ABSTRACT
Understanding the socio-psychological impact of living in high-rise, densely populated urban areas is essential for evaluating the quality of interior urban spaces within vertical urbanism, a field commonly known as ‘Urbanology’. This area combines urban psychology, anthropology and social science, often utilizing empirical methods such as surveys and interviews. This research offers scientific research methods that utilize electroencephalogram (EEG) and an artificial intelligence (AI) generator to establish a system and formula for accessing neuroscientific datasets. The goal is to establish a connection between emotions and the spatial conditions of urban environments.
Introduction
In order to comprehend the quality of urban space in terms of users’ cognitive and emotional responses, it is crucial for urban planners, designers, and architects to recognize that each user’s experience is distinctive and personal. It is important to note that the uniqueness of each experience is partly attributed to the fact that cognition itself varies from person to person.
In this research, two primary aspects are focused on. Firstly, it explores methods for measuring human emotions to evaluate the quality of built environments and assess users’ needs, demands and satisfaction. Secondly, it aims to develop a system and formula for accessing neuroscientific data sets, allowing for the design language that connects users’ emotions with spatial urban conditions.
Various neuroscientific perspectives have been utilized in the research methodology to comprehend the responses of users to urban areas and spatial typologies in densely populated and vertical sites in Hong Kong, encompassing both positive and negative reactions. The study specifically examines multi-layered interior public spaces at the meso-scale within the context of the high-rise and densely populated urban environment. Hong Kong is selected as a focus area for this study of layered, multi-level public and quasi-public realm of interior spaces, serviced by covered walkways due to the compact, hilly terrain and tropical climate in Hong Kong that inspires this requirement for a connective tissue between buildings that is stepped rather than flat and enclosed or covered.
Overview of cognitive psychology and human–computer interaction (HCI)
One of the first noteworthy publications of the topic was Simmel’s 1903 essay The Metropolis and Mental Life. In Citation1967, Ulric Neisser coined the term ‘cognitive psychology’. Simultaneously, the pioneering architect Christopher Alexander et al. (Citation1977) began to develop an urban and architectural design coding system and ‘pattern language’ intended to cater for the complex phenomena of human life by referencing patterns within both nature and the built environment. This field of study has since yielded numerous interventions that have proven beneficial not only to psychology but also to various other disciplines. This research includes Stanley Milgram’s focus on the psychology of city life in The Individual in a Social World (Citation1977), Peter Walker offers forward-thinking perspectives on human settlements and urban life (Citation2005), and Keva Miller and Michael Phillips critically evaluated the available research linking urban stress and mental health (Citation2005).
Cognitive psychology is frequently explored by professionals in fields such as education, business, and design, and has played a significant role in human–computer interaction (HCI) research by offering psychological principles that can be applied to develop models for understanding and predicting human performance (Green, Davies, and Gilmore Citation1996). Cognitive psychology shares similarities with design in its approach to problem-solving, as both disciplines are goal-oriented and focused on addressing issues from the outset. In the urban context, the term ‘cognition’ is defined as the mental processes that take place in the brain, including thinking, attention, language, learning, memory and perception. These processes are not discrete abilities, rather they are a raft of different, interacting skills which together allow us to function (Clarkson Citation2021).
The basis of the pattern language and structure of nature proposed by Alexander has informed zoning, diversity, land-use and building typology that institutes a new coding regime. And furthermore, the complex structure of the human life, in the form of settlement patterns, economic processes, social patterns, interaction with complex ecosystems, and sustainable development that collectively can be understood as urban morphogenesis.
However, this architecture and urban coding system has experienced only limited development in the built environment since the 1970s, whilst cognitive psychology and HCI have undergone significant advancements in neuroscience and computer science research. These disciplines serve as analytical tools for processing raw data typically associated with information technology, such as software programming, websites, and applications. While a search for ‘code’ or ‘coding’ mainly brings up results related to computing, the concept of coding extends far beyond that exclusive focus. In a broader context, coding simply refers to assigning codes for classification purposes, and codes can be found in various domains, including science, nature, genetics, literature, art, security, human language, fashion, social interaction, law, public policy, urban science and technology.
Stefanidi et al. (Citation2023) categorized and grouped HCI subfields into 12 topics, which include: 1) the design and user experience, 2) HCI research, 3) interaction design for children, 4) artificial intelligence and machine learning, 5) games and play, 6) work and creativity, 7) accessibility, 8) well-being and health, 9) human-robot interaction, 10) automotive user interface, 11) specific application areas, and 12) specific modalities.
Within the realm of computing, the term ‘code’ pertains to a collection of instructions written in a specific programming language. While coders have the ability to introduce novel and previously undefined pieces of code, they must adhere to the guidelines of the language and frequently incorporate sequences of code from established sources that have proven to fulfil specific functions.
Based on a stochastic compilation of DOI-assigned publications sourced from the Semantic Scholar database, ensuring a temporal span from 1890 to 2023, a comparison of the chronological development flow among three distinct domains of HCI can be delineated from . Each domain’s assemblage encompasses approximately 250 publications. It reveals that the domain of ‘human centricity’ only attained prominent recognition in the early 2010s and peaked in 2020. In contrast, the domain of ‘computer science’ has been extensively researched since the 1970s, whilst research pursuits pertaining to the domain of ‘urban psychology’ has only begun increasing since the 1990s. An Excel document encompassing the aforementioned publications has been appended with this paper for readers’ further references.
