ABSTRACT
Diagnostic criteria for dyslexia describe specific reading difficulties, and single-deficit models, including the phonological deficit theory, have prevailed. Children seeking diagnosis, however, do not always show phonological deficits, and may present with strengths and challenges beyond reading. Through extensive neurological, neuropsychological, and academic evaluation, we describe four children with visuospatial, socio-emotional, and attention impairments and spared phonology, alongside long-standing reading difficulties. Diffusion tensor imaging revealed white matter alterations in inferior longitudinal, uncinate, and superior longitudinal fasciculi versus neurotypical children. Findings emphasize that difficulties may extend beyond reading in dyslexia and underscore the value of deep phenotyping in learning disabilities.
1. Introduction
Developmental dyslexia (henceforth dyslexia), a specific learning disability characterized by pervasive difficulties in learning to read, despite typical motivation, intelligence, and instruction (Lyon et al., Citation2003) is common, with a 5–17% prevalence of between 5.6 and 6.6%, depending on the diagnostic criteria employed (Di Folco et al., Citation2022). Models of dyslexia have largely focused on mapping a single deficit that could explain the observed reading difficulties with candidates including phonological impairment (Stanovich, Citation1988; Swan & Goswami, Citation1997), slow automatized naming (Wolff et al., Citation1990), and reduced verbal working memory capacity (Berninger et al., Citation2006).
Many individuals with trouble reading, however, do not show these purported underlying language deficits (Pennington et al., Citation2012; Reid et al., Citation2007), implying substantial variation in the underlying cognitive features (Di Betta & Romani, Citation2006; Ellis, Citation1985; Martin, Citation1995; McCloskey & Rapp, Citation2000), and alternative approaches acknowledge that learning to read relies on multiple processes (Bishop & Snowling, Citation2004; Pennington, Citation2006; Wolf & Bowers, Citation1999). Some accounts therefore propose upstream differences in vision (or visual attention) and audition, which may have secondary effects on phonology and reading (Bosse et al., Citation2007; De Luca et al., Citation2002; Eden, Stein, etal., Citation1996; Facoetti et al., Citation2005; Goswami, Citation2011; Watson & Willows, Citation1993).
Existing accounts, however, provide incomplete explanations for the frequent presentation of non-linguistic differences in dyslexia, including psychiatric (Hendren et al., Citation2018) and visuospatial (McCloskey & Rapp, Citation2000; Ramus et al., Citation2003; White et al., Citation2006) features. Such cases are usually explained as reflecting comorbidity, for example, a co-occurrence of other neurodevelopmental conditions (e.g., Snowling, Citation2012), such as attention deficit hyperactivity disorder (ADHD; Germanò et al., Citation2010). Comorbidity positions additional non-reading features as distinct from dyslexia, indicating the presence of multiple diagnostic categories. As many as 40% of school-age children who meet the criteria for one neurodevelopmental disorder also meet the criteria for another (Kadesjö & Gillberg, Citation2002; McArthur et al., Citation2000; Rochelle & Talcott, Citation2006; Willcutt & Pennington, 2000), however, suggesting this is far from a rare occurrence. Clinical research has a tendency to discretize and focus on “pure” cases who only show a single clear phenotype, but this may distort our understanding of how strengths and vulnerabilities develop in tandem. For example, in a study (Saksida et al., Citation2016) it was concluded that visual processing variables did not explain any additional variance in literacy in children with dyslexia after accounting for phonological variables, but participants with other co-occurring challenges, including inattention, were excluded. To better understand how performance across domains is related and the associated neuroanatomy, investigations of so-called “atypical” cases are needed.
Dyslexia has been associated with structural and functional differences in the left cerebral hemisphere (Caverzasi et al., Citation2018; Rimrodt et al., Citation2010; Silani et al., Citation2005), in line with findings suggesting that mature reading is supported by a left-hemisphere network of frontal, temporoparietal, and occipitotemporal cortical regions (Fiez & Petersen, Citation1998; Turkeltaub et al., Citation2002). Given the disparate regions involved, reading likely also requires adequate communication between regions. Indeed, reduced functional and structural connectivity between reading network regions has been observed in dyslexia (Finn et al., Citation2014; Rimrodt et al., Citation2010). In particular, reduced integrity of the left arcuate fasciculus, which connects temporoparietal and inferior frontal regions, and the inferior fronto-occipital fasciculus, linking occipital and temporal regions, has been observed (Cui et al., Citation2016; Rimrodt et al., Citation2010; Vandermosten et al., Citation2012). Many of these brain associations, however, have not replicated (Meisler & Gabrieli, Citation2022; Ramus et al., Citation2018), with small sample sizes and variable methodology cited as potential explanations. Moreover, subtypes of dyslexia with distinct cognitive impairments may relate to distinguishable anatomical markers (Jednoróg et al., Citation2014; Pecini et al., Citation2011; van Ermingen-Marbach et al., Citation2013) which likely introduces heterogeneity into group studies that collapse across subtypes.
A focus on mapping the language profiles of individuals with dyslexia has undoubtedly contributed tremendously to our understanding of the academic challenges these individuals face, and potential remediation strategies for these challenges. This focus has been constrained, however, by the prevailing single deficit accounts of the condition and the narrow focus on “pure” cases and those with a primary phonological deficit. A thorough understanding of the underlying causes of reading impairment, which can account for the heterogeneous profiles of individuals who carry this diagnosis, continues to elude us. A lack of homogeneity at the level of underlying cognitive deficit(s) may preclude the rationale for group-based studies (Badian, Citation1997; Ho et al., Citation2002). As an alternative, deep phenotyping involves the fine-grained individual-basis examination of the multiple discrete components that comprise a phenotype and is a key tool in precision medicine (Delude, Citation2015). Such an approach has already proven effective in uncovering the existence of visuospatial difficulties in dyslexia (McCloskey & Rapp, Citation2000). Here, we describe a series of four children that show both marked reading difficulties commensurate with the diagnostic criteria for dyslexia, in addition to several other strengths and challenges not included in the diagnostic criteria. Family, birth, and developmental histories for each of these cases are reported, as well as the results of neurological, neuropsychological, and academic testing. Segmentation of major white matter pathways bilaterally was performed, and compared with a group of neurotypical children.
2. Methods
2.1 Participants
The study sample is formed of two groups of participants: the four cases demonstrating atypical dyslexia, and a comparison group of neurotypical children (n = 18). Almost half the participants were monolingual English speakers (41%), 18% were Bilingual English speakers, 14% had some exposure to an additional language other than English, and 27% were absent information on language background. All participants underwent magnetic resonance imaging (MRI) and data from the neurotypical participants were used solely for the interpretation of neuroimaging data. Detailed case reports, cognitive, and academic data are only provided for the four atypical cases. All procedures were approved by the local institutional ethical review board and conducted in agreement with the Declaration of Helsinki. Guardians provided informed consent for their participation in the research and the children provided their assent.
2.1.1 Selection of illustrative cases for case report
A total of 270 pediatric cases were seen at the University of California San Francisco (USCF) Dyslexia Center during the period 2014–2020. Of these 270, 37 showed evidence of both dyslexia and co-occurring visuo-spatial, visuo-motor, or socio-emotional challenges. In this case series, we focus on describing in detail four illustrative cases. All four cases were native English speakers and monolingual. See for demographic information and presenting diagnoses at the time of evaluation for each of these four children. See Case reports section 3.1 for detailed descriptions of the history and current presenting concerns of the four cases.
Table 1. Demographic characteristics, early life history, and neurological signs on examination.
2.2 Procedure
Each of the four cases underwent a detailed evaluation by a multidisciplinary team at the UCSF Dyslexia Center, which included family and developmental histories, neuropsychological evaluation, neurological examination, and academic testing. Primary caregivers completed the Behavioral Assessment System for Children (BASC-2; Reynolds & Kamphaus, Citation2004), the Vanderbilt ADHD Diagnostic Parent Rating Scale (Wolraich et al., Citation2003), and the Sensory Profile (Dunn, Citation1999).
Nonverbal reasoning was measured with the Matrix Reasoning section of the Wechsler Abbreviated Scales of Intelligence (WASI, Psychological Corporation, Citation1999). Receptive vocabulary was measured using Receptive One-Word Picture Vocabulary Test – fourth edition (ROWPVT-4; Martin & Brownell, Citation2011), to provide an index of verbal reasoning ability. Short-term and working memory capacity was measured using digit and spatial spans, forwards and backwards, from the Wechsler Intelligence Scale for Children – Integrated – fourth edition (WISC-IV Integrated; Wechsler et al., Citation2004). The Word Generation subtest from the NEPSY Developmental Neuropsychological Assessment – second edition (NEPSY-II; Korkman et al., Citation2007) was used to derive scores of semantic and phonemic fluency. Phonological awareness was gauged using Segmentation, Sound Blending, and Sound Awareness measures from the Woodcock Johnson IV Test of Achievement (Schrank et al., Citation2014). Rapid automized naming was measured using the Rapid Picture Naming task from the Woodcock Johnson IV Test of Achievement (Schrank et al., Citation2014). Memory for words was measured with the Woodcock Johnson IV Test of Achievement subtest of the same name (Schrank et al., Citation2014).
