Archives of Clinical Neuropsychology, 19(2), 203–214. https://doi.org/https://doi.org/10.1016/S0887-6177(03)00039-8
- Tong, Z. Y., Overton, P. G., & Clark, D. (1996). Stimulation of the prefrontal cortex in the rat induces patterns of activity in midbrain dopaminergic neurons which resemble natural burst events. Synapse, 22(3), 195–208. https://doi.org/https://doi.org/10.1002/(SICI)1098-2396(199603)22:3<195::AID-SYN1>3.0.CO;2-7
- Toy, W. A., Petzinger, G. M., Leyshon, B. J., Akopian, G. K., Walsh, J. P., Hoffman, M. V., Vuckovic, M. G., & Jakowec, M. W. (2014). Treadmill exercise reverses dendritic spine loss in direct and indirect striatal medium spiny neurons in the 1-methyl-4-phenyl-1,2,3,6-tetrahydropyridine (MPTP) mouse model of Parkinson’s disease. Neurobiology of Disease, 63, 201–209. https://doi.org/https://doi.org/10.1016/j.nbd.2013.11.017
- Underwood, C. F., & Parr-Brownlie, L. C. (2021). Primary motor cortex in Parkinson’s disease: Functional changes and opportunities for neurostimulation. Neurobiology of Disease, 147, 105159. https://doi.org/https://doi.org/10.1016/j.nbd.2020.105159
- Van, der, Pluijm, M., Cassidy, C., Zandstra, M., Wallert, E., ., De, Bruin, K., Booij, J., de Haan, L., Horga, G., & van, de, Giessen, E. (2021). Reliability and reproducibility of neuromelanin-sensitive imaging of the substantia Nigra: A comparison of three different sequences. Journal of Magnetic Resonance Imaging, 53(3), 712–721. https://doi.org/https://doi.org/10.1002/jmri.27384
- Van, Domburg, P. H., & ten, Donkelaar, H. J. (1991). The human substantia nigra and ventral tegmental area. A Neuroanatomical Study with Notes on Aging and Aging Diseases. Advances in Anatomy Embryology and Cell Biology, 121, 1–132.
- Vučković, M. G., Li, Q., Fisher, B., Nacca, A., Leahy, R. M., Walsh, J. P., Mukherjee, J., Williams, C., Jakoweac, M. W., & Petzinger, G. M. (2010). Exercise elevates dopamine D2 receptor in a mouse model of Parkinson’s disease: In vivo imaging with [18F]fallypride. Movement Disorders, 25(16), 2777–2784. https://doi.org/https://doi.org/10.1002/mds.23407
- Wengler, K., He, X., Abi-Dargham, A., & Horga, G. (2020). Reproducibility assessment of neuromelanin-sensitive magnetic resonance imaging protocols for region-of-interest and voxelwise analyses. NeuroImage, 208, 116457. https://doi.org/https://doi.org/10.1016/j.neuroimage.2019.116457