Figure 1. The chronological development flows compare HCI in computer science and HCI in human centre design. Source: Drawn by the authors, 2023.
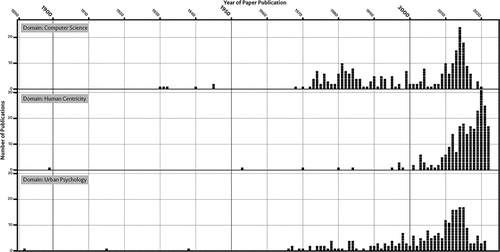
Similarly, urban development adheres to identifiable languages, which are most evident in the varying architectural forms and styles that evolve across different locations and time periods. Until the twentieth century, these architectural elements primarily shaped street-focused urbanism. When transferred from architecture to the broader and intricate realm of urban design, these elements can be regarded as code snippets. While they may include novel and inventive components, they are predominantly composed of well-established codes already utilized elsewhere.
Zhou and Yu (Citation2023) conducted a comprehensive analysis of the existing literature on the design of AI-based technologies. From this analysis, they derived design implications and integrated them into a human-centred design framework for AI technologies, as illustrated in . This framework aims to prioritize human needs and address their concerns effectively by integrating four key dimensions comprehensively into consideration: Machine Learning (ML), Stakeholders, Context, and User Experience (UX) Values. Each dimension encompasses various components and characteristics that have a significant impact on user experiences. This framework has revealed important design considerations and research directions that were previously overlooked and are rapidly gaining research attention, particularly in relation to fostering emotional interaction as a form of emotional engineering, and understanding users’ responses for the Human Centred Design of AI-based technologies to increase user satisfaction (Poteko and Doupona Citation2023).
Figure 2. The generic human centred UX design framework for AI technologies (with permission of Zhou and Yu Citation2023).
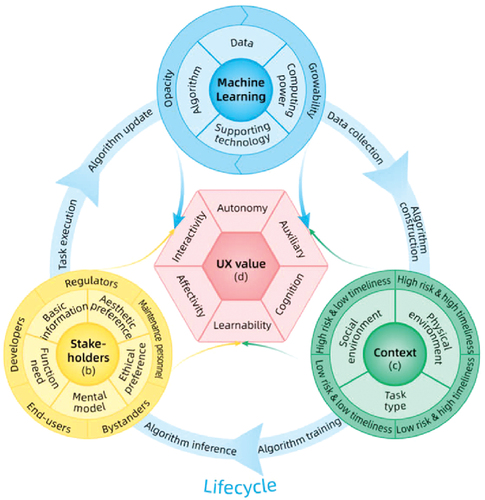
This form of architecture and parameters of urban design can be categorized with three primary aspects.
Shaping – The shaping process involves selecting various urban design elements, such as building styles, block types, materials, and public space features, and ensuring that they come together cohesively. This creates a coherent relationship between the components themselves and the overall development.
Positioning – Urban design elements should be positioned strategically in relation to one another based on specific parameters that establish suitable and desirable relationships. Examples include street walls that effectively define and enclose spaces while still accommodating sustainable movement, as well as the incorporation of street trees and spaces for social activities.
Supporting – Supporting the development of a unified and cohesive entity that surpasses individual components by fostering a nurturing process over time. This approach ensures that new places are not fragmented, disjointed, or constructed in a piecemeal manner. Instead, they are integrated and exhibit a sense of ‘wholeness’ at every stage of their evolution, as famously described by Christopher Alexander et al. (Citation1977).
Christopher Alexander et al. (Citation1977) expressed the idea that our built environment and architecture are a nurturing environment that can emerge through the common understanding of patterns and the development of pattern languages (‘a genetic code’ to best describe their core structural properties or requirements that make the world more liveable). Observation of built environments helps identify patterns that have particular impacts on the wellbeing of people. In this regard, codes play a vital role by empowering living structure to represent these communities as a coherent and holistic environment. By providing a comprehensive and strong framework, urban design codes enable proactive and constructive shaping of urban areas, focusing on specific locations.
A wireframe of this nature possesses the potential to encompass elements that align with William Whyte’s depiction of ‘a social fabric of stifling monotony (Citation1958)’, as well as elements that contribute to a sustainable, inclusive, and enriching approach to urbanism that centres on place. However, rather than focusing on broader urbanistic considerations, there has been a tendency to associate coding with the neo-traditional outcomes of the New Urbanist movement in the United States and developments such as Poundbury in the UK (Carmona Citation2023). Consequently, codes have often been linked to the stylistic preferences commonly associated with such projects. However, just as the choice of a programming language in computer programming does not dictate the nature of the project being coded, in the built environment it is not solely the tool that shapes the outcomes. It is instead the content – the individual components and parameters – that are inputted into the code.