Reading of real- and pseudo-words was assessed with untimed single-word reading measures (i.e., Letter-Word Identification and Word Attack) from the Woodcock Johnson IV Test of Achievement (Schrank et al., Citation2014) as well as the timed Test of One-Word Reading Efficiency – second edition (TOWRE-2; Torgesen et al., Citation2012) and the Gray Oral Reading Test – fifth edition (GORT-5; Wiederholt & Bryant, Citation2012), which measures paragraph reading ability. Specifically, both the ability to recognize familiar words and “sound out” unfamiliar words were assessed. For familiar words, the Letter-Word Identification subtest of the Woodcock Johnson Tests of Achievement requires participants to read a list of real words of increasing difficulty, tapping word-identification skills. The Sight Word Efficiency (SWE) subtest of the TOWRE-2 is similar to the letter-word identification subtest of the Woodcock Johnson IV Test of Achievement, assessing the number of real printed words that can be accurately read within 45 s. For unfamiliar words, the Word Attack subtest of the Woodcock Johnson IV Test of Achievement requires participants to pronounce nonsense words of increasing complexity, tapping the ability to apply phonetic and decoding skills to unfamiliar words. Similarly, the Phonetic Decoding Efficiency (PDE) subtest of the TOWRE-2 requires participants to do the same within 45 s. Paragraph reading was assessed using the GORT-5, which requires participants to read passages of text aloud and answer open-ended questions on the content. Scores reflect reading rate, fluency, accuracy, and comprehension.
Visuospatial processing was assessed using the Judgment of Line Orientation (Benton et al., Citation1975), Beery-Buktenica Test of Visuomotor Integration – fourth edition (short form; Beery & Beery, Citation2010), and copy and recall of the Rey-Osterrieth Complex Figure (Osterrieth, Citation1944). Judgment of Line Orientation requires the participant to indicate which of 11 printed lines match the visual angle of two target lines (Benton et al., Citation1975). The task is repeated for a total of 30 trials. Copy of the Complex Figure (Osterrieth, Citation1944) requires participants to reproduce a complex line drawing by copying freehand, thus providing a measure of visuospatial constructional ability as well as organizational strategy. Recall is completed 3 minutes later and requires the participant to draw from memory the original figure, providing an indication of visuospatial memory. The Beery-Buktenica Test of Visuomotor Integration is a test of the participant’s ability to coordinate visual and motor abilities. In this short-form version, the participant is required to copy freehand 15 geometric forms of increasing complexity.
Visuomotor tracking and divided attention was assessed using the Children’s Colored Trails, A and B (Williams et al., Citation1995). Mathematical ability was assessed using the Woodcock Johnson IV Tests of Achievement Calculations subtest. Participants also completed a novel computerized visuospatial navigation task, which required them to learn a series of 15 turns through a virtual neighborhood, which they navigated by turning a steering wheel and selecting their chosen direction. Participants could make use of both egocentric cues (e.g., I turn to my left after I turn to the right) and allocentric cues (e.g., I turn left after the general store) to aid navigation. They had a total of 15 attempts to correctly complete the route. Feedback was provided on each trial via auditory and visual signals that an incorrect turn had been attempted. Additionally, participants’ recall of the route was assessed after a 35–45-min delay. The number of attempts that it took participants to learn the route (i.e., completion of all 15 turns without error) was recorded (maximum = 15), as well as the number of turns that they recalled after the 35–45-minute delay (maximum = 15).
Unless otherwise stated, all scores reported in the Results represent percentile scores using published norms. Discretized norms are not currently available for the poorest copy performance on the Rey-Osterrieth Complex Figure, therefore in order to enable comparison with the other measures collected, new percentile scores were imputed as follows. For percentile scores of ≥16, 11–16, 6–10, 2–5, and ≤1, scores of 20, 13.5, 8, 3.5, and 0.5 were imputed, respectively. In addition, norms are not currently available for the novel visuospatial navigation task; we therefore report whether participants learnt the route and whether they successfully replicated it after the delay period. Children without a history of academic challenges, forming the neurotypical cohort, underwent an abridged evaluation comprising nonverbal reasoning measured with Matrix Reasoning, and timed single-word reading on the TOWRE-2 to verify their group membership.
2.3 Neuroimaging
The comparison group was formed to aid in interpretation of white matter segmentation results, therefore, all participants, including the four cases, underwent a brain magnetic resonance imaging (MRI) acquisition. To closely match the demographic characteristics of the cases, 18 right-handed neurotypical children (50% male; M age = 8.7, SD age = 0.8), aged between 7 and 10 years, were selected from the UCSF Dyslexia Center cohort. Comparison groups were matched to the cases at the group level on age [W = 36.5, p = 1] and sex [χ2(2) = 0.12, p = .724].
A high-resolution T1-weighted three-dimensional image and diffusion-weighted images (DWI) were acquired for each participant within 12 months of cognitive evaluation. Fifteen participants were scanned on a 3-T Siemens Trio scanner equipped with a 12-channel head/neck coil and 21 on a 3-T Siemens Prisma scanner equipped with a 64-channel head/neck coil (Siemens, Erlangen, Germany). Scanner use was randomly distributed among the groups [χ2(2) = 2.79, p = .095]. The protocol included a T1-weighted three-dimensional sagittal Magnetization Prepared Rapid Acquisition Gradient Echo (MPRAGE) sequence with the following parameters: 160 sagittal slices; TR/TE/TI = 2300/2.98/900 ms, 1 × 1 × 1 mm3 voxel size, field of view (FOV): 256 × 240 mm2, flip angle = 9 deg, parallel imaging acceleration factor (iPAT) = 2. DWI data were acquired with a single-shot spin-echo echo-planar imaging (SS-EPI) sequence with the following parameters for the Trio scanner: 55 axial slices, in-plane resolution of 2.2 × 2.2 mm2, slice thickness of 2.2 mm, TR/TE = 8000/109 ms, FOV: 220 × 220 mm2, flip angle = 90 deg; 64 noncollinear diffusion sensitization directions at b = 2000 s/mmm2 and 1 volume at b = 0 s/mmm2, iPAT = 2; and the following parameters for the Prisma scanner: 69 axial slices, in-plane resolution of 2 × 2 mm2, slice thickness of 2 mm, TR/TE = 2420/72.20 ms, FOV: 200 × 200, flip angle = 85 deg; 96 noncollinear diffusion sensitization directions at b = 2500 s/mmm2 and 10 volumes at b = 0 s/mmm2, iPAT = 2 and multiband factor of 3. Images were visually inspected by two experienced neuroradiologists for quality control purposes and to exclude the presence of artifacts and brain abnormalities; all were negative.
Automated bilateral segmentation of major white matter pathways comprising arcuate fasciculus, superior longitudinal fasciculus (segments I and II [SLF-IP]; temporoparietal segment [SLF-TP], Kamali et al., Citation2014), frontal aslant, uncinate fasciculus, inferior fronto-occipital fasciculus (IFOF), and inferior longitudinal fasciculus (ILF) was performed. The automated segmentation pipeline is described in detail in Jordan et al. (Citation2021). We focused on structural asymmetry, measured with laterality indexes (number of voxels), and tract integrity, measured with fractional anisotropy (FA). Outliers in the comparison group were considered to be ± two standard deviations from the mean level in the tract; these indexes were removed. Two-sample t-tests were performed to analyze group differences in tract metrics; non-parametric Mann–Whitney tests were substituted in instances where the data deviated significantly from normality. Readers should note that due to the exploratory nature of the study, we did not perform corrections for multiple comparisons.
Results
3.1 Case reports
3.1.1 Case 1
A right-handed male with a previous diagnosis of dyslexia, was 9 years old at the time of evaluation. Concerns about his development first arose in preschool, as he seemed delayed relative to his peers. He would become upset when asked to write, including his own name. He obtained a diagnosis of dyslexia prior to second grade and enrolled in a specialized school for children with learning differences in the third grade. Maternal and paternal grandparents were suspected of having dyslexia, and maternal grandparents had a history of anxiety.
Case 1 reported sensory sensitivity to certain clothing patterns and tags. He reported that plaid patterns bothered him, and he was a picky eater. Responses on the Sensory Profile indicated a definite difference in vestibular processing, emotional and social responses to sensory stimulation, and behavioral outcomes of sensory processing. He had some ongoing motor stereotypies such as hand-flapping when excited. Coordination difficulties pertained mainly to writing, and he offered that he “finds it hard to get ideas on paper.” He also experienced some left–right confusion, and a mild delay in learning to tie his shoe laces, acquiring this skill by age nine. Neurological examination indicated bilateral slow fine finger movements that were poorly fractionated (i.e., difficulty isolating movement of each finger) with some mirroring and significant dystonic posturing during stressed gait.
On testing, his poorest visuospatial performance was observed in judgment of line orientation with a score indicative of borderline impairment, while visuomotor integration scores were in the average range. Language testing revealed phonological awareness, rapid automized naming, and memory for words in the average range, with the lowest performance in rapid picture naming at the 16th percentile. See for the results of academic and neuropsychological testing and for a summary of parents’ responses to questionnaire measures for all cases. He did well on the real-world test of navigation, learning the route by the seventh attempt and remembering all turns after the delay period. In tests of reading, he showed performance in the bottom fifth percentile on timed tests of single-word reading, both for real and non-words. Paragraph reading fluency, rate, accuracy, and comprehension were in the low average range. Indeed, he reported that his favorite academic subject was now reading, and he particularly liked adventure books. Learning a second language, however, proved very difficult for him.
Table 2. Scores on standardized neuropsychological and academic tests. Performance is indicated in terms of percentile (range = 0–100).
Table 3. Parent-reported behavior on questionnaires. Scores reflect standard scoring criteria for the Vanderbilt and Sensory Profile (a) and percentile (range = 0–100) for the BASC-2 (b).
Case 1 was well known at school for having a messy desk, distracted behavior, and fidgeting. He met criteria for ADHD inattentive subtype on the Vanderbilt questionnaire. Emotionally, he reported sometimes feeling sad and lonely. Responses on the BASC-2 indicated clinically significant withdrawal, and in addition suggested risk for anxiety, depression, and functional communication impairment. He prefers quieter one-to-one play to raucous fast-paced group play. At night, it could take him up to an hour to fall asleep, although it was unclear if this was related to over-activity or worry. Encounters with our multidisciplinary team of evaluators evidenced a typical amount and appropriate use of facial expressions, eye contact, and communicative gestures.
3.1.2 Case 2
A right-handed female, was 9 years old at the time of evaluation and previously received diagnoses of dyslexia and dyscalculia. A developmental history indicated early psychomotor differences: she was ambidextrous until age 3 years, tying her shoelaces proved difficult to master, and she remained uncoordinated to the present day. She had mild early difficulty with articulation, but otherwise her parents reported no concerns about her ability to communicate verbally. There was a family history of neurodevelopmental disorders. Her older sister carried diagnoses of dyslexia and dyscalculia and suspected ADHD. Her father suspected he had dyslexia although she had no formal diagnosis, and her mother suspected she had dyscalculia.
Concerns about Case 2’s academic performance arose in the first grade, when teachers noted that she was frequently reversing numbers and letters. She has since participated in a number of interventions, including the Wilson Reading System, and is reported to have made significant progress. She attended a mainstream elementary school but received ongoing mathematics and reading support. She reported that mathematics is her most difficult subject, alongside reading.
On testing, both her nonverbal reasoning and receptive vocabulary were in the high average range. Phonological awareness, rapid automized naming, and memory for words performance were in the average range, with a sound blending score in the superior range (93rd percentile). Scores on timed tests of single real- and non-word reading fell in the fifth percentile. Her paragraph reading rate, fluency, and comprehension fell within the average range, with reading accuracy in the low average range. On tests of visuospatial functioning, her scores were varied. Her judgment of line orientation was very poor, indicating ability in the lowest percentile, and she failed to learn the route in the real-world navigation task. Calculations testing also indicated impairment. She did a little better on the figure copy and recall, with performance commensurate with borderline impairment. By contrast, no impairment was observed in visuomotor integration, with an average range score. A radar plot comparing key academic and cognitive scores across domains in the four cases is shown in .
Figure 1. Radar plot of key percentile scores across cognitive, language, and academic domains in the four cases of dyslexia.
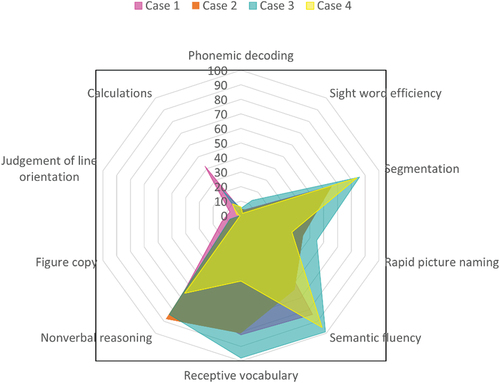
Parent responses to the Sensory Profile indicated a definite difference on a number of subscales, including vestibular processing, sensory processing related to endurance and tone, and sensory modulation related to body position and movement. Socially, her parents reported introversion when meeting new people, but she was described as extremely loyal and empathetic given sufficient time. Starting and maintaining conversations was very difficult, and she sometimes felt rejected by her peers. Her mother offered that “she gives of herself constantly, sometimes to her own detriment.” She often worried, was tense, and feared humiliation in front of her peers. Her mother also reported that she suffered from anxiety around bedtime and experienced nightmares once or twice a week. Scores on the BASC-2 indicated clinically significant anxiety and risk for functional communication impairment. Vanderbilt scores were not indicative of ADHD. Encounters with our multidisciplinary team of evaluators evidenced a typical amount and appropriate use of facial expressions, eye contact, and communicative gestures.
3.1.3 Case 3
A right-handed female, was 8 years old at the time of evaluation. She carried previous diagnoses of dyslexia, ADHD, and anxiety. A developmental history revealed that she was delayed in learning to dress herself and continued to have difficulties with putting on socks and tying shoelaces. Her handwriting was messy, and she had to be reminded to write slowly. It took her a long time to learn to skip and ride a bike. Neurological examination indicated that rapid finger tapping, opening and closing of the fist, and pronation-supination movements were slow on the right side and accompanied by some mirroring on the left. The family history was significant for learning differences and mood disturbance. Her mother had a history of depression and suspected dyslexia despite no formal diagnosis. A younger sister was undergoing speech therapy for articulation difficulties and was currently awaiting an evaluation for dyslexia.
Concerns about Case 3’s learning first arose toward the end of kindergarten, as she struggled to differentiate the written form of six and nine. In the first grade, teachers observed delay in reading and letter-reversal when writing. Since kindergarten she had received twice weekly tutoring, which proved helpful, and since second grade she has received small group tutoring outside the classroom, focused on reading, writing, and mathematics, for 90 minutes a day, four times a week. This year she began attending a specialized school for children with learning differences. In her spare time, she enjoyed building things and imaginative, pretend play.
Receptive vocabulary was notably in the very superior range (see ), and her mother described her as very verbal. She did occasionally mix up syllables. Her nonverbal reasoning was in the high average range. On standardized tests, real-word reading was poorer than non-word reading, indicating borderline impaired versus low average performance, respectively. Weaknesses in the accuracy and fluency of paragraph reading were observed, with scores in the low average range. In concordance with Cases 1 and 2, on visuospatial testing her judgment of line orientation was impaired. Figure copy was also borderline impaired. By contrast, phonological awareness, memory for words, and rapid automized naming scores were in the average range, with high average performance observed in segmentation and sound blending. She also did well on the real-world test of navigation, learning the route by the seventh trial, and remembering all but one of the turns after the delay.
A number of attentional challenges, including distraction and daydreaming, were reported by her parents. She had difficulty following multi-step instructions and quickly became irritated and frustrated. Her mother reported that she often had difficulty recalling recent events, such as forgetting what they discussed having for dinner over the course of the day, and wondered if this was due to limited attention during the initial conversation. Scores on the Vanderbilt indicated that she continued to meet criteria for an ADHD inattentive subtype diagnosis (see ).
Emotionally, she feared being alone and often wanted someone to be in the room with her as she fell asleep. Parent responses to the BASC-2 questionnaire indicated that she was in the at-risk range on a number of socio-emotional scales, including social skills, functional communication, emotional self-control, and negative emotionality, and in the clinically significant range for withdrawal. She also scores in the at-risk range for a developmental social disorder. She endorsed sensory sensitivity to clothing tags and parent responses to the Sensory Profile indicated a definite difference on all subscales apart from two. During spontaneous speech she evidenced reduced prosodic variation, and overall reduced loudness. Despite these features suggesting a possible autism spectrum disorder, her capacity for back-and-forth communication, and absence of restricted repetitive patterns of behavior or interests, as well as the absence of reported social differences in early childhood, precluded such a diagnosis.
3.1.4 Case 4
A right-handed female, was 9 years old at the time of evaluation. At age seven she received diagnoses of dyslexia, ADHD, and dyspraxia. Developmental differences were first observed in early life, and she received intervention at a feeding clinic for fine motor control and solid feeding difficulties for a year beginning at age two and a half. Her teachers in preschool also noticed that she struggled to hold a pencil, continuing to employ a fisted grasp rather than the more typical finger-based grasps at this age. Her parents believed her difficulties with reading were masked early on because she would memorize the content of books to give the impression that she was reading along and were ultimately indicated by repeated testing over time.
Fine motor challenges continued, and she did not feel coordinated. She received occupational therapy from the age of five, focused on sensory integration, visual processing, and proprioception. Following this intervention she successfully held a pencil correctly, and learnt how to buckle and tie shoes. Her parents reported that messy handwriting and trouble with hand-eye coordination during sports continued. Case 4 had a family history involving both learning differences and psychiatric symptoms. Both her father and sister had dyslexia. Her maternal grandmother developed psychosis and paranoia beginning in her fifties, before committing suicide at the age of 63. Maternal uncles carried diagnoses of schizophrenia and obsessive-compulsive disorder, and possible personality disorder.
Case 4 had received extensive intervention, including occupational therapy as described above, as well as reading therapy once a week, and an intensive summer reading course at age six. She had also attended a specialized school for children with learning differences since second grade. Her parents volunteered that she was easily overwhelmed by new experiences and that when she started school at age four, she would cry for 2 hours every afternoon, due to feeling overwhelmed by the experience. This behavior was ongoing, and she was receiving cognitive-behavioral therapy, with the goal of increasing her emotion regulation abilities in novel circumstances. In line with this, responses to the BASC-2 placed her within the at-risk range for emotional self-control and negative emotionality.