The potential of codes to facilitate site or area-wide strategies extends beyond mere aesthetic considerations. Their purpose lies in coordinating elements such as connectivity, movement, green spaces, biodiversity, the quality of public spaces, distribution of land use, diversity in building forms and types, and promoting walkability, among others. It is crucial to recognize that codes are products of design and, like any strategic planning framework or architectural project they are associated with, they will inherently carry values.
Similar to how programming languages are characterized by syntax (form) and semantics (meaning), codes within the built environment can also be understood as consisting of two elements that correspond to form and meaning. Firstly, there are various ‘components’ that collectively form the physical elements that define a specific place. These components are accompanied by ‘parameters’ which serve as instructions to ensure that the essential connections between the components are correct, thereby creating coherence and an appropriate adaptability to local conditions.
The utilization of parameters concerning both form and meaning in the framework is crucial, as it can prevent unfavourable urbanistic outcomes. For instance, the presence of blank facades within the interior public realm at the third floor may lead to negative effects. However, with the appropriate adjustment of parameters associated with its intended use, the impact can be improved. One potential solution could involve incorporating active uses such as wrapping big box formats, increasing the visual complexity and coherence of the facade with the surrounding context, enhancing openness to increase user engagement, or integrating parking facilities that match the intended use of bland facades.
The rationale behind the growing utilization of ‘coding’ as a deliberate process of code generation and a specific subset of urban design governance practices stems from the belief that the intelligent and careful pre-determination and subsequent application of appropriate codes in specific locations can enhance the likelihood of achieving better design outcomes. However, according to Dovey (Citation2016), different individuals may have contrasting perspectives on what is considered right or perceived as an atrocity. This highlights that codes, which stem from societal influences, necessitate agreement and deliberation. The process of developing codes involves conflict and negotiation. Put simply, codes are political entities, shaped and impacted by human viewpoints, thus making them susceptible to scrutiny and questioning.
Based on this approach, statistical analysis and multimodal studies are conducted for each step using two distinct processes: from electroencephalogram (EEG) signal to AI generation based on elements, colours, and urban morphology. Since the early 1990s, cognitive neuroscientists have utilized methods including electroencephalogram (EEG) to explore the cognitive dimensions of brain activity by observing variations in cerebral blood oxygenation. Employing EEG technology holds great promise for deepening our comprehension of how individuals mentally and emotionally react to intentionally created environments. Expanding on this motion, the primary goal of this study is to establish a scientific research methodology that integrates cognitive measurement and modelling into the examination of urban structures and systems, specifically focusing on the human experience.
This analysis can provide insights into what could be the most appropriate design solution in relation to users’ emotional responses to the conditions of the built environment. For instance, if a certain passageway is observed to frequently accommodate a large crowd, it may be necessary to widen its width accordingly. Additionally, areas where people often feel gloomy, unpleasant, or frustrated should be reassessed in terms of layout to increase usage and enhance the overall spatial quality. In certain scenarios, the formulation of hyper-morphology can serve as a guide or inspiration for space design. These findings can be useful for form-finding in architectural design and optimizing routes in urban design to enhance urban mobility, cost efficiency of construction, and overall urban user experience.
These research methodologies offer a framework for assessing the vertical public realm and spaces by considering emotional responses to them. They can be utilized during the design process to monitor and manage the quality of the vertical and volumetric built environment by digitally modelling the spatial conditions of the built environment and utilizing EEG to measure the emotional responses to this spatial model whilst viewing it on screen or via VR goggles. The EEG data can then be used by an AI generator to categorize emotional and spatial cognition.
Research method
To conduct the EEG testing, this research designed various scenarios and image scenes that simulated different conditions and parameters within built environments (). This allowed for the investigation of the relationship between spatial cognition and six distinct emotions: ‘Amazement’, ‘Joy’, ‘Amusement’, ‘Unpleasantness’, ‘Frustration’, and ‘Gloom’. The reasons for selecting the six emotions are expounded as follows: As stated in one precursory paper, the well-established arousal – valence model originally proposed by Russell (Citation1979) was employed, which is the most common method for human emotion categorization (Choi et al. Citation2023). In this model, emotions are categorized into four quadrants. While the x-axis denotes the level of arousal or the intensity of the emotion, the y-axis indicates the valance of emotions, or the degree of positivity and negativity of the emotion. In detail, emotions of high arousal and high valence include ‘Amazement’, ‘Amusement’ and ‘Joy’; emotions of high arousal and low valence include ‘Fear’, ‘Anger’ and ‘Distraction’; emotions of low arousal and high valence include ‘Content’, ‘Relaxedness’ and ‘Calm’; and emotions of low arousal and low valence include ‘Frustration’, ‘Gloom’, ‘Boredom’ and ‘Sleepiness’. Among them, emotions of low arousal and high valence were excluded from further investigation because they generally imply people’s neutrality towards the quality of the built environment, whereas emotions exhibiting strong positivity or negativity, such as ‘Amazement’ and ‘Frustration’ can clearly highlight the quality of the built environment. Therefore, ‘Amazement’, ‘Joy’, ‘Amusement’, ‘Unpleasantness’, ‘Frustration’, and ‘Gloom’ were selected from the remaining three quadrants of emotions as the optimal representation of strong emotional states triggered by the built environment. Supplementarily, the selection process was also influenced by the types of brain waves associated with each emotion, which will be explained in the third section.