Her mother reported that she had a strong vocabulary, although it could take her some time to organize her thoughts. On testing, her receptive vocabulary and nonverbal reasoning scores were in the average range. Her reading scores, with the exception of Woodcock-Johnson Word Attack, fell in the 16th percentile or below, with the majority below the 10th percentile. Phonological awareness, memory for words, and rapid automized naming, however, were in the average range, with a high average segmentation score. Her parents reported that she was not permitted to go anywhere alone, as they feared she would get lost, and indeed, on the test of real-world navigation, she was not able to learn the route. She obtained a score of 12/15 correct turns by trial 10 but was not able to replicate this or improve further on subsequent trials. However, she did recall 11 of the turns after the delay period, which indicated good retention of what she was able to learn. Figure copy and recall scores were both within the impaired range, suggesting deficits in both encoding and retention of visuospatial information. Judgment of line orientation performance was also in the borderline impaired range.
She reported ongoing sensory sensitivity to rough clothing, and clothing tags, as well as certain food textures. For example, she would not eat yogurt or pudding-type foods. She also experienced headaches after prolonged reading, particularly small font. Parent responses to the Sensory Profile indicated a definite difference in behavioral outcomes of sensory processing, and a probable difference in auditory processing, multisensory processing, emotional and social responses to sensory stimulation, and sensory processing related to endurance and tone. There were no findings on neurological examination. As with the other cases included here, encounters with our multidisciplinary team of evaluators evidenced a typical amount and appropriate use of facial expressions, eye contact, and communicative gestures.
3.2 White matter tractography
Statistical comparison of laterality indexes revealed significantly greater rightward structural asymmetry in the ILF in the atypical cases compared with neurotypical controls [W = 56, p = .022] (). There were no significant differences in structural asymmetry in the remaining pathways (see S1). Regarding tract integrity, the four atypical cases showed significantly lower FA in the left uncinate fasciculus [t (13.44) = 2.71, p = .017] () and significantly greater FA in left [t (10.21) = 4.68, p < .001] and right [t (17.92) = 4.36, p < .001] SLF-TP (). There were no significant differences in tract integrity in the remaining pathways (see S2). Reconstructions of each case’s tracts can be viewed in S3.
Figure 2. The four atypical presentations of dyslexia showed significantly greater structural asymmetry in the inferior longitudinal fasciculus (ILF) relative to neurotypical controls. Groups were matched on age, sex, and handedness. Asterisk denotes significant difference relative to neurotypical controls at *p<.05.
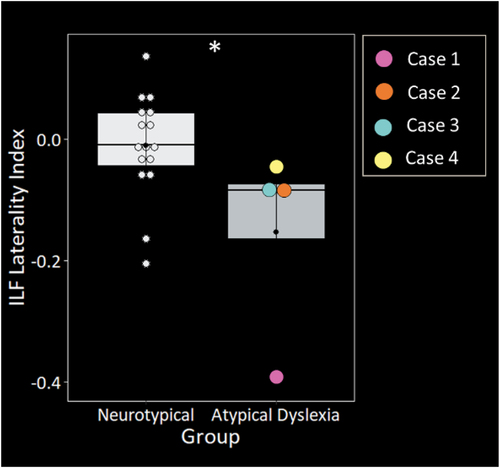
Figure 3. The four atypical presentations of dyslexia showed significantly lower fractional anisotropy (FA) in the left uncinate fasciculus (A), and significantly greater FA bilaterally in the temporoparietal superior longitudinal fasciculus (SLF-TP) (B & C). Groups were matched on age, sex, and handedness. Asterisks denote significant differences relative to neurotypical controls at *p<.05; **p<.001.
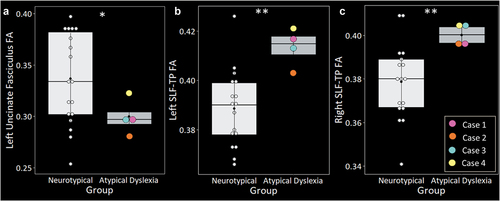
4. Discussion
Here, we present findings from detailed multidisciplinary evaluations of four children with dyslexia. Differences were extensive and not limited to reading. All four of the cases described here carry existing diagnoses of dyslexia and continue to experience severe difficulties in reading, despite extensive remediation. Specifically, all four cases showed reading performance below the fifth percentile on at least one standardized measure and average intelligence or above. In addition, they showed a number of other strengths and difficulties that cross cognitive domains. Testing revealed that all four cases showed performance within the borderline impaired range or below on at least one standardized test of visuospatial processing, socio-emotional problems, and sensory sensitivity. Strikingly, although reading was the primary presenting concern for these children, for three of the four cases, visuospatial performance was poorer than the lowest reading score. Moreover, all cases showed phonological awareness, rapid automized naming, and memory for words within the average to superior range, which stands counter to the notion that these skills are the core underlying deficit(s) in dyslexia. All four cases had developmental concerns prior to the second grade, most frequently in the form of motor delay. This contrasts typical cases of dyslexia, who are not normally identified until after the first few years of formal reading instruction. When early signs are present, they normally take the form of difficulties with speech and language (Richardson et al., Citation2003, Citation2009).
The measure on which we most consistently observed poor performance in these children was judgment of line orientation; this was also where three out of four cases displayed their poorest visuospatial performance, with two cases performing below the lowest percentile. Impairments on this task have been described sporadically in dyslexia (Eden, Stein, et al., Citation1996) while others report visuospatial strengths (Attree et al., Citation2009; Brunswick et al., Citation2010), indicating that there may be subtypes of dyslexia that dissociate in visuospatial ability (Bakker et al., Citation1990), with the children included here representing those with the greatest difficulties. These cases also showed other characteristics that are not part of the diagnostic criteria for dyslexia. All experienced sensory sensitivities, psychomotor difficulties, and socio-emotional challenges, particularly withdrawal, reduced functional communication, and signs of impaired emotion regulation. While these features have been shown to occur to a greater extent in those with learning differences than the general population (Dove & Dunn, Citation2008; Elksnin & Elksnin, Citation2004; Sharfi et al., Citation2015; Taur et al., Citation2014), they have not been extensively studied in dyslexia.
Heterogeneous and atypical presentations present a challenge for single deficit models of dyslexia and are also not fully accounted for by the majority of multiple deficit models either. Some authors have attempted to organize symptoms in neurodevelopmental disorders into primary, secondary, correlated, or artifactual features (Pennington & Ozonoff, Citation1991). It is clear that the additional features described in these cases are not primary to a canonical phonological dyslexia, as many individuals who carry the diagnosis appear not to experience challenges in visuospatial, sensory, socioemotional, or attention domains. What is less clear, however, is whether these represent correlated (arising from the same etiology, but not universal or specific), secondary (arising as a result of primary reading impairment or correlated symptoms), or artifactual (unassociated to primary phenotype) features of dyslexia. Genetic studies have found shared heritability for dyslexia and ADHD, particularly inattentive symptoms (Doust et al., Citation2022; Gialluisi et al., Citation2021), and so this correspondence is known not to be artifactual. The same has yet to be conclusively demonstrated, however, for disorders of affect, visuospatial challenges, and sensory sensitivities, although a shared familial risk for anxiety and dyslexia has been found (Willcutt, Citation2014). Affective challenges are often thought to result from academic struggles (e.g., Carroll & Iles, Citation2006), while others argue that anxiety distracts from learning and interferes with cognitive processes necessary for reading, leading to increased risk for reading disorder (Bryan et al., Citation2004). Evidence for a bidirectional relationship has also been found (Grills-Taquechel et al., Citation2012).
Alterations in white matter organization have previously been associated with dyslexia (although see Meisler & Gabrieli, Citation2022) and the current tractography analyses contribute to an emerging picture of neuroanatomical heterogeneity in dyslexia (Banfi et al., Citation2019; Jednoróg et al., Citation2014; Pecini et al., Citation2011; van Ermingen-Marbach et al., Citation2013). Specifically, compared to neurotypical children, we observed greater rightward asymmetry in volume of the ILF in children with atypical dyslexia, alongside greater FA bilaterally in the SLF-TP, and reduced FA in the left uncinate fasciculus. The uncinate fasciculus contributes to a ventral reading network (Schlaggar & McCandliss, Citation2007), connecting the anterior temporal lobe to the frontal lobe (Catani et al., Citation2002). Disruption in the uncinate fasciculus has been linked to abnormalities in social behavior across neuropsychiatric disorders, including ASD (Kumar et al., Citation2010; Pugliese et al., Citation2009), psychopathy (Craig et al., Citation2009), antisocial personality disorder (Sundram et al., Citation2012), and social anxiety (Baur et al., Citation2013; Phan et al., Citation2009). The ILF is a long-range association tract, connecting occipital cortex and anterior temporal lobes along the ventral visual stream (Catani et al., Citation2003), and has unsurprisingly been implicated in visual perception organization and object recognition (Catani et al., Citation2003; Ortibus et al., Citation2012; Schmahmann & Pandya, Citation2006). In the right hemisphere, it is crucially involved in connecting several regions involved in face processing (e.g., fusiform face area, occipital face area, superior temporal sulcus, and amygdala), and lesions to the right ILF have been associated with face processing impairments (Thomas et al., Citation2009). Alterations in the ILF have previously been associated with visuospatial differences in ASD (Boets et al., Citation2018), and its atypical lateralization in the cases presented here, given their noted visuospatial and social difficulties, is intriguing.
Similarly, alterations in the SLF, particularly elevated FA, have been described in the context of visuospatial differences in Williams syndrome (Hoeft et al., Citation2007), a neurodevelopmental disorder characterized by visuo-constructive challenges alongside relative sparing, and often great interest, in social interaction (Järvinen et al., Citation2013; Martens et al., Citation2008). Several factors may drive increases in FA, including increased myelination, but also decreased axonal diameter, packing density, and branching (Beaulieu, Citation2002). The temporoparietal component of the SLF connects the inferior parietal lobe with the superior temporal gyrus at the temporoparietal junction and is thought to contribute to higher cognitive functions and language comprehension (Bernal & Altman, Citation2010; Catani et al., Citation2005; Schmahmann et al., Citation2007). The SLF-TP has not previously been associated with dyslexia, but it is found to be abnormal in the logopenic variant of primary progressive aphasia (Galantucci et al., Citation2011), which is characterized by word-finding and sentence repetition difficulties and phonological errors in spontaneous speech and on confrontation naming (Gorno‐Tempini et al., Citation2004; Henry & Gorno-Tempini, Citation2010). Future work will need to investigate how the cognitive and language profiles of non-phonological dyslexia relate to alterations in this white matter pathway.
Caution is of course warranted in the interpretation of white matter differences at the single subject level. We identified lateralization differences primarily in one tract, the ILF, but it should be noted that more subtle group differences may also be present. Moreover, while all images were aligned in participants’ native space, and visually checked for quality assurance, DTI is an indirect method of visualizing white matter anatomy, and it is always possible that tracts are incorrectly reconstructed. That being said, the results presented here certainly represent an initial step in understanding the neuroanatomy of non-phonological dyslexia and suggest that it may not be desirable to include dyslexic participants with heterogeneous underlying cognitive profiles alongside one another in group-based neuroimaging studies. Larger studies are now needed to examine these associations more thoroughly, including associations with cognition and behavior.
Executive functions are considered integral to competent reading and many models of reading now incorporate executive functioning components (see Butterfuss & Kendeou, Citation2018, for a review). For example, cognitive control mechanisms such as shifting guide the search for semantic meaning (Graesser et al., Citation1994), and inhibit irrelevant information (Albrecht & O’Brien, Citation1993; Kintsch, Citation1988), while working memory supports the retention of narrative (Trabasso et al., Citation1989). Moreover, executive functions including selective and sustained attention, inhibition, and visuospatial short-term memory are impaired in young children at risk for dyslexia (Gooch et al., Citation2014) and predict future reading ability (Thompson et al., Citation2015). Brain regions associated with executive functions are also associated with reading ability. Based on these findings, it has been suggested that executive functioning impairments may precede reading disability in children with dyslexia (Farah et al., Citation2021). The majority of this work has considered relatively “pure” cases of dyslexia and excluded those with co-occurring challenges; future longitudinal work should therefore explore the developmental time-course of language and non-language-based differences in children presenting with more complex profiles of strength and weakness, in order to better understand the relationships between dyslexia and non-reading difficulties, and associated neuroanatomy.
The children described here share some features with ASD. A diagnosis of ASD requires both social communication difficulties and restrictive, repetitive patterns of behavior (American Psychiatric Association, Citation2013). This is often manifested as sensory differences, an insistence on sameness, reduced nonverbal communicative behavior such as eye contact, and a difficulty with sharing affective states. Similarly, all of the children described here show definite sensory sensitivities, and three show functional communication impairments. Only the male case in this series shows motor stereotypies (Case 1), and ASD prevalence estimates do show that repetitive behavior is more common in males than females (Hattier et al., Citation2011). The socio-emotional differences these children experience appear to be milder than those seen in ASD, with normal eye contact, use of gesture, and facial expression, and perhaps increased social sensitivity rather than too little. Only one case (Case 3) scored in the at-risk range for a developmental social disorder on the BASC-2, a subscale that was designed to reflect the DSM-IV-TR (American Psychiatric Association, Citation2000) diagnostic criteria for autistic disorder and Asperger’s disorder. Other features described here, such as executive functioning impairments, are not specific to ASD, and are seen across many neurodevelopmental conditions. For an ASD diagnosis to be given, symptoms, including social communication differences, must be present in early life, which was not reported in these children. Instead, early concerns centered on psychomotor delay. Other subtle observations, such as an enjoyment of imaginative play (Case 3) and an absence of restricted interests, are also problematic for an ASD diagnosis.
We have seen a total of 37 out of 270 children at the UCSF Dyslexia Center during the 6-year period of recruitment for this report that presented with symptoms affecting the domains described here. This population is, however, heterogeneous, and not all show the exact same profile of strengths and weaknesses. Indeed, the four cases described here are not identical. While they demonstrated a number of common features, mathematical ability, and divided attention and visuomotor tracking as measured with the Colored Trails test, were variable. Earlier and more accurate identification of these children may help structure interventions that are better tailored to their overall learning profiles, and not just the elements that are most readily identified in academic settings. Reading disability had already been identified in all four children, and inattention in two out of three, but only one had a diagnosis of an affective disorder. The interventions they were currently receiving might help to remediate their reading disability, but this may leave visuospatial skills, sensory modulation, and emotion regulation abilities vulnerable to continued divergence. Challenges with feeding themselves and holding writing implements were among the first noted concerns for several. Greater screening, based on these characteristics, may be needed in children showing early developmental differences. Similarly, research on how these children change with development, and whether they are at greater risk of affective disorders compared to typical phonological dyslexia, is needed. A diagnosis of dyslexia only captures reading disability, and not the visuospatial, socioemotional, attentional, and sensory differences these children experience. Providing multiple diagnostic labels, while perhaps capturing the majority of symptoms, does little to elucidate how these symptoms relate to each other or map how they should be comprehensively remediated. The mechanisms underlying these diffuse symptoms likely intertwine and overlap. The more completely we understand these learners, with their widely disparate abilities and weaknesses, the better we will be able to intervene. The vulnerability of these children to socioemotional challenges, whether reflecting the heightened burden of impairment across multiple domains, or a primary feature of the phenotype, suggests tailored interventions are greatly needed. Increasing awareness of the associated features of dyslexia will also hopefully improve identification; the observation of a delay in learning to read should not preclude investigation of other skills.
NCS-OA_22-141-File002.docx
Download MS Word (889.4 KB)Acknowledgements
We thank the children and their families for participating in our research and our funding sources for their support (see Funding for details).
Disclosure statement
No potential conflict of interest was reported by the author(s).
Data availability statement
The conditions of our ethics approval do not permit public archiving of anonymized study data. Data generated by the UCSF Dyslexia Center are available upon request. Data requests can be submitted through the UCSF MAC Resource Request form: http://memory.ucsf.edu/resources/data. Access will be granted to named individuals in accordance with ethical procedures governing the reuse of sensitive data. All requests will undergo UCSF regulated procedure thus require submission of a Material Transfer Agreement (MTA) which can be found at http://memory.ucsf.edu/resources/data. No commercial use would be approved.
Supplementary material
Supplemental data for this article can be accessed online at https://doi.org/10.1080/13554794.2022.2145905
Additional information
Funding
References
- Albrecht, J. E., & O’Brien, E. J. (1993). Updating a mental model: Maintaining both local and global coherence. Journal of Experimental Psychology: Learning, Memory, and Cognition, 19(5), 1061. https://doi.org/10.1037/0278-7393.19.5.1061
- American Psychiatric Association. (2000). Diagnostic and Statistical Manual of mental Disorders (4thedition ed.). American Psychiatric Association. [ Text Revision (DSM-IV-TR)].
- American Psychiatric Association. (2013). Diagnostic and statistical manual of mental disorders (5th ed.). Author.
- Attree, E. A., Turner, M. J., & Cowell, N. (2009). A virtual reality test identifies the visuospatial strengths of adolescents with dyslexia. CyberPsychology & Behavior, 12(2), 163–168. https://doi.org/10.1089/cpb.2008.0204
- Badian, N. A. (1997). Dyslexia and the double deficit hypothesis. Annals of Dyslexia, 47(1), 69–87. https://doi.org/10.1007/s11881-997-0021-y
- Bakker, D. J., Bouma, A., & Gardien, C. J. (1990). Hemisphere-specific treatment of dyslexia subtypes: A field experiment. Journal of Learning Disabilities, 23(7), 433–438. https://doi.org/10.1177/002221949002300707
- Banfi, C., Koschutnig, K., Moll, K., Schulte‐körne, G., Fink, A., & Landerl, K. (2019). White matter alterations and tract lateralization in children with dyslexia and isolated spelling deficits. Human brain mapping, 40(3), 765–776. https://doi.org/10.1002/hbm.24410
- Baur, V., Brühl, A. B., Herwig, U., Eberle, T., Rufer, M., Delsignore, A., Hänggi, J. (2013). Evidence of frontotemporal structural hypoconnectivity in social anxiety disorder: A quantitative fiber tractography study. Human brain mapping, 34(2), 437–446.
- Beaulieu, C. (2002). The basis of anisotropic water diffusion in the nervous system—a technical review. NMR in Biomedicine, 15(7–8), 435–455. https://doi.org/10.1002/nbm.782
- Beery, K. E., & Beery, N. A. (2010). The Beery-Buktenica Developmental Test of Visual–Motor Integration: Administration, scoring, and teaching manual (6th ed.). Pearson.