Figure 3. Correlation analysis between spatial cognition and six emotions using pretrained AI and generative AI (source: Authors 2023).
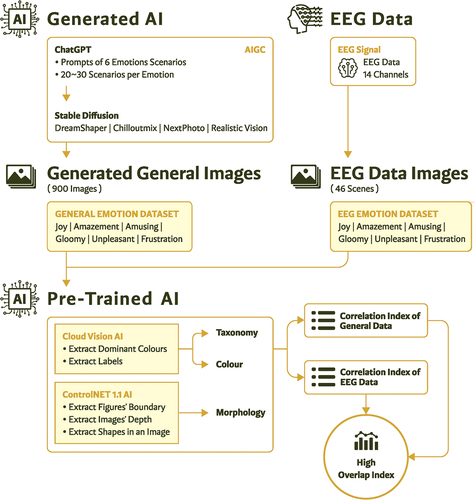
Preparation of 3D modelling, using 9 parameters and 4 variations
Virtual reality simulations and models enable us to analyse task performance and social interactions among users within the experimental parameters. Several studies have been conducted to examine the emotional patterns specific to each location.
The massing of the surrounding building typology with detailed environmental capital that contain varying ranges of sun, light and darkness, density of usage, walkability, accessibility, permeability, greenery, and sounds.
The multi-layered interior pedestrian circulation networks that are directly and indirectly accessible to the public with different ranges and user densities.
The proximity of amenities for Economic capital and catchments at different layers and levels.
Social capital, interaction, and cultural aesthetics.
Based on the existing studies and researches, the understanding of context can evolve, as urban design concepts may themselves evolve over time. In vertical and compact cities, context can also be considered as more complicated and multi-layered than in a less vertical more widely spread urban context. As noted by a number of theorists including Cullen (Citation1968), Lynch et al. (Citation1970), Gehl and Koch (Citation1987), Whyte (Citation2009), urban context normally pertains to surroundings such as streets, blocks, and neighbourhoods. In a vertical and compact city, context becomes three-dimensional, covering the skyline, the changing perspective at various heights and the interactions between different volumes. On the other hand, traditionally, social context relates to ground-floor activities, while volumetrically, social context can expand vertically. The notion of ‘ground’ can be reconceptualized at multiple levels, where vertical spaces can have many squares and plazas reaching skywards. The perception of context from this lens in three-dimensional urban interiors can enhance users’ livelihoods and social activities (Choi et al. Citation2023). Based on this argument, this research further developed its parameters to evaluate users’ emotional responses in different environmental conditions.
As shown in , the 3D simulation was set up with nine parameters and four variations. The parameters are social and spatial factors, including greenery, accessibility, walkability, permeability, diversity, economic catchment, safety, light, and cultural identity/aesthetics.
For each parameter, there are four variations as indicators of the intensity or visual coverage of that parameter. The variation starts from the minimum level of 20%, rising in 20% increments of intensity until reaching the maximum level of 80%. The intensity of the nine parameters was varied in the following ways, as listed in :
For greenery, each increase in variation level denotes an increase in the quantity, size and type of plants in the given space. At 20%, there are a few green pots in the space; at 40%, some grasses and herbs can be added to the ground; at 60%, shrubs and colourful flowers are added; and at 80%, climbing plants and trees are added.
For accessibility, each increase in variation level denotes a higher level of physical legibility for people to easily understand and navigate through the given space. At 20%, tactile paving is added; at 40%, entrance/exit signages are installed; at 60%, floor information signages and wayfinding kiosks are installed; and at 80%, a reception desk or information counter is added.
For walkability, each increase in variation level denotes a greater extent to which people can move around in the given space, including vertical accessibility and horizontal accessibility, which means the frequency in accessibility to doors and lifts. At 20%, walls are placed to block people’s path and line of sight, leaving only one or two directions for passage; at 40%, the walls are reduced, exposing more doors, escalators and lifts for people to pass through; at 60%, the walls are further reduced, creating more open space for people to walk; and at 80%, the space is left open, removing all non-essential physical obstacles blocking people’s paths.
For permeability, each increase in variation level denotes higher visibility, and greater openness of the given space. At 20%, retail facilities are densely arranged on both sides of the corridor, leaving a very narrow space for circulation in between; at 40%, the corridor is widened to increase circulation space; at 60%, the corridor is further widened, and the central void is increased; and at 80%, a large open space is introduced with minimal visual obstructions.
For diversity, each increase in variation level denotes enhanced richness of potential experiences in the given space. This includes a greater variety and number of facilities or functions that cater to different age groups and social identities, such as spaces specifically designed for children, women, the elderly and minority groups. These facilities and amenities include exhibitions, sports areas, pet zones and art installations. At 20%, 40%, 60%, and 80%, different amenities and spaces for different groups are increasingly introduced.
For economic catchment, each increase in variation level denotes a higher appearance of facilities that promote personal consumption in the given space, including restaurants, shops, small vendors, event spaces, clinics, gyms, kids’ play areas and cinemas. At 20%, 40%, 60%, and 80%, different spaces of consumption are increasingly introduced, with simulations of more luxurious and larger venues emerging at or after 60%.
For safety, each increase in variation level denotes a higher attention to the removal of physical hazards in the given space, involving passive prevention of slips, trips and falls. At 20%, the floor is wet and slippery, and the coating is overly glossy and causes occasional glares. The humps on the floor also pose additional risk; further, the handrail is absent on the staircase; at 40%, the floor is dry but remains slippery with humps, and handrail is still absent on the staircase; at 60%, the floor is dry but remains slippery with humps, but handrails have been installed at the staircase; and at 80%, the floor is now even and has been re-paved with anti-skid materials; Additionally, fences and extra handrails are installed where necessary.
For light, each increase in variation level denotes a rise in indoor light levels in the given space, subject to variations in both artificial and natural lighting. At 20%, natural lighting is low, resembling dawn, while artificial lighting is turned off; at 40%, natural lighting is similar to an overcast morning, with some partial artificial lighting; at 60%, natural lighting is close to full daylight and artificial lighting is partially turned on with some increases from the previous 40%; and at 80%, the interior space is fully illuminated with direct sunlight, and all artificial lights are turned on.
For cultural identity/aesthetics, each increase in variation level denotes a more comprehensive incorporation of elements that reflect the local culture, aesthetics and traditions in the given space. They can help resonate with people by evoking distinctive cultural traits and identity, thus enhancing the belonging of specific user groups by visual attractiveness and familiarity by their background experiences and life. At 20%, 40%, 60%, and 80%, different cultural elements are added. To mitigate subjective bias in choosing culture elements, the study carefully considers factors such as National Symbols, Cultural Values, Language, Artistic Traditions, and Religion of the study region. The integration of cultural elements at each level aims to enrich the visual scope of the scenes, ensuring that the collective influence of all cultural components creates a harmoniously enhanced experience.
To capture a broader spectrum of human emotional responses, some simulation scenes are intentionally designed to be more extreme than typical real-life situations. For instance, safety features like slippage and fall protection are omitted in scenarios with 20% and 40% Safety ratings. Likewise, accessing a public commercial space during closed hours, with lights off and shops shuttered (referenced as Light 20%), presents an atypical experience. Though not common, such extreme scenarios are plausible in real life. Exploring these conditions helps determine how unusual scenes might elicit positive emotions such as surprise or negative feelings like fear or displeasure. Additionally, the study incorporates both temporary elements (e.g., signages, indoor plants, trolleys) to modify parameters like Diversity, Accessibility, Economic Catchment, and Culture, and more permanent features to enhance Greenery, Walkability, Safety, Permeability, and Lighting, aiming to understand their impact on emotional responses.
Table 1. Detailed explanation of the criteria of variations of different parameters (source: Authors 2023).
Based on these four variations in intensity to nine parameters a series of 3D simulations were modelled using the Rhinoceros 3D modelling tool and rendered through D5 Render, an interactive and photorealistic 3D renderer. In Rhinoceros, nine parent layers are created for each parameter, and within each parent layer, there are four sublayers with each holding the items/elements/modifications of one of the variations of its parent parameter. The model is imported to D5 Render, where textures and lighting are applied, and animated human models are incorporated into the scene. This user group is selected to reflect the demographic and mix of ethnicities of the area.
Human understanding of space includes physical properties, but is also shaped by our movement and experiences (Dix et al. Citation2022). In this research, the static camera position in the simulation video allows participants to grasp the fundamental characteristics of a space in a brief period. Prior to the formal EEG experiment, render outputs as video clips of 3, 5, and 8 seconds, each featuring a distinct variation, were pre-tested. Using an EEG headset to monitor cognitive responses, it was determined that a 5-second duration per variation optimally balances giving participants enough time to recognize and process the content cognitively without the distraction of boredom from prolonged exposure to unchanging scenes. This approach enables the efficient collection of data, with participants’ brain activity data from the EEG subjected to time-frequency analysis. This data is then categorized into different emotions on a moment-by-moment basis (Choi et al. Citation2023). The study aims to establish a ‘correlation index’ linking spatial characteristics with the emotions they evoke. Specifically, this index examines morphology, colour and taxonomy of space, further quantifying emotional responses in relation to specific conditions within vertical and volumetric environments using 20 key indicators. Ultimately the ‘correlation index’ served as an ‘assessment tool’ for designers and managers to monitor users’ emotions and assist in predicting their spatial and emotional patterns.
The case study for this research was CapitaSpring in Singapore, a 51-story, 250 m tall building with an area of 93,000 sqm. A total of 40 participants were involved in the testing process following a five-step procedure:
Participants were asked to relax in a comfortable chair, as shown in ;
Between 16 and 25 electrodes were then attached to the scalp with a special paste, or a cap containing the electrodes;
Participants were asked to relax and be still;
The recording might be stopped periodically to allow for rest and repositioning;
This study was carried out by an experienced EEG technician, with 10 mins preparation and 20 mins test.