- Benton, A., Hannay, H. J., & Varney, N. R. (1975). Visual perception of line direction in patients with unilateral brain disease. Neurology, 25(10), 907. https://doi.org/10.1212/WNL.25.10.907
- Bernal, B., & Altman, N. (2010). The connectivity of the superior longitudinal fasciculus: A tractography DTI study. Magnetic resonance imaging, 28(2), 217–225. https://doi.org/10.1016/j.mri.2009.07.008
- Berninger, V. W., Abbott, R. D., Thomson, J., Wagner, R., Swanson, H. L., Wijsman, E. M., & Raskind, W. (2006). Modeling phonological core deficits within a working memory architecture in children and adults with developmental dyslexia. Scientific Studies of Reading, 10(2), 165–198. https://doi.org/10.1207/s1532799xssr1002_3
- Bishop, D. V., & Snowling, M. J. (2004). Developmental dyslexia and specific language impairment: Same or different? Psychological Bulletin, 130(6), 858. https://doi.org/10.1037/0033-2909.130.6.858
- Boets, B., Van Eylen, L., Sitek, K., Moors, P., Noens, I., Steyaert, J., Wagemans, J. (2018). Alterations in the inferior longitudinal fasciculus in autism and associations with visual processing: A diffusion-weighted MRI study. Molecular autism, 9(1), 1–14. https://doi.org/10.1186/s13229-018-0188-6
- Bosse, M. L., Tainturier, M. J., & Valdois, S. (2007). Developmental dyslexia: The visual attention span deficit hypothesis. Cognition, 104(2), 198–230. https://doi.org/10.1016/j.cognition.2006.05.009
- Brunswick, N., Martin, G. N., & Marzano, L. (2010). Visuospatial superiority in developmental dyslexia: Myth or reality? Learning and Individual Differences, 20(5), 421–426. https://doi.org/10.1016/j.lindif.2010.04.007
- Bryan, T., Burstein, K., & Ergul, C. (2004). The social-emotional side of learning disabilities: A science-based presentation of the state of the art. Learning Disability Quarterly, 27(1), 45–51. https://doi.org/10.2307/1593631
- Butterfuss, R., & Kendeou, P. (2018). The role of executive functions in reading comprehension. Educational Psychology Review, 30(3), 801–826. https://doi.org/10.1007/s10648-017-9422-6
- Carroll, J. M., & Iles, J. E. (2006). An assessment of anxiety levels in dyslexic students in higher education. The British Journal of Educational Psychology, 76(3), 651–662. https://doi.org/10.1348/000709905X66233
- Catani, M., Howard, R. J., Pajevic, S., & Jones, D. K. (2002). Virtual in vivo interactive dissection of white matter fasciculi in the human brain. Neuroimage, 17(1), 77–94. https://doi.org/10.1006/nimg.2002.1136
- Catani, M., Jones, D. K., Donato, R., & Ffytche, D. H. (2003). Occipito‐temporal connections in the human brain. Brain, 126(9), 2093–2107. https://doi.org/10.1093/brain/awg203
- Catani, M., Jones, D. K., & Ffytche, D. H. (2005). Perisylvian language networks of the human brain. Annals of Neurology, 57(1), 8–16. https://doi.org/10.1002/ana.20319
- Caverzasi, E., Mandelli, M. L., Hoeft, F., Watson, C., Meyer, M., Allen, I. E., Mukherjee, P., Gandini Wheeler-Kingshott, C. A. M., Marco, E. J., Mukherjee, P., Miller, Z. A., Miller, B. L., Hendren, R., Shapiro, K. A., Gorno-Tempini, M. L., & Papinutto, N. (2018). Abnormal age-related cortical folding and neurite morphology in children with developmental dyslexia. NeuroImage: Clinical, 18, 814–821. https://doi.org/10.1016/j.nicl.2018.03.012
- Craig, M. C., Catani, M., Deeley, Q., Latham, R., Daly, E., Kanaan, R., Murphy, D. G. (2009). Altered connections on the road to psychopathy. Molecular psychiatry, 14(10), 946–953. https://doi.org/10.1038/mp.2009.40
- Cui, Z., Xia, Z., Su, M., Shu, H., & Gong, G. (2016). Disrupted white matter connectivity underlying developmental dyslexia: A machine learning approach. Human brain mapping, 37(4), 1443–1458. https://doi.org/10.1002/hbm.23112
- De Luca, M., Borrelli, M., Judica, A., Spinelli, D., & Zoccolotti, P. (2002). Reading words and pseudowords: An eye movement study of developmental dyslexia. Brain and Language, 80(3), 617–626. https://doi.org/10.1006/brln.2001.2637
- Delude, C. M. (2015). Deep phenotyping: The details of disease. Nature, 527(S14–S15), S14–15. https://doi.org/10.1038/527S14a
- DiBetta, A. M., & Romani, C. (2006). Lexical learning and dysgraphia in a group of adults with developmental dyslexia. Cognitive neuropsychology, 23(3), 376–400. https://doi.org/10.1080/02643290442000545
- DiFolco, C., Guez, A., Peyre, H., & Ramus, F. (2022). Epidemiology of reading disability: A comparison of DSM-5 and ICD-11 criteria. Scientific Studies of Reading, 26(4), 337–355. https://doi.org/10.1080/10888438.2021.1998067
- Doust, C., Fontanillas, P., Eising, E., Gordon, S. D., Wang, Z., Alagöz, G., Molz, B., 23andMe Research Team, Quantitative Trait Working Group of the GenLang Consortium, Pourcain, B. S., Francks, C., Marioni, R. E., Zhao, J., Paracchini, S., Talcott, J. B., Monaco, A. P., Stein, J. F., Gruen, J. R., Olson, R. K., Luciano, M. (2022). Discovery of 42 Genome-Wide Significant Loci Associated with Dyslexia. Nature Genetics, 54(11), 1621–1629. https://doi.org/10.1038/s41588-022-01192-y
- Dove, S., & Dunn, W. (2008). Sensory processing in students with specific learning disabilities: Findings and implications for assessment and intervention planning. Journal of Occupational Therapy, Schools, & Early Intervention, 1(2), 116–127. https://doi.org/10.1080/19411240802312798
- Dunn, W. (1999). Sensory Profile. The Psychological Corporation.
- Eden, G. F., Stein, J. F., Wood, H. M., & Wood, F. B. (1996). Differences in visuospatial judgement in reading-disabled and normal children. Perceptual and Motor Skills, 82(1), 155–177. https://doi.org/10.2466/pms.1996.82.1.155
- Elksnin, L. K., & Elksnin, N. (2004). The social-emotional side of learning disabilities. Learning Disability Quarterly, 27(1), 3–8. https://doi.org/10.2307/1593627
- Ellis, A. W. (1985). The cognitive neuropsychology of developmental (and acquired) dyslexia: A critical survey. Cognitive neuropsychology, 2(2), 169–205. https://doi.org/10.1080/02643298508252865
- Facoetti, A., Lorusso, M. L., Cattaneo, C., Galli, R., & Molteni, M. (2005). Visual and auditory attentional capture are both sluggish in children with developmental dyslexia. Acta neurobiologiae experimentalis, 65(1), 61–72.
- Farah, R., Ionta, S., & Horowitz-Kraus, T. (2021). Neuro-behavioral correlates of executive dysfunctions in dyslexia over development from childhood to adulthood. Frontiers in Psychology, 3236.