The EEG data from the study was then collected and analysed to establish a correlation index. This index was then used to develop a coding system to evaluate general emotional responses, encompassing both positive and negative feelings to the four variations of nine parameters defined above. The interpretation of EEG data and its correlation with AI generators’ spatial cognition formula served as the coding system that suggested a common framework applicable to a wide range of international cases. The applicability of this approach for developers and designers to define a more coherent urban design structure, while still allowing for diverse and distinct interpretations, has been emphasized by leading urban designer and director of the Collaborative City Marcus Wilshire.
The EEG test results included distinct spikes in the participants’ cognitive activity. highlights how participants’ sensory and spatial cognition was strongly aroused when cultural aesthetics, diversity, accessibility, and light reached a certain level. By compiling the analysis of EEG data from multiple participants, it could be preliminarily determined which parameters are more likely to elicit a cognitive response. Details on how the EEG data was interpreted are discussed in the following section.
The result identifies emotions in relation to the spatial condition that has influenced and been impacted by participants’ immediate emotional response in real-time, but not influenced by social and cultural backgrounds, However, this research scope does not consider the influence of different social and cultural backgrounds, which can be discovered by an fMRI test. In addition, the survey did not consider the role of memory or language in the measurement of response; this would have required a different form of cognitive study.
Data analysis process
As shown in , the EEG data is initially recorded via electrodes attached to participants’ scalp to capture the electrical activities of their brain. Subsequently, the electrical activities are processed and interpreted into five distinct types of brain waves, each characterized by different brain states. Specifically, the Gamma wave indicates problem-solving and concentration, the Beta wave indicates anxiety, external attention and a relaxed state, the Alpha wave indicates reflective and passive attention during a restful state; the Theta wave indicates inward-focused drowsiness, and finally, the Delta wave indicates sleepiness and dreaming (Abhang, Gawali, and Mehrotra Citation2016). Since this study requires participants to stay sober and active, the Delta wave was therefore excluded from the analysis. Additionally, specific emotions are associated with these brain waves based on the location of the electrodes. Lastly, time stamps of emotion elicitation are synchronized with those of the 3D simulation scenes, establishing the foundation for determining the correlation index between emotions and spatial qualities.
Without AI the EEG data analysis is a labour-intensive and inefficient process, with researchers required to visually inspect colour noise images representing the brain wave data, and manually identify and select the orange and reddish patches that signifies heightened brain activity. However, with the assistance from ML-based image recognition, this process can be significantly expedited. In this approach, key features are identified and extracted from the EEG data and be input into a ML model. For this study, pixels with colour wavelengths between 565 and 740 nanometres (yellow and red) are extracted from the input EEG images. This pixel-based recognition technique, which recognizes the red, green, and blue (RGB) values, has been widely established for computer vision applications (Zhong et al. Citation2021). Then, the ML model categorizes these pixels into distinct groups, allowing for further extraction and analysis of additional image sets. Due to time constraints, the study utilized a pre-trained model for this study. This series of ML-aided processes serves as an exemplary demonstration of how the integration of AI in urban design and research can enhance overall efficiency and objectivity. encapsulates the ML process in a flow chart.
The outcome of the evaluation tool through these series of research methodologies provides a feasible and operational evaluator for designers to evaluate the vertical public realm and spaces following based on emotional responses to them, both during the design stages and as a means to monitor and manage the quality of the vertical and volumetric built environment in use. below describes how the ‘Correlation index’ is built up via the data analysis from EEG as a final index that includes overlapping values for the taxonomy of urban elements, morphology and colour.
Findings
From the findings, human-centred design enhancement can be achieved by applying codes to a particular location through the creation of a masterplan or specific architectural, landscape, or infrastructure interventions that align with the established code. In the context of codes, there are four key terms that contribute to our comprehension: ‘three-dimensional’, ‘illustrated’, ‘directive’, and ‘precise’. Importantly, when these characteristics are taken collectively within the code, they indicate a type of guidance that is likely to have a more substantial influence.
In detail, the findings of the study highlight the most influential spatial and environmental conditions emotionally as ‘walls’, ‘ceiling’, ‘flooring’ and ‘user density’, especially in relation to greenery, walkability, accessibility (both vertical and horizontal) and lighting. The availability of a ‘sky view’, information or help centres, seating and furniture were measured and proved to be of more minor significance. From these findings, summarizes 10 key design elements to enhance the quality of the vertical and volumetric built environment.
Table 2. Findings of emotional connections of spatial and environmental conditions within multi-layered interior public realms and public spaces (source: Authors 2023).
First, interior public space at lower level and within the lower level of commercial space indicate that a moderate percentage of greenery between 20% and 40% creates a higher emotional response and cognitive reaction.
Second, an increase in the frequency of elevators and pathway options that increase the opportunity of walkability and transfer to different levels results in increased positive spatial cognition.
Third, and importantly, walkability levels between 40% and 60% at commercial and public transportation entranceways increase positive cognition, and more than 60% of walkability within the commercial area from lower level to middle level create position cognition. Conversely, an increased diversity in public open space and lobby areas create negative emotions.
Fourth, below 20% of permeability at lower floor levels create excitement and an increased cognition.
Fifth, options for vertically and horizontally linked walkable and accessible areas of increased width create negative cognition. Less wide and clearly legible access routes with a clear signage and wayfinding system increase positive cognition.