- Fiez, J. A., & Petersen, S. E. (1998). Neuroimaging studies of word reading. Proceedings of the National Academy of Sciences, 95(3), 914–921. https://doi.org/10.1073/pnas.95.3.914
- Finn, E. S., Shen, X., Holahan, J. M., Scheinost, D., Lacadie, C., Papademetris, X., Constable, R. T. (2014). Disruption of functional networks in dyslexia: A whole-brain, data-driven analysis of connectivity. Biological psychiatry, 76(5), 397–404. https://doi.org/10.1016/j.biopsych.2013.08.031
- Galantucci, S., Tartaglia, M. C., Wilson, S. M., Henry, M. L., Filippi, M., Agosta, F., Gorno-Tempini, M. L. (2011). White matter damage in primary progressive aphasias: A diffusion tensor tractography study. Brain, 134(10), 3011–3029. https://doi.org/10.1093/brain/awr099
- Germanò, E., Gagliano, A., & Curatolo, P. (2010). Comorbidity of ADHD and dyslexia. Developmental Neuropsychology, 35(5), 475–493. https://doi.org/10.1080/87565641.2010.494748
- Gialluisi, A., Andlauer, T. F., Mirza-Schreiber, N., Moll, K., Becker, J., Hoffmann, P., Ludwig, K. Schulte-Körne, G. (2021). Genome-wide association study reveals new insights into the heritability and genetic correlates of developmental dyslexia. Molecular psychiatry, 26(7), 3004–3017. https://doi.org/10.1038/s41380-020-00898-x
- Gooch, D., Hulme, C., Nash, H. M., & Snowling, M. J. (2014). Comorbidities in preschool children at family risk of dyslexia. Journal of Child Psychology and Psychiatry, 55(3), 237–246. https://doi.org/10.1111/jcpp.12139
- Gorno‐tempini, M. L., Dronkers, N. F., Rankin, K. P., Ogar, J. M., Phengrasamy, L., Rosen, H. J., Miller, B. L. (2004). Cognition and anatomy in three variants of primary progressive aphasia. Annals of Neurology: Official Journal of the American Neurological Association and the Child Neurology Society, 55(3), 335–346. https://doi.org/10.1002/ana.10825
- Goswami, U. (2011). A temporal sampling framework for developmental dyslexia. Trends in cognitive sciences, 15(1), 3–10. https://doi.org/10.1016/j.tics.2010.10.001
- Graesser, A. C., Singer, M., & Trabasso, T. (1994). Constructing inferences during narrative text comprehension. Psychological Review, 101(3), 371. https://doi.org/10.1037/0033-295X.101.3.371
- Grills-Taquechel, A. E., Fletcher, J. M., Vaughn, S. R., & Stuebing, K. K. (2012). Anxiety and reading difficulties in early elementary school: Evidence for unidirectional-or bi-directional relations? Child Psychiatry & Human Development, 43(1), 35–47. https://doi.org/10.1007/s10578-011-0246-1
- Hattier, M. A., Matson, J. L., Tureck, K., & Horovitz, M. (2011). The effects of gender and age on repetitive and/or restricted behaviors and interests in adults with autism spectrum disorders and intellectual disability. Research in Developmental Disabilities, 32(6), 2346–2351. https://doi.org/10.1016/j.ridd.2011.07.028
- Hendren, R. L., Haft, S. L., Black, J. M., White, N. C., & Hoeft, F. (2018). Recognizing psychiatric comorbidity with reading disorders. Frontiers in Psychiatry, 101. https://doi.org/10.3389/fpsyt.2018.00101
- Henry, M. L., & Gorno-Tempini, M. L. (2010). The logopenic variant of primary progressive aphasia. Current Opinion in Neurology, 23(6), 633. https://doi.org/10.1097/WCO.0b013e32833fb93e
- Ho, C. S. H., Chan, D. W. O., Tsang, S. M., & Lee, S. H. (2002). The cognitive profile and multiple-deficit hypothesis in Chinese developmental dyslexia. Developmental Psychology, 38(4), 543. https://doi.org/10.1037/0012-1649.38.4.543
- Hoeft, F., Barnea-Goraly, N., Haas, B. W., Golarai, G., Ng, D., Mills, D., Reiss, A. L. (2007). More is not always better: Increased fractional anisotropy of superior longitudinal fasciculus associated with poor visuospatial abilities in Williams syndrome. Journal of Neuroscience, 27(44), 11960–11965. https://doi.org/10.1523/JNEUROSCI.3591-07.2007
- Järvinen, A., Korenberg, J. R., & Bellugi, U. (2013). The social phenotype of Williams syndrome. Current Opinion in Neurobiology, 23(3), 414–422. https://doi.org/10.1016/j.conb.2012.12.006
- Jednoróg, K., Gawron, N., Marchewka, A., Heim, S., & Grabowska, A. (2014). Cognitive subtypes of dyslexia are characterized by distinct patterns of grey matter volume. Brain structure & function, 219(5), 1697–1707. https://doi.org/10.1007/s00429-013-0595-6
- Jordan, K. M., Lauricella, M., Licata, A. E., Sacco, S., Asteggiano, C., Wang, C., Sudarsan, S. P., Watson, C., Scheffler, A. W., Battistella, G., Miller, Z. A., Gorno‐tempini, M. L., Caverzasi, E., & Mandelli, M. L. (2021). Cortically constrained shape recognition: Automated white matter tract segmentation validated in the pediatric brain. Journal of Neuroimaging, 31(4), 758–772. https://doi.org/10.1111/jon.12854
- Kadesjö, B., & Gillberg, C. (2002). Developmental coordination disorder in Swedish 7-year-old children. In Hertzig, M. E., Farber, E. A. (eds), Annual Progress in Child Psychiatry and Child Development 2000-2001 (pp. 317–334). Routledge.
- Kamali, A., Flanders, A. E., Brody, J., Hunter, J. V., & Hasan, K. M. (2014). Tracing superior longitudinal fasciculus connectivity in the human brain using high resolution diffusion tensor tractography. Brain structure & function, 219(1), 269–281. https://doi.org/10.1007/s00429-012-0498-y
- Kintsch, W. (1988). The role of knowledge in discourse comprehension: A construction-integration model. Psychological Review, 95(2), 163. https://doi.org/10.1037/0033-295X.95.2.163
- Korkman, M., Kirk, U., & Kemp, S. (2007). NEPSY II: Clinical and interpretive manual. Harcourt Assessment, PsychCorp.
- Kumar, A., Sundaram, S. K., Sivaswamy, L., Behen, M. E., Makki, M. I., Ager, J., Janisse, J. Chugani, H. T., & Chugani, D. C. (2010). Alterations in frontal lobe tracts and corpus callosum in young children with autism spectrum disorder. Cerebral Cortex, 20(9), 2103–2113. https://doi.org/10.1093/cercor/bhp278
- Lyon, G. R., Shaywitz, S. E., & Shaywitz, B. A. (2003). A definition of dyslexia. Annals of Dyslexia, 53(1), 1–14. https://doi.org/10.1007/s11881-003-0001-9
- Martens, M. A., Wilson, S. J., & Reutens, D. C. (2008). Research Review: Williams syndrome: A critical review of the cognitive, behavioral, and neuroanatomical phenotype. Journal of Child Psychology and Psychiatry, 49(6), 576–608. https://doi.org/10.1111/j.1469-7610.2008.01887.x
- Martin, R. C. (1995). Heterogeneity of deficits in developmental dyslexia and implications for methodology. Psychonomic Bulletin & Review, 2(4), 494–500. https://doi.org/10.3758/BF03210984
- Martin, N. A., & Brownell, R. (2011). Receptive one-word picture vocabulary test. Academic Therapy Publications.
- McArthur, G. M., Hogben, J. H., Edwards, V. T., Heath, S. M., & Mengler, E. D. (2000). On the “specifics” of specific reading disability and specific language impairment. Journal of Child Psychology and Psychiatry, and Allied Disciplines, 41(7), 869–874. https://doi.org/10.1111/1469-7610.00674
- McCloskey, M., & Rapp, B. (2000). A visually based developmental reading deficit. Journal of Memory and Language, 43(2), 157–181. https://doi.org/10.1006/jmla.2000.2724
- Meisler, S. L., & Gabrieli, J. D. (2022). A large-scale investigation of white matter microstructural associations with reading ability. Neuroimage, 249, 118909. https://doi.org/10.1016/j.neuroimage.2022.118909
- Ortibus, E. L. S., Verhoeven, J., Sunaert, S., Casteels, I., De Cock, P., & Lagae, L. (2012). Integrity of the inferior longitudinal fasciculus and impaired object recognition in children: A diffusion tensor imaging study. Developmental Medicine & Child Neurology, 54(1), 38–43. https://doi.org/10.1111/j.1469-8749.2011.04147.x
- Osterrieth, P. A. (1944). Le test de copie d’une figure complexe. Archives de psychologie, 30, 206–356.
- Pecini, C., Biagi, L., Brizzolara, D., Cipriani, P., DiLieto, M. C., Guzzetta, A., Toestti, M. & Chilosi, A. M. (2011). How many functional brains in developmental dyslexia? When the history of language delay makes the difference. Cognitive and Behavioral Neurology, 24(2), 85–92. https://doi.org/10.1097/WNN.0b013e318222a4c2
- Pennington, B. F. (2006). From single to multiple deficit models of developmental disorders. Cognition, 101(2), 385–413. https://doi.org/10.1016/j.cognition.2006.04.008
- Pennington, B. F., & Ozonoff, S. (1991). Neuroscientific perspective on continuity and discontinuity in developmental psychopathology. In D. Cicchetti & S. L. Toth (Eds.), Models and Integrations (pp. 17–130). University of Rochester Press.
- Pennington, B. F., Santerre Lemmon, L., Rosenberg, J., MacDonald, B., Boada, R., Friend, A., Leopold, D. R. Samuelsson, S., Byrne, B., Wilcutt, E. G., & Olson, R. K. (2012). Individual prediction of dyslexia by single versus multiple deficit models. Journal of Abnormal Psychology, 121(1), 212. https://doi.org/10.1037/a0025823
- Phan, K. L., Orlichenko, A., Boyd, E., Angstadt, M., Coccaro, E. F., Liberzon, I., & Arfanakis, K. (2009). Preliminary evidence of white matter abnormality in the uncinate fasciculus in generalized social anxiety disorder. Biological psychiatry, 66(7), 691–694. https://doi.org/10.1016/j.biopsych.2009.02.028
- Psychological Corporation. (1999). Wechsler Abbreviated Scale of Intelligence. Author.