Sixth, an increased level of spatial permeability and legibility result in a stronger spatial cognition
Seventh, public open spaces that include increased brightness levels and shaded areas create a higher cognitive response and reaction. Interestingly, contrasting dark and light colour combinations for in-between transitional spaces such as escalators, stairs, lift cars and bridge produce a positive cognition.
Eighth, increased greenery within routes for vertical accessibility create excitement and increased cognition.
Ninth, within routes and spaces for vertical accessibility including escalators and lifts, moderate sounds and greenery, and natural lighting (below 40%) increase spatial cognition.
Tenth and lastly, images with a cultural reference and aesthetic produce a response in both sensory processing and spatial cognition.
In summary, the study evaluated the multi-layered interior public realms and public spaces, and explored the emotional connections and responses associated with them. By using various parameters and their variations, the assessment demonstrated the potential for a comprehensive tool to measure users’ emotional responses to different spatial conditions. These findings were based on EEG testing of cognitive responses to four variations across nine parameters: Greenery, Accessibility, Walkability, Permeability, Diversity, Economic Catchment, Safety, Light, and Cultural Identity/Aesthetics. This emphasized the importance of a spatial network that prioritizes walkability and visibility, easy accessibility from street level to the interior public realm, and the use of navigation systems to enhance walkability and permeability. It also highlighted the significance of vertical permeability, including the quality of visual and physical connections between floors to enhance walkability and accessibility. Additionally, cultural aesthetics and economic catchment were identified as key parameters for diversity and richness of spatial cognition, along with the importance of natural light and greenery at elevated levels.
Considering this, the application of assessment frameworks based on neuroscientific research data and analysis, combined with artificial intelligence, has proven to be effective not only in measuring the key performance indicators (KPIs) of a project but also in the wider domain of human-centred design (HCD).
Conclusion
As urban life becomes increasingly intertwined with these high-rise buildings, the ways in which we live in and around them have continuously evolved. However, in the academic realm, studies on high-rises primarily focus on technical aspects. Many studies explore the construction, maintenance, and sustainability of high-rise buildings. The main subject of study has been the individual high-rise building itself, while the investigation of how people experience and inhabit these towers has been lacking. Gifford (Citation2007) highlighted the limited interest and lack of research on the consequences of living in high-rise buildings, with little new research on this topic since the 1990s. Only recently has there been a renewed focus on vertical space within the urban landscape. Scholars increasingly criticize the predominant emphasis on horizontal analysis and advocate for a consideration of the often-neglected verticality (Graham Citation2016; Graham and Hewitt Citation2012; Harris Citation2014).
Expanding upon the recent studies regarding ‘vertical urbanism’, this scoping study aims to provide a comprehensive evaluation of research on ‘high-rise living’. The focus lies on examining the ‘living experiences’ of residents and the social phenomena that have emerged within this changing urban environment. By reviewing existing literature on this subject, the paper seeks to elucidate the existing gaps in knowledge and shed light on what has and has not been studied in this particular field.
The term ‘vertical’ introduces a new research direction that examines the lives of people along a different axis, considering both upward and downward extensions of built space (Graham and Hewitt Citation2012). Verticality, vertical urbanism, and volumetric urbanism highlight the increased depth and height in urban environments, inviting exploration of new perspectives on city life and new dimensions of segregation and division. While previous studies on high-rises focused on planning, economic analysis, architectural analysis, or sustainability, the emphasis on vertical urbanism adopts a ‘neuroscientific’ approach to understand residents’ cognitive and emotional engagement in high-rise living environments. This approach also addresses debates within urban studies regarding new scientific research methods, particularly concerning urban verticality.
Considering that, the statement that describes codes as a broad term could be expanded on encompassing various tools that establish design parameters to enhance the direction of development (Carmona Citation2018). In this context, the code can be viewed as a reference point in art; establishing the structural framework for shaping three-dimensional forms with flexible materials, and becoming foundation for the proposed urban environment. This involves understanding how to utilize and manipulate variables, data structures, and the essential elements of urban environment that enhance its sense of place.
In conclusion, this research establishes a systematic and statistical method to evaluate the quality of living environment in vertical and volumetric urbanism for a human-centred design (HCD) approach. It provides a measurement of neural mechanisms to support scientific research methods for urban design with cognitive neuroscientists, using the multi-level public and quasi-public space within both commercial and residential living complexes in Singapore as a testing ground towards the design of vertical and volumetric urbanism. Moreover, this research emphasizes the significance of human-computer interaction (HCI), specifically highlighting the role of ‘artificial intelligence and machine learning’ as tools for both AI-enabled urban design and research. The integration of AI and ML can aid in the development of evaluation systems and design principles to enhance a human-centred design approach in urban design practices. By incorporating these tools and principles, crucial design considerations and research directions have emerged, particularly concerning emotional interactions and understanding user responses.
Disclosure statement
No potential conflict of interest was reported by the authors.
Additional information
Funding
References
- Abhang, P. A., B. W. Gawali, and S. C. Mehrotra. 2016. “Technological Basics of EEG Recording and Operation of Apparatus.” Introduction to EEG- and Speech-Based Emotion Recognition 19–50. https://doi.org/10.1016/b978-0-12-804490-2.00002-6.
- Alexander, C., S. Ishikawa, and M. Silverstein. 1977. A Pattern Language: Towns, Buildings, Construction. New York: Oxford University Press.
- Carmona, M. 2018. “Principles for Public Space Design, Planning to Do Better.” Urban Design International 24 (1): 47–59. https://doi.org/10.1057/s41289-018-0070-3.
- Carmona, M. 2023. “Coding Urban Design: Constructing a Wireframe for a Place-Focused Urbanism.” Progress in Planning 176:100775. https://doi.org/10.1016/j.progress.2023.100775.
- Choi, H. S., G. Bruyns, W. Zhang, T. Cheng, and S. Sharma. 2023. “Spatial Cognition and Three-Dimensional Vertical Urban Design Guidelines—Cognitive Measurement and Modelling for Human Centre Design.” Urban Science 7 (4): 125. https://doi.org/10.3390/urbansci7040125.
- Clarkson, J. 2021. “What is Cognition?” Dementias Platform UK. Accessed May 10. https://www.dementiasplatform.uk/news-and-media/blog/what-is-cognition.
- Cullen, G. 1968. Townscape. London: Architectural Press.
- Dix, A., S. Gill, J. Hare, and D. Ramduny-Ellis. 2022. Comprehension of Space. OUP Academic. August 11. https://doi.org/10.1093/oso/9780198718581.003.0012.
- Dovey, K. 2016. Urban Design Thinking: A Conceptual Toolkit. London: Bloomsbury.
- Gehl, J., and J. Koch. 1987. Life Between Buildings: Using Public Space. Washington: Island Press.
- Gifford, R. 2007. “The Consequences of Living in High-Rise Buildings.” Architectural Science Review 50 (1): 2–17. https://doi.org/10.3763/asre.2007.5002.
- Graham, S. 2016. “Vanity and Violence.” City 20 (5): 755–771. https://doi.org/10.1080/13604813.2016.1224503.
- Graham, S., and L. Hewitt. 2012. “Getting off the Ground.” Progress in Human Geography 37 (1): 72–92. https://doi.org/10.1177/0309132512443147.
- Green, T. R. G., S. P. Davies, and D. J. Gilmore. 1996. “Delivering Cognitive Psychology to HCI: The Problems of Common Language and of Knowledge Transfer.” Interacting with Computers 8 (1): 89–111. https://doi.org/10.1016/0953-5438(95)01020-3.
- Harris, A. 2014. “Vertical Urbanisms.” Progress in Human Geography 39 (5): 601–620. https://doi.org/10.1177/0309132514554323.
- Lynch, K., B. T. Editors, and S. Michael. 1970. City Sense and City Design: Writings and Projects of Kevin Lynch. MIT Press. https://books.google.com/books?id=6LUhsu6Ap1EC.
- Milgram, S. 1977. “Subject Reaction: The Neglected Factor in the Ethics of Experimentation.” The Hastings Center Report 7 (5): 19. https://doi.org/10.2307/3560719.
- Miller, K. M., and M. H. Phillips. 2005. “Correlates of Urban Stress and Mental Health: The Impact of Social Context on Resilience.” Journal of Social Distress and Homelessness 14 (1–2): 46–64. https://doi.org/10.1179/105307805807066301.
- Neisser, U. 1967. Cognitive Psychology. New York: Appleton-Century-Crofts.
- Poteko, K., and M. Doupona. 2023. “Walking the City – A Case Study on the Emancipatory Aspects of Walking.” Sport in Society 26 (9): 1585–1601. https://doi.org/10.1080/17430437.2023.2173067.
- Russell, J. A. 1979. “Affective Space Is Bipolar.” Journal of Personality and Social Psychology 37 (3): 345–356. https://doi.org/10.1037/0022-3514.37.3.345.
- Stefanidi, E., M. Bentvelzen, P. W. Woźniak, T. Kosch, M. P. Woźniak, T. Mildner, S. Schneegass, H. Müller, and J. Niess. 2023. “Literature Reviews in HCI: A Review of Reviews.” In Proceedings of the 2023 CHI Conference on Human Factors in Computing Systems. https://doi.org/10.1145/3544548.3581332.
- Walker, P. A. 2005. “Political Ecology: Where is the Ecology?” Progress in Human Geography 29 (1): 73–82. https://doi.org/10.1191/0309132505ph530pr.
- Whyte, W. H. 1958. The Exploding Metropolis. Garden City, NY: Doubleday.
- Whyte, W. H. 2009. City: Rediscovering the Centre. Philadelphia: University of Pennsylvania Press.
- Zhong, C., X. Bai, B. Dong, and H. Li. 2021. “Image Extraction Based on Machine Learning and Image Recognition and Analysis Technology.” Journal of Physics: Conference Series 2037 (1): 012115. https://doi.org/10.1088/1742-6596/2037/1/012115.
- Zhou, Z., and J. Yu. April 23, 2023. “Adapt a Generic Human-Centered AI Design Framework in Children’s Context.” In CHI 2023 Workshop on Child-centred AI Design: Definition, Operation and Considerations. Hamburg, Germany. New York, NY: ACM. ArXiv Abs/2304.01232.