- Pugliese, L., Catani, M., Ameis, S., Dell’Acqua, F., de Schotten, M. T., Murphy, C., Robertson, D. Deeley, Q., Daly, E., Murphy, D. G. M. (2009). The anatomy of extended limbic pathways in Asperger syndrome: A preliminary diffusion tensor imaging tractography study. Neuroimage, 47(2), 427–434. https://doi.org/10.1016/j.neuroimage.2009.05.014
- Ramus, F., Altarelli, I., Jednoróg, K., Zhao, J., & DiCovella, L. S. (2018). Neuroanatomy of developmental dyslexia: Pitfalls and promise. Neuroscience & Biobehavioral Reviews, 84, 434–452. https://doi.org/10.1016/j.neubiorev.2017.08.001
- Ramus, F., Rosen, S., Dakin, S. C., Day, B. L., Castellote, J. M., White, S., & Frith, U. (2003). Theories of developmental dyslexia: Insights from a multiple case study of dyslexic adults. Brain, 126(4), 841–865. https://doi.org/10.1093/brain/awg076
- Reid, A. A., Szczerbinski, M., Iskierka‐kasperek, E., & Hansen, P. (2007). Cognitive profiles of adult developmental dyslexics: Theoretical implications. Dyslexia, 13(1), 1–24. https://doi.org/10.1002/dys.321
- Reynolds, C. R., & Kamphaus, R. W. (2004). BASC-2 behavioral assessment system for children manual (2nd ed.). AGS Publishing, Circle Pines, MN.
- Richardson, U., Kulju, P., Nieminen, L., & Torvelainen, P. (2009). Early signs of dyslexia from the speech and language processing of children. International Journal of Speech-Language Pathology, 11(5), 366–380. https://doi.org/10.1080/17549500903147545
- Richardson, U., Leppänen, P. H., Leiwo, M., & Lyytinen, H. (2003). Speech perception of infants with high familial risk for dyslexia differ at the age of 6 months. Developmental Neuropsychology, 23(3), 385–397. https://doi.org/10.1207/S15326942DN2303_5
- Rimrodt, S. L., Peterson, D. J., Denckla, M. B., Kaufmann, W. E., & Cutting, L. E. (2010). White matter microstructural differences linked to left perisylvian language network in children with dyslexia. Cortex, 46(6), 739–749. https://doi.org/10.1016/j.cortex.2009.07.008
- Rochelle, K. S., & Talcott, J. B. (2006). Impaired balance in developmental dyslexia? A meta‐analysis of the contending evidence. Journal of Child Psychology and Psychiatry, 47(11), 1159–1166. https://doi.org/10.1111/j.1469-7610.2006.01641.x
- Saksida, A., Iannuzzi, S., Bogliotti, C., Chaix, Y., Démonet, J. F., Bricout, L., Billard, C., Nguyen-Morel, M. -A., Le Heuzey, M. -F., Soares-Boucaud, I., George, F., Ziegler, J. C., & Ramus, F. (2016). Phonological skills, visual attention span, and visual stress in developmental dyslexia. Developmental Psychology, 52(10), 1503. https://doi.org/10.1037/dev0000184
- Schlaggar, B. L., & McCandliss, B. D. (2007). Development of neural systems for reading. Annual Review of Neuroscience, 30(1), 475–503. https://doi.org/10.1146/annurev.neuro.28.061604.135645
- Schmahmann, J. D., & Pandya, D. N. (2006). Fiber Pathways of the Brain. Oxford University Press.
- Schmahmann, J. D., Pandya, D. N., Wang, R., Dai, G., D’Arceuil, H. E., De Crespigny, A. J., & Wedeen, V. J. (2007). Association fibre pathways of the brain: Parallel observations from diffusion spectrum imaging and autoradiography. Brain, 130(3), 630–653. https://doi.org/10.1093/brain/awl359
- Schrank, F. A., Mather, N., & McGrew, K. S. (2014). Woodcock-Johnson IV Tests of Achievement. Riverside.
- Sharfi, K., Rosenblum, S., & Brattico, E. (2015). Sensory modulation and sleep quality among adults with learning disabilities: A quasi-experimental case-control design study. Plos One, 10(2), e0115518. https://doi.org/10.1371/journal.pone.0115518
- Silani, G., Frith, U., Demonet, J. F., Fazio, F., Perani, D., Price, C., Frith, C. D., & Paulesu, E. (2005). Brain abnormalities underlying altered activation in dyslexia: A voxel based morphometry study. Brain, 128(10), 2453–2461. https://doi.org/10.1093/brain/awh579
- Snowling, M. J. (2012). Changing concepts of dyslexia: Nature, treatment and comorbidity [Editorial]. Journal of Child Psychology and Psychiatry, 53(9), e1–3. https://doi.org/10.1111/j.1469-7610.2009.02197.x
- Stanovich, K. E. (1988). Explaining the differences between the dyslexic and the garden-variety poor reader: The phonological-core variable-difference model. Journal of Learning Disabilities, 21(10), 590–604. https://doi.org/10.1177/002221948802101003
- Sundram, F., Deeley, Q., Sarkar, S., Daly, E., Latham, R., Craig, M., Raczek, M. Fahy, T., & Picchioni, M. (2012). White matter microstructural abnormalities in the frontal lobe of adults with antisocial personality disorder. Cortex, 48(2), 216–229. https://doi.org/10.1016/j.cortex.2011.06.005
- Swan, D., & Goswami, U. (1997). Phonological awareness deficits in developmental dyslexia and the phonological representations hypothesis. Journal of Experimental Child Psychology, 66(1), 18–41. https://doi.org/10.1006/jecp.1997.2375
- Taur, S., Karande, S., Saxena, A. A., Gogtay, N. J., & Thatte, U. M. (2014). Use of computerized tests to evaluate psychomotor performance in children with specific learning disabilities in comparison to normal children. The Indian Journal of Medical Research, 140(5), 644.
- Thomas, C., Avidan, G., Humphreys, K., Jung, K. J., Gao, F., & Behrmann, M. (2009). Reduced structural connectivity in ventral visual cortex in congenital prosopagnosia. Nature neuroscience, 12(1), 29–31. https://doi.org/10.1038/nn.2224
- Thompson, P. A., Hulme, C., Nash, H. M., Gooch, D., Hayiou‐thomas, E., & Snowling, M. J. (2015). Developmental dyslexia: Predicting individual risk. Journal of Child Psychology and Psychiatry, 56(9), 976–987. https://doi.org/10.1111/jcpp.12412
- Torgesen, J. K., Wagner, R., & Rashotte, C. (2012). Test of word reading efficiency–second edition (TOWRE-2). AGS.
- Trabasso, T., Van den Broek, P., & Suh, S. Y. (1989). Logical necessity and transitivity of causal relations in stories. Discourse processes, 12(1), 1–25. https://doi.org/10.1080/01638538909544717
- Turkeltaub, P. E., Eden, G. F., Jones, K. M., & Zeffiro, T. A. (2002). Meta-analysis of the functional neuroanatomy of single-word reading: Method and validation. Neuroimage, 16(3), 765–780. https://doi.org/10.1006/nimg.2002.1131
- Vandermosten, M., Boets, B., Poelmans, H., Sunaert, S., Wouters, J., & Ghesquiere, P. (2012). A tractography study in dyslexia: Neuroanatomic correlates of orthographic, phonological and speech processing. Brain, 135(3), 935–948. https://doi.org/10.1093/brain/awr363
- van Ermingen-Marbach, M., Grande, M., Pape-Neumann, J., Sass, K., & Heim, S. (2013). Distinct neural signatures of cognitive subtypes of dyslexia with and without phonological deficits. NeuroImage: Clinical, 2, 477–490. https://doi.org/10.1016/j.nicl.2013.03.010
- Watson, C., & Willows, D. M. (1993). Evidence for a visual-processing-deficit subtype among disabled readers. In Willowss, D. M., Corcos, E., Kruk, R. S. (Eds), Visual Processes in Reading and Reading Disabilities, Erlbaum Hillsdale, NJ: pp. 287–309.
- Wechsler, D., Kaplan, E., Fein, D., Kramer, J., Morris, R., Delis, D., & Maerlender, A. (2004). Wechsler Intelligence Scale for Children-Integrated. In San Antonio. The Psychological Corporation.
- White, S., Milne, E., Rosen, S., Hansen, P., Swettenham, J., Frith, U., & Ramus, F. (2006). The role of sensorimotor impairments in dyslexia: A multiple case study of dyslexic children. Developmental science, 9(3), 237–255. https://doi.org/10.1111/j.1467-7687.2006.00483.x
- Wiederholt, J. L., & Bryant, B. R. (2012). Gray oral reading test: Examiner’s manual (5th ed.). Pro-Ed.
- Willcutt, E. G. (2014) Behavioral genetic approaches to understand the etiology of comorbidity. In Rhee, S., Ronald, A. (eds), Behavior Genetics of Psychopathology Springer: pp. 231–252.
- Williams, J., Rickert, V., Hogan, J., Zolten, A. J., Satz, P., D’Elia, L. F., Asarnow, R. F., Zaucha, K., & Light, R. (1995). Children’s color trails. Archives of Clinical Neuropsychology, 10(3), 211–223. https://doi.org/10.1093/arclin/10.3.211
- Wolf, M., & Bowers, P. G. (1999). The double-deficit hypothesis for the developmental dyslexias. Journal of Educational Psychology, 91(3), 415. https://doi.org/10.1037/0022-0663.91.3.415
- Wolff, P. H., Michel, G. F., & Ovrut, M. (1990). Rate variables and automatized naming in developmental dyslexia. Brain and Language, 39(4), 556–575. https://doi.org/10.1016/0093-934X(90)90162-A
- Wolraich, M. L., Lambert, W., Doffing, M. A., Bickman, L., Simmons, T., & Worley, K. (2003). Psychometric properties of the Vanderbilt ADHD diagnostic parent rating scale in a referred population. Journal of Pediatric Psychology, 28(8), 559–568. https://doi.org/10.1093/jpepsy/jsg046